Abstract
Free full text

Targeting the miR-221-222/PUMA/BAK/BAX pathway abrogates dexamethasone resistance in multiple myeloma
Abstract
Despite recent therapeutic advances that have doubled the median survival time of patients with multiple myeloma (MM), intratumor genetic heterogeneity contributes to disease progression and emergence of drug resistance. MicroRNAs (miRs), are noncoding small RNAs that play important roles in the regulation of gene expression, and have been implicated in cancer progression and drug resistance. We investigated the role of the miR-221-222 family in dexamethasone(Dex)-induced drug resistance in MM using the isogenic cell lines, MM1R and MM1S, which represent models of resistance and sensitivity, respectively. Analysis of array comparative genome hybridization (aCGH) data revealed gain of chromosome X regions at band p11.3, wherein the miR-221-222 resides, in resistant MM1R cells but not in sensitive MM1S cells. DNA copy number gains in MM1R cells were associated with increased miR-221-222 expression and downregulation of p53-upregulated modulator of apoptosis (PUMA) as a likely pro-apoptotic target. We confirmed PUMA mRNA as a direct target of miR-221-222 in MM1S and MM1R cells by both gain- and loss- of function studies. In addition, miR-221-222 treatment rendered MM1S cells resistant to Dex, whereas anti-miR-221-222 partially restored the Dex sensitivity of MM1R cells. These studies have uncovered a role for miR-221-222 in MM drug resistance, and suggest a potential therapeutic role for inhibitors of miR-221-222 binding to PUMA mRNA as a means of overcoming Dex resistance in patients. The clinical utility of this approach is predicated on the ability of anti-sense miR-221-222 to increase survival while reducing tumor burden, and is strongly supported by the metastatic propensity of MM1R cells in preclinical mouse xenograft models of MM. Moreover, our observation of increased levels of miR-221-222 with decreased PUMA expression in MM cells from patients at relapse versus untreated controls suggests an even broader role for miR-221-222 in drug resistance, and provides a rationale for the targeting of miR-221-222 as a means of improving patient outcomes.
INTRODUCTION
Despite recent advances in treatment, multiple myeloma (MM) it remains incurable due to tumor progression and the emergence of resistance (1). Therefore, in order to develop more effective treatments and improve patient outcome, it is imperative to better understand the cellular and molecular mechanisms mediating drug resistance in MM. Many treatment regimens include novel agents in combination with Dex; unfortunately, however, MM cells often become Dex-resistant (1).
The exact basis for the beneficial mechanism of action of glucocorticoids in cancer treatment has not been fully and definitively elucidated, although the apoptotic pathway is considered to be the main target. It is thought that glucocorticoid-induced apoptosis is initiated via activation of transcription of death-specific genes, and inhibition of the apoptotic cascade is believed to occur via negative modulation of proinflammatory cytokines that block transcription of death-specific genes (2). During prolonged exposure to Dex, it is believed that resistance apparently stems from downregulation of glucocorticoid receptor (GR) gene expression (3). Although only one GR gene has been identified, several GR proteins (e.g. GRα and GRβ can be generated by alternative splicing of the mRNA. GRα is expressed at relatively higher levels than GRβ in most tissues and plays a major role in Dex-induced apoptosis. However, the mechanism of GRα downregulation in Dex-resistant patients remains somewhat ambiguous (3). Other mechanisms of induced Dex resistance have been proposed and include: i) overexpression of the ABC transporter that will decrease intracellular Dex levels, leading to resistance (4); ii) blocking of the pro-apoptotic effect of Dex by cytokines secreted by the bone marrow (BM) microenvironment, or via binding of MM cells to BM stroma, in either case inducing cell adhesion-mediated resistance (5).
MicroRNAs (miRNAs) are small (~22nt) noncoding RNAs that negatively regulate protein-coding gene expression by enhancing degradation or inhibiting translation of mRNAs (6,7). Disregulation of miRNA expression is frequently detected in MM and has been associated with increased metastatic potential and poor clinical outcome, suggesting an important role of miRNAs in MM disease progression (8). MiR-221 and miR-222 are highly homologous miRNAs encoded on the X-chromosome (9) and designated as the miR-221-222 cluster. This cluster has been found to be over-expressed in a large variety of human cancers, including hematologic malignancies such as MM (10). It has been shown that miR-221-222 promotes oncogenesis by down-regulating the expression of tumor suppressors such as the pro-apoptotic protein p53-upregulated modulator of apoptosis (PUMA) and the bcl2-interacting mediator of cell death (BIM) (11). Here, we have used the isogenic cell lines MM1R and MM1S, which represent resistance and sensitivity, respectively, to Dex, in order to delineate a pathogenetic role of the miR-221-222 cluster in promoting Dex resistance in MM via downregulation of PUMA, and inhibition of apoptosis.
EXPERIMENTAL PROCEDURES
Cell lines and human tumor tissues and RNA isolation
MM1S and MM1R cell lines were obtained from the American Type Culture Collection (ATCC).
miRNA profiling in MM1S and MM1R cells
Total RNA was isolated from MM1R and MM1S cells grown in tissue culture or isolated from xenografts using Trizol reagents (Invitrogen), and subjected to Q-RT-PCR anlysis or whole-genomic miRNA profiling using TaqMan® Low Density microRNA Array (AM1792, Invitrogen). Expression of each miRNA was presented directly using their threshold cycle number (Ct). Upregulated miRNAs are with low Ct number while downregulated miRNAs are with high Ct number.
Quantitative-RT-PCR and immunoblot analyses
Quantitive-RT-PCR for evaluating miR-221, miR-222 and PUMA mRNA levels was performed as previously described (12). The primers for miR-221(#000524), miR-222(#000525), U44(#001094), PUMA(#Hs00248075_m1), and GAPDH (#Hs99999905_m1) were purchased from Applied Biosystems (ABI). Immunoblots were obtained as previously described (13). Primary antibodies included: anti-PUMA (#4976, Cell Signaling), anti-BAX (#2772, Cell Signaling), anti-Cleaved Caspase 3 (#9661, Cell Signaling), anti-PARP (#9542, Cell Signaling), anti-BID (#sc-11423, Santa Cruz), anti-BAK (#06-536, Millipore), anti-P53 (#SC-126, Santa Cruz) and anti-BIM (#202000, Calbiochem). Horseradish peroxidase (HRP)-conjugated secondary antibodied included: anti-rabbit IgG (#W4011, Promega), anti-mouse IgG (#W4021, Promega), and anti-Actin (#sc-1615, Santa Cruz).
Plasmids and 2’-OMe-modified anti-miR-221/222
Viral expression vectors of miR-221-222 (V-miR-221-222) were created by PCR utilizing normal human DNA and the following pair of primers: V-miR-221-222 (F)(EcoR1) TAGCGAATTCGCTCCCCAGAAGGCAAAGGAT, and V-miR-221-222(R)(Not1) CTTCGCGGCCGCTGGTGAGACAGCCAATGGAG, then cloned into the EcoR1 and Not restriction sites of pCDH-CMV-EF1-GFP viral vector (#CD511B-1, System Biosciences). The packaging system was used according to the manufacturer's protocol. The sequences of as-miR-222 and as-miR-221 were 5’-ACCCAGUAGCCAGAUGUAGCU-3’, and 5’-GAAACCCAGCAGACAAUGUAGCU-3’, respectively. Scrambled 2’-OMe-modified RNA (5’-AAGGCAAGCUGACCCUGAAGU-3’) was used as a negative control.
As-miR-221-222 sponge plasmid
The following oligos were obtained from Integrated DNA Technologies: as-221-222-EN(F) 5’-AATTCGAAACCCAGCAGACAATGTAGCTACCCAGTAGCCAGATGTAGCTGAAACCCAGCAGACAATGTAGCTACCCAGTAGCCAGATGTAGCTGAAACCCAGCAGACAATGTAGCTACCCAGTAGCCAGATGTAGCTGAAACCCAGCAGACAATGTAGCTACCCAGTAGCCAGATGTAGCTgc-3’, as-221-222-EN(R) 5’-GGCCGCAGCTACATCTGGCTACTGGGTAGCTACATTGTCTGCTGGGTTTCAGCTACATCTGGCTACTGGGTAGCTACATTGTCTGCTGGGTTTCAGCTACATCTGGCTACTGGGTAGCTACATTGTCTGCTGGGTTTCAGCTACATCTGGCTACTGGGTAGCTACATTGTCTGCTGGGTTTCg-3’. The oligos were dispensed into annealing buffer and inserted into the EcoRI site of pCDH-CMV-EF1-GFP (#CD511B-1, System Biosciences). The construct is pCDH-CMV-as-miR-221-222-EF1-GFP with 3 repeat of antisense of miR-221 and miR-222 sequences (5’-GAAACCCAGCAGACAATGTAGCTACCCAGTAGCCAGATGTAGCT-3’), and is refered to as V-as-miR-221-222-GFP.
Argonaute 2 (AGO2) binding RNA immunoprecipitation (RIP) quantitative-RT-PCR
AGO2 binding RNA immunoprecipitation analysis were performed as described (14). MM1S V-GFP and MM1S V-miR-221-222-GFP stable transduced cells (3×107 per RIP experiment) were washed with ice-cold phosphate buffer solution (PBS) and dispensed into 1 ml of cell lysis buffer (50 mM Tris-HCl PH 7.5, 150 mM NaCl,1 mM EDTA, 1% NP 40) containing Protease inhibitor (#5871S, Cell Signaling) and RNase inhibitor (#N808-0119, Invitrogen) for 20 min on ice. Lysates were microcentrifuged at maximum speed for 20 min at 4°C, and supernatants were collected and pre-cleared with 20 μl of Protein A and G Dynabeads coupled with 5 μg of normal Rabbit IgG (#SC-3888, Santa Cruz). The beads were removed by centrifugation and the supernatants incubated with Dynabeads A and G coupled with 5 μg of anti-AGO2-specific antibody (#2897S, Cell Signaling) or 5 μg IgG isotype control antibody (#SC-3888, Santa Cruz) overnight at 4°C, followed by three time washes with washing buffer (50 mM Tris-HCL Ph 7.5, 300 mM NaCl, 1mM EDTA, 1% NP 40, Protease inhibitor). After pulldown by centrifugation, RNA was extracted using 1000 μl TRIZOL reagent (Invitrogen). Quantitative RT-PCR detection of pulled-down PUMA mRNA and miR-221/222 was performed as described in the section of Q-RT-PCR.
PUMA Open Reading Frame (ORF) overexpression virus
PUMA cDNA lacking a 3’UTR was amplified from pHA-PUMA plasmid (15) (Plasmid #16588, Addgene) using the following pair of primers: PUMA-V-dsRed-F-EcoR1, 5’-GCTAGCGAATTCGCCGCCACCATGGCCCGCGCACGCCAG-3’ and PUMA-V-dsRed-R-BamH1, 5’-CCGCGGATCCATTGGGCTCCATCTCGGG-3’. The PCR products were purified and cloned into the EcoR1 and BamH1 restriction sites of the pCDH-CMV-EF1-dsRed viral vector (herein referred as V-PUMA-dsRed), and to allow sorting by flow cytometry using the red-fluorescent protein dsRed as a marker.
BH3 domain profiling in MM cells
MM1R V-dsRed and MM1R V-PUMA-dsRed stable transduced cells were subjected to BH3 profiling as previously described (16). Briefly, cells were permeabilized with digitonin and exposed to BH3 peptides on a 384-well plate. Loss of mitochondrial transmembrane potential loss induced by the peptides was measured over a period of 3 hrs with the help of the radiometric dye JC-1 and a Tecan plate reader. Mitochondrial membrane depolarization, expressed as a percentage of control values, was calculated from the area under the curve for each peptide, and was normalized relative to the solvent-only control, dimethyl sulfoxide (DMSO), designated as 0%, and, as a positive control, the ionophore carbonyl cyanide p-trifluoromethoxyphenylhydrazone (FCCP), designated as 100%.
Locked nucleic acid (LNA) miRNA in situ hybridization (LNA-ISH) and imunostaining
LNA-ISH was performed and analyzed as previously described (12) following the instruction of DIG Nucleic Acid Detection Kit (#11175041910, Roche). The sequences of digoxigenin (DIG)-labeled oligos were: LNA-miR-222 (5’-DIG-accCaGtAgCcAgaTgTAgct-3’), and LNA-miR-221 (5’-DIG-gaaacCcaGcaGacAaTGtAGct-3’). Capital letters indicate LNA modification.
Imunostains were performed according to our routine procedures (12). Briefly, formalin-fixed tissues were embedded in paraffin, sectioned, and stained with hematoxilin and eosin (H&E). Four-micron sections of formalin-fixed tissue were used for immunohistochemical analysis after baking at 60°C for 1 hour, deparaffinization, and rehydration. The sections were then blocked for peroxidase activity with 3% hydrogen peroxide in methanol for 10 min, washed under running water for 5 min, and finally pressure-cooked at 123°C in citrate buffer (DAKO Target Retrieval Solution, S1699) for antigen retrieval. The slides were cooled for 15 min, transferred to Tris-saline (TBS), and incubated with primary antibodies (5 μg/ml) or the corresponding IgG fraction of pre-immune serum overnight at 4°C in blocking solution consisting of containing 3% bovine serum albumin (BSA) in phosphate-buffered saline (PBS). Anti-human primary specific antibodies included: PUMA (#AB9643, AbCam), BAX (#5023P, Cell signaling), and BAK (#6947S, Cell signaling), cleaved caspase 3 (#9664; Cell Signaling), and were visualized with the aid of the corresponding biotinylated antibody coupled to streptavidin-peroxidase complex (Vector Labs). Optimal antibody concentrations were used according to recommendations of the manufacturer. Incubations were carried out under a CO2-humidified atmosphere at room temperature, and slides were incubated with VECTASTAIN Universal ABC Kit (Vector) for 30min and rinsed with PBS between each incubation. The sections were developed using 3,3’-diaminobenzidine (DAB) (Sigma-Aldrich) as the substrate, and were counterstained with Mayer's Hematoxylin. Frozen and formalin-fixed paraffin-embedded human primary MM cells were obtained from the Tissue Procurement Facility at DFCI in accord with IRB protocols. For cleaved caspase 3 immunostains, cells were first spun down onto slides using a cytocentrifuge (Shandon), then fixed in methanol:acetone for 2 min, and washed in PBS prior to immunostaining.
Cell viability assay
Cell viability was assessed with 3-(4,5-dimethylthiazol-2-yl)-2,5-diphenyltetrazolium bromide(MTT) as previously described (17). 1×104 MM1S V-GFP and MM1S V-miR-221-222-GFP stable transduced cells were seeded onto a 96-well plate, and after 24 hrs of incubation were treated with Dex (20μg/ml, Sigma-Aldrich), Bortezomib (PS-341; Velcade) (10 nM, LC laboratories), lenalidomide (1μM, AVA Chem), melphalan (1 μM, Sigma-Aldrich), Doxorubicin (25 ng/ml, Sigma-Aldrich) or DMSO/Ethonol alone as control for 48 h and then subjected to MTT assay.
In vitro luciferase reporter assay
Two reporter plasmids that could recognize the miR-221-222 target PUMA 3’UTR were constructed into pmiR-REPORT plasmid (#AM5795, Life technology), pmiR-PUMA-wt and pmiR-PUMA-mut, that served as conserved and nonconserved target sites of PUMA 3’-UTR, respectively. The sequences were as follows: pmiR-PUMA-wt(F), CGCGTGACTTTCTCTGCACCATGTAGCAGACTTTCTCTGCACCATGTAGCAGACTTTCTCTGCACCATGTAGCAGGATCCA; pmiR- PUMA-wt(R), AGCTTGGATCCTGCTACATGGTGCAGAGAAAGTCTGCTACATGGTGCAGAGAAAGTCTGCTACATGGTGCAGAGAAAGTCA; pmiR-PUMA-Mut(F), CGCGTGACTTTCTCTGCACCTACATCGTGACTTTCTCTGCACCTACATCGTGACTTTCTCTGCACCTACATCGTGGATCCA; pmiR- PUMA-Mut (R), AGCTTGGATCCACGATGTAGGTGCAGAGAAAGTCACGATGTAGGTGCAGAGAAAGTCACGATGTAGGTGCAGAGAAAGTCA. The primers were annealed and inserted into the pmiR-Reporter construct (Ambion). Empty pmiR plasmid (pmiR-0) served as a negative control. Triplicate samples of 1×104 MM1S and MM1R cells in 24-well plates were transfected using lipofectamine-2000 (Invitrogen) with 0.1μg of the reporter plasmids and 0.05 μg of Renilla control plasmid (Promega). Six hours after transfection, the cells were fed with fresh DMEM with 10% FBS and incubated overnight. Cell extracts were then prepared, and luciferase assays were performed using the Dual Luciferase Reporter Assay System (Promega). Luciferase activities were normalized with respect to parallel Renilla activities.
Mouse xenograft models of tumor burden and metastasis
5 ×106 MM1S cells stably transduced with V-miR-221-222-GFP or V-GFP and MM1R cells, stably transduced with V-as-miR-221-222-GFP or V-GFP, were injected via the tail vein into CB17.Cg-PrkdcscidLystbg-J/Crl mice (Code 250, Charles River) to establish a disseminated human MM xenograft model as previously described (12). Survival was evaluated from the first day of tumor injection until death. All mice were intraperitoneally injected with 9mg/kg Dex-21-phosphate disodium salt (Sigma-Aldrich) on days 1~4, 9~12, and 17~20. Mice were observed daily and sacrificed when hind limb paralysis was detected. Hind limb paralysis and tumor burden were used as an endpoint. To assess in vivo cell proliferation, apoptosis, and expression of miR-221-222 target genes, GFP-positive tumor samples were excised for immunohistochemical analysis, as in previous studies (12). All experiments involving animals were pre-approved by the DFCI Institutional Animal Care and Use Committee.
The MM1R-Luc-GFP tumor dissemination mouse model
Totally 15 NOD/SCID mice were injected via the tail vein with 5×106 MM1R-Luc-GFP cells (Gift from Dr. Constantine S Mitsiades, at DFCI), and one week after injection, the mice were randomized to seperate to control and treated groups and treated by intraperitoneal injection once a week with vehicle, RNA-LANCErII (BioScience) to control mice, or 1:1 as-miR-221 and as-miR-222 (as-miR-221-222 mix) (100 pmol total, pre-mixed in RNA-LANCErII) to treatment group mice. All mice were intraperitoneally injected with 9 mg/kg Dex-21-phosphate disodium salt (Sigma-Aldrich) on days 2~5, 9~12, 17~20, and 25~28. Tumor development was monitored by whole-body imaging using a Xenogen system. Mice were evaluated every week after initiation of treatment, and survival was evaluated from the day of tumor injection until death.
Statistical analysis
Differences between groups were analyzed by the unpaired Student's t test (with the exeption of survival curves). Kaplan-Meier survival curves were generated using Prism software and compared using a logrank test. In all stastistical analyses, p≤0.05 considered statistically significant.
RESULTS
Differential expression of miR-221-222 in MM1S and MM1R myeloma cell lines
To study the mechanism of Dex resistance in MM, two isogenic cell lines were previously generated (18): the parental cell line MM1S and the resistant MM1R subline. To investigate a possible role of miRNAs in promoting Dex resistance in MM1R cells, we first performed genome-wide miRNA expression analysis in MM1R and MM1S cells using miRNA arrays. We found that 10 miRNAs were upregulated (Fig 1A), whereas 12 others were downregulated in MM1R cells (Fig 1B) versus MM1S cells. These results prompted us to examin CGH array data from the Broad Institute's Multiple Myeloma Genomics Portal (http://www.broadinstitute.org/mmgp/home) (19). Copy numbers were log2-transformed prior to plotting using the Broad's Integrative Genome Browser. Interestingly, analysis of the data revealed copy number gains in the X-chromosome of MM1R as compared with MM1S cells, without significant copy number differences in other chromosomal regions (Fig 1C, top). Interestingly, miR-222 was among the upregulated miRNAs in MM1R cells. Increased levels of miR-221 and miR-222 expression in MM1R cells were confirmed using quantitative reverse transcriptase-PCR (Q-RT-PCR) (Fig 2F, see below). More detailed examination of the X-chromosome revealed that band p11.3, in which the miR-221-222 cluster resides, has a normal gene copy number in MM1S cells but is tetraploid in MM1R cells (Fig 1C, bottom). Taken together, these findings indicate that gene copy numbers contribute to higher expression of the miR-221-222 in MM1R cells than in MM1S cells, suggesting a possible pathogenetic role for this cluster in Dex resistance.

Analysis of miRNA expression profiling using TaqMan® Low Density microRNA Array in MM1S and MM1R cells reveals that several miRNAs are upregulated with low Ct number (A) while others are downregulated with high Ct number (B) in MM1R cells as compared with MM1S cells. Ct means threshold cycle number. (C) Top: CGH array analysis of MM1S and MM1R cells shows gene copy number gains on the X-chromosome in MM1R as compared with MM1S cells. Bottom: Closer examination of the X-chromosome reveals that band p11.3, where the miR-221-222 cluster resides, has a normal gene copy number in MM1S cells (heatmap showing in light red color) but is tetraploid in MM1R cells (heatmap showing in dark red color) (bottom).

(A) Immunoblot analysis of PUMA expression in MM1S and MM1R cells. (B) Immunoblot analysis showing increased expression of PUMA in MM1R cells lentivirally transduced with a vector encoding PUMA (V-PUMA-dsRed) as compared with mock transduced cells (V-dsRed). (C) Immunocytochemical analysis of PUMA (top) and cleaved caspase-3 (middle) expression in MM1R cells transduced with V-PUMA-dsRed and V-dsRed and treated (bottom) with Dex or untreated (middle). (D) BH3 profiling of MM1R cells transduced with V-PUMA-dsRed and V-dsRed. (E) miR-221 and miR-222 were predicted to bind to the 3’UTR region of human PUMA mRNA. The seed sequence of the miR-221-222 binding site on the PUMA 3’UTR, highly conserved in human (Hsa), mouse (Mm) and catfish (Cf), was replaced by the unrelated artificial sequence shown in red on the pmiR-PUMA-mut reporter plasmid. (F) Comparative Q-RT-PCR analysis of miR-221 (left), miR-222 (middle) and PUMA mRNA (right) expression in MM1S and MM1R cells. * p<0.05, ** p<0.01.
PUMA plays a key role in the Dex resistance in MM1R cells
Since Dex promotes MM cell death through induction of apoptosis (20); we next examined expression levels of pro-apoptotic factors in MM1S and MM1R cells using immunoblot analysis. As shown in Fig 2A and Fig S1, PUMA expression was significantly decreased in MM1R cells as compared with MM1S cells. In agreement with the wild type status of p53 in these two cell lines (21), equal levels of p53 protein were detected by immunoblots. To further verify that PUMA plays a major role in promoting Dex resistance in MM1R cells, we lentivirally transduced MM1R cells with vectors expressing dsRed protein alone as control (V-dsRed) or dsRed in combination with PUMA (V-PUMA-dsRed). To exclude possible endogenous miRNA regulation of transduced PUMA, the mRNA encoding PUMA lacked 3’UTR sequences. After flow cytometric sorting of dsRed positive cells, stably V-PUMA-dsRed- and V-dsRed- transduced cells were first examined by western blot (Fig 2B) and immunohistochemical (Fig 2C, top) analysis to confirm increased PUMA expression in V-PUMA-dsRed cells, and then evaluated them for apoptosis in the absence or presence of Dex. As shown in Fig 2C, apoptosis observed by cleaved caspase-3 staining was greater in MM1R V-PUMA-dsRed cells than in V-dsRed cells, especially after treatment with Dex. In addition, BH3 profiling revealed that MM1R V-PUMA-dsRed cells were more primed to undergo apoptosis than V-dsRed cells (Fig 2D), further highlighting the role of PUMA as a mediator of Dex resistance in MM1R cells.
The 3’UTR of PUMA mRNAs contains two binding sites for miR-221-222
We next investigated whether PUMA mRNA expression is regulated by miRNAs. We first used miRNA target prediction databases (TargetScan) to identify possible miRNAs targeting PUMA among the upregulated miRNAs in our MM1S and MM1R miRNA profiling data (Fig 1A). Interestingly, miR-221 and miR-222 were the top candidates that could target PUMA. Bioinformatics analysis indicated that miR-221 and miR-222 share a single binding site on the 3’UTR of PUMA mRNA, which turns out to be conserved across different species (Fig 2E), suggesting a possible role for these miRNAs in regulating PUMA expression. The inverse correlation of miR-221-222 and PUMA mRNA expression in MM1S and MM1R cells, as evaluated by Q-RT-PCR, further support this possibility (Fig 2F).
To directly demonstrate a physical and functional interaction between miR-221-222 and the 3’UTR of PUMA, we made reporter constructs containing empty (pmiR-0), wild-type (pmiR-PUMA-wt), and mutant (pmiR-PUMA-mut) sequences of the 3’UTR of PUMA mRNA (Fig 2E). The constructs were transfected into MM1S cells, which were subsequently mock-treated with cel-miR-67 or treated miR-221-222 (Fig 3A). After documenting enforced expression of both miR-221 (Fig 3A, top) and miR-222 (Fig 3A, middle) by Q-RT-PCR, we observed that wild-type, but not mutant, PUMA reporter activity was inhibited by miR-221-222 (Fig 3A, bottom). To further define the role of targeting miR-221-222 as a way to abrogate Dex resistance, MM1R cells transduced with pmiR-0, pmiR-PUMA-wt, or pmiR-PUMA-mut reporter vectors were treated with control (scrambled miR) or anti-sense (as) miR-221-222 (2’-OMe as-miR-221-222) oligos (Fig 3B). After confirming by Q-RT-PCR that expression of both miR-221 (Fig 3B, top) and miR-222 (Fig 3B, middle) was efficiently knocked down, we observed that wild-type, but not mutant, PUMA reporter activity was restored (Fig 3B, bottom).
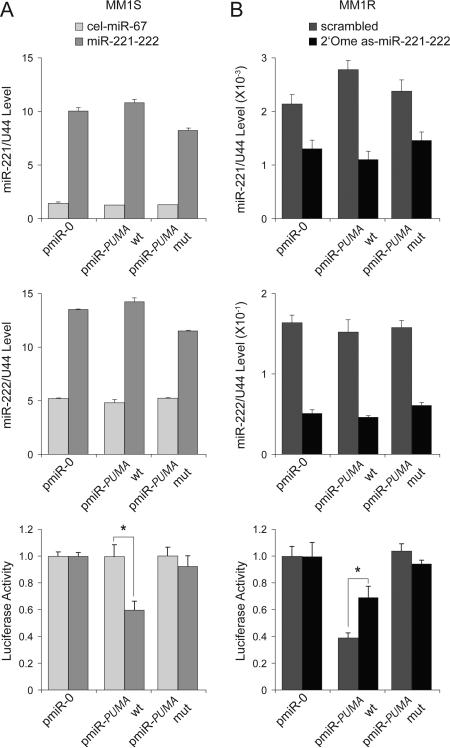
(A) Q-RT-PCR analysis of miR-221 (top) and miR-222 (middle) expression as well as reporter activity (bottom) in MM1S cells mock-transfected (cel-miR-67) or transfected with miR-221-222. Wild-type (wt), but not mutant (mut), pmiR-PUMA 3′UTR reporter activity was inhibited by miR-221-222. * p<0.05. (B) Q-RT-PCR analysis of miR-221 (top) and miR-222 (middle) expression as well as reporter activity (bottom) in MM1R cells mock-transfected (scrambled mRNA) or transfected with 2’-OMe as-miR-221-222. Wild-type, but not mutant, pmiR-PUMA 3′UTR reporter activity was increased by knockdown of miR-221-222. * p<0.05.
Since the AGO2/Dicer complex is known to recruit functional miRNAs (22), we performed the AGO2 pull-down RNA Q-RT-PCR assay to further evaluate whether PUMA mRNA is the target of miR-221-222 in MM cells. As expected from our previous studies (Fig. 4), after ectopic upregulation of miR-221 and miR-222 expression in MM1S cells and transduction with V-miR-221-222-GFP, there was significant downregulation of PUMA mRNA in comparison with MM1S cells tranduced with control V-GFP (Fig S2A). In addition, we observed that miR-221-222 and PUMA mRNA levels increased in pulled-down AGO2 complexes from MM1S cells transduced with V-miR-221-222-GFP (Fig S2B), indicating that upregulation of miR-221-222 recruits PUMA mRNA to the AGO2/Dicer complex.

Q-RT-PCR analysis of miR-221 (A) and miR-222 (B) expression in MM1S cells lentivirally transduced with empty vector (V-GFP) or V-miR-221-222-GFP. ** p<0.01. Analysis of PUMA expression at the mRNA (C) and protein (D) level in MM1S cells lentivirally transduced with empty vector (V-GFP) or with V-miR-221-222-GFP. * p<0.05. (E) Immunoblot analysis of PARP and caspase 3 activation in MM1S cells lentivirally transduced with empty vector (V-GFP) or with V-miR-221-222-GFP, in the presence or absence of Dex. (F) Viability assay of MM1S cells lentivirally transduced with empty vector (V-GFP) or with V-miR-221-222-GFP after treatment with Dex. ** p<0.01. (G) Immunocytochemical analysis of cleaved caspase-3 expression in MM1S cells lentivirally transduced with empty vector (V-GFP) or with V-miR-221-222V-GFP, then treated with Dex or left untreated.
miR-221-222 downregulates both PUMA mRNA and protein expression in MM1S cells in vitro
To examine whether miR-221 and miR-222 could induce Dex resistance in MM cells, MM1S cells were lentivirally transduced with vectors expressing GFP alone (V-GFP) or GFP and miR-221-222 (V-miR-221-222-GFP). After flow cytometric sorting of GFP-positive cells, stably-transduced MM1S V-miR-221-222-GFP and MM1S V-GFP cell lines were expanded, and expression of both miR-221 and miR-222 was verified by Q-RT-PCR (Fig 4A and B). PUMA mRNA expression and protein levels were also evaluated by Q-RT-PCR (Fig 4C) and immunoblot analysis (Fig 4D), respectively. Most importantly, ectopic expression of miR-221-222 can render MM1S cells resistant to Dex as documented by PARP and caspase 3 activation detected by western blot analysis (Fig 4E), as well as by viability assays (Fig 4F). Stably-transduced cells were also examined by immunohistochemical analysis to assess the extent of apoptosis in V-miR-221-222-GFP cells in the absence or presence of Dex. As shown in Fig 4G, apoptosis as evaluated by cleaved caspase 3 expression, decreased in MM1S cells lentivirally transduced with V-miR-221-222-GFP versus V-GFP, especially after treatment with Dex.
Knockdown of miR-221 and miR-222 in MM1R cells partially restores PUMA expression and Dex sensitivity in vitro
To evaluate the therapeutic role of miR-221-222 in vivo, we next generated sponge lentivirus in order to obtain long-lasting knockdown of mature miR-221 and miR-222 expression in vivo. We transduced MM1R cells with either V-GFP as a control or anti-sense miR-221-222 (V-as-miR-221-222-GFP) and assessed knockdown efficiency by Q-RT-PCR and western blot analysis. As shown in Figs 5A and B, expression of both mature miR-221 and mature miR-222 was efficiently knocked down. PUMA expression was found to be restored in V-as-miR-221-222-GFP cells as compared with stably V-GFP-transduced MM1R cells checked by Q-RT-PCR (Fig 5C) as well as immunoblot (Fig 5D) analysis. Most importantly, knockdown of miR-221-222 was able to re-sensitize MM1R cells to Dex as assesed by apoptosis (Figs 5E and G) and viability (Fig 5F) assays. Stably-transduced cells were also examined by immunohistochemical analysis to confirm restoration of PUMA expression in V-as-miR-221-222-GFP MM1R cells, and to document apoptosis in the absence or presence of Dex. As shown in Fig 5G, cleaved caspase 3 immunostaining for apoptosis was increased in MM1R cells lentivirally transduced with V-as-miR-221-222-GFP versus V-GFP-transduced controls, especially after treatment with Dex.

Q-RT-PCR analysis of miR-221 (A) and miR-222 (B) expression in MM1R cells lentivirally transduced with control vector (V-GFP) or V-as-miR-221-222-GFP vector. ** p<0.01. Analysis of PUMA expression at mRNA (C) and protein (D) levels in V-GFP and V-as-miR-221-222-GFP MM1R cells. * p<0.05. (E) Immunoblot analysis of PARP and caspase 3 activation in MMR1 cells virally transduced with V-GFP or V-as-miR-221-222-GFP and treated with Dex or left untreated. (F) Viability assay of MMR1 cells transduced with V-GFP and V-as-miR-221-222-GFP MM1R cells and treated with Dex. * p<0.05. (G) Immunocytochemical analysis of PUMA and cleaved caspase 3 expression in V-GFP and and 2’-OMe modified anti-miR-221-222-GFP MM1R cells treated with Dex or left untreated.
miR-221-222 induces Dex resistance in vivo
To further confirm the role of miR-221-222 in promoting Dex resistance in vivo, MM1S cells stably transduced with V-GFP or V-miR-221-222-GFP, as well as MM1R cells stably transduced with V-GFP or V-as-miR-221-222-GFP, were injected via the tail vein in our mouse model of MM dissemination (12). As in our in vitro studies showing that MM1S V-miR-221-222-GFP cells became Dex-resistant (Fig 4), mice injected with MM1S V-miR-221-222-GFP cells and treated with Dex were observed to have shorter survival times than control mice injected with MM1S V-GFP cells (Fig 6A). The levels of miR-221 (Fig S3A), miR-222 (Fig S3B) and PUMA (Fig S3C) in excised GFP-positive tumor xenografts were analyzed by Q-RT-PCR. Since PUMA is a proapoptotic factor that may be involved in other drug responses, we evaluated whether miR-221-222 could enhance resistance to other drugs used in MM. Interestingly, we found that overexpresion of miR-221-222 increased MM1S cell survival after treatment with valcade, lenalidomide, melphalan and doxorubicin (Fig S4).
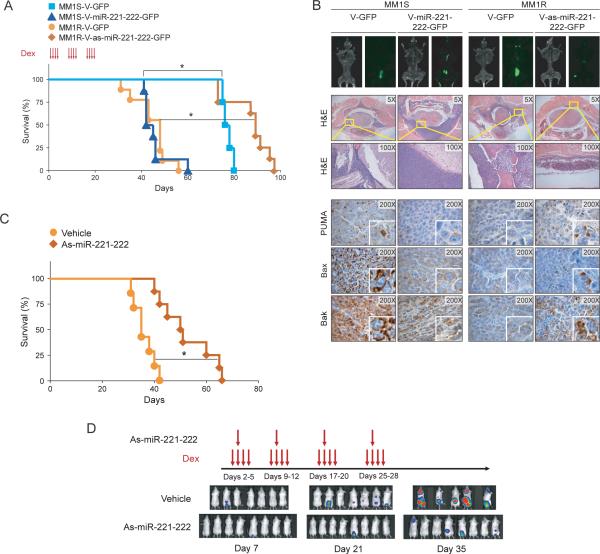
(A) Kaplan-Meier survival plots of mice injected via the tail vein with lentivirally transduced MM1S V-GFP, MM1S V-miR-221-222-GFP, MM1R V-GFP, or MM1R V-as-miR-221-222-GFP cells and treated with Dex (red arrows). * p<0.05. (B) Tumor burden at day 30 after tumor injection of mice, as evaluated by whole-body flurorescence imaging (top); standard histology (midle); and immunohistochemical (bottom) analysis of PUMA, BAX and BAK expression in GFP-positive tumors. As-miR-221-222 treatment increases survival and decreases tumor burden in MM1R-Luc-GFP bearing mice. (C) Kaplan-Meier survival plots of mice treated with vehicle or with 2’-OMe modified as-miR-221-222 plus Dex (red arrows) tail vein injection of MM1R-Luc-GFP cells. * p<0.05. (D) Xenogen images of vehicle and as-miR-221-222 treated mice at different time points (7, 21 and 35 days) after vein tail injection of cells.
As-miR-221-222 abrogate Dex resistance of MM1R cells in vivo
In contrast to preceding experiment, mice transplanted with MM1R V-as-miR-221-222-GFP cells and treated with Dex showed increased survival when compared with control mice transplanted with MM1R V-GFP cells (Fig 6A). These changes in survival were associated with a lower tumor burden in mice transplanted with V-as-miR-2221-221-GFP cells than in mice transplanted with V-GFP cells as evaluated by whole-body imaging (Fig 6B, top) as well as histological examination (Fig 6B, middle). We also observed that downstream target genes of miR-221-222, including PUMA, BAK and BAX, were downregulated in V-miR-221-222-GFP MM1S tumors and up regulated in V-as-miR-221-222-GFP MM1R tumors in vivo (Fig 6B, bottom). These results demonstrated that low miR-221-222 expression sensitized the MM1R cells to Dex-induced growth arrest and apoptosis, thereby prolonging survival in tumor-bearing mice.
As-miR-221-222 treatment increases survival of MM1R cells in vivo
We next performed a mouse xenograft experiment to evaluate the therapeutic effect of in vivo delivery of 2’-OMe modified antisense-miR-221-222 (as-miR-221-222). We evaluated tumor growth in mice transplanted with MM1R-luc-GFP cells after intraperitoneal delivery of as-miR-221-222 using lipid nanoparticles in combination with Dex. As shown in Fig. 6C, survival increased in mice treated with as-miR-221-222 compared with mice treated with vehicle alone (52.4±10.2 days vs 36.1±4.1 days, p<0.05.), and was associated with decreased tumor burden as evaluated by Xenogen imaging (Fig 6D).
High levels of miR-221-222 expression correlates with low levels of PUMA expression in MM patient samples
The above results prompted us to investigate the relationship between miR-221-222 and PUMA mRNA levels in a large set of MM cells from patients to assess the role of these molecules in drug resistance more broadly. We used published data sets GSE16558 (23) for which both miRNA and mRNA gene expression profiling were available. Interestingly, this analysis showed a significant (p=0.001) and inverse relationship between miR-221 and PUMA mRNA expression (Fig 7A). We further investigated this relationship using Q-RT-PCR in eighteen MM patients for whom clinical information, mRNA samples, and bone marrow biopsies were available (Supplementary Table S1). Of these, eight were from untreated, newly diagnosed patients, and ten were from patients with relapsed refractory MM. As shown in Fig 7B, higher levels of miR-221 with corresponding lower levels of PUMA mRNA were observed in MM cells from relapsed refractory versus newly diagnosed patient. In the case of miR-222 and PUMA mRNA levels, this inverse correlation was less evident; however, samples from two refractory patients had relatively high levels of miR-222 expression with lower levels of PUMA mRNA expression. One sample (MM2) had high levels of both miR-221 and miR-222. To further investigate the relationship between miR-221-222 and the downstream targets PUMA, Bak and Bax, we performed LNA-ISH and immunohistochemical stains, respectively, on BM biopsies. Interestingly, an inverse correlation was observed between miR-221-222 expression and PUMA, BAK and BAX expression. Two representative cases, a newly diagnosed MM (MM2) and a refractory MM (MM10), are show in Fig 7C. Taken together, the results revealed higher levels of miR-221 and/or miR-222 expression with corresponding lower levels of PUMA expression in a subset of samples from refractory MM patients as compared with those from untreated, newly diagnosed MM. Therefore, the miR-221-222 cluster, by regulating expression of the pro-apoptotic regulator PUMA, may more broadly mediate drug resistance leading to disease progression in MM.
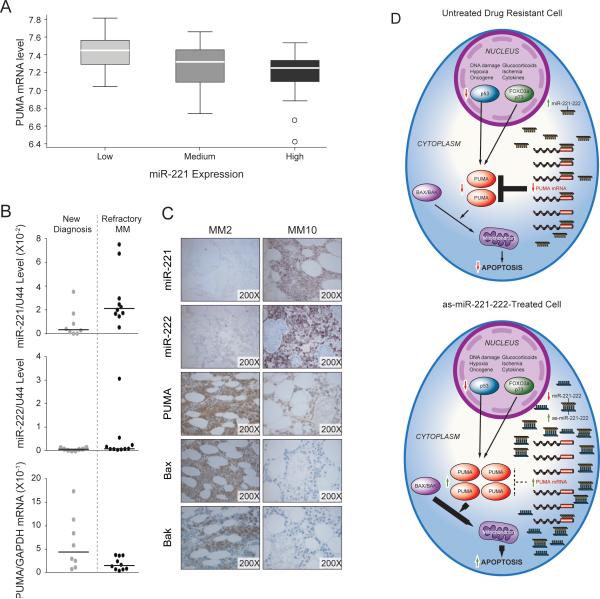
(A) PUMA mRNA expression is inversely correlated with miR-221 expression in MM cells; analysis based on published data set GSE16558 (p = 0.001). (B) miR-221-222 levels are associated with lower PUMA mRNA levels in MM cells from patients with refractory disease. (C) Inverse correlation between miR-221-222 and PUMA protein expression by LNA-ISH and immunohistochemical analysis of BM biopsies from MM patients with newly diagnosed or refractory disease. Two representative cases are shown, new (MM2) and refractory myeloma (MM10). (D) Schematic model for upregulation of the pro-apoptotic protein PUMA by anti-miR-221-222 therapy in MM. Anti-mR-221-222 therapy, by increasing the level of PUMA, which is downstream of GR and p53, may abrogate drug reistance associated with p53 inactivation and decreased GR expression.
DISCUSSION
Disease progression in MM is due partly to development of drug resistance. Dex is an effective therapeutic agent against MM; however, resistance eventually develops, signaling the arrival of unwelcome relapse. Previous studies have shown that tumor cells from steroid-resistant MM patients have lower expression of the GR than those from steroid-sensitive patients, but the molecular mechanisms for clinical Dex resistance remain poorly understood. In this study, we offer the first evidence for a role of the miR-221-222 cluster in mediating Dex resistance and disease progression in MM. Importantly we provide compelling preclinical proof-of-concept experiments setting the stage for a novel anti-sense pharmacologic strategy to abrogate Dex resistance specifically linked to enhanced expression of the miR-221-222 cluster that downregulates PUMA expression. The latter is a Bcl-2 homology 3 (BH3)-only Bcl-2 family member, and a critical mediator of p53-dependent and –independent (FOXO3a and p73) apoptosis induced by a wide variety of stimuli, such as: genotoxic stress, deregulated oncogene expression, toxins, altered redox status, growth factor/cytokine withdrawal and infection while at the same time triggering drug resistance (24). Our experiments show that direct targeting of the miR-221-222 cluster can abrogate this general mechanism of drug resistance, and because its target PUMA is a downstream regulator of apoptosis, it is likely that as-miR-221-222 therapy should not only overcome Dex resistance but also resistance to other drugs, including novel agents such as Lenolinamide, as well as p-53-dependent and -independent mechanisms of drug resistance (Fig 7D).
miR-221 and miR-222 are highly homologous miRNAs encoded as a cluster from a genomic region on the X-chromosome (9). They are widely overexpressed and involved in the pathogenesis of many human cancers including thyroid papillary carcinoma (25), glioblastoma (26), colorectal (27), lung (28), pancreas (29), ovarian (30), breast (17), gastric (31), liver (32), and, chronic lymphocytic leukemia (33), and lymphoma (34). Accumulating in vitro evidence implicates the miR-221-222 cluster as an oncogene that bypasses cell quiescence and increases the survival (35), proliferation (36) and metastatic potential of cancer cells (37). This cluster has also been shown to promote oncogenesis via downregulation of several tumor suppressor proteins including p27 (38,39), p57 (35), PTEN (31), PUMA (40), BIM (11), TIMP3 (41), and many others (26,42). We and others have also found that miR-221-222 is highly expressed in MM (10,43), and we first time found that miR-221-222 targets PUMA in multiple myeloma cells. However, in the referred study (44), it was found that PTEN, BIM, p27 and p57 were also targets of miR-221-222 in other MM cell lines, indicating that the target of these miRNAs are cell type-specific and/or dependent on the physiological or pathological state of the cells.
Our studies further indicate that inhibition of miR-221-222 via anti-sense therapy offers an enticing approach by which to overcome drug resistance more broadly. In Dex resistance as it operates in the MM1S/MM1R cellular model, the target PUMA is downstream of the GR. Therefore, downregulated expression of PUMA mRNA via as-miR-221-222 therapy could trigger apoptosis independently of the status of the GR receptor. In addition, p53 mutations, which are highly recurrent in MM, and are associated with primary drug resistance (45), as-miR-221-222 treatment may abrogate drug resistance and promote apoptosis even in the presence of p53 mutation by restore the expression of PUMA mRNA. Furthermore, our studies documenting higher levels of miR-221-222 in refractory than in newly diagnosed MM patients suggest that as-miR221-222 therapy may prevent, or at least delay, Dex resistance in particular and perhaps resistance more broadly in multiple drugs such as valcade, lenalidomide, melphalan and doxorubicin. With regard to the possiblity of overcoming MM resistance to Lenolinamide (46) using as-miR-221-22 therapy, it is worth mentioning that this effect is also expected to take place by regulating PUMA levels and apoptosis rather than regulating the levels of Cerebron (CRBN) and the binding proteins (IKZF-1 and IKZF-3) as no consensus bindings sites for miR-221-222 are present in the 3′ UTR of CRBN mRNA (data not shown). Although our studies indicate that as-miR-221-222 could be used as a therapy to overcome drug resistance in MM patients, additional preclinical studies will be needed to further validate this hypotheis. In addition, these studies should include the development of better delivery systems that could provide tumor specific uptake of miRNAs and avoid off-target effects.
Finally, miR-221-222 expression may be a useful prognostic marker in diffuse large B-cell lymphoma (DLBCL) (47), papillary thyroid carcinoma (48), and lung cancer (49). In light of our findings, use of these miRNAs to predict prognosis and clinical response to Dex and/or other drugs warrants similar evaluation in patients with MM.
Supplementary Material
1
2
3
4
5
6
Acknowledgements
Grant support: J.J.Z is supported by a Multiple Myeloma Research Foundation (MMRF) research fellow award and by a Pathway to Independence Award (1K99CA172292) from the National Institutes of Health (NIH). T.N.C is supported by a Multiple Myeloma Research Foundation (MMRF) research fellow award. Z.B.C and Z.W. is supported by a China Scholarship Council (CSC) award. D.R.C. is supported by a MMRF senior award from the Doctors Cancer Foundation and a research grant (1R01CA151391) from the National Institutes of Health (NIH).
Footnotes
Disclosure of Potential Conflicts of Interest
No potential conflicts of interest were disclosed.
Authorship contributions
J.J.Z initiated, designed and performed experiments, analyzed data, and wrote the paper; Z.B.C., Y.H,. X.W., J.L., M.C., and T.N.C performed experiments and analyzed data; Y.T.T, N.C.M., and Y.H provided clinical samples. J.C. contributed experimental reagents. A.L., K.C.A. critically reviewed the paper. R.D.C. initiated the project, analyzed data, and wrote the paper.
References
Full text links
Read article at publisher's site: https://doi.org/10.1158/0008-5472.can-15-0457
Read article for free, from open access legal sources, via Unpaywall:
https://cancerres.aacrjournals.org/content/canres/75/20/4384.full.pdf
Citations & impact
Impact metrics
Citations of article over time
Alternative metrics
Smart citations by scite.ai
Explore citation contexts and check if this article has been
supported or disputed.
https://scite.ai/reports/10.1158/0008-5472.can-15-0457
Article citations
An overview of multiple myeloma: A monoclonal plasma cell malignancy's diagnosis, management, and treatment modalities.
Saudi J Biol Sci, 31(2):103920, 30 Dec 2023
Cited by: 0 articles | PMID: 38283805 | PMCID: PMC10818257
Review Free full text in Europe PMC
MicroRNA-Mediated Regulation of Histone-Modifying Enzymes in Cancer: Mechanisms and Therapeutic Implications.
Biomolecules, 13(11):1590, 28 Oct 2023
Cited by: 5 articles | PMID: 38002272
Review
G6PD promotes cell proliferation and dexamethasone resistance in multiple myeloma via increasing anti-oxidant production and activating Wnt/β-catenin pathway.
Exp Hematol Oncol, 11(1):77, 21 Oct 2022
Cited by: 10 articles | PMID: 36271440 | PMCID: PMC9587560
Role of microRNAs and long non‑coding RNAs in glucocorticoid signaling (Review).
Int J Mol Med, 50(6):147, 11 Nov 2022
Cited by: 2 articles | PMID: 36367164 | PMCID: PMC9662139
Review Free full text in Europe PMC
A domino-like localized cascade toehold assembly amplification-based DNA nanowire for microRNA imaging in living cells.
Chem Sci, 13(48):14373-14381, 23 Nov 2022
Cited by: 2 articles | PMID: 36545151 | PMCID: PMC9749110
Go to all (55) article citations
Data
Data behind the article
This data has been text mined from the article, or deposited into data resources.
BioStudies: supplemental material and supporting data
Similar Articles
To arrive at the top five similar articles we use a word-weighted algorithm to compare words from the Title and Abstract of each citation.
Ectopic microRNA-150-5p transcription sensitizes glucocorticoid therapy response in MM1S multiple myeloma cells but fails to overcome hormone therapy resistance in MM1R cells.
PLoS One, 9(12):e113842, 04 Dec 2014
Cited by: 24 articles | PMID: 25474406 | PMCID: PMC4256227
MiR-222 targeted PUMA to improve sensitization of UM1 cells to cisplatin.
Int J Mol Sci, 15(12):22128-22141, 02 Dec 2014
Cited by: 19 articles | PMID: 25474084 | PMCID: PMC4284698
Study on the Association Between miRNA-202 Expression and Drug Sensitivity in Multiple Myeloma Cells.
Pathol Oncol Res, 22(3):531-539, 21 Dec 2015
Cited by: 17 articles | PMID: 26689580
Role of micro-RNAs in drug resistance of multiple myeloma.
Oncotarget, 7(37):60723-60735, 01 Sep 2016
Cited by: 30 articles | PMID: 27494872 | PMCID: PMC5312415
Review Free full text in Europe PMC
Funding
Funders who supported this work.
NCI NIH HHS (5)
Grant ID: 1R01CA151391
Grant ID: K99 CA172292
Grant ID: P50 CA100707
Grant ID: R00 CA172292
Grant ID: R01 CA151391