Abstract
Background
An excessive inflammatory response after myocardial infarction (MI) increases myocardial injury. The toll-like receptor (TLR)-4 is activated by the recognition of endogenous ligands and is proinflammatory when there is myocardial tissue injury. The apoptosis inhibitor of the macrophage (AIM) is known to provoke an efflux of saturated free fatty acids (FFA) due to lipolysis, which causes inflammation via the TLR-4 pathway. Therefore, this study investigated the hypothesis that AIM causes a proinflammatory response after MI.Methods and results
The left anterior descending coronary artery was ligated to induce MI in both AIM-knockout (AIM(-/-)) and wild-type (WT) mice. After 3 days, the inflammatory response from activation of the TLR-4/NFκB pathway was assessed, and infarct size was measured by staining with triphenyltetrazolium chloride. In addition, left ventricular remodeling was examined after 28 days. Although the area at risk was similar between AIM(-/-) and WT mice, the infarct size was significantly smaller in AIM(-/-) mice (P=0.02). The heart weight-to-body weight ratio and myocardial fibrosis were also decreased in the AIM(-/-) mice, and the 28-day survival rate was improved (P<0.01). With the reduction of plasma FFA in AIM(-/-) mice, myocardial IRAK4 and NFκB activity were decreased (all P<0.05). Moreover, there was a reduction in myeloperoxidase activity and inducible nitric oxide synthase as part of the inflammatory response (P<0.01, P=0.03, respectively). Furthermore, NFκB DNA-binding activation via TLR-4, neutrophil infiltration, and inflammatory mediators were decreased in AIM(-/-) mice.Conclusions
The deletion of AIM reduced the inflammatory response and infarct size and improved survival after myocardial infarction.Free full text

Deletion of Apoptosis Inhibitor of Macrophage (AIM)/CD5L Attenuates the Inflammatory Response and Infarct Size in Acute Myocardial Infarction
Abstract
Background
An excessive inflammatory response after myocardial infarction (MI) increases myocardial injury. The toll‐like receptor (TLR)‐4 is activated by the recognition of endogenous ligands and is proinflammatory when there is myocardial tissue injury. The apoptosis inhibitor of the macrophage (AIM) is known to provoke an efflux of saturated free fatty acids (FFA) due to lipolysis, which causes inflammation via the TLR‐4 pathway. Therefore, this study investigated the hypothesis that AIM causes a proinflammatory response after MI.
Methods and Results
The left anterior descending coronary artery was ligated to induce MI in both AIM‐knockout (AIM−/−) and wild‐type (WT) mice. After 3 days, the inflammatory response from activation of the TLR‐4/NFκB pathway was assessed, and infarct size was measured by staining with triphenyltetrazolium chloride. In addition, left ventricular remodeling was examined after 28 days. Although the area at risk was similar between AIM−/− and WT mice, the infarct size was significantly smaller in AIM−/− mice (P=0.02). The heart weight–to–body weight ratio and myocardial fibrosis were also decreased in the AIM−/− mice, and the 28‐day survival rate was improved (P<0.01). With the reduction of plasma FFA in AIM−/− mice, myocardial IRAK4 and NFκB activity were decreased (all P<0.05). Moreover, there was a reduction in myeloperoxidase activity and inducible nitric oxide synthase as part of the inflammatory response (P<0.01, P=0.03, respectively). Furthermore, NFκB DNA‐binding activation via TLR‐4, neutrophil infiltration, and inflammatory mediators were decreased in AIM−/− mice.
Conclusions
The deletion of AIM reduced the inflammatory response and infarct size and improved survival after myocardial infarction.
Introduction
Myocardial infarction (MI) causes an inflammatory response that plays a crucial role in infarct repair and subsequent damage.1, 2, 3 However, excessive inflammation generates reactive oxygen species (ROS) in the injured myocardium,4, 5 including complement initiation and neutrophil infiltration,6, 7, 8 which can trigger an inflammatory cascade through cytokine expression and direct damage of cardiac myocytes.1 Therefore, it is important to prevent activation of radical inflammatory pathways to reduce infarct size and promote cardiac repair, which can preserve cardiac function.
Apoptosis inhibitor of the macrophage (AIM)/CD5L protein is a member of the scavenger receptor cysteine‐rich domain superfamily (SRCR‐SF), which directly inhibits apoptosis of macrophages. Furthermore, AIM is also a direct target for antiapoptotic regulation by LXR/RXR heterodimers.9 AIM was shown to induce an efflux of saturated free fatty acids (FFAs), such as palmitic and stearic acid, due to lipolysis in obese adipose tissue, which leads to the production of inflammatory cytokines and neutrophil recruitment through toll‐like receptor (TLR)‐4 activation.10, 11, 12 This can lead to chronic and sustained inflammation, which is closely associated with insulin resistance and progression of atherosclerosis and contributes to future cardiovascular events.13, 14 In chronic inflammation due to AIM, the TLR‐4/nuclear factor‐kappa B (NFκB) pathway, activated by endogenous extracellular FFAs, plays an important role in the development of inflammation.15, 16
TLR‐4 is activated by the recognition of not only pathogen‐associated molecular patterns, such as bacterial lipopolysaccharide (LPS),17 but also endogenous host‐derived ligands including heat shock protein 60 (HSP60), high‐mobility group box 1 (HMGB‐1), extradomain degradation products of the extracellular matrix (ECM), and free fatty acids (FFA) in innate immunity.14, 15, 16, 18, 19, 20, 21, 22
They are produced as a result of cell death or injury, which stimulates cell surface TLRs, resulting in an inflammatory response. Our previous studies as well as studies by other investigators demonstrated that TLR‐4 is involved in the proinflammatory response to ischemic myocardial tissue injury without the need for any microbial pathogens.23, 24
However, it is still unclear what triggers this response and enhances the inflammatory signals including TLR‐4 signaling in MI. Therefore, deletion of AIM can decrease FFA and may reduce the signaling through TLR‐4 in the process of myocardial injury. Based on this hypothesis, we investigated whether the deletion of AIM affects the degree of myocardial damage or the inflammatory response following myocardial injury using AIM knockout (AIM−/−) mice.
Methods
Experimental Animals
AIM−/− mice were obtained from Prof Toru Miyazaki at Tokyo University. The AIM−/− mice were generated by the targeting vector that was prepared using a 7‐kb SpeI‐BamHI fragment. It was inserted into the disrupted NcoI site in exon 3, which encodes the second SRCR domain.25 AIM−/− without the recognition sequence of BamHI was confirmed using cutting by a BamHI restriction enzyme and agarose gel electrophoresis. The primer used had the following sequences: F, AIM4000F 5′‐AAT GGT TCT TCA TAT AGC TTT GGT–3′; R, AIM4400R 5′‐GAT GTG GTA CAT ATA CAC AAT AGA TCT‐3′. Moreover, we confirmed no AIM protein expression by Western blotting. AIM−/− mice had been backcrossed to C57BL/6 for at least 13 generations before being used for experiments.
The C57BL/6 mice, which were used as a control group, were purchased from Kyudo Co, Ltd (Tosu, Japan). Animals were housed in a specific pathogen‐free environment. All experiments were performed in accordance with the “Position of the American Heart Association on Research Animal Use” and Saga University Standing Committee rules on animal research.
Surgery to Achieve Myocardial Infarction
Mice (aged 12–16 weeks) were anesthetized with ketamine (100 mL/kg) and xylazine (10 mg/kg) and intubated for mechanical ventilation. A left thoracotomy was performed to expose the heart, and MI was achieved by ligation of the left anterior descending coronary artery using an 8‐0 silk suture. After the chest wall was closed, the mice were extubated, and the hearts were harvested at 3 and 28 days after surgery.
Measurement of Infarct Size
The mice were anesthetized as described above at 3 days, and a catheter was inserted into the aortic root via the internal carotid artery. Then, 1% Evans blue was infused into the aorta and coronary arteries to assess the area at risk. The hearts were sliced transversely into 2‐mm‐thick sections and incubated with 1% triphenyltetrazolium chloride solution (TTC) (Sigma Aldrich Chemical, Buchs, Switzerland) at 37°C for 15 minutes, as previously described.23 The viable myocardium was stained brick red by the TTC, the area at risk (AAR) was the myocardial tissue not stained by Evan's blue, and the infarct area was that portion of the AAR that was not stained by TTC. The AAR and infarct size (IS) were measured in each section using Image J software.
Echocardiography and Hemodynamic Analysis
Echocardiographic analysis was performed at 28 days using an echocardiographic machine with a 15‐MHz transducer (Toshiba, Tokyo, Japan), From 2‐dimensional echocardiography in the short‐axis view, the left ventricular end‐diastolic diameter (LVEDD) and end‐systolic diameters (LVESD) were measured, and fractional shortening (%FS) was calculated as [(LVEDD−LVESD)/LVEDD×100 ], as previously desribed.23 A 1.2 Fr catheter (Transonic Systems Inc, Ithaca, NY) was inserted into the left ventricle through the right carotid artery, and the hemodynamic values were measured.
Histological Analysis
Samples were taken from cardiac tissue around the infarct region and fixed in 4% formalin for 24 hours before cryopexy. Left ventricular sections (5 μm) were stained with hematoxylin‐eosin. In addition, the collagen density was assessed using Masson trichrome stain, as previously described.23 The total area of fibrosis was analyzed quantitatively using a BZ‐X710 all‐in‐one microscope (KEYENCE, Osaka, Japan). In immunohistochemistry stains using the neutrophil‐specific maker Gr‐1 (Ly‐6G; #550291, 1:10, BD BioScience, SanJose, CA), we analyzed neutrophil infiltrations on hearts from 3 mice in each group. Secondary antibodies consisted of biotinylated anti‐rat antibodies, followed with streptavidin‐peroxidase staining kit (Histofine Simple Stain MaxPO Multi, Nichirei, Tokyo, Japan) and visualized with a peroxidase substrate solution containing 3,3′‐diaminobenzidine (Simple Stain DAB, Nichirei, Tokyo, Japan).
Myocardial Myeloperoxidase Activity Assay
The myocardial myeloperoxidase (MPO) activity was measured as an index of myocardial neutrophil infiltration with the Neutrophil Myeloperoxidase Activity Assay Kit (#600620, Cayman Chemical, Ann Arbor, MI) according to the manufacturer's instructions.23
Thiobarbituric Acid Reactive Substances Assay
Lipid peroxidation is a mechanism of cell damage by reactive oxygen species derived from polyunsaturated fatty acids, and thiobarbituric acid reactive substances (TBARS) are end products of the lipid peroxidation. We measured TBARS levels as an index of oxidative stress with myocardial tissues using The OxiSelect™ TBARS Assay kit (STA‐330, Cell Biolabs, San Diego, CA).
Western Immunoblotting Assay
Heart sections were homogenized in cytoplasmic and nuclear extraction regents (Thermo Scientific, Hudson, NH) containing protease inhibitors. Samples of myocardium lysate were resolved on SDS‐PAGE according to a standard protocol. After transfer to membranes, the samples were immunoblotted with primary antibodies followed by secondary antibodies conjugated to horseradish peroxidase, and bands developed with ECL Plus Western Blotting Detection Regents (GE Healthcare, Buckinghamshire, UK). Enhanced chemiluminescence was detected with an LAS‐3000 image analyzer (Fujifilm, Tokyo, Japan), and band density was quantified using Image J software. The following primary antibodies were used: NFκB p65 (#3034, 1:250), IRAK4 (#4363, 1:500), FASN (#3189, 1:500) (Cell Signaling Technology, Danvers, MA), β‐actin I‐19 (sc‐1616, 1:1000), Histon H1 AE‐4 (sc‐8030, 1:500) (Santa Cruz Biotechnology, Santa Cruz, CA), and iNOS (#610432, 1:1000) (BD Transduction Laboratories, Lexington, KY).
Nuclear Factor‐kB Activity Analysis
The DNA‐binding activity of NFκB p65 was measured from the nuclear extract using a TransAM NFκB Transcription Factor ELISA Kit, according to the manufacturer's protocol (Active Motif, Carlsbad, CA).
Plasma Free Fatty Acid Assay
The concentrations of plasma FFAs were determined by using the EnzyChrom Free Fatty Acid Assay Kit (BioAssay Systems, Hayward, CA). The FFA was enzymatically converted to acyl‐CoA and subsequently to H2O2, and FFA concentrations were measured using a colorimetric procedure for H2O2.
Statistical Analysis
Data are expressed as mean±SEM. Comparisons between the groups were made using a 2‐way analysis of variance (ANOVA), Tukey post hoc test. A P‐value <0.05 was deemed statistically significant. Survival rates were analyzed by the Kaplan–Meier method. There was no adjustment for multiple endpoints. All statistical analyses were performed using the statistics package of SPSS software (version 22; IBM SPSS, Chicago, IL).
Results
Reduced Myocardial Infarct Size in AIM Knockout Mice
The deletion of AIM was confirmed using gel electrophoresis and Western blotting in AIM−/− mice (Figure 1A). On day 3 after MI, the AAR/LV was not different between the AIM−/− and WT mice (AAR/LV; 43.2±1.5% vs 40.8±2.3%; P=0.56). However, the IS/LV and IS/AAR were significantly smaller in AIM−/− than in WT mice (IS/LV; 20.8±0.6% vs 27.8±1.9%; P=0.02, IS/LV; 8.5±0.1% vs 11.1±0.9%; P=0.02) (Figure 1B). The survival rate at 28 days after MI in AIM−/− mice was significantly greater than that in WT mice (80.0% vs 40.0%, respectively; P=0.039) (Figure 1C).
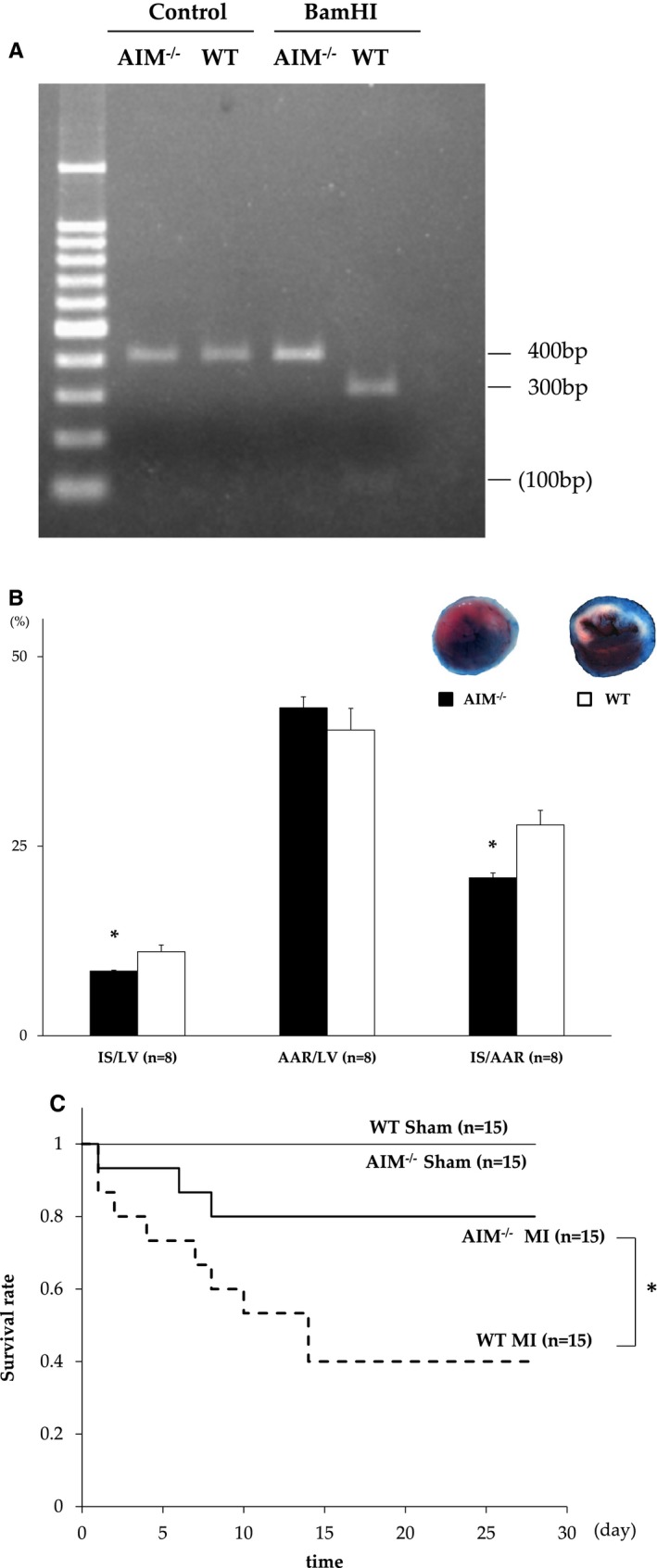
A, AIM knockout (AIM−/−) mice without the recognition sequence of BamHI were confirmed using cutting by a BamHI restriction enzyme in gel electrophoresis. PCR product (400 bp) amplified from the AIM−/− allele was not digested, yielding only the 400‐bp band, whereas the wild‐type (WT) allele was digested into 100+300 bp. B, Myocardial infarct (MI) size and area at risk after MI between AIM−/− (n=8) and WT mice (n=8). The blue area is perfused tissue, the red and white area is the area at risk (AAR), and the white area is infarcted tissue (IS). The graphs show the IS as a percentage of the left ventricular (LV) area (IS/LV), the AAR as a percentage of LV area (AAR/LV), and IS as a percentage of AAR (IS/AAR). C, Kaplan‐Meier survival estimates in AIM−/− MI mice (n=15) and WT MI mice (n=15) 28 days after MI. All of AIM−/− Sham mice (n=15) and WT Sham mice (n=15) survived. AIM indicates apoptosis inhibitor of the macrophage. *P<0.05.
We evaluated the influence of AIM− deletion on cardiac dysfunction after 28 days. The reduction in %FS in the AIM−/− mice was not as remarkable as that in WT mice. In hemodynamic analyses, systolic blood pressure and positive dp/dt after MI were higher in the AIM−/− mice. In addition, the heart weight–to–body weight ratio was significantly lower in the AIM−/− than in the WT mice (Table). Furthermore, myocardial interstitial fibrosis was significantly less in the AIM−/− than in the WT mice after MI (P=0.02) (Figure 2A and and2B).2B). These findings indicate that the deletion of AIM led to a reduction in infarct size and systolic dysfunction and to less subsequent myocardial remodeling. Myocardial infarction elicits an intense inflammatory response with ROS and neutrophil infiltration. Neutrophils and monocytes have abundant MPO, and MPO‐derived ROS damages myocardial tissue.26 There was less MPO activity as a quantitative assessment of neutrophil infiltration in the heart from AIM−/− than from WT mice (0.13±0.003 vs 0.16±0.006 unit/100 mg tissue; P<0.01) (Figure 2C). And TBARS levels as markers of ROS were lower in AIM−/− mice (0.95±0.07 vs 1.55±0.21; P=0.04) (Figure 2D).
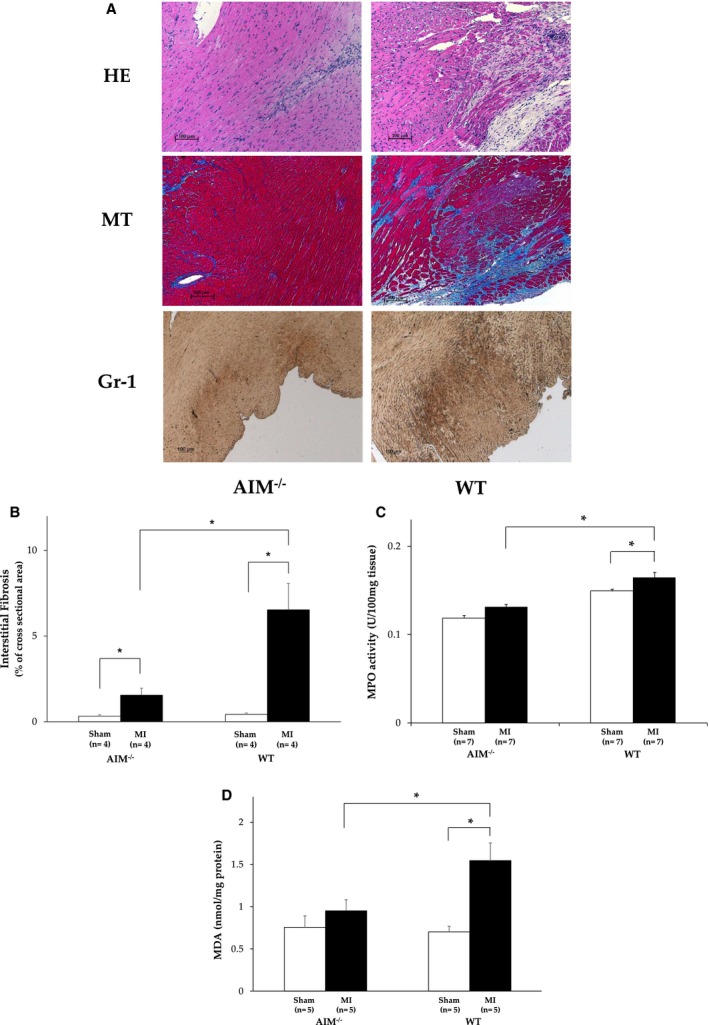
A, The histological sections of hearts from AIM knockout (AIM−/−) mice (n=4) and wild‐type (WT) mice (n=4) after myocardial infarction (MI). Each section was stained with hematoxylin‐eosin (HE), and Masson trichrome (MT) stains. Sections were stained with Gr‐1 antibody as a neutrophil‐specific marker. B, The fibrosis was measured by quantitation with Masson trichrome staining. C, Myeloperoxidase (MPO) activity in hearts from AIM knockout (AIM−/−) mice and wild‐type (WT) mice. Units of MPO activity in hearts were expressed as units per 100 mg tissue weight. D, Lipid peroxidation products malondialdehydes (MDA) as an index of oxidative stress. Units were nanomole per milligram of protein. AIM indicates apoptosis inhibitor of the macrophage. *P<0.05.
Table 1
Differences in Echo Parameters, Hemodynamics, and Cardiac/Body Weights between AIM Knockout (AIM−/−) Mice and Wild‐Type (WT) Mice after 28 Days
Parameter | AIM−/−+Sham | AIM−/−+MI | WT+Sham | WT+MI |
---|---|---|---|---|
BW, g | 28.0±0.5 | 28.3±0.5 | 28.9±0.6 | 29.7±0.8 |
HW, mg | 185.7±8.0 | 222.3±17.5a | 176.1±12.4 | 283.0±16.4b |
HW/BW, mg/g | 6.62±0.23 | 7.85±0.55a, b | 6.07±0.38 | 9.52±0.55b |
HR, bpm | 477.4±23.4 | 473.0±16.2 | 454.1±14.0 | 439.0±26.4 |
Systolic BP, mm Hg | 91.0±7.5 | 94.9±2.7a, b | 104.2±7.5 | 78.8±3.0 |
Max dp/dt | 4573.2±86.3 | 4066.7±148.7a, b | 5089.2±577.4 | 3492.3±199.9b |
LVDd, mm | 3.24±0.29 | 3.60±0.19 | 3.29±0.11 | 3.61±0.14 |
LVDs, mm | 2.06±0.23 | 2.48±0.22 | 2.21±0.08 | 2.88±0.14 |
IVS, mm | 0.81±0.09 | 0.81±0.04 | 0.84±0.07 | 0.83±0.04 |
PW, mm | 0.79±0.04 | 0.85±0.06 | 0.78±0.05 | 0.76±0.03 |
FS, % | 32.5±1.18 | 28.4±2.58a, b | 34.8±1.4 | 20.5±1.42b |
AIM indicates apoptosis inhibitor of the macrophage; bpm, beats/min; BW, body weight; FS, ratio of left ventricular fractional shortening; HR, heart rate; HW, heart weight; IVS, thickness of interventricular septum; LVDd, diastolic dimension of left ventricle; LVDs, systolic dimension of left ventricle; MI, myocardial infarction; PW, thickness of posterior wall.
The Effect of AIM Deletion on Inflammation via the TLR‐4/NFκB Pathway
Previously, it was shown that AIM‐induced lipolysis provokes an efflux of FFAs in adipose tissue, which activates the TLR‐4 pathway and causes chemokine production.11 Therefore, we examined whether the inflammatory response via the TLR‐4 pathway was attenuated after MI in AIM−/− mice, because we have shown previously that TLR‐4 deficiency reduces the inflammatory response that is associated with expansion of myocardial injury after myocardial ischemia reperfusion.23 In AIM−/− mice, plasma FFAs were decreased as compared with WT mice after 3 days, although there was no difference in baseline FFAs (224.1±11.6 μmol/L vs 299.7±16.2 μmol/L; P<0.01) (Figure 3A). In addition, we measured cytoplasmic fatty acid synthase (FAS) expression with Western blotting, and cytoplasmic FAS expression was increased in the AIM−/− mice (P=0.04) (Figure 3B).
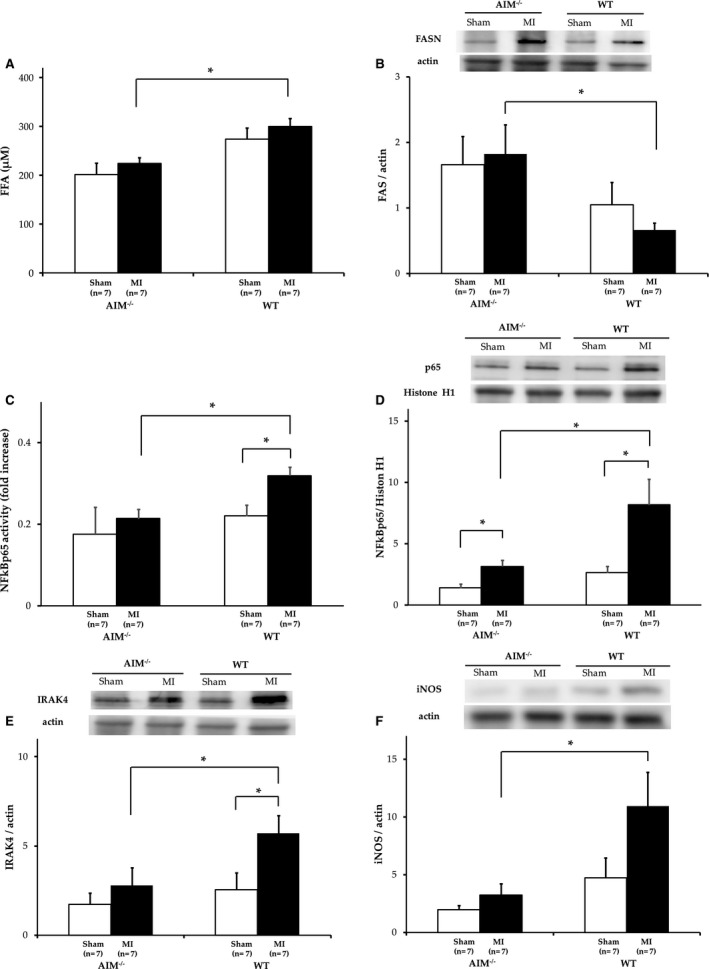
A, Serum concentrations of free fatty acid (FFA) were expressed. B, Fatty acid synthase (FASN) in hearts from AIM−/− mice and wild‐type (WT) mice measured by Western blot analysis. C, NFκB activity was measured by NFκB DNA binding assay. D, NFκB activity was quantified by Western blot analysis. E, Interleukin receptor‐associated kinase 4 (IRAK4) was measured by Western blot analysis. F, Inducible nitric oxide synthase (iNOS) was measured by Western blot analysis. All data without serum FFA concentrations were obtained in hearts from AIM−/− mice and WT mice after myocardial infarction (MI) 3 days. AIM indicates apoptosis inhibitor of the macrophage. *P<0.05.
After MI, the NF‐κB activity in myocardial nuclear extracts was significantly less in AIM−/− than in WT mice (P<0.01), and NF‐κB p65 protein expression in Western blot analysis was decreased in spite of there being no significant difference between the 2 groups before MI (P=0.04) (Figure 3C and and3D).3D). In addition, IL‐1 receptor‐associated kinase (IRAK)‐4,27 which is essential for NFκB activation in TLR‐4–mediated signal transduction, was also significantly less in AIM−/− mice than in WT mice (P=0.02) (Figure 3E). In AIM−/− mice, the reduction in IRAK‐4 linked to NFκB may mean less inflammation via the TLR‐4/NFκB pathway after left anterior artery ligation. We next examined whether the reduction of activation of the TLR‐4/NFκB pathway led to suppression of cytokine production. Inducible nitric oxide synthase (iNOS) induced by inflammatory cytokines produces a high level of nitric oxide and causes myocardial damage through ROS such as peroxynitrate, although this plays a beneficial role in the early defensive inflammatory response against reperfusion injury.28 After MI, iNOS expression was significantly decreased in AIM−/− mice compared with WT mice (P=0.03) (Figure 3F). Therefore, in summary, the reduction of plasma FFAs by AIM deletion attenuated the inflammatory response via TLR‐4 activation after MI, and this subsequently improved cardiac function and the survival rate (Figure 4).

A diagram that shows less inflammation is associated with expansion of cardiac injury by AIM deletion after myocardial infarction (MI). AIM knockout (AIM−/−) mice have decreased free fatty acids (FFA) due to lipolysis, which leads to TLR‐4 inactivation and the subsequent suppression of inflammation. AIM indicates apoptosis inhibitor of the macrophage; IRAK4, Interleukin receptor‐associated kinase 4; LV, left ventricular; MPO, myocardial myeloperoxidase; NFκB, nuclear factor‐κB; ROS, reactive oxygen species; TLR4, Toll‐like receptor‐4.
Discussion
We demonstrated that the deletion of AIM reduced infarct size and the inflammatory response due to neutrophil infiltration after coronary artery ligation in mice. This decreased inflammatory response resulted in a reduction in interstitial fibrosis, heart weight, and the magnitude of cardiac remodeling, and this subsequently improved the survival rate. It suggested that AIM played an important role for the sustained inflammation and the progression of cardiac remodeling after MI.
AIM and TLR‐4 Signaling in MI
Irreversible ischemic injury after MI results in myocardial necrosis, which prolongs and expands the inflammatory response. The deleterious signals released from necrotic cells and the extracellular matrix amplify the inflammatory response by activation of TLR signaling.29, 30 Several studies have shown that TLR‐4 signaling is activated by cardiac injury, which promotes an inflammatory cascade through the TLR‐4/NFκB pathway.1 Previously, we demonstrated that TLR‐4 caused a proinflammatory response that expanded myocardial injury after myocardial ischemia‐reperfusion. This proinflammatory response included complement initiation, neutrophil infiltration, and ROS generation.23 TLR‐4–deficient mice exhibited a smaller infarct size with suppression of inflammatory reactions and less adverse remodeling following MI.31 However, the trigger of this response is unknown, and it is also not clear what activated TLR4 signaling after MI.
On the other hand, AIM provokes release of FFAs due to lipolysis from adipose tissue via CD36‐mediated endocytosis, and FFAs play a major role in the activation of NFκB via the TLR‐4 pathway as the endogenous ligand in a chronic inflammatory response.15 Our results suggested that NF‐κB and IRAK‐4 in the TLR‐4/NF‐κB pathway were activated after coronary ligation, but there was less of a response in AIM−/− mice compared with WT mice. As the infiltration of neutrophils and subsequent production of ROS expand myocardial tissue injury,1 the activities of MPO and iNOS were also decreased in AIM−/− mice after MI. The kinase activity of IRAK‐4 was reported to be essential for regulation of a TLR‐4–mediated innate immune response, leading to activation of NFκB in MyD88, dependent and independent of the signaling pathway.27, 32, 33 The suppression of the TLR‐4/NFκB inflammatory cascade via IRAK‐4 resulted in less activity of MPO and iNOS in mice that lacked AIM. These data suggest that the smaller infarct size and attenuation of cardiac remodeling reflected the suppression of inflammation after MI in AIM−/− mice. We thought that the increase in plasma FFAs due to AIM might have activated a proinflammatory response via the TLR‐4 pathway after MI. Otherwise, in the presence of neutrophil infiltration and cytokine expression due to AIM via TLR‐4, it is possible that the endogenous deleterious signals including HSP 60, HMGB‐1, and ECM‐derived components caused a stronger inflammatory response via the same TLR‐4 pathway responsible for neutrophil infiltration after MI.
Role of Fatty Acids in Myocardial Ischemia
The major energy source of myocardium depends on the production of adenosine triphosphate (ATP) via oxidation of FFAs rather than glucose in aerobic metabolism34; however, ATP production shifts more to glycolysis in the anaerobic state that develops during myocardial ischemia,35 which results in increasing lactate and cytoplasmic calcium leading to the impaired generation of ATP.36, 37 Furthermore, catecholamine stimulation during myocardial ischemia increases the concentration of circulating FFAs as a result of lipolysis with the breakdown of triglycerides into FFAs and glycerol by catecholamine‐sensitive lipase in adipose tissue.38, 39 The ischemic myocardium is exposed to hypoxia due to excessive oxygen consumption during FFA metabolism, which reduces the myocardial uptake of glucose that is required for anaerobic glycolysis and mitochondrial ATP production.40, 41, 42 The excessive uptake of FFAs into myocardium contributes to cardiac function impairment in the ischemic process, which can subsequently increase infarct size and mortality.38, 43
We demonstrated that the circulating FFAs in AIM−/− mice were significantly lower in AIM−/− mice than WT mice after MI, and the release of FFAs was attenuated with an increase of FAS in AIM−/− mice. The reduced concentration of FFAs in AIM−/− mice not only was caused by the reduction of lipolysis, despite the activation of FAS through incorporation of AIM into adipocytes at baseline, but also might have been influenced by the inactivation of catecholamine‐sensitive lipase due to a reduction in catecholamine concentrations. On the other hand, the activation of FAS, together with a decrease in FFAs, was observed in AIM−/− mice. It was reported that the inhibition of FAS activity due to AIM caused lipolysis in adipocytes without the activation of the cAMP/AMPK signaling pathway, hormone‐sensitive lipase, and adipose triglyceride lipase.11 Although it is known that there is an important interaction between AIM and FAS, the FAS activity might be attenuated by negative feedback from the increase in FFAs after MI.
Finally, remodeling is correlated with infarct size and impairs cardiac function after MI, which can lead to heart failure and an increase in mortality during the chronic phase. TLR‐4 plays important roles in inflammation and matrix turnover and contributes to LV healing and remodeling in animal models of MI.24 These findings are consistent with our results of less depression of LV contractility, suppression of interstitial fibrosis, reduced heart weight, and an improved survival rate in AIM−/− mice compared with WT mice. These beneficial effects were due to a reduction of the inflammatory response via the TLR‐4 pathway.
Limitations
The deleterious signals including HSP60, HMGB‐1, and ECM are known to activate the inflammatory response as ligands for TLR‐4. It was possible in this present study that these deleterious signals increased after myocardial injury and produced a much stronger inflammatory response. However, we demonstrated a reduced FFA concentration and inflammatory response after MI, and this subsequently reduced the infarct size in AIM−/− mice. Therefore, these results provide evidence for the proinflammatory effects of AIM, which might be useful for the treatment of MI in the future.
Conclusion
In summary, AIM‐deficient mice demonstrated a smaller infarct size and less of an inflammatory response via inflammatory pathways after MI. These data provide the first evidence that AIM plays an important role in the inflammatory responses after myocardial injury. Therefore, the inhibition of AIM can prevent not only cardiovascular disease in chronic inflammation but also functional impairment after MI.
Sources of Funding
Jun‐ichi Oyama belongs to the endowed Department of Fukuda Denshi Co, Ltd. Koichi Node received remuneration including lecture fees from AstraZeneca, Astellas Pharma Inc, Nippon Boehringer Ingelheim Co, Ltd, Pfizer Inc., MSD K.K., Daiichi Sankyo Company, Ltd, Kowa Pharmaceutical Co, Ltd, Takeda Pharmaceutical Co., Ltd., Novartis Pharmaceuticals Japan, Mitsubishi Tanabe Pharma Corporation, and Dainippon Sumitomo Pharma Co., Ltd. Koichi Node also received scholarship funds or donations granted by Astellas Pharma Inc, Nippon Boehringer Ingelheim Co, Ltd, Daiichi Sankyo Company, Ltd, Takeda Pharmaceutical Co, Ltd, Mitsubishi Tanabe Pharma Corporation, and MSD K.K.
Disclosures
None.
Acknowledgments
We thank Toru Miyazaki, Satoko Arai (Tokyo University), and Masaki Ohmuraya (Kumamoto University) for helpful advice. We also thank Masami Haraguchi, Ruriko Imaizui, and Haruna Nagao for their technical assistance.
Notes
(J Am Heart Assoc. 2016;5:e002863 10.1161/JAHA.115.002863) [Europe PMC free article] [Abstract] [Google Scholar]
References
Articles from Journal of the American Heart Association: Cardiovascular and Cerebrovascular Disease are provided here courtesy of Wiley
Full text links
Read article at publisher's site: https://doi.org/10.1161/jaha.115.002863
Read article for free, from open access legal sources, via Unpaywall:
https://www.ahajournals.org/doi/pdf/10.1161/JAHA.115.002863
Citations & impact
Impact metrics
Citations of article over time
Alternative metrics
Article citations
Administration of an antibody against apoptosis inhibitor of macrophage prevents aortic aneurysm progression in mice.
Sci Rep, 14(1):15878, 10 Jul 2024
Cited by: 0 articles | PMID: 38982113 | PMCID: PMC11233551
The effects of apoptosis inhibitor of macrophage in kidney diseases.
Eur J Med Res, 29(1):21, 04 Jan 2024
Cited by: 0 articles | PMID: 38178221 | PMCID: PMC10765713
Review Free full text in Europe PMC
CD5L aggravates rheumatoid arthritis progression via promoting synovial fibroblasts proliferation and activity.
Clin Exp Immunol, 213(3):317-327, 01 Oct 2023
Cited by: 1 article | PMID: 37191481
Apoptosis inhibitor of macrophage (AIM)/CD5L is involved in the pathogenesis of COPD.
Respir Res, 24(1):201, 17 Aug 2023
Cited by: 1 article | PMID: 37592330 | PMCID: PMC10433671
IL-38 attenuates myocardial ischemia-reperfusion injury by inhibiting macrophage inflammation.
Immun Inflamm Dis, 11(6):e898, 01 Jun 2023
Cited by: 8 articles | PMID: 37382260 | PMCID: PMC10266135
Go to all (25) article citations
Data
Similar Articles
To arrive at the top five similar articles we use a word-weighted algorithm to compare words from the Title and Abstract of each citation.
Apoptosis inhibitor of macrophage depletion decreased M1 macrophage accumulation and the incidence of cardiac rupture after myocardial infarction in mice.
PLoS One, 12(11):e0187894, 09 Nov 2017
Cited by: 19 articles | PMID: 29121663 | PMCID: PMC5679665
Toll-like receptor-2 modulates ventricular remodeling after myocardial infarction.
Circulation, 108(23):2905-2910, 01 Dec 2003
Cited by: 186 articles | PMID: 14656915
Targeted cardiac overexpression of A20 improves left ventricular performance and reduces compensatory hypertrophy after myocardial infarction.
Circulation, 115(14):1885-1894, 26 Mar 2007
Cited by: 77 articles | PMID: 17389268
The comprehensive role of apoptosis inhibitor of macrophage (AIM) in pathological conditions.
Clin Exp Immunol, 212(3):184-198, 01 Jun 2023
Cited by: 5 articles | PMID: 36427004 | PMCID: PMC10243866
Review Free full text in Europe PMC