Abstract
Free full text

Colonic tolerance develops in the iliac lymph nodes and can be established independent of CD103+ dendritic cells
Abstract
Tolerance to harmless exogenous antigens is the default immune response in the gastrointestinal tract. Although extensive studies have demonstrated the importance of the mesenteric lymph nodes (MLN) and intestinal CD103+ dendritic cells (DCs) in driving small intestinal tolerance to protein antigen, the structural and immunological basis of colonic tolerance remain poorly understood. We show here that the caudal and iliac lymph nodes (ILN) are inductive sites for distal colonic immune responses and that colonic T cell-mediated tolerance induction to protein antigen is initiated in these draining lymph nodes and not in MLN. In agreement, colonic tolerance induction was not altered by mesenteric lymphadenectomy. Despite tolerance development, CD103+CD11b+ DCs which are the major migratory DC population in the MLN, and the tolerance-related retinoic acid-generating enzyme RALDH2 were virtually absent from the ILN. Administration of ovalbumin (OVA) to the distal colon did increase the number of CD11c+MHCIIhi migratory CD103−CD11b+ and CD103+CD11b− DCs in the ILN. Strikingly, colonic tolerance was intact in Batf3-deficient mice specifically lacking CD103+CD11b− DCs, suggesting that CD103− DCs in the ILN are sufficient to drive tolerance induction after protein antigen encounter in the distal colon. Altogether, we identify different inductive sites for small intestinal and colonic T-cell responses and reveal that distinct cellular mechanisms are operative to maintain tolerance at these sites.
INTRODUCTION
The intestine is continuously exposed to foreign antigens. The intestinal immune system must maintain a balance between quiescence and activation, fulfilling immediate demands for protection against potential pathogens, but tolerance to harmless proteins from food and commensal microbiota. Although this intricate decision making process takes place along the entire length of the gastrointestinal tract, the nature of the antigens encountered and the distribution of immune cells varies markedly between the different regions of the intestine (reviewed by Mowat and Agace).1 Whereas food antigens predominate in the upper small intestine, the terminal ileum and colon are exposed mostly to microbial antigens. Accordingly, failure to maintain tolerance to food proteins leads to small intestinal disease, while loss of tolerance to microbial antigen mostly causes disease restricted to the terminal ileum or colon.2, 3 In recent years, advances have been made in understanding the anatomical, physiological and immunological mechanisms underlying small intestinal tolerance to protein antigen. However, the structural and immunological basis of colonic tolerance to protein antigen remains poorly understood.
Peyer's patches (PP), mesenteric lymph nodes (MLN) and liver-draining lymph nodes4, 5 are inductive sites for small-intestinal T-cell responses. Within 24 to 48h after feeding a harmless protein, naive T cells differentiate into functionally suppressive tolerogenic T cells at these sites and acquire gut-homing capacity.6, 7 The MLN are essential for the resulting systemic tolerance,8 which relies partly on intrinsic properties of the resident stromal cells,4, 9, 10 as well as the arrival of antigen-carrying dendritic cells (DCs) from the small intestinal lamina propria.8 Three functionally distinct DC subsets migrate via afferent intestinal lymph to the MLN in an C-C motif chemokine receptor 7 (CCR7)-dependent manner.8, 11 These migratory DCs consist of two CD103+ subsets (CD103+CD11b+CD8α− and CD103+CD11b−CD8α+), and a population of CD103−CD11b+CX3CR1int DCs.12, 13 In particular, CD103+ DCs have been shown to induce the differentiation of suppressive Foxp3+ regulatory T cells (Treg) in the MLN. Expression of the retinoic-acid (RA)-producing enzyme retinal dehydrogenase 2 (RALDH2) and the expression of TGFβ-activating integrin αvβ8 contribute to increased Foxp3+ Treg development.14-16 Synthesis of RA by CD103+ DCs also induces the expression of α4β7 integrin and the chemokine receptor CCR9 on differentiating T cells, allowing them to home selectively to the small intestinal lamina propria.17-20
The proportion of Foxp3+ Treg cells in colonic lamina propria has been reported to be approximately three times higher compared to the small intestinal lamina propria,21 but where these colonic T cells are first induced is less well characterized. Previous studies indicate that the CD103+CD11b+ DCs which make up the majority of DCs in the small intestinal lamina propria are rare in the colonic mucosa and the colon is also relatively devoid of CD103+ DCs having RALDH2 activity.19, 22 Moreover, homing of colonic T cells to the large intestine can occur in a RA-independent manner and is under the control of the orphan G-protein coupled receptor GPR15 and CCR6.23-25 Together these data led us to hypothesize that the structural and immunological basis of colonic tolerance may be different from small intestinal tolerance.
Here we demonstrate that application of the model antigen ovalbumin (OVA) to the distal colon induces systemic tolerance. Importantly, DC-mediated antigen transport and de novo Foxp3+ Treg differentiation after colonic OVA administration occur in the iliac and caudal lymph nodes (ILN), whereas after oral OVA administration these events take place in the MLN. The ILN-derived DCs comprise only two main subsets of migratory DC, CD103+CD11b− and CD103−CD11b+ DCs, with the CD103+CD11b+ DC subset being virtually absent. In Batf3−/− mice specifically lacking CD103+CD11b− DCs, the sole presence of CD103−CD11b+ DCs is sufficient to induce colonic tolerance. These data identify different inductive sites for small intestinal and colonic T-cell responses and reveal that distinct cellular mechanisms are operative to maintain T cell-mediated tolerance in the small and large intestine.
RESULTS
The iliac lymph nodes are inductive sites for colonic T-cell responses
Oral tolerance to food proteins depends on antigen transport from the small intestine to the draining MLN, where DCs initiate adaptive immune responses by priming naive T cells.3, 6, 8 In the early 1970s, it has been described that lymphatic drainage from the small and large intestine is distinct,26, 27 but this has been overlooked when studying immune responses in the large intestine. To identify the major site of antigen presentation following intracolonic antigen administration, Alexa-Fluor 488-labeled OVA (OVA-488) was administered directly into the distal colon of BALB/c mice by inserting a canula via the rectum. After 20h, colonically applied fluorescently labeled OVA was exclusively associated with CD11chigh cells in the caudal and iliac lymph nodes (collectively denoted as ILN), whereas orally applied antigen was associated with CD11chigh cells in the MLN (Figure 1a-c). To establish that this antigen drainage to ILN elicited a productive T-cell response, mice were adoptively transferred with CFSE-labeled naive OVA-specific T cells (CD4+KJ1.26+Rag−/−) and one day later, received a single dose of OVA either orally or intracolonically. In agreement with the site of antigen drainage, oral OVA elicited a T-cell response exclusively in the MLN while intracolonic OVA led to antigen-specific T-cell proliferation in the ILN (Figure 1d,e). Considering the disparate requirements of T-cell homing to the small and large intestine mediated by CCR9 and GPR15, respectively, we next determined expression of these intestinal homing receptors in whole lymph node cell preparations. As shown in Figure 1f, Gpr15 mRNA was highly expressed in the ILN, whereas Ccr9 expression was significantly higher in the MLN. Taken together, our data clearly demonstrate that T-cell responses to oral or colonic antigens are spatially segregated and that the iliac lymph nodes are the inductive site for antigen-specific T-cell responses in the distal large intestine.
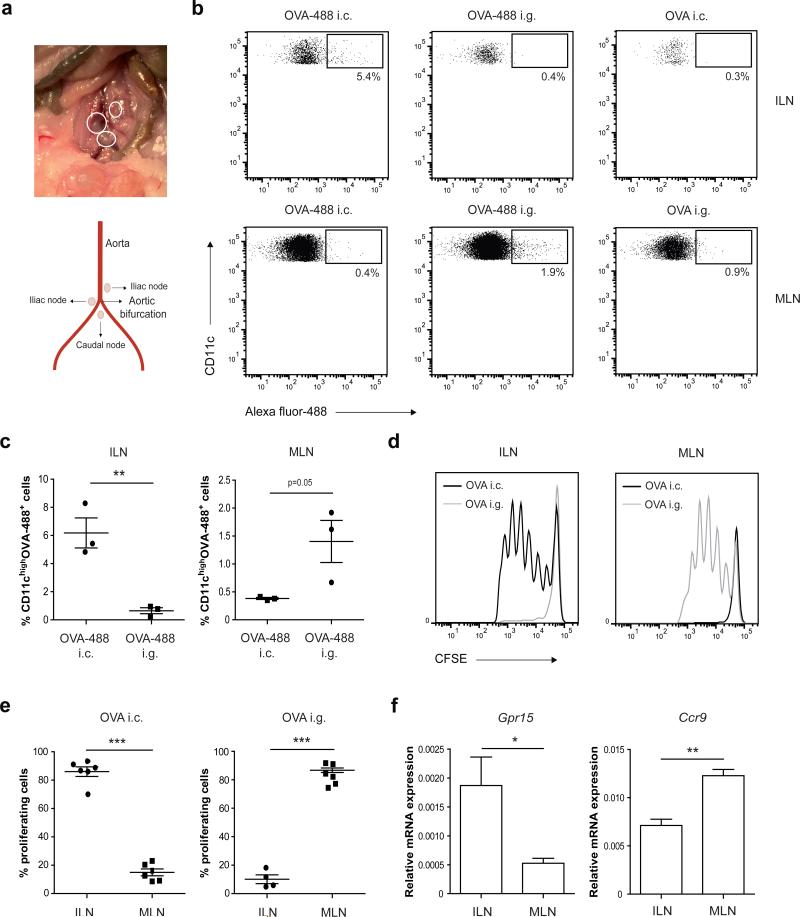
(a) Schematic illustration of the location of colon-draining ILN. (b, c) BALB/c mice received 3.5 mg OVA orally (i.g.) or 1.7 mg OVA intracolonically (i.c.), either labeled with Alexa Fluor-488 succinimidyl ester or unlabeled. Twenty hours after OVA administration, MLN and ILN were digested using liberase/DNAse and single cell suspensions were stained for CD11c and analyzed for Alexa-Fluor-488+ cells by flow cytometry. (b) Representative dot plots and (c) percentage CD11chighOVA-488 positive cells determined by flow cytometry are shown. Results are depicted as mean plus SEM and are representative of two independent experiments using 3 mice per experiment. **P<0.01 versus control, by Mann-Whitney U test. (d, e) CD4+KJ1.26+ OVA-specific T cells were purified from DO11.10 transgenic × Rag−/− mice and labeled with CFSE. Subsequently, BALB/c mice were given 6 × 106 CFSE-labeled T cells intravenously and one day later, received 70 mg OVA either i.g. or i.c. 72h after OVA administration, MLN and ILN were analyzed for antigen-specific T-cell proliferation by measuring CFSE dye dilution. (d) Histogram plots of CFSE fluorescence of OVA-specific TCR-transgenic CD4+ T cells and (e) percentage proliferating cells determined by flow cytometry are shown. The data shown are from two independent experiments and are depicted as mean plus SEM. ***P< 0.001 versus control, by Mann-Whitney U test. (f) Whole cell preparations of lymph nodes were analyzed for expression of Gpr15 and Ccr9 mRNA by quantitative PCR analysis. Values are mean plus SEM for 3 mice per group. *P< 0.05, **P<0.01 versus control, by Student's t-test.
Colonic administration of protein antigen results in de novo Foxp3+ Treg induction in the colon-draining lymph nodes and induces systemic tolerance
Having identified the inductive site for distal colonic T-cell responses, we next determined whether colonic administration of harmless antigen induces systemic immune tolerance via the induction of Treg cells in the ILN. In untreated mice, transcript levels of Foxp3 in the ILN (Supplementary Figure S2a) and the percentage of CD4+Foxp3+ Treg cells (Supplementary Figure S2b) were similar to that in the MLN. Both draining lymph nodes also had comparable Tgfb1 mRNA expression (Supplementary Figure S2c). We subsequently investigated whether naive T cells can be converted into Foxp3+ Treg cells in the ILN after colonic administration of protein antigen. BALB/c mice were adoptively transferred with CFSE-labeled OVA-specific naive D011.10 T cells and one day later, received OVA either orally or intracolonically, with the generation of antigen-specific Foxp3+ Treg cells being assessed in the respective draining lymph nodes 72h later. Compared to a non-mucosal route such as intramuscular administration, an enhanced frequency of Foxp3+ Treg cells was detected in the ILN of mice given OVA intracolonically, although the frequency was slightly lower than in the MLN of OVA fed mice (Figure 2a,b). No proliferation or Foxp3+ induction was observed in the MLN and ILN after intracolonic or oral OVA administration, respectively (Supplementary Figure S2d). To demonstrate that colonic OVA can elicit systemic tolerance in the same way as oral antigen, a classical delayed type hypersensitivity (DTH) response was induced after administration of OVA either orally or intracolonically. As shown in Figure 2c, colonic OVA administration resulted in decreased ear swelling when compared with control mice and to a similar extent as the decrease seen after feeding OVA. In addition, to demonstrate that the differentiated ILN T cells are tolerogenic, CD4+ ILN T cells were purified from DO11.10 reconstituted mice at 72h after intracolonic OVA treatment and transferred to naive acceptor mice that were subjected to a DTH response. ILN CD4+ T cells derived from colonic OVA-treated mice significantly suppressed the OVA-specific DTH response in BALB/c acceptor mice when compared to mice that received purified CD4+ T cells isolated from non-mucosal lymph nodes after intramuscular OVA administration (Figure 2d). To conclusively demonstrate that colonic tolerance does not depend on the MLN, all nodes along the length of the superior mesenteric artery were surgically excised by microdissection. Six weeks after surgery, mice received OVA intracolonically and were subjected to a DTH response to assess tolerance development. As shown in Figure 2e, MLN were not required for colonic tolerance induction, as intracolonic OVA resulted in an equally decreased ear swelling in MLN-resected mice and non-resected mice when compared to DTH control mice. Consistent with this, in MLN-resected mice and non-resected mice, intracolonic OVA elicited equal suppression of IFNγ release by re-stimulated cells from the inguinal lymph nodes which drain the immunization site (Figure 2f). Together these data unequivocally demonstrate that colonic protein administration induces functional Treg differentiation in the ILN which mediates subsequent systemic tolerance.
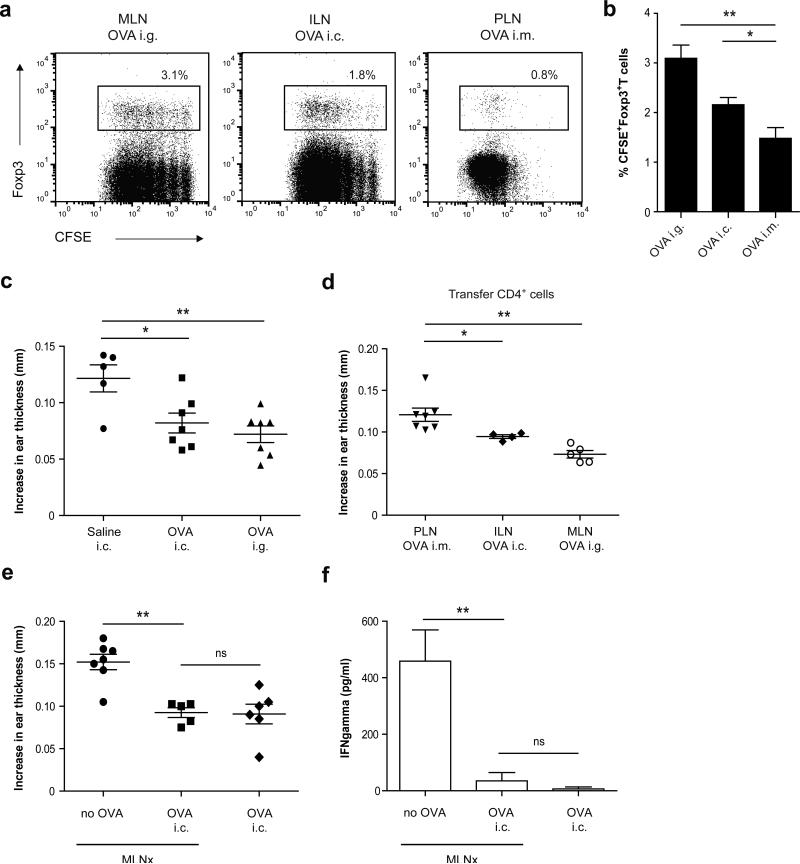
(a, b) CD4+KJ1.26+ OVA-specific T cells were purified from DO11.10 transgenic × Rag−/− mice and labeled with CFSE. Subsequently, BALB/c mice were given 6 × 106 CFSE-labeled T cells intravenously and one day later, received 70 mg OVA either i.g., i.c. or 400 μg OVA intramuscularly (i.m.). 72 h after OVA administration, draining lymph nodes (MLN for i.g., ILN for i.c. and popliteal/inguinal lymph nodes for i.m.) were isolated and analyzed for CD4+KJ1.26+CFSE+Foxp3+ T cells by flow cytometry. Values are depicted as mean plus SEM from 2 independent experiments with 4-8 mice per experimental condition. *P< 0.05, **P<0.01 versus control, by one-way ANOVA. (c) Mice were treated with saline, 25 mg OVA i.g. or i.c. and five days later were sensitized subcutaneously in the tail base with 100 μg OVA emulsified in Incomplete Freund's adjuvant. Five days after sensitization, mice were challenged with 10 μg OVA in the auricle of both ears. After 24 h, increases in ear thickness in both ears were determined and compared with values before challenge. Values are mean plus SEM for 5-7 mice per experimental condition. *P< 0.05, **P< 0.01 versus control, by one-way ANOVA. (d) Mice were adoptively transferred with 6 μ 106 CFSE-labeled CD4+KJ1.26+ T cells and one day later, received 70 mg OVA i.g., i.c. or 400 μg OVA i.m. 72 h after OVA administration, draining lymph nodes were isolated and enriched for CD4+ T cells. Subsequently, 1 × 105 CD4+KJ1.26+ T cells were transferred to naive second acceptor mice and one day after the transfer, recipient mice were sensitized subcutaneously in the tail base with 100 μg OVA emulsified in Incomplete Freund's adjuvant. After 5 days, mice were challenged with 10 μg OVA in the auricle of both ears. After 24h, increases in ear thickness in both ears were determined and compared with values before challenge. Data are mean plus SEM for 4-7 mice per experimental condition. *P< 0.05, **P< 0.01 versus control, by one-way ANOVA. (e, f) MLNs were surgically excised (MLNx) and after 6 weeks mice were treated with 25 mg OVA i.c. Three days later, mice were sensitized subcutaneously in the tailbase with 100 μg OVA emulsified in Incomplete Freund's adjuvant. At day 8, mice were challenged with 10 μg OVA in the auricle of both ears. (e) After 24h, increases in ear thickness in both ears were determined and compared with values before challenge. (f) Cells purified from the inguinal lymph nodes after induction of the DTH reaction were restimulated for 48h with OVA protein. IFNγ levels were determined in supernatant by ELISA. Completeness of mesenteric lymphadenectomy was confirmed in all MLNx animals at autopsy. The results are depicted as mean plus SEM for 5-7 mice per experimental condition. **P< 0.01 versus control, using one-way ANOVA.
Colon-draining lymph nodes lack CD103+CD11b+ migratory DC
To assess which DC populations contribute to colonic tolerance induction, we characterized the CD11c+MHCIIhigh migratory DC subsets in the colon-draining lymph nodes (see Supplementary Figure S1 for gating strategy). In untreated mice, the ILN-derived CD11c+MHCIIhigh migratory DCs comprised two main DC subsets, CD103+CD11b− and CD103−CD11b+ DCs. Strikingly, the CD103+CD11b+ DCs that are present in large numbers in the MLN were absent from ILN (Supplementary Figure S1). In line with our data identifying ILN as inductive sites for colonic immune responses, colonically applied OVA caused a significant increase in the total number of CD11c+MHCIIhigh migratory DCs in the ILN (Figure 3a,b). However, in contrast to oral OVA which increased the CD103+CD11b+ and CD103+CD11b− migratory DCs in the MLN (Figure 3c,d), colonic antigen administration led to an increase in CD103+CD11b− and CD103−CD11b+ DCs in the ILN while the CD103+CD11b+ DC population remained absent under these conditions (Figure 3c,d). To exclude that the absence of CD103+CD11b+ DCs was related to the structural characteristics of the applied protein antigen, we administered the TLR7/8 ligand R848 known as a potent inducer of DC migration. Even after colonic administration of R848, the colon-draining lymph nodes were still devoid of CD11c+MHCIIhi migratory CD103+CD11b+ DCs (Supplementary Figure S3a,b). Since migration of DCs from the small intestinal lamina propria to the MLN is CCR7-dependent, we subsequently determined CCR7 expression on the migratory DC subsets in the colon-draining lymph nodes. Irrespective of their location, CCR7 expression was detected on all CD103+ and CD103− DC subsets (Figure 3e, Supplementary Figure S3c), consistent with them having migrated from the mucosa to the colon-draining and small intestine draining lymph nodes. These findings demonstrate that the DC subset composition in the ILN is distinct from that in the MLN with a striking absence of migratory CD103+CD11b+ DCs.
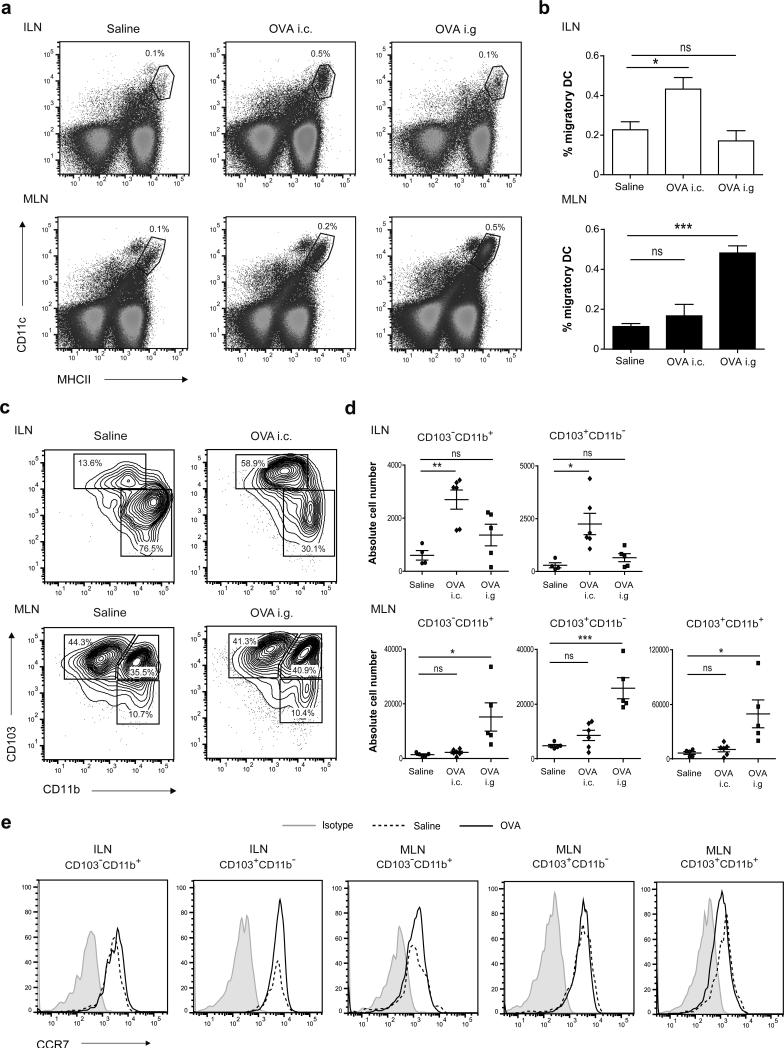
Mice received 70 mg OVA i.g. or i.c. and 16-18h later, MLN and ILN were digested using liberase/DNAse and single cell suspensions were stained for CD45, F4/80, CD11c, MHCII, CD11b, CD103 and CCR7. (a,b) Live CD45+F4/80− single cells were gated and then analyzed for CD11c+MHCIIhigh migratory DCs. (a) Representative dot plots and (b) percentage CD11c+MHCIIhigh migratory DCs determined by flow cytometry are shown. The data shown are from three independent experiments (3 mice pooled for each experimental condition per experiment) and depicted as mean plus SEM. ***P< 0.001 versus control, by one-way ANOVA. (c) The CD11c+MHCIIhigh migratory DC population was further analyzed by assessing the expression of CD103 and CD11b. (d) Absolute cell numbers of CD103+CD11b− and CD103−CD11b+ DCs from ILN (upper panel) and CD103+CD11b+, CD103+CD11b− and CD103−CD11b+ DCs from MLN (lower panel) after OVA administration. The data shown are from three independent experiments using cells pooled from 2-3 mice for each experimental condition per experiment. *P< 0.05, **P< 0.01 ***P< 0.001 versus control, using one-way ANOVA. (e) Migratory DC subsets were stained for CCR7 after OVA i.g. (MLN) or i.c. (ILN). Representative histogram plots are shown of two independent experiments.
Dendritic cells in the colon-draining and small intestine draining lymph nodes share lineage-specific transcription factors, but differ in their RALDH2 expression
We next investigated whether the migratory DCs found in the colon-draining ILN differed in phenotype from those in the MLN. We first examined expression of interferon regulatory factor (IRF)4 and IRF8, transcription factors which specify the development and maintenance of CD11b+ and CD11b− DCs in the intestine and elsewhere.28-30 As described previously, IRF4 was highly expressed in the CD103+CD11b+ DC population derived from the MLN. In addition, the CD103−CD11b+ DC populations from both MLN and ILN expressed intermediate levels of IRF4 (Figure 4a). IRF8 was expressed exclusively in the CD103+CD11b− DC subset of both ILN and MLN (Figure 4b). These data demonstrate that the distinct DC subsets from the colon-draining and small intestine draining lymph nodes share transcriptional regulatory programs irrespective of their location. We subsequently determined the expression of known enzymes that promote regulatory responses to orally administered antigens, such as cyclooxygenase-2 (COX-2)31 and RALDH214 responsible for generating PGE2 and RA, respectively. Thereto, we sorted CD103+CD11b−, CD103−CD11b+ and CD103+CD11b+ DCs from MLN and ILN (see Supplementary Figure S1 for gating strategy). Selective expression of Itgae (CD103) mRNA confirmed the sorting efficiency (data not shown), and similar to flowcytometric analysis (Figure 3e), the chemokine receptor Ccr7 was detectable in all sorted cell populations (data not shown). In the MLN, transcription of Ptgs2 (COX-2) was observed in the CD103+CD11b+ DCs in MLN, and to a lesser extent the CD103−CD11b+ DCs, with almost no expression by CD103+CD11b− DCs. A similar pattern was seen in the colon-draining ILN, where only the CD103− DCs exhibited Ptgs2 transcription (Figure 4c). In parallel, Ptgs2 mRNA was transcribed preferentially in whole lymph node preparations from both ILN and MLN compared with non-mucosal peripheral lymph nodes (Figure 4d). Strikingly, although Aldh1a2 (RALDH2) was highly transcribed by both subsets of CD103+ DCs from the MLN, significantly lower levels were found in CD103+CD11b− DCs from the colon-draining ILN (Figure 4e). In agreement, Aldh1a2 transcripts were barely detectable in whole cell preparations of either ILN or peripheral lymph nodes (Figure 4f). Thus, although the CD11b+ and CD11b− DCs from the ILN and MLN share lineage-specific developmental transcription factors, the CD103+CD11b− DCs from both draining sites show striking differences in their RALDH2 expression.
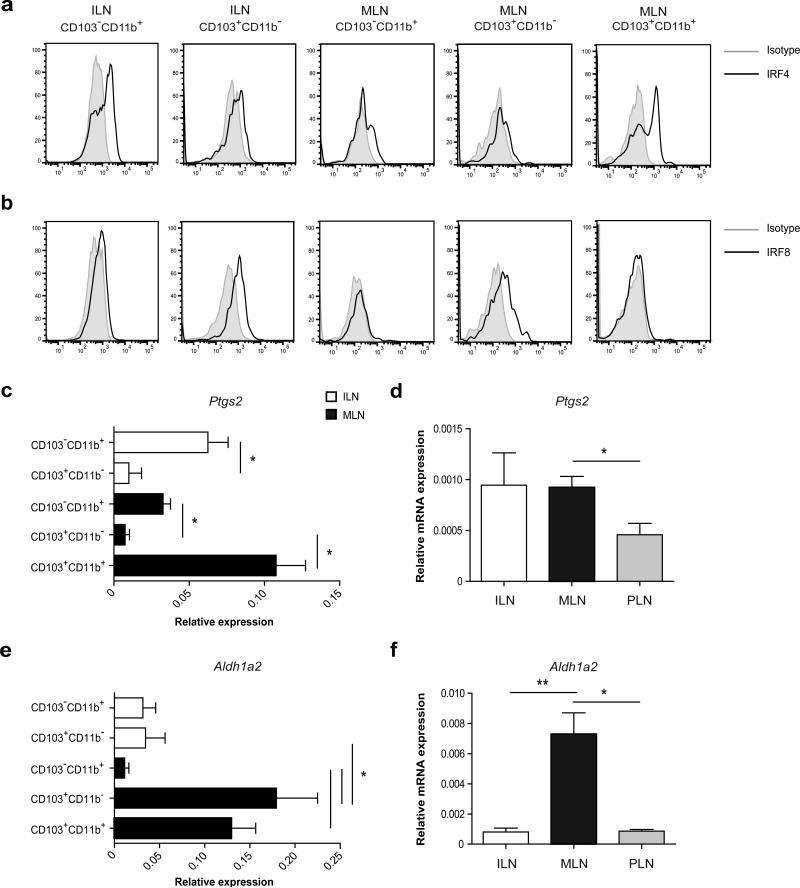
(a, b) MLN and ILN were digested using liberase/DNAse and single cell suspensions were stained for CD45, F4/80, CD11c, MHCII, CD11b, CD103. Live CD45+F4/80− single cells were gated and further analyzed for the expression of CD103 and CD11b within the CD11c+MHCIIhigh migratory DCs. The DC subsets were then analyzed for (a) IRF4 or (b) IRF8 expression by intracellular staining. The results shown are from one of two experiments using cells pooled from 8 mice. (c, e) CD103+ and CD103− DC subsets were purified by flow cytometric cell sorting and expression of (c) Ptgs2 (e) aldh1a2 mRNA was determined by quantitative PCR analysis. Values are depicted as mean plus SEM from 4 independent experiments using cells pooled from 20-30 mice per experiment. *P< 0.05 versus control, by one-way ANOVA. (d, f) Whole cell preparations of lymph nodes were analyzed for expression of (d) Ptgs2 and (f) aldh1a2 mRNA by quantitative PCR analysis. The peripheral lymph nodes (PLN) include the inguinal, axillary, brachial lymph nodes. Values are mean plus SEM for 3-7 mice per group. *P< 0.05, **P< 0.01 versus control, by one-way ANOVA.
Batf3−/− mice that specifically lack CD103+CD11b− DCs develop normal tolerance after colonic antigen administration
Given that the CD103+CD11b+ DCs are absent from the colon-draining lymph nodes and that Aldh1a2 was barely detectable in the CD103+CD11b− DCs, whereas CD103−CD11b+ DCs expressed substantial levels of Ptgs2, we hypothesized that CD103−CD11b+ DCs are sufficient to maintain tolerance to colonic proteins. Therefore, tolerance induction was studied in Batf3−/− mice that lack CD103+CD11b− DCs in the MLN because of their developmental dependence on Batf3.32, 33 Consistent with this, ILN-derived CD103+CD11b− DCs from wild-type mice expressed significantly higher Batf3 mRNA levels compared with CD103−CD11b+ DCs (Supplementary Figure S4a) and ILN from Batf3−/− mice completely lacked the CD103+CD11b− DC population. As a result CD103−CD11b+ DCs were the sole migratory DC population in these nodes (Figure 5a) even after colonic antigen administration (Supplementary Figure S4b,c). In untreated mice, the ILN from Batf3−/− mice had a normal population of CD4+Foxp3+ Treg cells compared to C57BL/6 wild-type mice (Figure 5b). To determine whether the CD103−CD11b+ DCs stimulate T-cell proliferation and Foxp3 induction, CFSE-labeled naive OVA-specific CD4+ T cells (CD4+OT-II Rag−/−) were cocultured with OVA-loaded Batf3−/− CD11c+ DCs in vitro. As shown in Figure 5c, the OT-II T cells underwent a similar number of divisions in the presence of Batf3−/− CD11c+ DCs compared to C57BL/6 wild-type CD11c+ DCs from ILN. Furthermore, the percentage of TGFβ-induced Foxp3+ Treg cells among the responding OT-II T cells was not significantly different between the ILN cultures containing Batf3−/− and C57BL/6 wild-type CD11c+ DCs (Figure 5d). These data suggest that the CD103−CD11b+ DCs from the ILN are capable of inducing Foxp3+ Treg cells normally. To prove that CD103−CD11b+ DCs can drive colonic tolerance induction in vivo, C57BL/6 Batf3−/− mice were subjected to a DTH response after having received OVA either orally or intracolonically. In Batf3−/− mice, induction of either colonic or oral tolerance elicited a significant suppression of ear swelling and the suppression was similar to that observed in C57BL/6 wild-type mice (Figure 5e). Compared with Batf3−/− mice treated with saline, CD4+ T cells from the inguinal lymph nodes draining the immunization site of orally or colonically tolerized Batf3−/− mice produced significantly less IFNγ after in vitro restimulation with OVA protein (Figure 5f), showing that systemic immunity to OVA is suppressed in colonically or orally tolerized Batf3−/− mice. Altogether, these data clearly demonstrate that CD103−CD11b+ DCs in the ILN are sufficient to drive mucosal tolerance induction after intracolonic protein antigen administration.
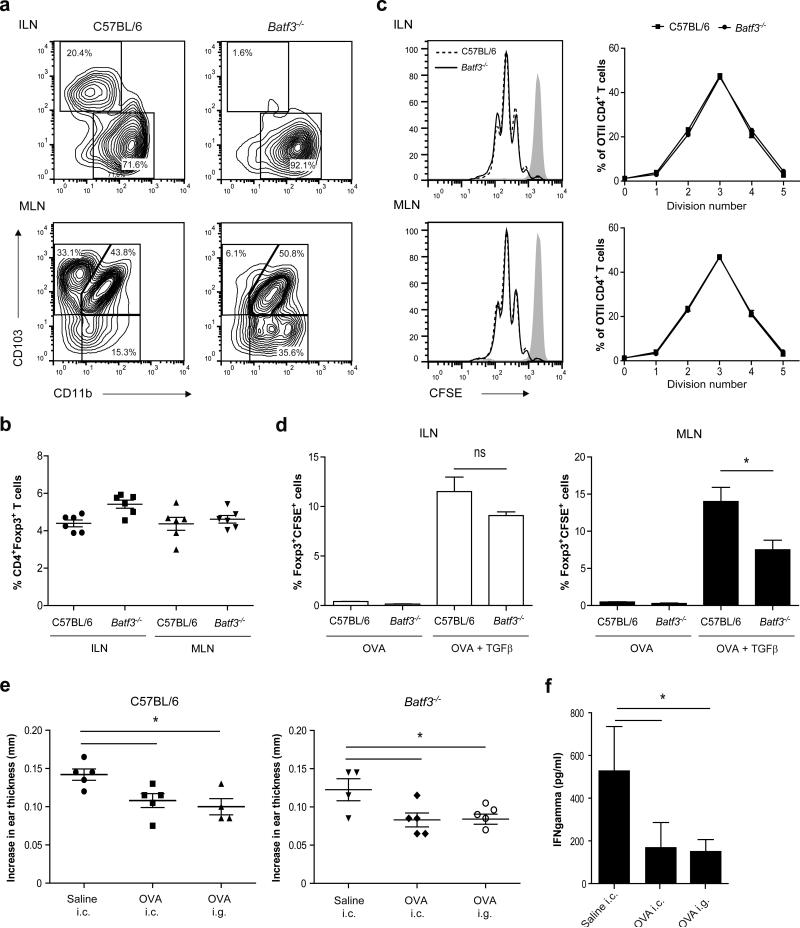
(a) MLN and ILN were isolated from C57BL/6 and Batf3−/− mice and single cell suspensions were stained for CD45, F4/80, CD11c, MHCII, CD11b, and CD103. Live CD45+F4/80− single cells were gated and further analyzed for the expression of CD103 and CD11b within the CD11c+MHCIIhigh migratory DCs. (b) The percentage of CD4+Foxp3+ T cells in MLN and ILN from untreated C57BL/6 and Batf3−/− mice was determined by flow cytometry. Values are depicted as mean plus SEM for 6 mice (c, d) Naive CD4+ OVA-specific T cells were purified from OTII transgenic × Rag−/− mice and labeled with CFSE. At the same time, CD11c+ cells were isolated from MLN and ILN of C57BL/6 and Batf3−/− mice by flow cytometric cell sorting and loaded with OVA protein for 1 hour. OVA-loaded CD11c+ cells were co-cultured with CFSE-labeled OVA-specific naive T cells for 72 hours. (c) Histogram plots of CFSE fluorescence of OVA-specific TCR-transgenic CD4+ T cells and percentage proliferating cells in each division peak. (d) Foxp3+CFSE+ T cells amongst CD4+ T cells in the presence or absence of TGFβ determined by flow cytometry. The results are means plus SD from triplicate cultures using cells pooled from 33 Batf3−/− mice or 45 C57BL/6 mice. *P< 0.05 versus control, by Student's t test. (e, f) Mice were treated with saline, 25 mg OVA i.g. or i.c. and three days later were sensitized subcutaneously in the tailbase with 100 μg OVA emulsified in Complete Freund's adjuvant. At day 8, mice were challenged with 10 μg OVA in the auricle of both ears. (e) After 48h, increases in ear thickness in both ears were determined and compared with values before challenge and (f) CD4+ T cells were purified from the inguinal lymph nodes of Batf3−/− mice after induction of the DTH reaction and were cocultured with splenic CD11c+ cells from C56BL/6 wild-type mice and restimulated for 48h with OVA protein. IFNγ levels were determined in supernatant by ELISA. The results are depicted as mean plus SEM for 4 or 5 mice per experimental condition. *P< 0.05 versus control, using one-way ANOVA.
DISCUSSION
We have identified the caudal and iliac lymph nodes as inductive sites for antigen-specific T-cell responses to harmless protein antigen that is encountered in the distal part (last 4 cm) of the colon. The ensuing T-cell response is independent of the MLN, functionally suppressive and has the capacity to confer antigen-specific systemic hyporesponsiveness. The composition and phenotype of the migratory DCs in the colon-draining lymph nodes differ from those in the MLN, with the CD103+CD11b+ DCs and the vitamin A-converting enzyme RALDH2 being virtually absent in the ILN. Despite these differences, the induction of colonic tolerance is robust and our data uncover a previously unknown role for CD103−CD11b+ DCs in this tolerogenic response.
Lymphatic drainage of the small and large intestine has already been studied in the early 70s26, 27 and revealed that the MLN drains the small intestine, cecum and the ascending colon. As recently described, individual nodes within the string of MLN drain the small intestine while others drain the ascending colon. In particular, the ascending colon is drained by the inferior mesenteric node lying in the mesentery and sometimes referred to as colonic mesenteric lymph nodes (cMLN).34 In contrast, lymph from descending colon and rectum drains to the caudal lymph nodes lying posterior of the abdominal aorta bifurcation. The iliac lymph nodes lying directly anterior of the bifurcation on either side of the distal aorta are crucial secondary drainage sites for the caudal lymph nodes.26, 27 The selective lymphatic drainage from the distal part of the colon to the caudal and iliac lymph nodes (collectively denoted as ILN) has largely been overlooked when studying immune responses in the colon and this was the focus of the current work. Oral tolerance induction by feeding protein requires active transport of antigen by intestinal CD103+ DCs to the draining MLN,8 together with local cues from stromal cells within the MLN to which the migratory DCs respond.4 Thus, there is an active involvement of the draining lymphoid organ in orchestrating a functionally suppressive T-cell response to harmless antigens encountered at the small intestinal surface. The identification of the iliac and caudal lymph nodes as organizing centers of tolerogenic T-cell responses to distal colonic antigens emphasizes compartmentalization of mucosal immune responses along the length of the gastrointestinal tract and raised the question whether tolerance induction in ILN is coordinated differently when compared to MLN. The most striking difference from the MLN was the virtual absence of CD103+CD11b+ DCs in the colon-draining ILN. This is in line with earlier studies reporting declining numbers of CD103+CD11b+ DCs from proximal-to-distal along the length of the mouse intestine.22 However, this has never been taken into account when assessing tolerance induction in the colon. In the MLN, CD103+CD11b+ and CD103+CD11b− DCs express high levels of the retinoic acid-metabolizing enzyme RALDH2 which endows these cells with enhanced capacity to induce Foxp3+ Treg cells with gut-homing properties. That the two CD103+ DC subsets may have redundant functions in Treg cell maintenance is supported by findings of unaltered numbers of small intestinal Foxp3+ Treg cells in mice lacking one or other of the CD103+ subsets,29, 33, 35 whereas fewer small intestinal lamina propria Treg cells are detected in huLangerin-DTA × Batf3−/− mice lacking both CD103+CD11b+ DCs and CD103+CD11b− DCs.36 Here, we demonstrate that even in the absence of CD103+CD11b+ DCs, Foxp3 induction and functional Treg development was achieved in the ILN to the same extent as in the MLN. We observed that both CD103+CD11b− and CD103−CD11b+ DCs accumulate in the colon-draining ILN after colonic OVA administration, suggesting that these two subsets take part in the induction of colonic tolerance. Using Batf3−/− mice lacking CD103+CD11b− DCs, we could demonstrate that CD103−CD11b+ DCs were effective in supporting TGFβ-mediated Foxp3+ Treg cell differentiation and inducing systemic hyporesponsiveness to colonically applied antigen. Small intestinal CD103− DCs expressing high levels of CD11c and intermediate levels of CX3CR1 have been described to migrate in intestinal lymph to the MLN. These bona fide CD103− DCs express CCR7, show aldehyde dehydrogenase activity and are able to induce CCR9 expression on dividing T cells.12 In addition, colonic lamina propria CD11b+ DCs are efficient at presenting antigens to CD4+ T cells, while colonic CD103+CD11b− DCs cross-present antigens to CD8+ T cells suggesting a division of labour between these cell populations.37 Whether the function of CD103−CD11b+ and CD103+CD11b− DCs for inducing Treg differentiation in ILN is redundant awaits further studies.
Epithelial cells and stromal cells expressing high levels of retinoic acid-metabolizing enzymes imprint CD103+ DCs with vitamin A-metabolizing activity to support Foxp3+ Treg differentiation and the induction of gut-homing receptors on T cells.4, 9, 10, 38 Considering the absence of Aldh1a2-expressing CD103+CD11b+ DCs in the ILN, the very low levels of Aldh1a2 in whole ILN, and the low concentrations of vitamin A in the colon,19, 39 it appears that the ILN-derived DCs do not require vitamin A as a cofactor for Treg induction and their tissue-specific homing imprinting capacity. Indeed, the orphan G-protein coupled receptor GPR15 known to control homing of T cells to the large intestine in an RA-independent manner was highly expressed in ILN while Ccr9 mRNA expression was reduced compared with MLN. TGFβ, which was equally present at mRNA level in the ILN and MLN may contribute to DC-mediated Foxp3+ Treg induction in both these lymph nodes. It has been demonstrated previously that small intestinal CD103+ DCs can induce Foxp3+ Treg cells independently of RA via integrin αvβ8-mediated activation of TGFβ.15, 16 Therefore, similar to their MLN counterpart, activated ILN-derived DCs may express the TGFβ-activating integrin αvβ8 providing them with the ability to induce Foxp3+ Treg cell differentiation with TGFβ alone. Additionally, ILN-derived DCs may have the capacity to enhance Foxp3 conversion through production of COX-2-dependent prostaglandin metabolites acting as cofactors for the TGFβ-driven induction of Foxp3+ Treg cells.31 In particular, CD103−CD11b+ DCs from the ILN that were sufficient to induce mucosal tolerance after colonic antigen administration transcribed high levels of Ptgs2, suggesting that COX-2-dependent arachidonic acid metabolites may play a role in maintaining colonic homeostasis.
The model antigen ovalbumin was used to directly compare antigen trafficking and immune responses to luminal antigen in small intestine and colon. Using the same antigen for both routes allowed identification of antigen drainage, the type of antigen-presenting cells involved and the subsequent initiation of a T-cell response to protein antigen. In vivo, the proportion of Foxp3+ Treg cells in colonic lamina propria is approximately three times higher compared to the small intestinal lamina propria.21 In contrast to small intestine, most lamina propria Foxp3+ Treg cells in the colon show specificity to microbial protein. Our results will now allow for detailed analysis of the respective draining lymph nodes to determine the mechanisms underlying these differences. Besides tolerogenic responses, intestinal DCs drive inflammatory T-cell responses to pathogenic antigen. In the colon, bacterial antigen is virtually always encountered with a concomitant TLR signal which can enhance the migration of DCs from the intestinal lamina propria.40, 41 Indeed, colonic R848 administration enhanced CD103+CD11b− and CD103−CD11b+ DC migration and upregulated CCR7 expression on these cells when compared to OVA administration. In our experiments, endotoxin-free OVA was used and uptake of soluble OVA by DC was likely dependent on the mannose receptor.42 In inflammatory conditions, the different DC subsets may act disparately. Small intestinal CD103+CD11b+ DCs produce IL-23 and IL-6 and support intestinal Th17 responses,29, 43, 44 while CD103+CD11b− DCs are capable of cross-presenting antigen, inducing CD8+ T cell proliferation, and driving IFNγ production.45 Moreover, small intestinal CD103− DCs are efficient at priming naive T cells and support OVA-induced IFNγ and IL-17 production in vitro.12 Having identified the inductive site for distal colonic T-cell responses now allows us to investigate how CD103− and CD103+ DCs from ILN drive effector T-cell and Treg responses to bacteria in the large intestine.
Besides the MLN, the celiac and portal lymph nodes draining liver, pancreas and stomach also receive lymph from the small intestine. Functionally suppressive Foxp3+ Tregs are induced in these liver-draining lymph nodes after OVA feed.5 Unexpectedly, we observed that oral tolerance after antigen feeding is sustained in mice that underwent mesenteric lymphadenectomy (Supplementary Figure S5). It cannot be excluded that these surgical interventions elicit alterations in lymphoid drainage which might explain these results. Alternatively, it is possible that PP and/or liver-draining lymph nodes may compensate for the lack of MLN as both are inductive sites for functional Tregs after oral OVA administration.5, 6
Collectively, this study identifies the ILN as inductive site for distal colonic immune responses to soluble antigens and shows that T cell-mediated tolerance induction in the small and large intestine relies on different local regulatory mechanisms. Furthermore, these findings are likely essential for understanding how intestinal host-defence and immunopathology develops in the distal part of the colon.
METHODS
Mice
Male and female 6-12 week old specific pathogen free BALB/c mice (Charles River, The Netherlands), DO11.10 transgenic (Tg) mice having a T-cell receptor specific for the ovalbumin (OVA) 323-339 peptide, DO11.10 Tg × Rag−/− mice, C57BL/6 mice (Jackson Laboratory), C57BL/6 Rag1−/− OT-II Tg mice and C57BL/6 Batf3−/− mice from Taconic Farms bred on contract with the National Institute of Allergy and Infectious Diseases (NIAID), were housed in top-filter cages and fed a standard diet and water ad libitum. Studies conducted with BALB/c, DO11.10 Tg, DO11.10 Tg × Rag−/− mice were approved by the animal experimental committee of the Erasmus University Medical Center Rotterdam. Experiments conducted with C57BL/6, C57BL/6 Rag1−/− OT-II Tg, C57BL/6 Batf3−/− mice were under animal study protocol approved by the NIAID Animal Care and Use Committee.
Antibodies
Antibodies used include CD45 (30-F11), MHCII (M5/114.15.2), CD103 (2E7), CD11c (HL-3), F4/80 (BM8), CD11b (M1/70), CD197 (4B12), CD4 (RM4-5), Foxp3 (FJK-16s), IRF4 (3E4), IRF8 (V3GYWCH), and appropriate isotype controls, all purchased from BD Pharmingen, eBioscience or Biolegend. The DO11.10 Tg TCR specific antibody (KJ1.26) was obtained from Invitrogen (Breda, The Netherlands). 7-AAD (Molecular probes) was used for dead cell exclusion.
Flow cytometry
Fcγ receptors were blocked by preincubation with saturating amounts of anti-mouse CD16/32 (BD Pharmingen) or normal mouse serum. All stainings were performed in PBS containing 2% heat inactivated new born calf serum at 4°C, except for the CCR7 staining which was performed at 37 °C. For intracellular Foxp3, IRF4 and IRF8 staining, cells were stained for extracellular markers, fixed and permeabilized with Cytofix/Cytoperm solution (BD Pharmingen) prior to intracellular staining. Stained cells were analyzed using FACSCanto™ II (BD biosciences) and FlowJo software.
Intracolonic and oral administration of protein antigen or TLR7/8 ligand
Mice were given 70 mg OVA (Calbiochem, San Diego, USA) or 10 μg R848 (InvivoGen, San Diego, CA, USA) in saline intragastrically (i.g., 200 μl) or intracolonically (i.c., 150 μl) using a cannula that was inserted 2.5 cm (C57BL/6 Batf3−/−, C57BL/6) to maximally 4 cm (BALB/c, D011.10) proximal to the anus. The dosage of OVA was previously established to induce OVA-specific proliferative responses of TCR transgenic T cells in vivo.6 Intramuscular (i.m) immunization was achieved by giving 400 μg OVA in 15 μl saline in each hind limb. For tracking experiments, OVA was labeled with Alexa Fluor-488 succinimidyl ester according to manufacturer's protocol (Molecular Probes, Leiden, The Netherlands). Mice received 3.5 mg or 1.7 mg OVA-Alexa Fluor-488 by i.g. and i.c. administration, respectively. Control mice received similar amounts of unlabeled OVA. Mice were sacrificed at the indicated time points.
Adoptive transfer of T cells
Spleens were isolated from DO11.10 Tg × Rag−/− mice and labeled with 2 μM 5,6-carboxy-succinimidyl-fluoresceine-ester (CFSE) (Molecular Probes). Purity of the OVA-specific T cell population was assessed using flow cytometry. Subsequently, BALB/c acceptor mice received 6 × 106 CFSE-labeled CD4+KJ1.26+ T cells in 100 μl saline by intravenous injection into the tail vein. One day after adoptive transfer, recipient mice were given OVA by the indicated routes and 72 hours later, lymph nodes were examined for division and Foxp3 positivity of transferred CD4+KJ1.26+ T cells.
Analysis and sorting of dendritic cells
Lymph nodes were isolated and digested with Liberase TM (Roche, Woerden, The Netherlands) in the presence of DNAse I (Roche) at 37 °C after which single cell suspensions were prepared. FACS analysis of the DC subsets was performed on the digested cell suspension according to the indicated gating strategy (Supplementary Figure S1). For DC subset purification, CD11c+ cells were enriched from the digested cell suspensions using a mouse DC enrichment kit (Dynal, Oslo, Norway) or CD11c+ DC MACS separation kit (Miltenyi Biotech). To obtain purified DC subsets, flow-cytometric cell sorting based on the expression of CD45, F4/80, MHCII, CD11c, CD11b and CD103 was performed on a FACSAria flow cytometric cell sorter (BD biosciences) according to the indicated gating strategy.
T cell differentiation assay
CD11chighMHCIIhigh DCs were purified from lymph nodes of C57BL/6 and Batf3−/− mice as described above. For the isolation of OVA-specific naive T cells, spleens were obtained from C57BL/6 Rag1−/− OT-II Tg mice. Naive CD4+ T cells were enriched using CD4+ T cell MACS separation kit (Miltenyi Biotech) and subsequently FACSsorted based on the expression of CD4, CD45RB and CD25 to obtain >99% pure population. The purified naive OVA-specific CD4+ T cells were labeled with CFSE (Invitrogen) at a final concentration of 2 μM. For the in vitro stimulation assays, dendritic cells were cultured with 2 mg/ml OVA protein for 1 hours at 37 °C. Subsequently, cells were washed extensively with sterile PBS after which the CFSE-labeled T cells were added at a 1:10 ratio in the presence or absence of 2 ng/ml hTGFβ (R&D Systems Inc). At 72 hours, cells were analyzed for division and Foxp3 expression.
Surgical removal of mesenteric lymph nodes
Mesenteric lymphadenectomy was carried out by microdissection along the length of the superior mesenteric artery to the aortic root.8, 46 Mice were anesthetized with 2-5% isoflurane in oxygen and abdominal tissues were wetted with sterile saline to prevent drying. After surgery, mice received Finadyne subcutaneously (2.5 mg/kg for 2 consecutive days) and animals’ activity was monitored for 1 week. Animals were allowed to recover for 6 weeks after surgery before mice were subjected to tolerance induction and a subsequent delayed-type hypersensitivity response. At the end of the experiment, successful mesenteric lymphadenectomy was established by two independent observers.
Delayed-type hypersensitivity response
BALB/c, C57BL6 or Batf3−/− mice received 25 mg OVA (Calbiochem, San Diego, USA) dissolved in saline either orally or intracolonically as described above. Three to five days after OVA administration, mice were sensitized subcutaneously in the tail base with 100 μg OVA emulsified in incomplete Freund's adjuvant (BALB/c) (Difco, BD. Alphen a/d Rijn, The Netherlands) or complete Freund's adjuvant (C57BL6 or Batf3−/− mice) (Sigma-Aldrich). Five days after sensitization, mice were challenged with 10 μg OVA in 10 μl saline in both ears. After 24h (BALB/c) or 48h (C57BL6 or Batf3−/− mice), increases in ear-thickness were determined and compared to values prior to challenge. To transfer tolerance to a second acceptor mice, BALB/c mice were adoptively transferred with 6 × 106 CFSE-labeled CD4+KJ1.26+ T cells and received OVA i.g., i.c. or i.m. one day later. At 72 h after OVA administration, draining lymph nodes were isolated and enriched for CD4+ T cells by the depletion of B cells, macrophages, monocytes and CD8+ T cells using rat antibodies against B220 (6B2), F4/80, CD11b (MAC-1), MAC-2, MHC-II and CD8 (53.6.72) and anti-rat magnetic Dynabeads (Invitrogen). Purity of the OVA-specific T cell population was assessed using flow cytometry and subsequently 1 × 105 CD4+KJ1.26+ T cells were transferred to naive second acceptor BALB/c mice. One day after the transfer, recipient mice were sensitized with OVA/IFA followed by a delayed-type hypersensitivity challenge in the ears 5 days later. After 24h, increases in ear-thickness were determined and compared to values prior to challenge.
OVA restimulation assay
CD4+ T cells were purified from inguinal lymph nodes (draining immunization site) of Batf3−/− mice after induction of a DTH reaction using the CD4+ T cell MACS separation kit (Miltenyi Biotech). For DC isolation, spleens from untreated C57BL/6 wild-type mice were isolated and digested with Liberase TL (Roche, Indianapolis, IN, USA) in the presence of DNAse I (Roche) at 37 °C. CD11c+ cells were purified from the digested cell suspensions using a CD11c+ DC MACS separation kit (Miltenyi Biotech). For in vitro restimulation, 1×104 CD11c+ cells were pulsed with 0.5 mg/ml OVA protein and cocultured with 1×105 CD4+ T cells for 48 hours. Alternatively, 5×105 cells from the inguinal lymph nodes of MLN-resected mice were restimulated with OVA protein. Subsequently, protein levels of IFNγ were measured in culture supernatant by mouse ELISA set kit (Biolegend, San Diego, CA, USA) according to the manufacturer's instructions. The sensitivity of the assay was 4 pg/ml.
RNA isolation, amplification and quantitative PCR analysis
Total RNA was extracted using the Nucleospin RNA-XS kit (Macherey-Nagel, Düren) and reverse transcribed into cDNA using a mix of random hexamers (2.5 μM), oligo(dT) primers (20 nM), dNTP (0.2 mM), M-MLV (200 units, Promega) and RNAsin (25 units, Promega). For DC subset analysis, RNA quality was ensured using the RNA6000 PicoAssay for the Bioanalyzer 2100 (Agilent) before RNA amplifications were performed using the Ovation PicoSL WTA system V2 (Nugen) according the manufacturers’ protocols. Quantitative real-time PCR was performed using the ABI/Prism 7900 sequence detection system (Applied Biosystems, Foster City, CA). Quantification of PCR signals was achieved by calculating the difference between the cycle threshold value (Ct) of the gene of interest with Ct value of their reference gene cyclophillin (ΔCt). The relative expression to cyclophilin for each gene was measured as 2(−ΔCt). Primer sets used were Ppia (Cyclophilin); Fw: 5’-AACCCCACCGTGTTCT-3’, Rv: 5’-CATTATGGCGTGTAAAGTCA-3’, Foxp3; Fw: 5’-ACCTGGGATCAATGTGG-3’, Rv: 5’-TGGCAGTGCTTGAGAAA-3’, Aldh1a2 (RALDH2); Fw: 5’-AGCCCATTGGAGTGTGT-3’, Rv: 5’-CCAGCCTCCTTGATGAG-3’, Ptgs2 (COX-2); Fw: 5'-ACCCGGACTGGATTCTAT-3', Rv: 5'-GCTTCCCAGCTTTTGTAA-3', Itgae (CD103); Fw: 5’- CAGGGGAGGAGAAGAAGT-3’, Rv: 5’-TGGCCTTCTGGAAGTCT-3’, Ccr7; Fw: 5’- TGGCTCTCCTTGTCATTT-3’, Rv: 5’-CCGCACATCCTTCTTG-3’, Batf3; Fw: 5’-CCCCAAGGACGATGAC-3’, Rv: 5’-AGCGGGCACATCTTCT-3’, Tgfb1; Fw: 5'-AATTCCTGGCGTTACCTT-3', Rv: 5'-ATTCCGTCTCCTTGGTTC-3', Gpr15; Fw: 5’-TCGGGAAAGCATAACAAG-3’, Rv: 5’-GGCCCAGTCACATTCAT-3’, Ccr9; Fw: 5'-TGCCCACAGAACTCACA-3', Rv: 5'-GCCCACAATGAACACAA-3'
Statistical analysis
Significance was determined using the Student's t-test or Mann-Whitney U test or for multiple groups, one-way analysis of variance (ANOVA) performed on GraphPad Prism 4.0 software (GraphPad Software, San Diego CA). P-values of 0.05 or less were regarded as significant.
ACKNOWLEDGMENTS
The authors gratefully acknowledge E. Stregevsky from the NIAID sorting facility, I. Hekking, M. Muijtjens, E. Fijneman from the ErasmusMC SkillsLab, and the animal caretakers at the Erasmus University Medical Center Rotterdam. This work was supported by the Dutch Digestive Foundation grant (WO 10-37) and Sophia Foundation grant 470.
Footnotes
The authors have no conflicting financial interests.
SUPPLEMENTARY MATERIAL is linked to the online version of the paper at http://www.nature.com/mi
DISCLOSURE
The authors declare no conflict of interest.
REFERENCES
Full text links
Read article at publisher's site: https://doi.org/10.1038/mi.2015.118
Read article for free, from open access legal sources, via Unpaywall:
https://www.nature.com/articles/mi2015118.pdf
Citations & impact
Impact metrics
Citations of article over time
Alternative metrics
Smart citations by scite.ai
Explore citation contexts and check if this article has been
supported or disputed.
https://scite.ai/reports/10.1038/mi.2015.118
Article citations
The renaissance of oral tolerance: merging tradition and new insights.
Nat Rev Immunol, 06 Sep 2024
Cited by: 0 articles | PMID: 39242920
Review
Regulatory T cells in the face of the intestinal microbiota.
Nat Rev Immunol, 23(11):749-762, 14 Jun 2023
Cited by: 17 articles | PMID: 37316560
Review
The intestinal microenvironment shapes macrophage and dendritic cell identity and function.
Immunol Lett, 253:41-53, 07 Jan 2023
Cited by: 7 articles | PMID: 36623708 | PMCID: PMC9907447
Review Free full text in Europe PMC
Diet, microbiota, and the mucus layer: The guardians of our health.
Front Immunol, 13:953196, 13 Sep 2022
Cited by: 33 articles | PMID: 36177011 | PMCID: PMC9513540
Review Free full text in Europe PMC
Effect of the Microbiome on Intestinal Innate Immune Development in Early Life and the Potential Strategy of Early Intervention.
Front Immunol, 13:936300, 19 Jul 2022
Cited by: 8 articles | PMID: 35928828 | PMCID: PMC9344006
Review Free full text in Europe PMC
Go to all (37) article citations
Data
Data behind the article
This data has been text mined from the article, or deposited into data resources.
BioStudies: supplemental material and supporting data
Similar Articles
To arrive at the top five similar articles we use a word-weighted algorithm to compare words from the Title and Abstract of each citation.
Mesenteric lymph node CD11b- CD103+ PD-L1High dendritic cells highly induce regulatory T cells.
Immunology, 152(1):52-64, 01 Jun 2017
Cited by: 34 articles | PMID: 28423181
Peripheral CD103+ dendritic cells form a unified subset developmentally related to CD8alpha+ conventional dendritic cells.
J Exp Med, 207(4):823-836, 29 Mar 2010
Cited by: 497 articles | PMID: 20351058 | PMCID: PMC2856032
A new subset of CD103+CD8alpha+ dendritic cells in the small intestine expresses TLR3, TLR7, and TLR9 and induces Th1 response and CTL activity.
J Immunol, 186(11):6287-6295, 27 Apr 2011
Cited by: 99 articles | PMID: 21525388
Subsets of migrating intestinal dendritic cells.
Immunol Rev, 234(1):259-267, 01 Mar 2010
Cited by: 63 articles | PMID: 20193024
Review
Funding
Funders who supported this work.
Intramural NIH HHS (1)
Grant ID: Z01 AI000833-10