Abstract
Free full text

Neuroinflammation triggered by β-glucan/dectin-1 signaling enables CNS axon regeneration
Significance
Damage to neuronal networks in the central nervous system typically results in permanent functional deficits; however, the regenerative capacity of injured neurons can be dramatically augmented by local innate immune responses. Here we investigated the molecular and cellular events that participate in immune-mediated repair of severed optic nerve axons in the mouse. We show that intraocular administration of particulate β-glucan engages the immune receptor dectin-1 expressed on retina-resident microglia and infiltrating leukocytes, to trigger enhanced axonal regeneration. Delayed administration of β-glucan by two days is as effective as administration at the time of injury, suggesting a large therapeutic window. These data elucidate a new pathway of immune-mediated neural repair that may be targeted to reverse neurological disability.
Abstract
Innate immunity can facilitate nervous system regeneration, yet the underlying cellular and molecular mechanisms are not well understood. Here we show that intraocular injection of lipopolysaccharide (LPS), a bacterial cell wall component, or the fungal cell wall extract zymosan both lead to rapid and comparable intravitreal accumulation of blood-derived myeloid cells. However, when combined with retro-orbital optic nerve crush injury, lengthy growth of severed retinal ganglion cell (RGC) axons occurs only in zymosan-injected mice, and not in LPS-injected mice. In mice deficient for the pattern recognition receptor dectin-1 but not Toll-like receptor-2 (TLR2), zymosan-mediated RGC regeneration is greatly reduced. The combined loss of dectin-1 and TLR2 completely blocks the proregenerative effects of zymosan. In the retina, dectin-1 is expressed by microglia and dendritic cells, but not by RGCs. Dectin-1 is also present on blood-derived myeloid cells that accumulate in the vitreous. Intraocular injection of the dectin-1 ligand curdlan [a particulate form of β(1, 3)-glucan] promotes optic nerve regeneration comparable to zymosan in WT mice, but not in dectin-1−/− mice. Particulate β(1, 3)-glucan leads to increased Erk1/2 MAP-kinase signaling and cAMP response element-binding protein (CREB) activation in myeloid cells in vivo. Loss of the dectin-1 downstream effector caspase recruitment domain 9 (CARD9) blocks CREB activation and attenuates the axon-regenerative effects of β(1, 3)-glucan. Studies with dectin-1−/−/WT reciprocal bone marrow chimeric mice revealed a requirement for dectin-1 in both retina-resident immune cells and bone marrow-derived cells for β(1, 3)-glucan–elicited optic nerve regeneration. Collectively, these studies identify a molecular framework of how innate immunity enables repair of injured central nervous system neurons.
Following injury to the adult mammalian central nervous system (CNS), severed axons fail to undergo spontaneous regeneration. The limited and transient growth response of injured CNS neurons is in part responsible for poor clinical outcomes following brain or spinal cord trauma. Neuron intrinsic (1) and extrinsic mechanisms (2) pose barriers to efficient CNS repair; however, there is accumulating evidence that, under certain circumstances, endogenous repair mechanisms can be unleashed by the induction of a local innate immune response (3, 4).
Retro-orbital optic nerve crush (ONC) is a widely used rodent model for investigating factors that influence axonal growth in the injured CNS (5). Normally, retinal ganglion cells (RGCs), the neurons that give rise to the optic nerve, do not extend lengthy axons beyond the injury site; however, robust axonal growth occurs after induction of intraocular inflammation via lens trauma (5) or intraocular (i.o.) injection of zymosan (3, 6), Pam3cys (7), or oxidized galectin-1 (8). This phenomenon is not restricted to the visual system, because injection of zymosan into dorsal root ganglia or spinal cord parenchyma triggers local inflammation and growth of injured or transplanted sensory neurons (9, 10). Macrophages (3, 9), neutrophils (11), and astrocytes (12) have been implicated in the proregenerative effects of inflammation. The benefits of neuroinflammation on axonal growth can be undermined by concurrent toxicity (9). A deeper understanding of these opposing effects will be important for exploiting immunomodulatory pathways to promote neural repair while minimizing bystander damage.
In the present study, we investigated the pathways that drive innate immune-mediated axon regeneration after ONC. We induced sterile inflammation in the vitreous on the day of injury by i.o. administration of zymosan or constituents of zymosan classified as pathogen-associated molecular patterns (PAMPs). PAMPs are highly conserved microbial structures that serve as ligands for pattern recognition receptors (PRRs). PRRs for zymosan are widely expressed on innate immune cells and include Toll-like receptors (TLRs) 1 and 2, complement receptor 3 (CR3), and the C-type lectin family members CLEC7A (dectin-1) and CLEC6A (dectin-2) (13, 14). Engagement of PRRs on myeloid cells, such as monocytes, macrophages, neutrophils, and myeloid dendritic cells (DCs), results in their activation and induces phagocytosis and oxidative burst, as well as cytokine and chemokine production. The mechanism by which PRR signaling confers regenerative properties to myeloid cells is poorly understood. Here we elucidate the PAMP–PRR interactions critical for zymosan-mediated axonal regeneration, and thereby introduce a panel of signaling molecules that may be targeted to promote posttraumatic neurorepair.
Results
Zymosan, But Not Lipopolysaccharide, Enables Immune-Mediated Axon Regeneration.
An i.o. injection of the yeast cell wall extract zymosan into the posterior chamber of the mouse eye triggers a local inflammatory response. Flow cytometry analysis of the cellular composition of vitreous infiltrates at 7 d after zymosan injection and ONC revealed the accumulation of large numbers of monocytes/macrophages (3), neutrophils (11), and DCs. Small numbers of B cells, CD4+ and CD8+ T cells, and natural killer (NK) cells were observed as well (Fig. 1A). We found that i.o. injection of lipopolysaccharide (LPS), a cell wall component of Gram-negative bacteria and selective ligand for TLR4 (15, 16), induced vitreous infiltrates with a similar cellular composition to those induced by zymosan. Moreover, we found no differences in reactive oxygen species (ROS) production of macrophages between eyes injected with LPS and those injected with zymosan (Fig. S1B). Remarkably, i.o. zymosan induced robust regrowth of severed RGC axons (3), whereas i.o. LPS failed to do so (Fig. 1 B–E). Because zymosan and LPS are recognized by different PRRs, this suggests that engagement of specific immune receptors is required to generate an inflammatory milieu conducive for CNS axon regeneration.
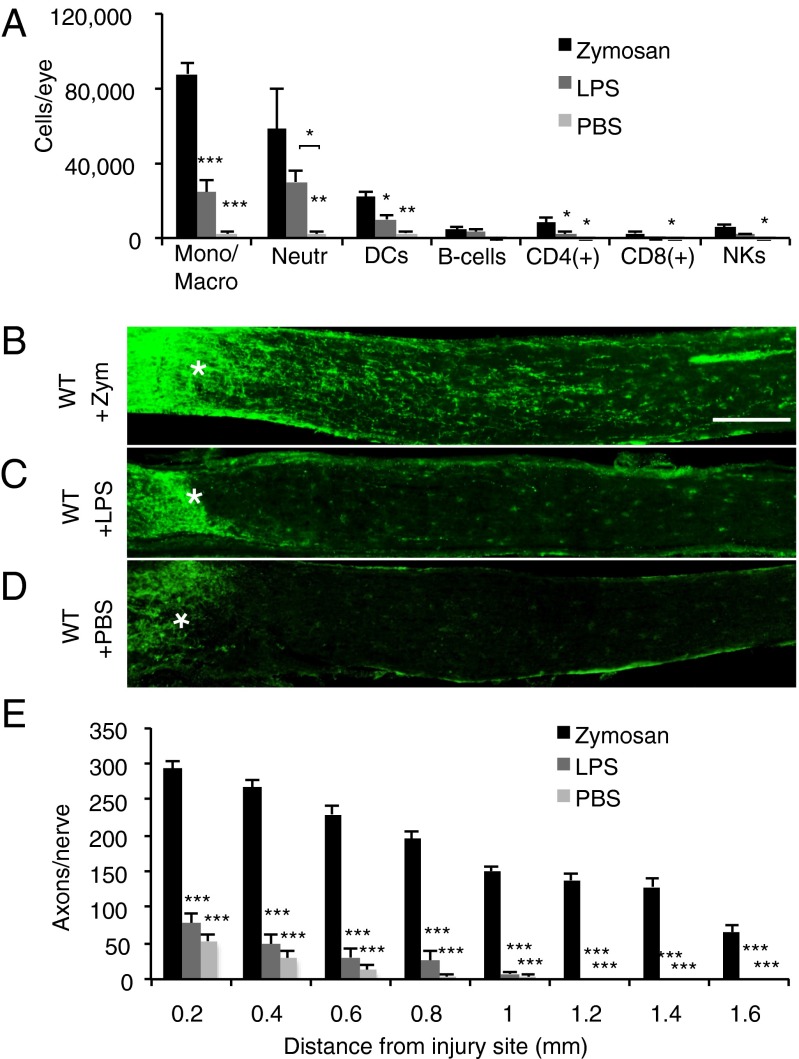
Zymosan, but not LPS, enables immune-mediated axon regeneration. (A) Flow cytometric analysis of immune cells accumulating in the eye of WT mice at 7 d post-ONC and i.o. zymosan (5 µL, 12.5 µg/µL) injection (n = 5 mice), i.o. LPS (3 µL, 5 µg/µL) injection (n = 3 mice), or i.o. PBS (5 μL) injection. (B–D) Longitudinal sections of WT mouse optic nerves at 2 wk after ONC and i.o. injection. Regenerating axons are visualized by anti-GAP43 immunofluorescence labeling. The injury site is marked with an asterisk. (Scale bar: 200 µm.) (B) WT mice with i.o. zymosan (n = 6) show robust axon regeneration. No significant regeneration is observed in WT mice with i.o. LPS (n = 4) (C) or WT mice with i.o. PBS (n = 5) (D). (E) Quantification of the number of GAP43+ axons per nerve at 0.2–1.6 mm distal to the injury site. Asterisks indicate a significant difference from zymosan-induced regeneration. Results are presented as mean ± SEM. ***P < 0.001; **P < 0.01; *P < 0.05, one-way ANOVA, Tukey’s post hoc test.
TLR2 and MyD88 Are Not Necessary for Zymosan-Elicited Axon Regeneration.
Zymosan has been used to induce sterile inflammation in animal models of peritonitis and arthritis. In these experimental paradigms, zymosan stimulates activation of myeloid cells via the TLR2/MyD88 pathway (14, 16, 17). TLR2 signaling also has been implicated in RGC axon regeneration, given that repeated i.o. injections of Pam3Cys, a synthetic agonist of TLR2, promotes axon growth after ONC (7). The importance of the TLR2/MyD88 pathway in zymosan-mediated axonal regeneration has not been explicitly demonstrated, however. Myeloid cells, but not lymphocytes, that infiltrate the eye by 7 d after i.o. zymosan and ONC express TLR2 (Fig. S2 A and B). Both retina-resident DCs and microglia express TLR2 during homeostasis (Fig. S2 C and D). The number of TLR2+ microglia and DCs increases by 4- and 14-fold, respectively, by day 7 post-ONC without i.o. zymosan (Fig. S2 E and F), demonstrating that ONC alone, in the absence of i.o. PAMPs, is sufficient to activate retinal immune cells. Surprisingly, i.o. administration of zymosan depleted of all its TLR2-stimulating properties (“depleted zymosan”) caused robust regeneration of GAP43+ RGC axons (Fig. S2I). The majority of TLR family members signal through the downstream adaptor MyD88; however, similar to TLR2−/− mice, i.o. zymosan in MyD88−/− mice subjected to ONC results in robust axonal regeneration, indistinguishable from that seen in WT mice (Fig. S2 J–L). Administration of i.o. PBS failed to elicit axonal extension beyond the lesion site in WT, TLR2−/−, or MyD88−/− mice (Fig. S3). Collectively, the foregoing findings demonstrate that TLR2 and MyD88 are dispensable for zymosan-elicited RGC axon regeneration.
Zymosan Promotes Axon Regeneration Through Dectin-1.
In addition to TLRs, several other zymosan receptors have been identified, including the β-glucan–binding transmembrane proteins CR3 (18) and dectin-1 (13). Regrowth of injured RGC axons was significantly attenuated in dectin-1−/− mice, but not in CR3−/−, mice, compared with WT mice (Fig. 2 A–F). To directly test whether the residual optic nerve regeneration observed in dectin-1−/− mice is TLR/MyD88-dependent, we generated dectin-1−/−;MyD88−/− compound mutants. Zymosan-elicited optic nerve regeneration was completely abolished in the dectin-1−/−;MyD88−/− mice (Fig. 2 D and F). To examine whether dectin-1 collaborates more specifically with TLR2, we generated dectin-1−/−;TLR2−/− compound mutants (Fig. S4), and found that zymosan-elicited optic nerve regeneration was fully abrogated in these mice as well (Fig. 2 E and F). Interestingly, the inflammatory responses triggered by i.o. administration of zymosan into WT, dectin-1−/−;MyD88−/−, and dectin-1−/−;TLR2−/− compound mutants at 7 d post-ONC are comparable in terms of both cell number and cell composition (Fig. 2G). Thus, reminiscent of our findings with i.o. LPS, these experiments demonstrate that intraocular inflammation does not always result in RGC regenerative growth.

Dectin-1 and TLR2 operate as partially redundant zymosan receptors. (A–E) Longitudinal sections of mouse optic nerves at 2 wk after ONC and i.o. injection of zymosan stained with anti-GAP43. The injury site is marked with an asterisk. (Scale bar: 200 µm.) (A and B) WT mice with i.o. zymosan (n = 5) (A) and complement receptor 3 null mice (CR3−/−) with i.o. zymosan (n = 3) (B) show robust and comparable axon regeneration. (C) In dectin-1−/− mice, i.o. zymosan (n = 5) results in significantly reduced regeneration. (D–E) The i.o. zymosan fails to induce axon regeneration in dectin-1−/−;MyD88−/− compound mutants (n = 7) and dectin-1−/−;TLR2−/− compound mutants (n = 6). (F) Quantification of the number of GAP43+ axons per nerve at 0.2–1.6 mm distal to the injury site. Data are presented as mean ± SEM. Asterisks directly above individual bars indicate a significant difference compared with WT + zymosan. ***P < 0.001; **P < 0.01; *P < 0.05, one-way ANOVA, Tukey’s post hoc test. (G) Comparison of the cellular composite of zymosan-induced inflammation in WT (n = 6 mice), dectin-1−/−;MyD88−/− (n = 6 mice), and dectin-1−/−;TLR2−/− (n = 3 mice) compound mutants. Independent of mouse genotype, similar numbers of macrophages/monocytes, neutrophils, DCs, B cells, and CD4+ and CD8+ T cells, but not of NK cells, were identified in the vitreous.
Theoretically, germline ablation of dectin-1−/−;TLR2−/− could adversely affect the health, and thus the regenerative capacity, of RGCs. To determine whether posttraumatic RGCs in dectin-1−/−;TLR2−/− mice can regenerate their axons, we knocked-down PTEN, and found long-distance axon regeneration after ONC (Fig. S4). Thus, RGCs of compound mutants are capable of regenerative growth in a conducive setting, but fail to do so after i.o. zymosan application.
β(1, 3)-Glucan Promotes Dectin-1–Dependent Axon Regeneration.
β-glucans are the ingredients of zymosan that complex with dectin-1. They exist as large polymers composed of linear β(1, 3) d-glycosidic linkages with occasional side chains bound by β(1, 6) d-glycosidic linkages. We found that in WT mice, i.o. administration of curdlan (Fig. 3 A and D), a particulate form of β(1, 3)-glucan, is as effective as zymosan (Fig. 1 B and E) in promoting RGC axon regeneration following ONC. Delayed administration of curdlan at 48 h after ONC was equally robust in triggering RGC axon regeneration (Fig. S5). The number and composition of infiltrating immune cells at 7 d after ONC and i.o. curdlan or i.o. zymosan are similar (Fig. S6A). Curdlan binds directly to dectin-1 but not to TLR2, and i.o. administration of curdlan in dectin-1−/− mice fails to induce RGC regeneration (Fig. 3 B and D). This indicates that curdlan, unlike zymosan, exerts its proregenerative effects solely through dectin-1, and that engagement of dectin-1 is necessary and sufficient for RGC axon regeneration.
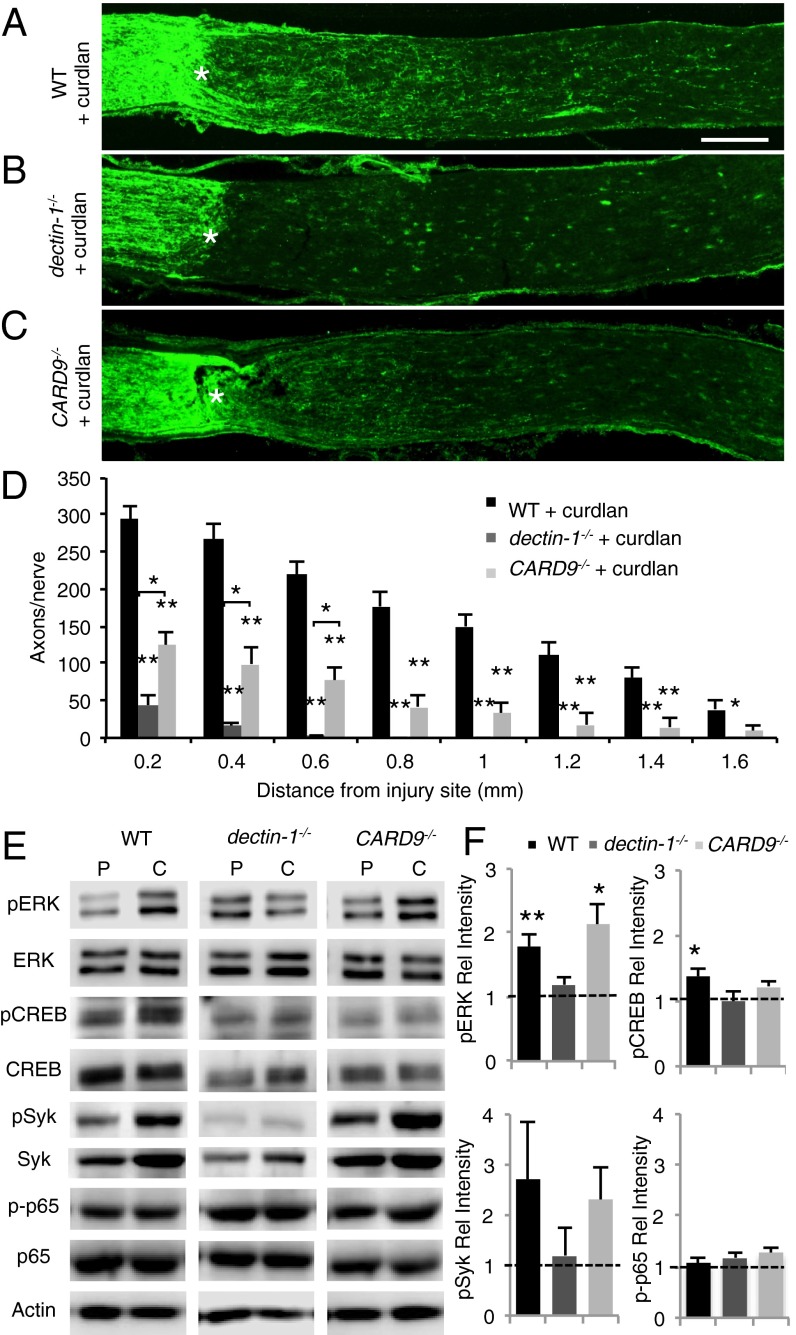
β(1, 3)-glucan promotes dectin-1–dependent axon regeneration. (A–C) Longitudinal sections of mouse optic nerves at 2 wk after ONC and i.o. curdlan (5 µL, 25 µg/µL) stained with anti-GAP43. The injury site is marked with an asterisk. (Scale bar: 200 µm.) (A) WT mice with i.o. curdlan (n = 7) show robust axon regeneration. (B) In dectin-1−/− mice (n = 12), i.o. curdlan fails to elicit a regenerative response. (C) In CARD9−/− mice with i.o. curdlan (n = 9), axon regeneration is significantly reduced, yet increased compared with dectin-1−/− mice at 0.2–0.6 mm distal to the injury site. (D) Quantification of GAP43+ axons at 0.2–1.6 mm distal to the injury site. Results are presented as mean ± SEM. Asterisks directly above individual bars indicate significance compared with WT + curdlan. **P < 0.001; *P < 0.05, one-way ANOVA, Tukey’s post hoc test. (E) Western blot analysis of adult mouse eye extracts at 6 h after ONC and i.o. injection of PBS or curdlan. (F) Quantification of Western blot band intensity relative to respective PBS-injected eyes. Compared with PBS-injected eyes, curdlan induces a significant increases in pERK and pSyk levels in WT and CARD9−/− eyes, but not in dectin-1−/− eyes. Curdlan significantly increases pCREB (S133) levels in WT eyes, but not in dectin-1−/− or CARD9−/− eyes. Curdlan does not increase phosphorylation of the NF-κB subunit p65 (S536) in any of the genotypes examined. Between three and five eyes from two separate experiments were analyzed for each condition and genotype. Data shown are mean ± SEM. **P < 0.01; *P < 0.05.
Curdlan Signals in a Dectin-1– and CARD9-Dependent Manner to Activate CREB.
Ligation of dectin-1 leads to activation of multiple downstream signaling events implicated in fungal immune defense, including phagocytosis of fungal particles, ROS production, and regulation of gene expression (19–21). One pathway, comprising spleen tyrosine kinase (syk) and caspase recruitment domain 9 (CARD9), couples dectin-1 to multiple downstream effectors (22, 23). We assessed the role of this pathway in PAMP-induced RGC axonal regeneration in CARD9−/− mice. In WT and CARD9−/− mice, but not dectin-1−/− mice, i.o. curdlan combined with ONC leads to a rapid increase in syk and p-syk, an important dectin-1 adaptor protein (Fig. 3E). A partial, yet significant reduction in regenerative RGC growth is seen in optic nerve sections of curdlan-injected CARD9−/− mice compared with WT mice (Fig. 3 C and D). This shows that CARD9 functions downstream of dectin-1, but also implies the existence of parallel, CARD9-independent signaling mechanisms.
Dectin-1–mediated activation of the MAP kinase pathway in bone marrow (BM)-derived macrophages leads to activation of cAMP response element-binding protein (CREB) (24). Biochemical analysis of eye lysates revealed rapid activation of extracellular signal-regulated protein kinase (Erk1/2) and CREB in WT mice, but not in dectin-1−/− mice, at 6 h after ONC and i.o. curdlan (Fig. 3 E and F). CARD9−/− mice exhibit increased activation of Erk1/2, but not of CREB (Fig. 3F). This places Erk1/2 activation downstream of dectin-1 and upstream of, or parallel to, CARD9. Dectin-1/CARD9 signaling can activate the canonical NF-κB pathway (20); however, we found no increase in NF-κB activity, as assessed by phosphorylation of p65 at S536 (Fig. 3 E and F). Taken together, these findings suggest that dectin-1/CARD9 signaling in myeloid cells participates in inflammation-mediated neuronal regeneration.
Dectin-1 Is Expressed by Retina-Resident and Blood-Derived Infiltrating Immune Cells.
We next sought to identify the cell type(s) that curdlan targets to elicit RGC axon regeneration. We used flow cytometry analysis to measure dectin-1 expression on retina-resident cells at baseline and after ONC alone, as well as on immune cells that infiltrate the eye in response to i.o. curdlan or zymosan in the setting of ONC. These experiments were performed in CX3CR1GFP/+ reporter mice in which microglia are GFP+. We found that dectin-1 is constitutively expressed at low levels on CD11b/CX3CR1GFP/+ microglia and CD11b+/CD11c+ retinal DCs in naïve eyes (Fig. 4A), and is up-regulated on both cell types at 7 d post-ONC (Fig. 4B). Following i.o. zymosan, dectin-1 is expressed on infiltrating monocytes/macrophages, neutrophils, and myeloid DCs, but not on lymphocytes (Fig. 4C). Immunohistochemical studies confirmed that dectin-1 is strongly expressed by myeloid cells that accumulate in the vitreous of zymosan-injected WT mice (Fig. 4D), but no labeling was observed in the RGC layer or on GFAP+ retinal astrocytes (Fig. 4E). Thus, dectin-1 signaling promotes RGC axon regeneration through an indirect, non–cell-autonomous mechanism.
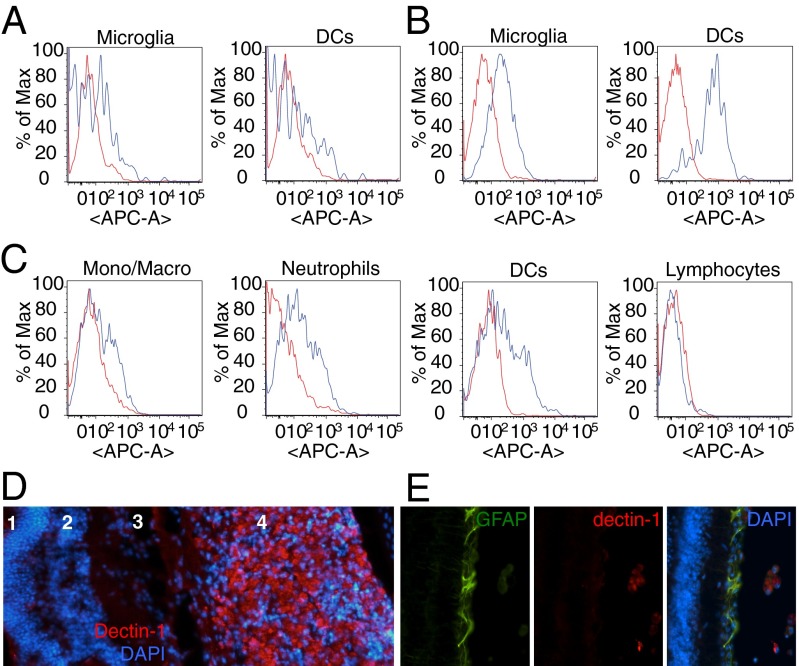
Dectin-1 is expressed on retina-resident and blood-derived myeloid cells. (A–C) Flow cytometry analysis of dectin-1 expression in the eye (blue line), compared with an isotype control antibody (red line). (A and B) Histogram of dectin-1+ microglia and DCs in the eyes of naïve mice (A) and 7 d post-ONC in the absence of zymosan (B). (C) Analysis of dectin-1+ cells in the eye at 7 d post-ONC and i.o. zymosan. (D) Cross-section through the eye at 14 d post-ONC and i.o. zymosan stained with anti–dectin-1 (red) and DAPI (blue). Many dectin-1+ cells are found in the vitreous (4), but not in the retina, including the outer nuclear layer (1), the inner nuclear layer (2), or the RGC layer (3). (E) Anti–dectin-1 immunolabeling is not observed on GFAP+ retinal cells.
Microglia and Infiltrating Myeloid Cells Rapidly Phagocytose Zymosan Particles.
Dectin-1 ligation induces phagocytosis (13). To identify the cell types that ingest zymosan in our experimental system, we injected Alexa Fluor 555-conjugated zymosan into the eyes of CX3CR1GFP/+ reporter mice immediately after ONC. At both 6 h and 18 h postinjection, CX3CR1-expressing microglia (Fig. S7A) and Ly6G+ neutrophils (Fig. S7B) stain positive for intracellular zymosan. At 2 h post-ONC and i.o. zymosan, GFP+ microglia are highly branched and negative for zymosan particles in retinal sections (Fig. S7 C and D). By 6 h postinjection, labeled zymosan particles are observed within GFP+ microglia with a more rounded morphology (Fig. S7 E–I), indicating that retina-resident microglia actively phagocytose zymosan.
Dectin-1 Is Required on Both Radioresistant Retina-Resident Cells and Infiltrating BM-Derived Cells for Curdlan-Induced Axon Regeneration.
The broad expression of dectin-1 by infiltrating myeloid cells, retina-resident microglia, and DCs raises the question of which of these cells contributes to immune-mediated RGC axon regeneration. To functionally assess the role of dectin-1 in radioresistant retina-resident cells (such as microglia) vs. radiosensitive hematopoietic cells (such as infiltrating monocytes/macrophages and neutrophils), we constructed reciprocal BM chimeric mice. Dectin-1−/− BM was transplanted into irradiated WT hosts [KO → WT] to restrict dectin-1 expression in the eye to retina-resident cells. Conversely, we generated [WT→KO] BM chimeras in which dectin-1 expression was restricted to blood-derived immune cells. [WT→ WT] and [KO→KO] chimeric mice served as positive and negative controls, respectively. Chimerism for dectin-1 was confirmed by flow cytometry (Fig. S8). As expected, we found that i.o. curdlan triggers regenerative growth of injured RGC axons in the [WT→ WT] mice (Fig. 5A), but not in the [KO→KO] mice (Fig. 5D). A significant reduction in the number of regenerating axons is seen in both the [KO→WT] and [WT→KO] chimeric mice (Fig. 5 B, C, and E). These studies demonstrate that dectin-1 function is necessary in both retina-resident and infiltrating immune cells for the full extent of curdlan-elicited RGC axon regeneration.

Dectin-1 expression is necessary on both radioresistant retinal cells and BM-derived infiltrating cells for curdlan-induced axon regeneration. Reciprocal BM chimeric mice were subjected to i.o. curdlan injection (5 µL, 25 µg/µL), and axon regeneration was assessed 2 wk later by anti-GAP43 labeling. The injury site in the optic nerve is marked with an asterisk. (Scale bar: 200 µm.) (A) WT mice that received WT donor BM (WT→WT) show robust curdlan-induced axon regeneration. (B–D) In contrast, WT mice that received dectin-1−/− BM (KO→WT) (B) show significantly less regeneration, comparable to that in dectin-1−/− mice that received WT BM (WT→KO) (C) and dectin-1−/− mice that received dectin-1−/− BM (KO→KO) (D). (E) Quantification of GAP43+ axons at 0.2–1.6 mm distal to the injury site (WT→WT + curdlan; n = 6 nerves, 6 mice; KO→WT + curdlan, n = 8 nerves, 5 mice; WT→KO + curdlan, n = 12 nerves, 8 mice; KO→KO + curdlan, n = 6 nerves, 4 mice). (F) Flow cytometry analysis of intraocular inflammation at 7 d after i.o. curdlan and ONC. Inflammation in WT→WT and WT→KO mice is comparable. Significantly decreased inflammation is observed in KO→WT and KO→KO mice. Results are presented as mean ± SEM. Asterisks indicate a significant difference from WT→WT mice. ***P < 0.001; **P < 0.01; *P < 0.05, one-way ANOVA, Tukey’s post hoc test.
Analysis of the immune infiltrate in the eye at 7 d post-ONC revealed that i.o. curdlan elicits robust vitreous inflammation and accumulation of myeloid cells in [WT→KO] mice, but not in [KO→WT] mice (Fig. 5F). The accumulation of dectin-1+ myeloid cells in the vitreous of [WT→KO] mice is not sufficient to promote optimal RGC axon regeneration, however (Fig. 5C). Conversely, dectin-1 expression on radioresistant retina-resident cells in [KO→WT] mice is dispensable for curdlan-induced i.o. inflammation, but is not sufficient to support optimal RGC axon regeneration (Fig. 5 B and F).
Discussion
In the present study, we have identified particulate β-glucan as the active ingredient in zymosan, capable of eliciting long-distance axon regeneration in a dectin-1–dependent manner. This is a novel finding, given that up to now, only TLR2 agonists have been shown to simulate the therapeutic effects of zymosan in the ONC model (3, 7). Moreover, our studies indicate that, although TLR2 and dectin-1 act in a complementary manner to promote axonal regrowth, dectin-1 is dominant. Particulate β-glucan engages dectin-1 on blood-derived myeloid cells, as well as on retina-resident cells, to enable RGC axon regeneration in a non–cell-autonomous manner. The dectin-1 downstream effector CARD9 is required for β-glucan–induced CREB activation and plays an important role in inflammation-mediated RGC axon regeneration. Of clinical interest, administration of β-glucan at the time of ONC or 2 d later promotes equally robust axonal growth, suggesting a large therapeutic window for β-glucan/dectin-1–elicited neurorepair.
It has been widely assumed that the vitreous inflammation induced by i.o. PAMPs is causally linked to enhanced RGC axon growth. Consistent with that contention, we found that β-glucan–mediated RGC axon regeneration is mitigated in [KO→WT] chimeric mice, in which radiosensitive hematopoietic cells are exclusively deficient in dectin-1. However, we also found impaired regeneration in [WT→KO] chimeric mice, implicating the participation of a radioresistant retina-resident cell in the repair process. These data suggest that full-blown neurorepair is dependent on multiple cell types that act via parallel, nonredundant mechanisms. The specific phenotypes of the retina-resident and infiltrating immune cells that promote regeneration via a dectin-1–dependent pathway remain to be elucidated. Other investigators have reported that activation of retinal astrocytes and Muller cells correlates with axonal regeneration (12); however, the only retinal cells that we found to express dectin-1 are microglia and resident DCs. A direct role of retinal microglia in RGC regeneration is further supported by our observation that those cells efficiently phagocytose zymosan particles. In animal models of white matter injury (25) and neurodegenerative disease (26), microglia facilitate remyelination and suppress destructive neuroimmune responses. Thus, microglia could promote dectin-1 mediated neurorepair by clearing cellular debris, releasing growth factors, or by regulating the toxic aspects of inflammation.
Although the inflammatory response triggered by i.o. curdlan activates RGC growth-promoting programs, we observed concurrent toxicity, reminiscent of experimental autoimmune uveitis (27). Curdlan causes retinal folding and detachment and, similar to zymosan in the spinal cord or DRGs (9), is associated with tissue damage. Thus, β-glucan/dectin-1 signaling is sufficient to mimic the proregenerative effects of zymosan, but causes concomitant pathology. In dectin-1−/− mice, curdlan-elicited RGC regeneration and retinal toxicity were no longer observed (Fig. S6), suggesting that the two processes may be coupled. The intensity of vitreous inflammation did not always correlate with extent of axonal growth, however; for example, i.o. zymosan induced comparable vitreous infiltrates in WT, dectin-1;MyD88, and dectin-1;TLR2 compound mutant mice, yet axonal regeneration was observed only in WT mice. Similarly, both i.o. LPS and i.o. zymosan led to strong vitreous inflammation and comparable ROS production, but LPS failed to promote RGC regeneration. This paradox could reflect the fact that a distinct subset of leukocytes, as-yet unidentified, has the proregenerative properties, and that this subset is relatively depleted in infiltrates of the compound mutants or following i.o. LPS administration.
Dectin-1+ monocyte/macrophages and neutrophils are universally the most prominent constituents of PRR-induced vitreous infiltrates. Both of these myeloid cells have been touted as candidates for the immune cell that facilitates RGC axon growth (3, 11); however, there is growing recognition of the heterogeneity of myeloid cells (25, 28, 29). For example, macrophages, and possibly microglia, can be polarized along a continuum of activation states, including the well-known proinflmmatiory (M1-like) and anti-inflammatory (M2-like) phenotypes. Myeloid cell polarization can positively or negatively impact repair after nervous system injury (30–32). Stimulus-specific transcriptional programs downstream of PRRs can modulate the macrophage phenotype (33). Transcription factors activated in a dectin-1/CARD9–dependent manner include NF-κB (19, 20), IRF5 (34), and CREB (21, 24, 35). Dectin-1/CARD9–dependent activation of CREB downstream of curdlan coincides with enhanced regenerative growth of injured RGCs. Dectin-1 and CREB signaling in macrophages mediates polarization toward an M2-like phenotype and in nonneural tissue has been shown to promote repair following injury (36). The partial loss of RGC axon regeneration in CARD9−/− mice suggests the involvement of additional, dectin-1–dependent pathways that function independent of CARD9.
We propose that activation of myeloid cells through β-glucan/dectin-1 leads to the expression and secretion of proregenerative factors that ultimately enable injured RGCs to switch to a proregenerative state and extend long axons. A growing list of molecules have been identified that directly or indirectly participate in inflammation-mediated axonal repair, including chemokines, “anti-inflammatory” cytokines, growth factors, and the calcium-binding protein oncomodulin (4, 6, 37, 38). Thus, it appears likely that multiple factors participate in β-glucan–elicited RGC axon regeneration. Future studies, including an in-depth analysis of the cellular and molecular milieu under inflammatory conditions that promote (e.g., β-glucan) or fail to promote (e.g., LPS) RGC axon regeneration, are needed to identify the myeloid cell types, their activation states, and growth factors underling inflammation-mediated neuronal repair. The molecular framework described here provides a strong platform for future studies aimed at understanding the cross-talk between the immune system and the nervous system and how this may be exploited to promote repair following injury or disease.
Materials and Methods
Optic Nerve Surgery.
All animal handling and surgical procedures were performed in compliance with local and national animal care guidelines and approved by the University of Michigan Committee on Use and Care of Animals. Optic nerves of adult C57BL/6 WT and transgenic mice were exposed and subjected to retro-orbital crush injury. To induce intraocular inflammation, PAMPs were injected in the posterior chamber of the eye (Fig. S1A). At 2 wk after ONC, mice were killed and optic nerves were removed, cryosectioned longitudinally, and subjected to anti-GAP43 immunofluorescence labeling.
Flow Cytometry.
At various time points after i.o. injection of PAMPs, mice were euthanized and eyes were removed. Retina and vitreous fluids were pooled and digested with collagenase-D. The resulting cell suspensions were incubated with fluorochrome-conjugated antibodies specific for subsets of leukocytes and subjected to flow cytometry analysis.
Construction of BM Chimeric Mice.
Recipient mice were lethally irradiated and given congenic (CD45.1 or CD45.2) BM from donor mice (5 million cells in 300 µL) via tail vein injection. At 6 wk after irradiation, the mice were subjected to optic nerve regeneration studies.
Acknowledgments
We thank Tobias Hohl for dectin-1;MyD88 and CARD9 mice, Larry Benowitz for anti-GAP43, and Zhigang He for AAV-shPTEN-GFP. This work was supported by the Charles A. Dana Foundation (B.M.S. and R.J.G.), Cellular and Molecular Biology Training Grant T32GM007315 (to K.T.B.), Ruth Kirschstein Fellowship F31NS081852 (to K.T.B.), Training Grant 3T32NS007222-31S1 (to K.S.C.), the Dr. Miriam and Sheldon G. Adelson Medical Foundation on Neural Repair and Rehabilitation (R.J.G.), and Veterans Administration Merit Review Awards 1I01RX000416 and 1I01BX001387 (to R.J.G. and B.M.S.), R01 NS081281 (to R.J.G.), and R01 NS057670 (to B.M.S.). B.M.S. is a Senior Scholar of the A. Alfred Taubman Medical Research Institute.
Footnotes
The authors declare no conflict of interest.
This article is a PNAS Direct Submission.
This article contains supporting information online at www.pnas.org/lookup/suppl/10.1073/pnas.1423221112/-/DCSupplemental.
References
Articles from Proceedings of the National Academy of Sciences of the United States of America are provided here courtesy of National Academy of Sciences
Full text links
Read article at publisher's site: https://doi.org/10.1073/pnas.1423221112
Read article for free, from open access legal sources, via Unpaywall:
https://www.pnas.org/content/pnas/112/8/2581.full.pdf
Citations & impact
Impact metrics
Citations of article over time
Alternative metrics
Article citations
Immunomodulation by the combination of statin and matrix-bound nanovesicle enhances optic nerve regeneration.
NPJ Regen Med, 9(1):31, 26 Oct 2024
Cited by: 0 articles | PMID: 39461953 | PMCID: PMC11513974
Knockout of Dectin-1 does not modify disease onset or progression in a MATR3 S85C knock-in mouse model of ALS.
Heliyon, 10(18):e37926, 14 Sep 2024
Cited by: 0 articles | PMID: 39323783 | PMCID: PMC11422021
Augmenting fibronectin levels in injured adult CNS promotes axon regeneration in vivo.
Exp Neurol, 379:114877, 27 Jun 2024
Cited by: 0 articles | PMID: 38944331
Cytokine polarized, alternatively activated bone marrow neutrophils drive axon regeneration.
Nat Immunol, 25(6):957-968, 29 May 2024
Cited by: 5 articles | PMID: 38811815
Retinal transplant immunology and advancements.
Stem Cell Reports, 19(6):817-829, 09 May 2024
Cited by: 0 articles | PMID: 38729155 | PMCID: PMC11297553
Review Free full text in Europe PMC
Go to all (85) article citations
Data
Data behind the article
This data has been text mined from the article, or deposited into data resources.
BioStudies: supplemental material and supporting data
Similar Articles
To arrive at the top five similar articles we use a word-weighted algorithm to compare words from the Title and Abstract of each citation.
Beta-glucan activates microglia without inducing cytokine production in Dectin-1-dependent manner.
J Immunol, 180(5):2777-2785, 01 Mar 2008
Cited by: 64 articles | PMID: 18292498
Barley beta-Glucan and Zymosan induce Dectin-1 and Toll-like receptor 2 co-localization and anti-leishmanial immune response in Leishmania donovani-infected BALB/c mice.
Scand J Immunol, 92(6):e12952, 27 Aug 2020
Cited by: 9 articles | PMID: 32748397
Toll-Like Receptors and Dectin-1, a C-Type Lectin Receptor, Trigger Divergent Functions in CNS Macrophages.
J Neurosci, 35(27):9966-9976, 01 Jul 2015
Cited by: 43 articles | PMID: 26156997 | PMCID: PMC4495245
Neuroinflammation, Microglia and Implications for Retinal Ganglion Cell Survival and Axon Regeneration in Traumatic Optic Neuropathy.
Front Immunol, 13:860070, 04 Mar 2022
Cited by: 34 articles | PMID: 35309305 | PMCID: PMC8931466
Review Free full text in Europe PMC
Funding
Funders who supported this work.
BLRD VA (1)
Grant ID: I01 BX001387
Dr. Miriam and Sheldon G. Adelson Medical Research Foundation (1)
Grant ID: PR18
HHS | NIH | National Institute of General Medical Sciences (1)
Grant ID: T32GM007315
HHS | NIH | National Institute of Neurological Disorders and Stroke (4)
Grant ID: R01NS081281
Grant ID: R01NS057670
Grant ID: 3T32NS007222-31S1
Grant ID: F31NS081852
NIAID NIH HHS (1)
Grant ID: T32 AI007413
NIGMS NIH HHS (2)
Grant ID: T32 GM007315
Grant ID: T32GM007315
NINDS NIH HHS (6)
Grant ID: 3T32NS007222-31S1
Grant ID: R01 NS057670
Grant ID: F31NS081852
Grant ID: T32 NS007222
Grant ID: R01 NS081281
Grant ID: F31 NS081852
RRD VA (1)
Grant ID: I01 RX000416
U.S. Department of Veterans Affairs (2)
Grant ID: 1I01RX000416
Grant ID: 1I01BX00138