Abstract
Purpose
The urothelium is a frontline sensor of the lower urinary tract, sampling the bladder lumen and stimulating an immune response to infectious and noxious agents. Pattern recognition receptors (PRRs) recognize such agents and coordinate the innate response, often by forming inflammasomes that activate caspase-1 and the release of interleukin-1. We have shown the presence of one PRR (NLRP3) in the urothelia and its central role in the inflammatory response to cyclophosphamide. The purpose of this study was to (1) assess the likely range of the PPR response by assessing the repertoire present in the rat bladder and (2) determine the utility of the MYP3 rat urothelia cell line for in vitro studies by assessing its PPR repertoire and functional responsiveness.Methods
Immunohistochemistry was performed for seven PPRs (NLRP1, NLRP3, NLRP6, NLRP7, NLRP12, NLRC4 and AIM2) on bladder sections and MYP3 cells. For functionality, MYP3 cells were challenged with the quintessential NLRP3 activator ATP and assessed for caspase-1 activation.Results
All PPRs examined were expressed in the bladder and localized to the urothelial layer with several also in the detrusor (none in the interstitia). MYP3 cells also expressed all PRRs with a variable intracellular location. ATP-stimulated caspase-1 activity in MYP3 cells in a dose-dependent manner was reduced by knockdown of NLRP3 expression.Conclusion
The results suggest that the bladder possesses the capacity to initiate an innate immune response to a wide array of uropathological agents and the MYP3 cells will provide an excellent investigational tool for this field.Free full text

The Potential Repertoire of the Innate Immune System in the Bladder: Expression of Pattern Recognition Receptors in the Rat Bladder and a Rat Urothelial Cell Line (MYP3 cells)
Abstract
Purpose
The urothelium is a frontline sensor of the lower urinary tract, sampling the bladder lumen and stimulating an immune response to infectious and noxious agents. Pattern recognition receptors (PRRs) recognize such agents and coordinate the innate response, often by forming inflammasomes that activate caspase-1 and the release of Interleukin-1β. We have shown the presence of one PRR (NLRP3) in the urothelia and its central role in the inflammatory response to cyclophosphamide. The purpose of this study was to 1) assess the likely range of the PPR response by assessing the repertior present in the rat bladder and 2) determine the utility of the MYP3 rat urothelia cell line for in vitro studies by assessing it’s PPR repertior and functional responsiveness.
Methods
Immunohistochemistry was performed for seven PPRs (NLRP1, NLRP3, NLRP6, NLRP7, NLRP12, NLRC4 and AIM2) on bladder sections and MYP3 cells. For functionality, MYP3 cells were challanged with the quinessential NLRP3 activator ATP and assessed for caspase-1 activation.
Results
All PPRs examined were expressed in the bladder and localized to the urothelial layer with several also in the detrusor (none in the interstitia). MYP3 cells also expressed all PRRs with a variable intracellular location. ATP stimulated caspase-1 activity in MYP3 cells in a dose-dependent manner that was reduced by knockdown of NLRP3 expression.
Conclusion
The results suggest that the bladder possesses the capacity to initiate an innate immune response to a wide array of uropathological agents and the MYP3 cells will provide an excellent investigational tool for this field.
Introduction
Classically the bladder urothelium was thought to serve as a passive barrier, separating the underlying tissue from harmful chemicals present in the urine. However, numerous studies have left little doubt that the urothelium is a highly engaged and interacting tissue that senses the microenvironment and responds by communicating via autocrine and paracrine pathways (1). While the urothelia may respond to many agents, there is particular importance in how it recognizes and responds to uropathogens that result in urinary tract infections (UTIs) such as E. Coli.
UTIs account for 6 to 7 million hospital visits per year (2, 3) and are second only to the common cold as the most prevalent form of human infection. This equates to a societal cost of over $3.5 billion US dollars per annum (4, 5) with females being disproportionately affected. Antibiotics have been the mainstay of UTI therapy for decades but the emergence of antibiotic resistance in first and second line therapies drives the need for novel treatments for these infections. The ability to use one’s own immune system to combat the invading uropathogens holds great promise as a therapeutic or even prophylactic strategy but currently there is insufficient information regarding how these bacteria are recognized and the mechanism of the immune response mounted against them.
The mammalian immune system consists of two major arms, the innate and the adaptive systems, both of which are known to be present and active in the urinary tract (6). Although the adaptive system possesses exquisite specificity, a considerable amount of time is required to mount a response and it is the innate system that first encounters invaders and initiates a defense. When encountering a pathogen the innate system does not recognize specific antigens but rather identify patterns in the nonself molecules known as Pathogen Associated Molecular Patterns or PAMPs. The receptors that recognize these PAMPs are known as Pattern Recognition Receptors or PRRs. Some of the PRRs also recognize patterns on molecules released from damaged or dying cells known as Damage (or Danger) Associated Molecular Patterns or DAMPs. In the latter case the resulting inflammatory response is known as sterile inflammation when it occurs in the absence of pathogens.
Based on functional and genetic relatedness, five classes of PRRs have been identified; Toll-like receptors (TLRs), Nod-like receptors (NLRs), C-type lectin receptors (CLRs), retinoic acid-inducible gene (RIG)-I-like receptors (RLRs), and absent in melanoma 2 (AIM2)-like receptors (ALRs) (7, 8). TLRs and CLRs are cell surface receptors that sample the extracellular milieu for PAMPs or DAMPs whereas the other PRRs are cytoplasmic and work to survey that compartment as well as cooperate with the cell surface receptors when a PAMP or DAMP is encountered. TLRs have been reported and extensively studied in the bladder urothelia for nearly 15 years and this work has shown that only 3 of the TLRs (TLR4, TLR5 and TLR11) contribute to the defense of the urinary tract (9). Given this rather large body of work, it is surprising that until recently no studies had reported the existence or functionality of any of the other PRRs in this tissue.
Although there are numerous interrelated pathways and mediators in the innate response mediated by PRRs, unquestionably one of the most important is the production of IL-1β a pro-inflammatory cytokine with a central role in the initiation and propagation of the inflammatory response. TLRs and NLRs have clear interdependent pathways that cooperate to produce this proinflammatory cytokine. When PAMPs are recognized by certain TLRs they trigger MAPK-dependent pathways that engage NF-κB and up-regulate production of pro-IL-1β. In a complementary manner, when certain NLRs are engaged by PAMPs (or in some cases DAMPs) they form a multimeric complex known as an inflammasome which results in the activation of caspase-1. Caspase-1 then cleaves pro-IL-1β to IL-1β which stimulates its release by a process known as pyroptosis. Caspase-1 can also cleave IL-18 (which is usually constitutively expressed) and both proinflammatory cytokines contribute to an inflammatory response to directly challenge the invading pathogen while recruiting the adaptive immune system into the full response. Consequently, TLRs and NLRs often work together to mount the innate immune response to invading pathogens.
As stated above, numerous studies have documented TLRs in the urothelia. However, there have only been two reports on the presence of any other PRR in this tissue. In an early tissue survey, Kummer et al. demonstrated that NLRP3, by far the best studied member of the NLR family, was expressed in the rat bladder (10). In a recent study, we confirmed the presence of NLRP3 in the rat bladder as well as documenting expression of NLRC4 and the associated molecules ASC and NAIP in the urothelia (11). ASC is an adaptor that bridges NLRP3 to caspase-1 while NAIP provides ligand specificity to NLRC4. We also demonstrated that these receptors formed active inflammasomes when the appropriate ligands were placed into the lumen of the bladder. Taking advantage of the FDA-approved NLRP3 inhibitor, glyburide, we were able to further show that the NLRP3 inflammsome played a critical role in the induction of inflammation and voiding pathology in a chemical-induced model of sterile inflammation. Thus, having established the importance of at least one NLR in the bladder, the next logical step was to ascertain what other NLR/PPRs are expressed in the urothelia.
We have restricted the current study to an analysis of the NLRs that form inflammasomes (often called “inflammasome sensors” (12). We have also included AIM2 because 1) it is the only “inflammasome sensor” that does not belong to the NLR family 2) it functions in many ways like an NLR and 3) it has domains similar to an NLR. Moreover, it responds to double stranded DNA in the cytosol and thus may be the sensor for invading viruses in addition to bacteria. Finally, we have repeated this analysis in an immortalized nontumorigenic rat urothelial cell line (MYP3) and assessed the ability to activate the innate immune response in these cells in vitro.
Materials and Methods
Antibodies
Table 1 lists the various primary antibodies used. All antibodies cross-reacted with their respective rat antigen. Isotype controls were obtained from Santa Cruz (Rabbit; SC-2027) and Invitrogen (Goat; 02-6202). All antibodies were used at a 1:200 dilution. Biotinylated secondary antibody was provided with the Vectastain ABC staining kits (Vector Labs, Burlingame, CA) and used as suggested.
Table 1
Antibodies used in the study. The target of each antibody is indicated along with its host, source (i.e. sequence used to originally produce the antibody), the company it was obtained from and the catalogue number. All antibodies cross-reacted with the rat-derived antigen
Target | Host | Source | Company | Catalog # |
---|---|---|---|---|
NLRP1 | Rabbit | Mouse | Santa Cruz | SC-66993 |
NLRP3 | Rabbit | Human | Santa Cruz | SC-66846 |
NLRP6 | Goat | Mouse | Santa Cruz | SC-50634 |
NLRP7 | Rabbit | Human | Sigma | SAB2900883 |
NLRP12 | Rabbit | Human | Santa Cruz | SC-00175 |
NLRC4 | Rabbit | Human | Aviva | AVARP00019 |
AIM2 | Rabbit | Mouse | Santa Cruz | SC-137967 |
Bladder methods
Animals
All animals were female Sprague Dawley Rats of approximately 200 g, and were purchased from Harlan (Frederick, MD, USA). Animals were acclimated (2 per cage) for at least 7 days in an AAALAC-approved facility with a 12:12 light-dark cycle and provided with food and water ad libitum. All experimental procedures were reviewed and approved by the Institutional Animal Care and Use Committee (IACUC) at the Medical University of South Carolina and were performed in accordance with the guidelines set forth in the NIH Guide for the Care and Use of Laboratory Animals, published by the Public Health Service of the USA.
Immunocytochemistry
Bladders were dissected, cleaned of surrounding fat and placed in 10% neutral buffered formalin overnight at 4 °C. Bladders were then transferred to 70% ethanol and stored at 4 °C for 1 – 7 days until they were embedded in paraffin blocks using standard techniques. Cross-sections (5um) from approximately the middle of the bladder were used for staining and were deparaffinized and hydrated through a graded alcohol series before being subjected to antigen retrieval (Vector labs citrate-based antigen unmasking solution for 30 min using the manufacturers recommended protocol; Vector Labs, Burlingame, CA). Slides were then placed into 3% H2O2 for 10 min to quench endogenous peroxidase. Following washing in PBS, sections were blocked with normal goat serum for 30 min and incubated with primary antibodies or the appropriate isotype control for 1 h. Slides were washed and then biotinylated secondary antibody added (provided with the Vectastain ABC staining kits; Vector Labs, Burlingame, CA). Slides were incubated for 30 min, washed and incubated for an additional 30 min with the Vectastain ABC reagent. Following an additional wash, slides were incubated in DAB (3, 3'-diaminobenzidine) peroxidase substrate solution until signal developed. Slides were then counterstained with hematoxylin, coverslipped and analyzed by light microscopy.
MYP3 cell methods
Culture
MYP3 cells (an immortalized non-tumorigenic rat urothelial cell line) were originally developed by Dr. Ryoichi Oyasu (Northwestern University, Chicago, IL) (13) and generously provided to us by Samuel M. Cohen through the lab of Laura L. Arnold, both at the University of Nebraska Medical Center, Omaha NE (14, 15). The cells were cultured (5% CO2, 95% air) in Ham’s F-12K medium supplemented with 10% fetal bovine serum, 10 µM non-essential amino acids, 10 ng/ml EGF, 10 µg/ml insulin, 5 µg/ml transferrin, 6.7 ng/ml Selenium, 100 U/ml penicillin, 100 µg/ml streptomycin, 2.7 mg/ml glucose and 1 µg/ml hydrocortisone using standard cell culture procedures.
Immunohistochemistry
Cells were plated at 100,000 cells per well in 0.5 ml cell culture media in 8 well BD Falcon culture slides (Cat # 354118; BD Biosciences, Franklin Lakes, NJ) and incubated overnight. The media was then removed and the cells fixed with 10% neutral buffered formalin for 10 min at room temperature. Wells were then rinsed in 70% ethanol followed by PBS. Cells were then blocked, incubated with primary and secondary antibodies, the signal developed and the wells analysed exactly as described above for tissue sections.
Caspase-1 Assay
Caspase-1 activity was measured by a method modified from our previously published protocol (11). In these studies cells were plated in black-walled 96 well cell culture plates at 50,000 cells per well in 90 µl complete media containing 200 ng/ml LPS (Esherichia coli 055:B5; Calbiochem-EMD Millipore, Billerica). LPS was included to “prime” the cells according to well established protocols of NLRP3 activation (16, 17). Following an overnight incubation ATP concentration were prepared as 10X stocks in media and 10 µl added to the appropriate wells. One hour later media was removed and 50 µl of of 10 mM MgCl2, 0.25% Igepal CA-630 added. The plates were incubated 5 min at room temperature before the addition of 50 µl 40 mM Hepes (pH 7.4), 20 mM NaCl, 2 mM EDTA, 20% Glycerol. The cells were then frozen at −70 C until analyzed. Cells were thawed and combined with 50 µl assay buffer (25 mm HEPES, 5% sucrose, 0.05% CHAPS (pH 7.5), 10 µl 100 mM dithiothreitol (dissolved in assay buffer) and 20 µl 1 mM substrate (N-Acetyl-Tyr-Val-Ala-Asp-7-amino-4-trifluoromethylcoumarin; Ac-DEVD-AFC; prepared as a 100 mM stock in DMSO, then diluted to 1 mM with assay buffer). Plates were sealed with film (Axygen inc., Union City, CA), wrapped in aluminum foil and incubated 24 h at 37 oC with gentle shaking. Fluorescence (Excitation 400 nm, Emission 505 nm) was then measured.
Transection
For transient knockdown of NLRP3, custom shRNA vector were prepared by Origene (Rockville, MD). The vector sequences were A: CTAAGAAGGACCAGCCAGAGTG-GAATGAT; B: TCCTAGCCAGGAAGATTATGTTGGACTG-G; Scram: CCGACTGTAAGCTACA-GATGCTGGAGTTA. The vectors were cloned in a pGFP-C-shLenti plasmid under a U6 promoter and sequenced for verification. E.Coli were transformed and grown (and plasmids isolated) using standard techniques. MYP3 cells were transfected using Nanojuice transfection reagent (EMD, Gibbstown, NJ) according to the manufacturer’s recommendations. Cells were transfected with 1 µg of the indicated construct or 0.5 µg of A and B for the A+B group. Cells were then incubated 24 h in growth medium, before being trypisinized and replated for the caspase-1 assay. The remaining cells were used for real-time PCR and Western analysis.
Quantitative Real-Time PCR
RNA was isolated and reverse-transcribed using standard methods. Real-time PCR was performed with 5 µl of a 1:20 dilution of reverse transcribed cDNA using Roche probe qPCR master mix in a LightCycler480 (Roche, Nutley, NJ). The cycling conditions for all genes were performed according to the manufacturer’s instructions: annealing temperature was 60°C. Triplicate reactions were run for each cDNA sample. The relative expression of each gene was quantified on the basis of Ct value measured against an internal standard curve for each specific set of primers using the software provided by the instrument manufacturer (Roche). After testing to define the optimal housekeeping gene (results not shown), tissue data and cell line samples were normalized to beta-actin. Primer sequences and Roche probe number: NLRP3, probe 67, forward primer atgagggtgctgtgtgagg, reverse primer aacagcattgatgggtcagtc; Beta-actin, probe 69, forward primer tgccctagacttcgagcaag, reverse primer ggcagctcatagctcttctcc..
Western Blot
Proteins were isolated, subjected Western blot analysis using standard techniques. Membranes were probed with a 1:200 dilution of a NLRP3 antibody (Abcam cat# ab109314).
Statistical analysis
Caspase-1 activity was assessed by a one-way analysis of variance followed by a Tukey's post-hoc analysis using GraphPad InStat software (La Jolla, CA). Real-time PCR results were compared to the Scram group using unpaired t-test.
Results
Figure 1 depicts NLRP1 staining in the normal rat bladder (top panels) as well as in the MYP3 cell line (bottom panels). In the bladder, staining was restricted to three main tissue types, the urothelial layer (indicated by brackets), the detrusor muscle (filled arrow) and in endothelial cells lining vascular structures (arrowhead). No staining was present in the interstitial cells. While staining appeared to be throughout the urothelia, in many areas it was particularly concentrated in the outermost layer referred to as the umbrella layer (see area indicated by the box). MYP3 cells also displayed robust staining of NLRP1 (Fig. 1 lower panels). In these cells the intracellular distribution varied from cell to cell. Indeed, within the same visual field cells could be found in which NLRP1 staining was concentrated in the nucleus, mostly excluded from the nucleus or found in both compartments; as indicated by the key.
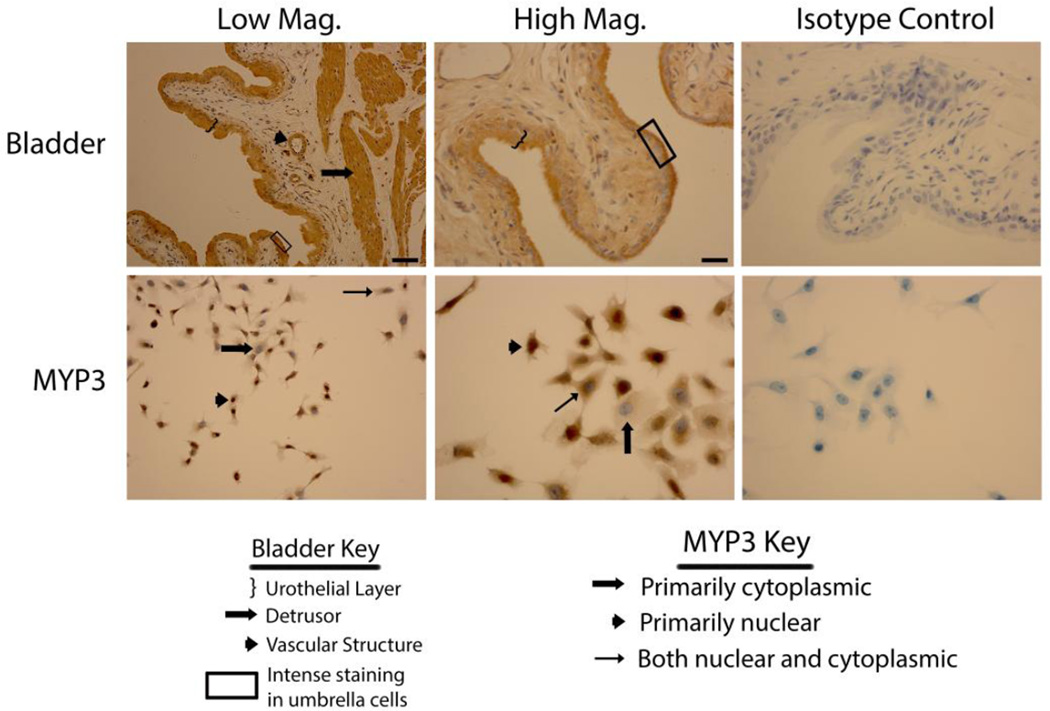
Staining of rat bladders and MYP3 cells for NLRP1. Upper Panels: Micrographs of bladder sections stained with NLRP1 (or isotype control) and photographed at low magnification (Low Mag) or high magnification (High Mag). Isotype controls are taken at the higher magnification. Lower panels: Micrographs of bladder sections stained with NLRP1 (or isotype control) and photographed at low magnification (Low Mag) or high magnification (High Mag). Isotype controls are taken at the higher magnification. Areas of interest are indicated by the key. Scale bar in the lower right corner of the top left Low Mag picture = 50 µm. Scale bar in the lower right corner of the top left High Mag picture = 25 µm.
When bladders were stained for NLRP3 (Fig. 2 upper panels) the staining appeared to be exclusively in the urothelial layer with little or no staining in the detrusor or endothelial cells. Some concentration of staining was apparent in the outermost layer of the urothelia, although this this was not as pronounced as with NLRP1. MYP3 cells also stained for NLRP3 (Fig. 2 lower panels) and displayed the same variable distribution patterns seen with NLRP1.
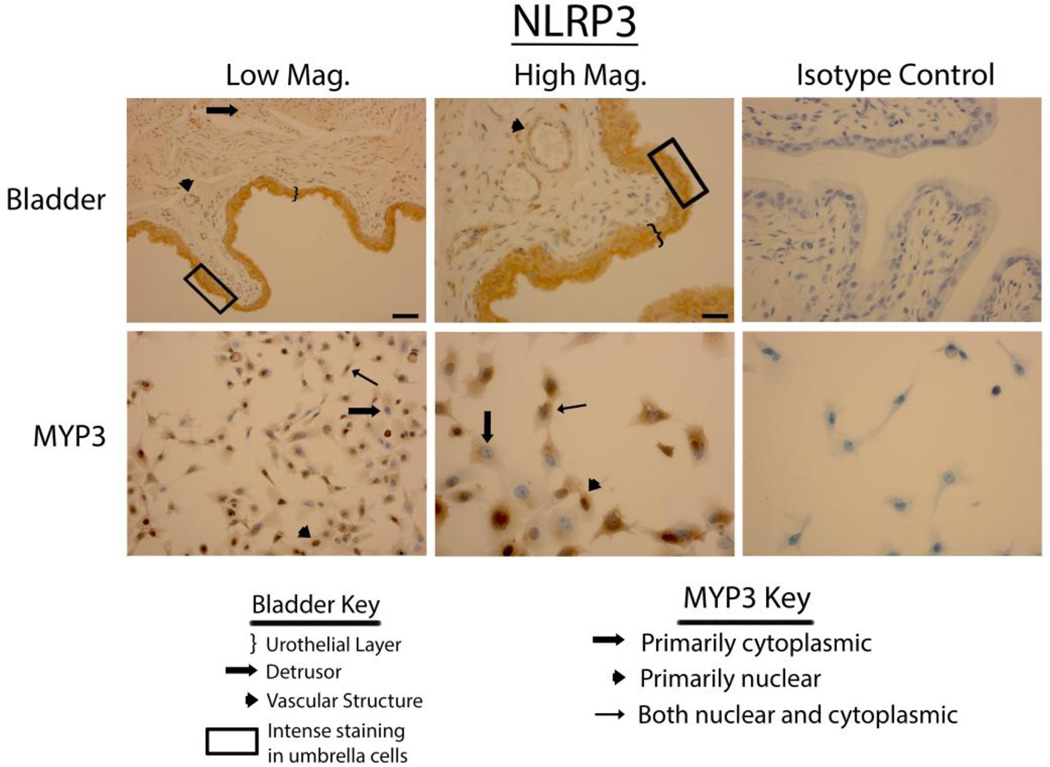
Staining of rat bladders and MYP3 cells for NLRP3. Upper Panels: Micrographs of bladder sections stained with NLRP3 (or isotype control) and photographed at low magnification (Low Mag) or high magnification (High Mag). Isotype controls are taken at the higher magnification. Lower panels: Micrographs of bladder sections stained with NLRP3 (or isotype control) and photographed at low magnification (Low Mag) or high magnification (High Mag). Isotype controls are taken at the higher magnification. Areas of interest are indicated by the key. Scale bar in the lower right corner of the top left Low Mag picture = 50 µm. Scale bar in the lower right corner of the top left
Bladders stained vigorously for NLRP6 (Fig. 3 upper panels), NLRP7 (Fig. 4 upper panels) and NLRP12 (Fig. 5 upper panels) and displayed a pattern virtually identical to that of NLRP1 (i.e. expressed in the urothelial layer, the detrusor muscle and vascular endothelial cells with little to no staining in the interstitial cells). Again, in the urothelial layer expression was often concentrated on the luminal edge. Likewise, expression of these three NLRs in the MYP3 cells (Fig. 3, ,44 and and5,5, lower panels) was concentrated in the nucleus, mostly excluded from the nucleus or found in both compartments. NLRC4 (Fig. 6 upper panels) was present in the bladder and concentrated in the urothelial layer with only light staining in the detrusor and vascular structures. Interestingly, distribution appeared fairly even across the urothelial layer and was not concentrated on the luminal surface. MYP3 cells also stained positive for this NLR (Fig. 6 lower panels) with the same varied pattern of distribution. Aim2 (Fig. 7) displayed the same pattern as the majority of NLRs (NLRP1, NLRP6, NLRP7 and NLRP12) in both the bladder (upper panels) and MYP3 cells (lower panels).
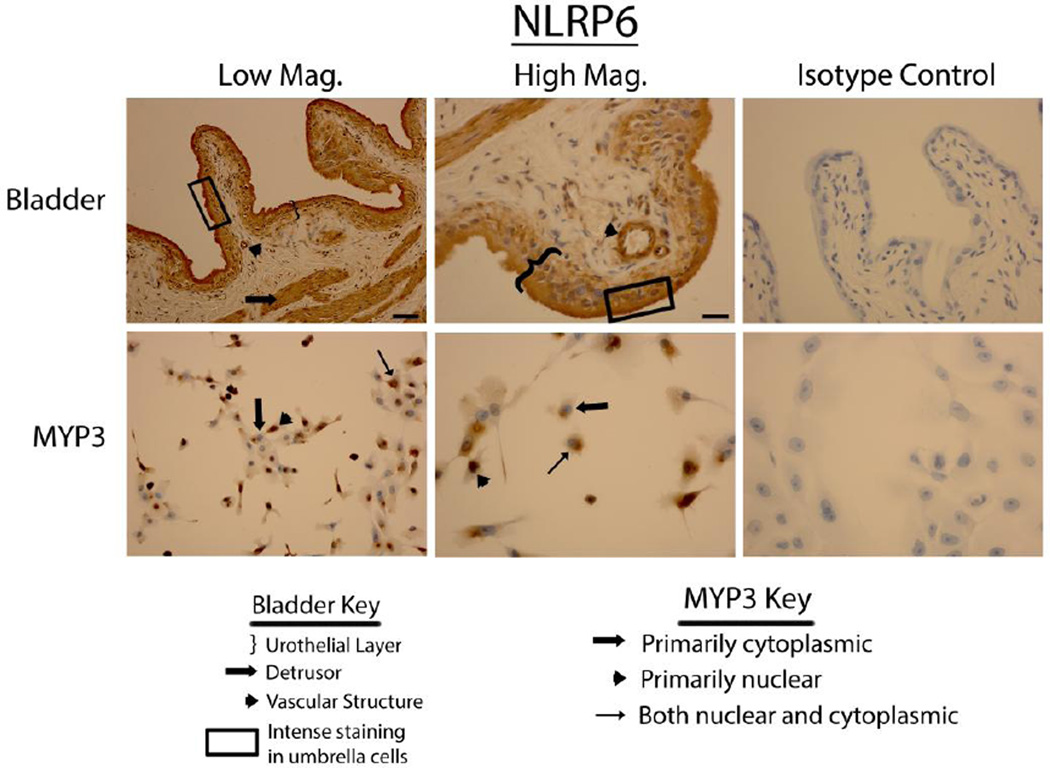
Staining of rat bladders and MYP3 cells for NLRP6. Upper Panels: Micrographs of bladder sections stained with NLRP6 (or isotype control) and photographed at low magnification (Low Mag) or high magnification (High Mag). Isotype controls are taken at the higher magnification. Lower panels: Micrographs of bladder sections stained with NLRP6 (or isotype control) and photographed at low magnification (Low Mag) or high magnification (High Mag). Isotype controls are taken at the higher magnification. Areas of interest are indicated by the key. Scale bar in the lower right corner of the top left Low Mag picture = 50 µm. Scale bar in the lower right corner of the top left High Mag picture = 25 µm
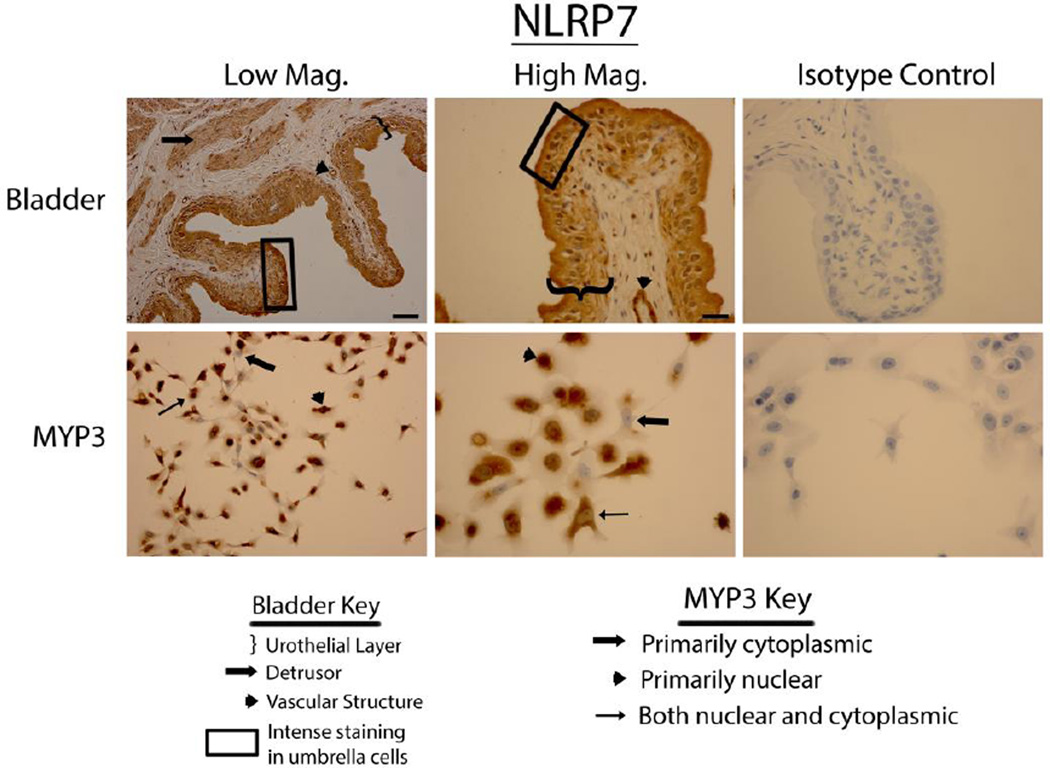
Staining of rat bladders and MYP3 cells for NLRP7. Upper Panels: Micrographs of bladder sections stained with NLRP7 (or isotype control) and photographed at low magnification (Low Mag) or high magnification (High Mag). Isotype controls are taken at the higher magnification. Lower panels: Micrographs of bladder sections stained with NLRP7 (or isotype control) and photographed at low magnification (Low Mag) or high magnification (High Mag). Isotype controls are taken at the higher magnification. Areas of interest are indicated by the key. Scale bar in the lower right corner of the top left Low Mag picture = 50 µm. Scale bar in the lower right corner of the top left High Mag picture = 25 µm
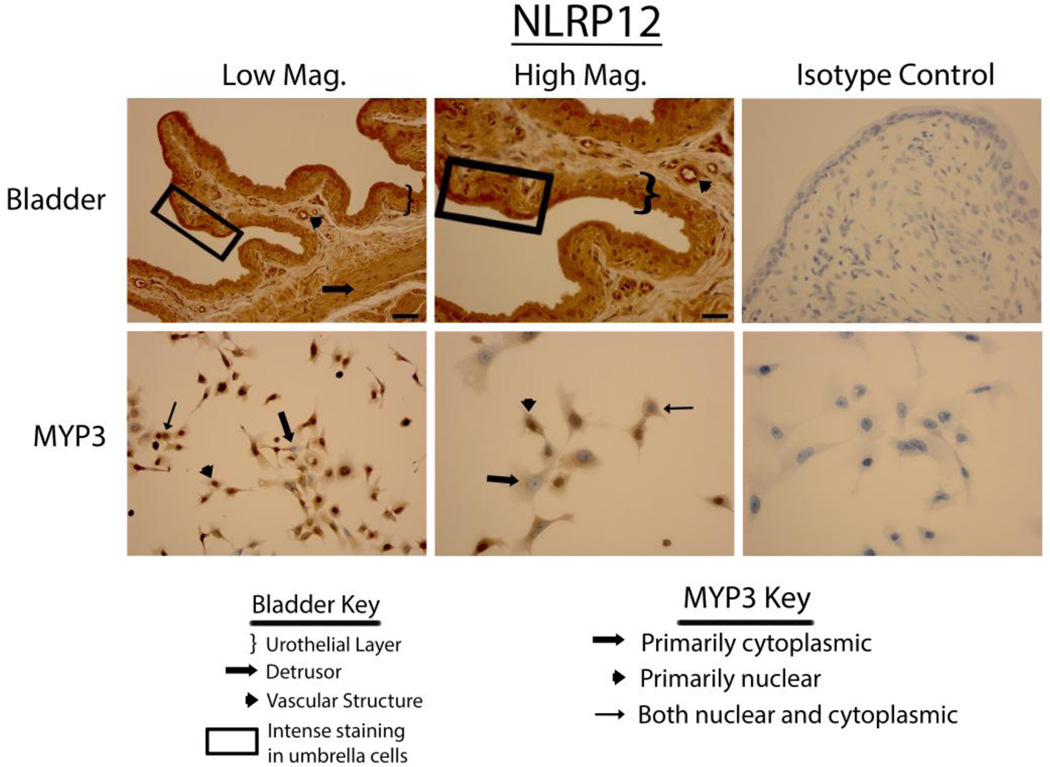
Staining of rat bladders and MYP3 cells for NLRP12. Upper Panels: Micrographs of bladder sections stained with NLRP12 (or isotype control) and photographed at low magnification (Low Mag) or high magnification (High Mag). Isotype controls are taken at the higher magnification. Lower panels: Micrographs of bladder sections stained with NLRP12 (or isotype control) and photographed at low magnification (Low Mag) or high magnification (High Mag). Isotype controls are taken at the higher magnification. Areas of interest are indicated by the key. Scale bar in the lower right corner of the top left Low Mag picture = 50 µm. Scale bar in the lower right corner of the top left High Mag picture = 25 µm
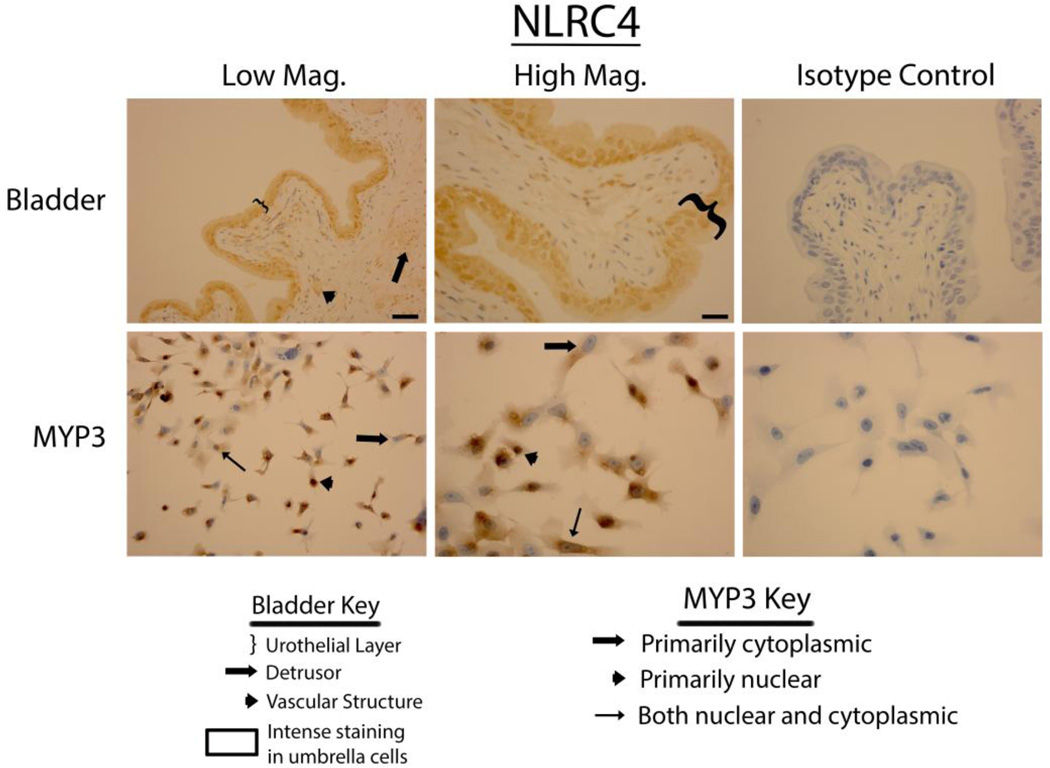
Staining of rat bladders and MYP3 cells for NLRC4. Upper Panels: Micrographs of bladder sections stained with NLRC4 (or isotype control) and photographed at low magnification (Low Mag) or high magnification (High Mag). Isotype controls are taken at the higher magnification. Lower panels: Micrographs of bladder sections stained with NLRC4 (or isotype control) and photographed at low magnification (Low Mag) or high magnification (High Mag). Isotype controls are taken at the higher magnification. Areas of interest are indicated by the key. Scale bar in the lower right corner of the top left Low Mag picture = 50 µm. Scale bar in the lower right corner of the top left High Mag picture = 25 µm
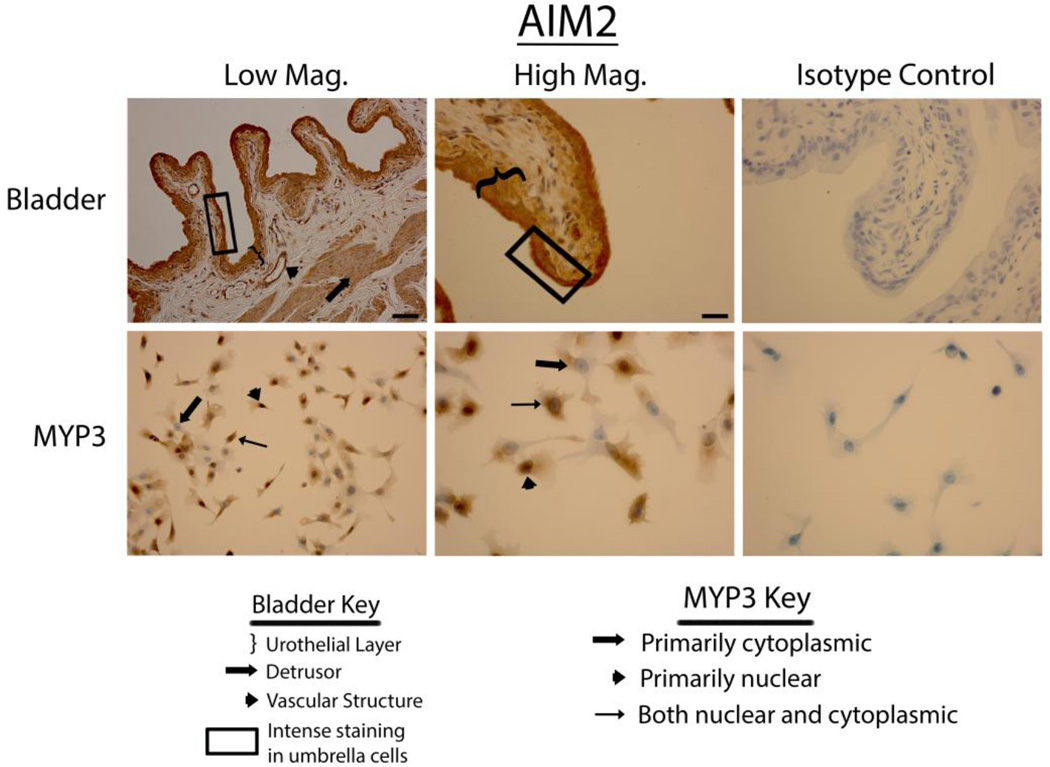
Staining of rat bladders and MYP3 cells for AIM2. Upper Panels: Micrographs of bladder sections stained with AIM2(or isotype control) and photographed at low magnification (Low Mag) or high magnification (High Mag). Isotype controls are taken at the higher magnification. Lower panels: Micrographs of bladder sections stained with AIM2 (or isotype control) and photographed at low magnification (Low Mag) or high magnification (High Mag). Isotype controls are taken at the higher magnification. Areas of interest are indicated by the key. Scale bar in the lower right corner of the top left Low Mag picture = 50 µm. Scale bar in the lower right corner of the top left High Mag picture = 25 µm
Our previous studies have demonstrated that NLRP3 and NLRC4 are functional in primary urothelial cells (11). To demonstrate that the innate response was functional in the MYP3 cells these cells were treated with increasing doses of extracellular ATP, the quintessential activator of NLRP3 (17). As shown in Figure 8, ATP stimulated caspase-1 in a dose-dependent manner, with significant effects seen at 1.25 and 2.5 mM. To demonstrate that this effect was due to NLRP3, expression of this receptor was knocked down using custom designed siRNA constructs. As shown in Figure 9A, transient transfection with a single construct (A or B) knocked down mRNA by ≈70% with a further reduction apparent when the constructs were combined (A+B; ≈90%). Similar effects were seen by western blot (Figure 9B) demonstrating that these constructs reduce the translated level of protein as well. The effect of the knockdown on caspase-1activity, both basal and ATP-stimulated, is shown in Figure 10. Both single constructs (A and B) decreased basal expression which was further decreased by the combination (A+B), although this decrease never reached significance. ATP significantly increased activity in both the wild type and scrambled transfected cells to the same degree. Both A and B decreased activity to levels not significantly different from wild type and scrambled values (either 0 mM or 2.5 mM). The combination (A+B) significantly reduced the 2.5 mM ATP activity from the activated wild type and scrambled values to a level not significantly different from any of the unstimulated levels.
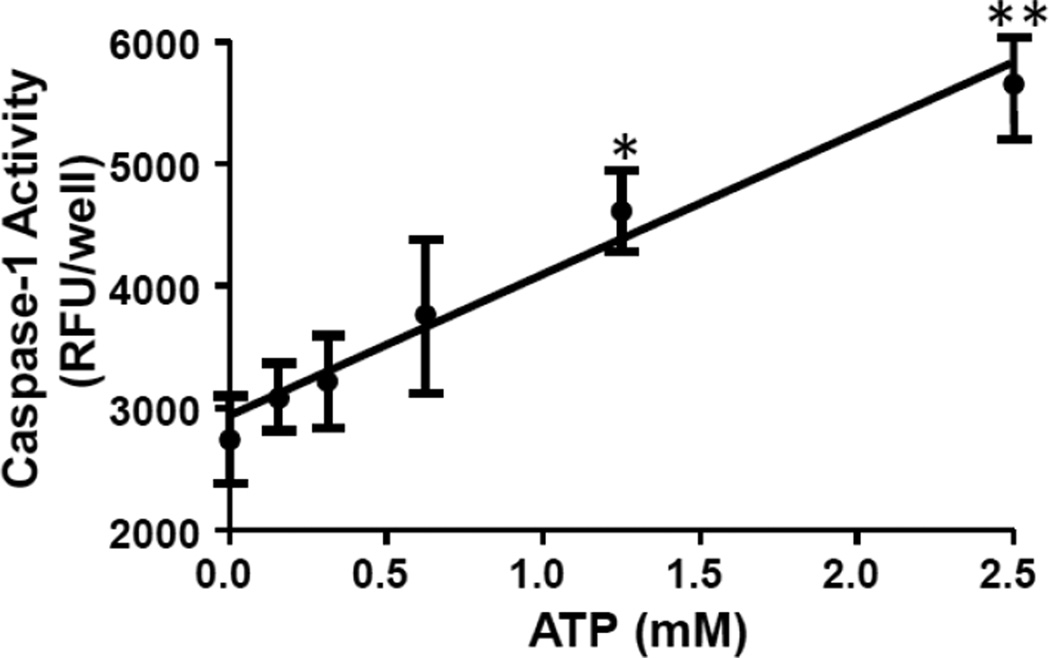
Caspase-1 activity in MYP3 cells is stimulated by extracellular ATP in a dose-dependent manner. As described in the materials and methods section, cells were plated and treated with various doses of ATP for 1 hr and Caspase-1 activity assessed. Points represent the mean±SEM. *p<0.05. **p<0.01 compared to the 0 mM ATP treatment as assessed by ANOVA and Tukey’s post-hoc test. N=12
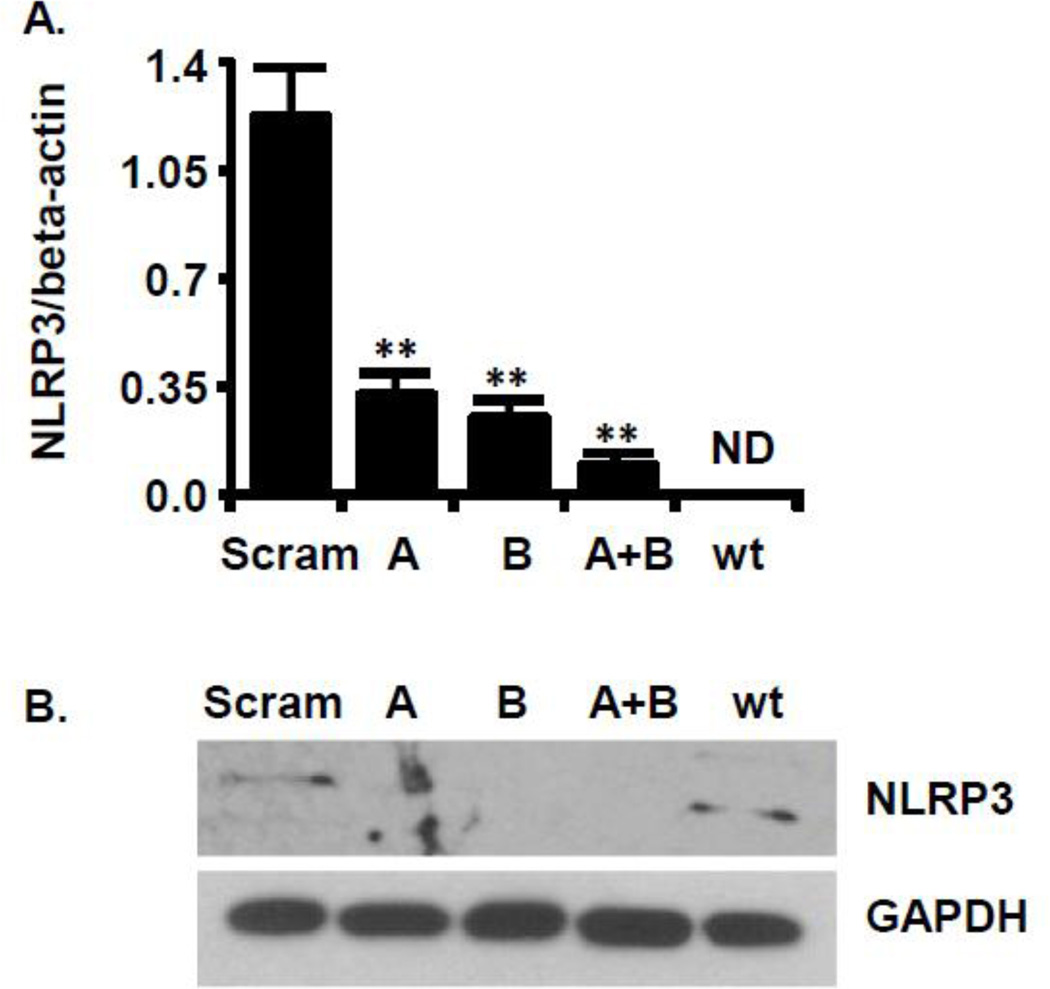
shRNA to NLRP3 decreases NLRP3 expression. MYP3 cells were transfected with one (A or B) both (A+B) constructs encoding shRNA to NLRP3, as described in the Material and Methods section. Scram was transfected with a construct recognizing no known mammalian sequence. Wild type (wt) were not transfected A. quantitative RT-PCR for NLRP3 Samples were normalized to beta-actin. Bars=mean± SEM. ** p<0.005 n=3. Stats: unpaired T-test compared to the Scram control, ND=Not determined. B. Western blot of the same groups in A probed with an antibody to NLRP3 or GAPDH (1:200 dilution)
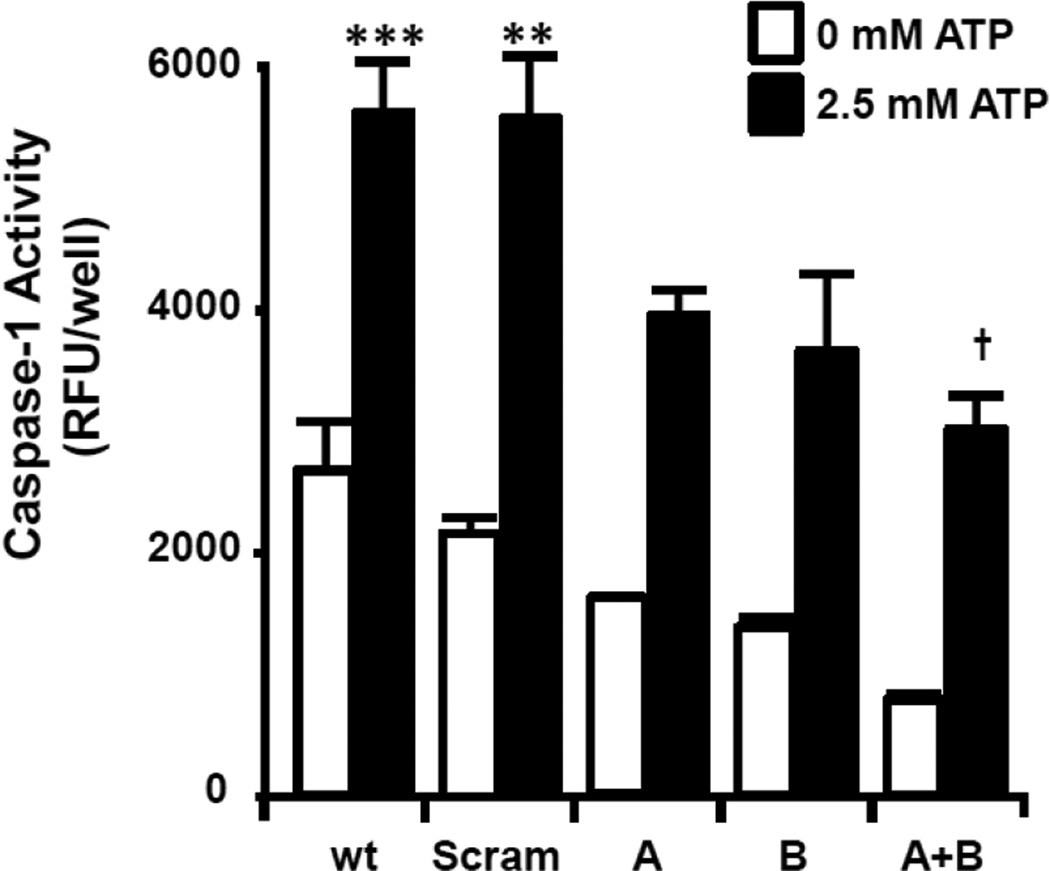
ATP-stimulated caspase-1 activity in MYP3 cells is reduced by shRNA to NLRP3. MYP3 cells were transfected with one (A or B) or both (A+B) constructs encoding shRNA to NLRP3, as described in the Material and Methods section. Scram was transfected with a construct of equal length but a scrambled sequences. Wild type (wt) were not transfected. Cells were plated for 24 before treatment with 0 or 2.5 mM ATP for 1 hr. Caspase-1 activity was then. Bars represent the mean±SEM. **p<0.01, ***p<0.001 compared to the wt, 0 mM ATP group. †p<0.01 compared to Scram treated with 2.5 mM ATP (0 mM ATP groups were not assessed for this statistic), N=12 for wild type, 3 for all other groups
Discussion
Staining for the various inflammasome sensors in this study revealed that they all had a nearly identical tissue distribution. Staining was localized in the urothelial layer and often concentrated in the umbrella cells (and/or luminal surface of the urothelial cells). Some PRRs were also present in the detrusor smooth muscle and vascular endothelial cells. The expression of all seven of these PRRs in the bladder suggests this organ possesses the capacity to respond to a large repertoire of PAMPs expressed by uropathogens and put up a formidable defense to invasion.
NLRP1 was the original inflammasome described (18) and is well known to respond to lethal toxin from Bacillus anthracis (antrax) (19). While anthrax infection is not a common problem in the urinary tract, this NLRP1 has also been found to respond to muramyl dipeptide (20, 21) which is a common peptidoglycan constituent of both gram-positive and gram-negative bacteria. Thus, this NLR may enable a response to a number of different uropathogens. Interestingly, Kummer et al. (10) did not find expression of NLRP1 in the bladder when he performed a survey of normal human tissues. There are many possible reasons for this discrepancy ranging from technical (difference in the antibodies used), to regulatory (it may have been upregulated in our rats but downregulated in the human samples), to fundamental species differences (the human bladder simply does not express NLRP1 whereas the rat bladder does). Reanalysis of human bladder, particularly in diseased and inflamed states, may clarify this issue.
NLRP3 has also been shown to respond to muramyl-dipeptide (22) but this is only one of many bacterial antigens that it recognizes (23). This NLR is by far the best studied PRR and has also been found to respond to numerous DAMPs making it the likely mediator of sterile inflammation in the bladder. Indeed, we have recently demonstrated the importance of this molecule in a rat model of hemorrhagic cystitis (11). In addition, one classic NLRP3 DAMP, monosodium urate, is a major component of urine and bladder stones underscoring the importance of this NLR for multiple causes of sterile bladder inflammation. Our finding of NLRP3 expression in the rat bladder confirms our previous findings and those of Kummer et al. (Kummer et al. 2007). MYP3 cells also demonstrated the expression of NLRP3, making them suitable for studying this inflammasome in urothelial cell culture.
NLRP6 is also present in the urothelia, detrusor smooth muscle and vascular structures within the bladder. However, no specific PAMP has yet been shown to activate this NLR (12). NLRP6 is best known for its role in guarding the integrity of the intestinal mucosa and regulating the colonic microbiota. (24–26). The mechanism of this regulation is poorly understood but may involve the production and/or processing of IL-18(25, 26). While urine was traditionally considered sterile and therefore free of microbes, numerous recent studies have shown that urine from asymptomatic people does indeed have a “normal” biotic flora (27, 28). This opens up the intriguing possibility that NLRP6 may help regulate the microbiota in the bladder as it does in the gut. In addition to maintaining the biota of the intestinal tract, NLRP6 regulates production of the protective mucus layer (mucins and glycosaminoglycans) by facilitating autophagy in the goblet cells which allows the release of mucus containing granules (29). In the bladder, surface mucus (mucins and glycosaminoglycans) acts as a barrier between urine and the underlying tissue and its dysfunction has been implicated in many bladder disorders including UTIs, calculus formation, radiation cystitis, carcinogenesis, and interstitial cystitis (30). If NLRP6 were found to play a role in defects of mucus production in the bladder it could make an excellent therapeutic target for these conditions.
NLRP7, found in the urothelial and muscle layers of the bladder, assembles an inflammasome in response to microbial acylated lipopeptides which are common components in a number of different bacteria. Thus, this NLR may participate in the innate response to a number of different invading pathogens. Interestingly, NLRP7-mediated production of IL-1β and IL-18 was required to block intracellular replication of bacteria in human macrophages (31). Therefore, there is the exciting possibility that this NLR mediates the response to intracellular bacterial communities (IBCs) that form in the urothelia cells after they have bypassed the initial mucus layer. Establishment of IBCs appears to be the critical step whereby acute UTI’s progress to chronic cystitis (32–34) and so understanding the action of this inflammasome could have high clinical relevance.
The importance of finding NLRP12 within the urothelium, detrusor muscle, and vascular tissue of the rat bladder is not clear at this point. The exact activating ligand for this poorly understood inflammasome has not been identified but this NLR is important for the recognition of Yersinia pestis, the causative microbe of the plague (35). Moreover, this NLR has also been found to be a negative regulator of NF-kB (36) suggesting it may have complex regulatory effects on the innate immune system. Speculation regarding its significance in the bladder is premature at this point and awaits further investigation.
NLRC4 is an important and well-studied NLR that populates evenly throughout the urothelium with minimal expression in the detrusor layer. It is the only one of the inflammasome sensors that directly binds pro-caspase-1 through its own endogenous caspase recruitment domain (CARD). NLRC4 responds to several bacterial components, in particular the inner rod protein PrgJ (or PrgJ-like proteins) of the Type III secretion system (T3SS) (37) and flagellin (Franchi 2006; Miao 2006) which is delivered into the cytoplasm using the T3SS (38). Sensitivity to a particular component appears to be determined by one of the NAIP proteins which actually bind to the respective ligand and facilitates the formation of an inflammasome with NLRC4 and caspase-1 (39–41). Humans have only one NAIP protein but rodents appear to have at least seven (42). Numerous studies have shown that rodent NAIP5 and 6 recognizes flagellin whereas NAIP1 and 2 mediate the response to needle and rod components of the T3SS (39–41). Human NAIP does not bind flagellin or inner rod proteins but does bind needle subunits of the T3SS such as Cpr1 or its homologs (41). Intriguingly, previous studies have shown that uropathogenic E. coli, by far the most common cause of UTI, expresses a T3SS (43) and thus would be likely to engage NLRC4 via NAIPs.
In addition to inflammasome formation, NLRC4, has been shown to elicit a rapid “eicosanoid storm” and eicosanoids have been implicated in several aspects of urinary tract infections (44–46). Previously we have identified the presence of NRLC4 in rat urothelia and its activation by intravesical exposure to flagellin (11). Data from this study confirm that finding and the demonstration of its presence in MYP3 show that this cell line is suitable for studying the properties of NLRC4 in the urothelium.
Aim2, found in the urothelium and detrusor muscle, is not an NLR but a member of the PYHIN family. It does, however form an inflammasome and activate caspase-1 in much the same way as the more classical inflammasomes such as NLRP3. The ligand for AIM2 is double-stranded DNA (47, 48). Exact DNA sequence is inconsequential as it may be recognized by this PRR so long as it is double stranded, of sufficient length (more than 80 bp) and present in the cytoplasm (49). Indeed, DNA of viral, prokaryotic and mammalian origin has been shown to activate AIM2 (48). Thus, AIM2 in the urothelium may participate in the response to bacterial pathogens that release their DNA into the cytoplasm (for example through cytosolic bacteriolysis (50, 51) while also acting as a critical sensor for viral pathogens.
Given the proximity of the bladder lumen to so many noxious agents, expression of such a vast array of PPRs by the urothelia makes them strategically positioned to maximize the innate response and minimize the time to that response. The mechanisms underlying the different infectious and sterile attacks, as well as the interaction of the various PRRs with each other, will be the matter of study for years to come. One additional striking observation was the presence of so many inflammasome sensors in the detrusor smooth muscle (NLRP1, NLRP6, NLRP7, NLRP12 and AIM2 with some light expression of NLRC4). Indeed, to the best of our knowledge this is the first study to demonstrate their expression in muscle of any type. In the bladder, they could represent a second “wall” of innate immunity to defend against pathogens that have invaded deep into the tissue or perhaps they could be performing tasks related to normal bladder physiology. Interestingly, the only inflammasome we found to be absent in the detrusor, NLRP3, is the only one that has an established presence in other muscle tissue types where its activation can produce significant consequences. For example, it is present in vascular smooth muscle where it’s recognition of cholesterol crystals (52) promotes vascular calcification and fibrosis (53). It has also been found in normal primary skeletal muscles (54) and in cardiac muscles (55) where it appears to mediate diabetes-induced myopathy. Understanding why it is excluded from detrusor muscle when all of the other PRRs were present is a matter for further inquiry.
This report is not an exhaustive study of the intracellular localization of inflammasomes but it does provide a snapshot of where they can be found under normal conditions. The histological sections suggest that all are in the cytoplasm (the expected location for inflammasome formation) while some may be present in the nucleus. For example, NLRC4 (Fig. 6) appears to be more concentrated in the nucleus than the cytoplasm whereas NLRP6 (Fig. 3) shows concentration in some nuclei and exclusion from others. Individual MYP3 cells showed widely variable intracellular locations for each of the PRRs. In each visual field, and for each PRR, cells could be found where localization was primarily nuclear, primarily cytoplasmic or distributed between both compartments. The reasons for this variability are unknown at this point but it is tempting to speculate that it may be related to their state of activation or they may be playing other roles that require nuclear localization, as proposed by Kummer et al. for NALP1 (10). Thus, future studies are needed to define how intracellular location of the various PRRs changes in response to activators and what the significance of those migrations might be. Activation studies on MYP3 cells in culture should provide an opportunity to initiate this line of inquiry.
Finally, the expression of the full repertoire of urothelia NLRs in the MYP3 cells suggest they may prove to be an excellent tool to investigate the various pathways involved. Critical to establishing their experimental value was the demonstration that caspase-1 could be activated in these cells by the addition of extracellular ATP, the prototypical activator of NLRP3. Moreover, expression and subsequent activation of NLRP3 could be reduced by transient transfection with shRNA targeting this NLR. Thus, the innate immune system ensemble represented by the immunocytochemistry data is very likely to functional in these cells. Certainly NLRP3 is functional and further studies will be undertaken to determine if the remaining NLRs are activatable.
This study demonstrates that the bladder possesses several different PRRs capable of recognizing pathogens or damaged host cells and provoking a response from the innate immune system. While the ability of these receptors to respond to important uropathogens or sterile attacks must still be determined, this study suggests that it is fertile ground for future exploration. Fortunately, as shown here, the MYP3 cells provide a suitable model for investigating these phenomena as they clearly express all of the PRRs contained in the rat urothelium.
Acknowledgements
The authors would like to thank Margret Romano for help with the immunocytochemistry. This work was supported by internal funds from the Medical university of South Carolina.
Footnotes
Conflict of Interest: The authors declare that they have no conflict of interest.
Ethical approval: All applicable international, national, and/or institutional guidelines for the care and use of animals were followed. All procedures performed in studies involving animals were in accordance with the ethical standards of the Medical University of South Carolina. All experimental procedures were reviewed and approved by the Institutional Animal Care and Use Committee (IACUC) at the Medical University of South Carolina and were performed in accordance with the guidelines set forth in the NIH Guide for the Care and Use of Laboratory Animals, published by the Public Health Service of the USA.
References
Full text links
Read article at publisher's site: https://doi.org/10.1007/s11255-015-1126-6
Read article for free, from open access legal sources, via Unpaywall:
https://europepmc.org/articles/pmc4774865?pdf=render
Citations & impact
Impact metrics
Citations of article over time
Alternative metrics
Smart citations by scite.ai
Explore citation contexts and check if this article has been
supported or disputed.
https://scite.ai/reports/10.1007/s11255-015-1126-6
Article citations
Specialized pro-resolution mediators in the bladder: effects of resolvin E1 on diabetic bladder dysfunction in the type 1 diabetic male Akita mouse model.
BMC Urol, 24(1):130, 21 Jun 2024
Cited by: 0 articles | PMID: 38907230 | PMCID: PMC11191353
Enzyme-induced hypoxia leads to inflammation in urothelial cells in vitro.
Int Urol Nephrol, 56(5):1565-1575, 22 Dec 2023
Cited by: 0 articles | PMID: 38133728
Formation of Double Stranded RNA Provokes Smooth Muscle Contractions and Structural Modifications in Bladder Ischemia.
Res Rep Urol, 14:399-414, 16 Nov 2022
Cited by: 1 article | PMID: 36415310 | PMCID: PMC9676006
The Urinary Microbiome; Axis Crosstalk and Short-Chain Fatty Acid.
Diagnostics (Basel), 12(12):3119, 10 Dec 2022
Cited by: 3 articles | PMID: 36553126 | PMCID: PMC9777402
Review Free full text in Europe PMC
Inflammation triggered by the NLRP3 inflammasome is a critical driver of diabetic bladder dysfunction.
Front Physiol, 13:920487, 25 Nov 2022
Cited by: 10 articles | PMID: 36505062 | PMCID: PMC9733912
Review Free full text in Europe PMC
Go to all (19) article citations
Similar Articles
To arrive at the top five similar articles we use a word-weighted algorithm to compare words from the Title and Abstract of each citation.
Inflammasomes are important mediators of cyclophosphamide-induced bladder inflammation.
Am J Physiol Renal Physiol, 306(3):F299-308, 27 Nov 2013
Cited by: 52 articles | PMID: 24285499 | PMCID: PMC4073918
Elevated hydrostatic pressure stimulates ATP release which mediates activation of the NLRP3 inflammasome via P2X4 in rat urothelial cells.
Int Urol Nephrol, 50(9):1607-1617, 11 Aug 2018
Cited by: 12 articles | PMID: 30099658 | PMCID: PMC6129973
The NLRP3 Inflammasome Mediates Inflammation Produced by Bladder Outlet Obstruction.
J Urol, 195(5):1598-1605, 18 Dec 2015
Cited by: 54 articles | PMID: 26707508 | PMCID: PMC4870136
Atypical Inflammasomes.
Methods Mol Biol, 1417:45-62, 01 Jan 2016
Cited by: 5 articles | PMID: 27221480
Review
Funding
Funders who supported this work.
Internal funds
NIDDK NIH HHS (1)
Grant ID: R01 DK103534