Abstract
Background
Mutations in Leucine-rich repeat kinase 2 (LRRK2) enhance levels of the autophosphorylated LRRK2 protein and are the most common known cause of inherited Parkinson's disease (PD). LRRK2 has been further implicated in susceptibility to idiopathic PD in genetic association studies.Objective
The objective of this study was to compare autophosphorylated Ser(P)-1292 LRRK2 levels from biobanked urine samples with clinical data in PD patients and controls.Methods
Ser(P)-1292 LRRK2 levels were measured from urine exosome fractions from 79 PD patients and 79 neurologically healthy controls enrolled in the Parkinson Disease Biomarker Program at the University of Alabama at Birmingham.Results
Ser(P)-1292 LRRK2 levels were higher in men than women (P < .0001) and elevated in PD patients when compared with controls (P = .0014). Ser(P)-1292 LRRK2 levels were higher in PD cases with worse cognition and correlated with poor performance in MoCA (r = -0.2679 [-0.4628 to -0.0482]), MDS-UPDRS subscales 1 and 2 (r = 0.2239 [0.0014-0.4252], 0.3404 [0.1276-0.5233], respectively), Epworth Sleepiness Scale (r = 0.3215 [0.1066-0.5077]), and Modified Schwab and England Activities of Daily Living Scales (r = -0.4455 [-0.6078 to -0.2475]). Ser(P)-1292 LRRK2 levels predicted those with worse cognitive impairment in PD patients with some success (c = 0.73).Conclusions
Urinary exosome Ser(P)-1292 LRRK2 levels are elevated in idiopathic PD and correlated with the severity of cognitive impairment and difficultly in accomplishing activities of daily living. These results implicate biochemical changes in LRRK2 in idiopathic PD. © 2016 International Parkinson and Movement Disorder Society.Free full text

Ser(P)-1292 LRRK2 in Urinary Exosomes is Elevated in Idiopathic Parkinson Disease
Abstract
Background
Mutations in Leucine-rich repeat kinase 2 (LRRK2) enhance levels of autophosphorylated LRRK2 protein and are the most common known cause of inherited Parkinson disease (PD). LRRK2 has been further implicated in susceptibility to idiopathic PD in genetic association studies.
Objective
To compare autophosphorylated Ser(P)-1292 LRRK2 levels from biobanked urine samples with clinical data in PD and controls.
Methods
Ser(P)-1292 LRRK2 levels were measured from urine exosome fractions from 79 PD and 79 neurologically healthy controls enrolled into the Parkinson Disease Biomarker Program at the University of Alabama at Birmingham.
Results
Ser(P)-1292 LRRK2 levels were higher in males than females (p<0.0001) and elevated in PD compared to controls (p=0.0014). Ser(P)-1292 LRRK2 levels were higher in PD cases with worse cognition and correlated with poor performance in MoCA (r=−0.2679 [−0.4628, −0.0482]), MDS-UPDRS subscales 1 and 2 (r=0.2239 [0.0014, 0,4252], 0.3404 [0.1276, 0.5233] respectively), Epworth Sleepiness Scale (r=0.3215 [0.1066, 0.5077]), and Modified Schwab and England Activities of Daily Living Scales (r=−0.4455 [−0.6078, −0.2475]). Ser(P)-1292 LRRK2 levels predicted those with worse cognitive impairment in PD patients with some success (c=0.73).
Conclusions
Urinary exosome Ser(P)-1292 LRRK2 levels are elevated in idiopathic PD and correlated with the severity of cognitive impairment and difficultly in accomplishing activities of daily living. These results implicate biochemical changes in LRRK2 in idiopathic PD.
Introduction
Genetic studies identified mutations in the leucine-rich repeat kinase 2 (LRRK2) gene as the most common known cause of autosomal-dominant PD1–5. The LRRK2 gene is also linked to idiopathic PD (i.e., cases without LRRK2 or other known genetic mutations) through genome-wide association studies6. LRRK2 protein includes two enzymatic domains proven to have GTPase and protein kinase activity. The kinase domain that harbors the most common LRRK2 mutation, G2019S, can direct intrinsic phosphorylation (i.e., autophosphorylation) in and nearby the GTPase domain7, 8. Pathogenic LRRK2 mutations including the R1441C, Y1699C, and G2019S mutation increase autophosphorylated LRRK2 protein levels in model systems8–11. One of the most abundant sites of autophosphorylation is the Ser-1292 residue nearby the GTPase domain8. Treatment with LRRK2 kinase inhibitors diminish levels of Ser(P)-1292 LRRK2 in model systems8, 12.
Our previous work demonstrated that LRRK2 protein can be purified from urinary exosomes in biobanked samples13. Recently we discovered that medicated PD patients with the G2019S-LRRK2 mutation show a ~5-fold elevation of autophosphorylated Ser(P)-1292 LRRK2 protein in urinary exosomes compared to non-carrier controls and medicated PD patients without the G0219S-LRRK2 mutation11. LRRK2 autophosphorylation has not been previously evaluated in a larger series of idiopathic PD cases. Here, exosome Ser(P)-1292 LRRK2 phosphorylation is measured in urine from both idiopathic PD cases and controls. Among PD cases, we assess the association between autophosphorylated LRRK2 levels and clinical motor and non-motor disease severity scales.
Methods
Study Subjects
Urine was obtained from study patients (81 diagnosed with PD [41 males] and 81 neurologically healthy controls [40 males]) enrolled at the University of Alabama at Birmingham (UAB) Movement Disorder Clinic. Subjects were selected from the UAB cohort for this study based on frequency matching of age and gender between cases and controls. Inclusion criteria for cases were a diagnosis of PD based on the United Kingdom Parkinson’s Disease Society Brain Bank Criteria. Inclusion criteria for healthy controls were ages of 35–85 years, lack of PD in first-degree blood relatives, and a lack of positive responses on more than three items in the PD Screening Questionnaire15. Exclusion criteria for all patients included atypical features indicative of progressive supranuclear palsy, multiple system atrophy, corticobasal degeneration, cerebellar signs, supranuclear gaze palsy, apraxia, disabling autonomic failure, neuroleptic treatment at time of onset of parkinsonism, active treatment with a neuroleptic at time of study entry, history of repeated strokes with stepwise progression of parkinsonism, history of repeated head injury, history of definite encephalitis, dementia, known severe anemia, kidney disease as determined by measurement of serum creatinine and/or medical records, urinary tract or bladder infection, or a serious comorbidity that would interfere with participation in the study. None of the participants carried a LRRK2 mutation (e.g., G2019S, R1441C/G, Y1699C) as determined with the NeuroX array following enrollment as described16. This study was approved by the UAB Institutional Review Board, and all subjects signed informed consents. All samples were processed by investigators blinded to PD status, and final curated datasets were achieved before associating clinical characteristics to sample values.
Clinical Evaluations
Demographics, PD characteristics, medications, medical history and family history of PD were collected. Levodopa equivalent daily dose was calculated as described17. Subjects were evaluated between 08:00 and 12:00 and PD patients were taking their usual medications. Motor assessment was conducted with the Movement Disorder Society Unified Parkinson’s Disease Rating Scale (MDS-UPDRS). Cognition was screened by the Montreal cognitive assessment (MoCA). Non-motor symptoms and performance were assessed by the Epworth sleepiness scale (Epworth SS), Parkinson’s Disease Questionnaire-39 (PDQ-39), and modified Schwab and England v1.0 Activities of Daily Living (Mod. S&E ADL v1.0) scale. All assessments were conducted by a single rater (R.G.C.) throughout the duration of the study.
Sample Collection and Handling
Samples were collected between January 2013 and December 2014 in a cross-sectional study as part of the Parkinson’s Disease Biomarker Program14 (https://pdbp.ninds.nih.gov). Urine samples were procured during the subject visit along with blood samples. Within two hours of collection, urine was aliquoted into 50 mL polypropylene tubes and stored at −80 C for future processing and exosome isolation.
Exosome Isolation
Exosomes were isolated as previously described11. Briefly, 50 mL polypropylene tubes were quick-thawed at 42 C at 100 RPM in a water bath. Urine was then centrifuged at 10,000 × g for 30 min in Beckman JA-14 rotors and the supernatant then centrifuged at 100,000 × g in Beckman UltraClear tubes for 2 hours in a Beckman SW-32 Ti rotor. The resultant exosome pellet was washed in a saline-buffered solution, resuspended into lysis buffer, and stored at −80 C for further analysis. Supplementary Figure S1 includes a cryo-electron micrograph of these isolated urinary exosomes following saline-buffered solution wash prior to exosomes lysis.
Immunoblotting and Quantitation
Samples were analyzed onto TGX gels (7.5%) SDS-PAGE, and transferred onto PVDF membrane (Millipore Immobilon-FL PVDF). Antibodies were applied to membranes (anti-TSG101 [Abcam poly rabbit], N241(anti-LRRK2) [Antibodies Inc], and MJFR-19 (anti-pS1292-LRRK2) [Abcam]). Membranes probed with TSG101 and N241 were blocked using Odyssey blocking buffer (LiCOR) for 1 hour at room temperature and then incubated overnight in primary antibody diluted in blocking buffer at 4 C on a shaker (1:2500 TSG101, 1:2000 N241, both ~1 μg mL−1 in solution). Membranes were washed 3 × 5 min in 1× tris-buffered tween solution and then incubated overnight with secondary antibodies. LiCOR secondary antibodies were used at 1:20,000 dilutions and consisted of Goat-anti mouse or anti rabbit IRDye 800CW and IRDye 680LT.
Blots were developed using a LiCOR Odyssey Scanner. Signals were recorded with LiCOR Image Studio. An exosomal pellet and a corresponding exosome protein marker (TSG101 protein) were detected in all specimens with the exception of two control subjects and two PD subjects where no protein could be measured for any of the markers. These four subjects were excluded from the study.
Exosome LRRK2 was therefore measured in 79 healthy controls and 79 PD patients (Table 1). Expanded immunoblots utilizing Ser(P)-1292 LRRK2, total LRRK2, and TSG101 antibodies in this study are included in Supplementary Figure S1. Total LRRK2 and Ser(P)-1292 LRRK2 signal are within a range of detection where a linear relationship existed between signal and target concentration (Supplementary Figure S1 C–D). This allows for minimal technical variation with this assay. Representative western blot images used to calculate Ser(P)-1292 LRRK2, total LRRK2, and TSG101 levels in the cohort study are presented in Supplementary Figure S2. No correlation was identified between the time in the freezer for the urine and observed values for any of the exosome proteins including Ser(P)-1292 LRRK2 levels (r = −0.0473 [−0.2035, 0.1111]).
Table 1
Demographics and clinical characterization of PD and control subjects
Descriptive Title | Control (n = 79)* | PD (n = 79)* | pa |
---|---|---|---|
Gender, Male (Female) | 40 (39) | 41 (38) | n.s.b |
Age (years), Median (Range) | 64 (36–77) | 65 (37–79) | n.s.c |
Caucasian, Number (%) | 76 (96.2) | 77 (97.5) | n.s.b |
Creatinine (mg dL−1), Mean (S.D.) | 0.96 (0.234) | 0.93 (0.179) | 0.333d |
Weight (kg), Mean (S.D.) | 82.7 (22.29) | 80.0 (17.86) | 0.407d |
Height (cm), Median (Range) | 170 (155–193) | 173 (150–193) | n.s.c |
Age at Diagnosis, Median (Range) | n/a | 57 (28–76) | n/a |
MDS-UPDRS, Median (Range) | |||
![]() | 4 (0–16) | 12 (1–28) | <0.0001c |
![]() | 0 (0–13) | 12 (1–33) | <0.0001c |
![]() | 0 (0–31) | 25 (2–52) | <0.0001c |
![]() | 0 (0–0) | 0 (0–12) | <0.0001c |
Modified S&E v1.0, Median (Range) | 100 (80–100) | 90 (40–100) | <0.0001c |
MoCA, Median (Range) | 26 (17–30) | 25 (12–30) | 0.0004c |
Education (%), High school, College, Masters, PhD | 12.7, 62.0, 20.3, 5.1 | 21.5, 60.8, 8.9, 8.9 | 0.1039e |
Dementia Family History [1°,2°], % | 26.6 | 22.8 | n.s.b |
PDQ-39, Median (Range) | |||
![]() | 0 (0–32.5) | 20 (0–92.5) | <0.0001c |
![]() | 0 (0–50) | 25 (0–79.2) | <0.0001c |
![]() | 8.3 (0–41.7) | 16.7 (0–83.3) | <0.0001c |
![]() | 0 (0–18.75) | 12.5 (0–100) | <0.0001c |
![]() | 0 (0–33.3) | 0 (0–75) | 0.0063c |
![]() | 6.25 (0–50) | 25 (0–81.3) | <0.0001c |
![]() | 0 (0–41.7) | 16.7 (0–100) | <0.0001c |
![]() | 8.3 (0–83.3) | 25 (0–91.7) | <0.0001c |
![]() | 4.4 (0–30.1) | 19.8 (0.3–76.9) | <0.0001c |
Epworth SS, Median (Range) | 6 (0–16) | 10 (0–24) | <0.0001c |
LEDD, Median (Range) | n/a | 700 (0–2500) | n/a |
PD = Parkinson disease; UPDRS = Unified Parkinson’s Disease Rating Scale; Modified S&E v1.0 = Modified Schwab and England v1.0 Activities of Daily Living Scale; MoCA = The Montreal Cognitive Assessment; PDQ-39 = Parkinson’s Disease Questionnaire-39; Epworth SS = Epworth Sleepiness Scale; LEDD = L-Dopa Equivalent Daily Dosage
Statistical Analysis
For continuous variables we compared controls and PD patients using Student’s t-test or Mann-Whitney U test when assumptions of a t-test may not be met. Fisher’s exact test was used to test the association between disease status and categorical variables. For assessing correlations, Pearson product-moment coefficients were calculated. As clinical measures of disease severity are highly correlated we report exact p-values as recommended by the Uniform Requirements for Manuscripts Submitted to Biomedical Journals18. In addition, we also report adjusted p values to account for potential false discovery using the Benjamini and Hochberg method19. Ser(P)-1292 LRRK2 levels were log10-transformed to better meet model assumptions and were included in an ANCOVA model adjusted for gender and total LRRK2 levels as model covariates (see Supplemental Table S2 for details on the gender relationship). PD cases alone, controls alone, and PD and controls combined, were stratified into quartiles based on Ser(P)-1292 LRRK2 levels and differences in clinical measures of disease severity between quartiles were compared with Mann-Whitney U test. All statistical analyses and graphs were generated in JMP Pro 10 (SAS Institute Inc., Cary, NC, USA) or GraphPad Prism 5.0 (GraphPad Software, San Diego, CA, USA). Significance was ascribed to p-value < 0.05, and all significance levels presented are two-tailed.
Results
Characteristics of study participants where exosome proteins could be measured (79 cases and 79 controls) are summarized in Table 1. Demographic characteristics were similar between cases and controls. MoCA scores as low as 17 (with a median of 26) were recorded in the healthy control population. In this cohort, no significant differences were observed in frequencies of alcohol consumption, smoking, benzodiazepine use, antidepressant use, non-steroidal anti-inflammatory use, or vitamin and supplement use, between cases and controls. In evaluation of exosome preparations from both cases and controls, TSG101 levels were similar in concentration (Mann Whitney U test, p = 0.2472), and in both males and females (Mann Whitney U test, p = 0.3048). These results indicate that exosome concentrations were similar between groups. In several control subjects, we evaluated Ser(P)-1292 LRRK2 levels in urinary exosomes over the course of several weeks as well as in afternoon versus morning collections of urine, and we found good intra-individual stability for Ser(P)-1292 LRRK2 levels in these subjects (Supplementary Figure S1 E–F).
Unadjusted Ser(P)-1292 LRRK2 levels in urinary exosomes were elevated in PD versus controls in the complete cohort (Figure 1, p = 0.0014). Gender affected Ser(P)-1292 LRRK2 levels (~3-fold higher median levels in males versus females, Supplementary Table S2). Ser(P)-1292 LRRK2 levels were elevated in PD females compared to female controls (~7.5-fold higher median values in PD, p = 0.0028, Mann-Whitney U). In contrast, there was a less-significant trend toward elevated Ser(P)-1292 LRRK2 levels in male PD cases compared to male controls (~1.5-fold higher median values in PD, p = 0.0822, Mann-Whitney U). We found no correlation between Ser(P)-1292 LRRK2 levels and patient age (r = 0.0473, p = 0.5587) or disease duration among those with PD (r = −0.0172, p = 0.8813). Levels of total LRRK2 protein positively correlated with Ser(P)-1292 LRRK2 levels (r = 0.44, p < 0.0001). When adjusting for the effects of gender and total LRRK2 levels, Ser(P)-1292 LRRK2 levels remained significantly elevated in the entire PD cohort compared to the entire control cohort (p = 0.0286).
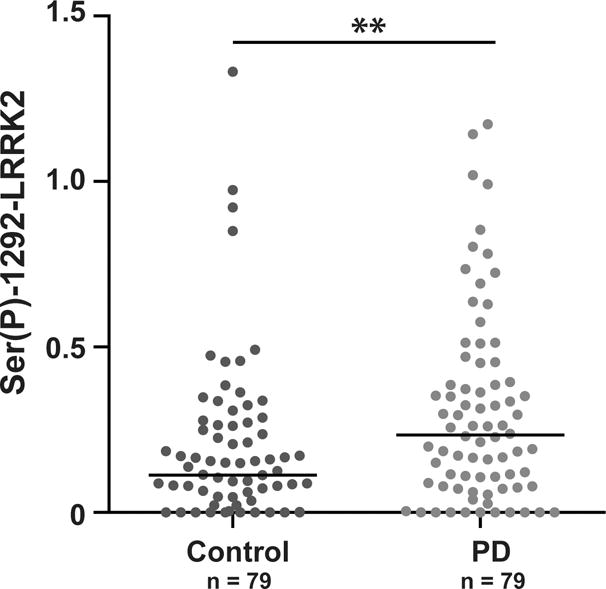
Urinary exosomes were isolated from 79 healthy and 79 PD patients and quantities of Ser(P)-1292 LRRK2 were determined relative to an exosome reference pool (defined as 1.0). Unadjusted values for Ser(P)-1292-LRRK2 are shown, ** p < 0.01, Mann-Whitney U-test.
The Ser(P)-1292 LRRK2 to total LRRK2 ratio in urinary exosomes were calculated and also elevated in PD versus controls (~1.5-fold higher median values in PD, p = 0.0074). Gender affected the ratio value as well (~1.6-fold higher median levels in males versus females). The ratio of phospho- to total LRRK2 was also elevated in PD females compared to female controls (~2.9-fold higher median values in PD, p = 0.008, Mann-Whitney U). Similar to raw Ser(P)-1292 LRRK2 levels, there was an elevated ratio in male PD cases compared to male controls but this was not significant (~1.6-fold higher median values in PD, p = 0.1907, Mann-Whitney U).
Table 2 summarizes correlations between Ser(P)-1292 LRRK2 levels and clinical measures of PD. Non-motor aspects of PD captured with MDS-UPDRS part II, and Epworth SS were significantly and positively correlated with Ser(P)-1292 LRRK2 levels after adjustment for false discovery (Table 2). Significant associations between Ser(P)-1292 LRRK2 levels and Modified S&E v1.0 were also identified. There was no association between Ser(P)-1292 LRRK2 levels and levodopa equivalent daily dose (r = 0.1512, p = 0.3933).
Table 2
Correlations between Ser(P)-1292 LRRK2 levels and clinical features in PD cases
Clinical Measure | Correlation Coefficient | 95% CI | p* | p** |
---|---|---|---|---|
Motor Assessments | ||||
MDS-UPDRS | ||||
![]() | 0.0918 | [−0.1334, 0.3081] | 0.4240 | 0.4543 |
Non-Motor Assessments | ||||
MDS-UPDRS | ||||
![]() | 0.2239 | [0.0014, 0.4252] | 0.0488 | 0.0813 |
![]() | 0.3404 | [0.1276, 0.5233] | 0.0023 | 0.0173 |
MoCA | −0.2679 | [−0.4628, −0.0482] | 0.0177 | 0.0664 |
Epworth SS | 0.3215 | [0.1066, 0.5077] | 0.0041 | 0.0205 |
Global Assessments | ||||
Modified S&E v1.0 | −0.4455 | [−0.6078, −0.2475] | <0.0001 | <0.0015 |
PDQ-39 | ||||
![]() | 0.2457 | [0.0245, 0.4440] | 0.0301 | 0.0813 |
![]() | 0.2242 | [0.0018, 0.4255] | 0.0484 | 0.0813 |
![]() | 0.2343 | [0.0124, 0.4342] | 0.0390 | 0.0813 |
![]() | 0.1171 | [−0.1082, 0.3310] | 0.3071 | 0.3768 |
![]() | 0.2044 | [−0.0190, 0.4083] | 0.0727 | 0.1091 |
![]() | 0.1827 | [−0.0415, 0.3894] | 0.1094 | 0.1492 |
![]() | 0.1125 | [−0.1128, 0.3269] | 0.3266 | 0.3768 |
![]() | 0.0728 | [−0.1522, 0.2906] | 0.5266 | 0.5266 |
![]() | 0.2250 | [0.0026, 0.4262] | 0.0477 | 0.0813 |
PD = Parkinson disease; UPDRS = Unified Parkinson’s Disease Rating Scale; Modified S&E v1.0 = Modified Schwab and England v1.0 Activities of Daily Living Scale; MoCA = The Montreal Cognitive Assessment; PDQ-39 = Parkinson’s Disease Questionnaire-39; Epworth SS = Epworth Sleepiness Scale; LEDD = L-Dopa Equivalent Daily Dosage
Patients with PD in the upper quartile of Ser(P)-1292 LRRK2 levels had worse performance in the Modified S&E v1.0 and Epworth SS scores than those in the lower quartile Ser(P)-1292 LRRK2 group, as well as a trending difference in MoCA scores (Table 3). However, worse performance in the MoCA was also detected in control subjects that had the highest levels of Ser(P)-1292 LRRK2 (Table 3).
Table 3
Selected clinical features stratified by Ser(P)-1292 LRRK2 levels
Descriptive Title | PD
| Controls
| Combined PD & Controls
| ||||||
---|---|---|---|---|---|---|---|---|---|
Low-1292 (n= 20) | High-1292 (n = 19) | p* | Low1292 (n = 19) | High-1292 (n = 19) | p* | Low-1292 (n = 39) | High-1292 (n = 39) | p* | |
MoCA | 25.5 (16–30) | 24 (17–29) | 0.0583 | 27 (20–30) | 25 (20–29) | 0.0459 | 27 (18–30) | 25 (17–29) | 0.0001 |
S&E v1.0 | 90 (60–100) | 80 (40–90) | 0.0143 | 100 (90–100) | 100 (90–100) | n.s. | 100 (60–100) | 90 (40–100) | 0.0004 |
Epworth SS | 9.5 (0–17) | 11 (5–22) | 0.0434 | 7 (0–13) | 4 (0–12) | n.s. | 7 (0–17) | 9 (3–22) | 0.0054 |
Low-1292 and High-1292 represent subjects within the lower or higher quadrant of Ser(P)-1292 LRRK2 levels for that group.
Summary statistics represent median (range). MoCA = The Montreal Cognitive Assessment; Modified S&E v1.0 = Modified Schwab and England v1.0 Activities of Daily Living Scale; Epworth SS = Epworth Sleepiness Scale
Among PD patients, Ser(P)-1292 LRRK2 levels predicted poor MoCA and S&E scores with some success (Figure 2). Ser(P)-1292 LRRK2 levels predicted the lower quartile of poor MoCA performance with a ROC area under the curve c-statistic of 0.731 (Figure 2B). Ser(P)-1292 LRRK2 levels were likewise elevated in PD patients with lower S&E scores (p = 0.0061, Figure 2C). Ser(P)-1292 LRRK2 levels predicted the lower quartile of poorer S&E scores with a ROC area under the curve of 0.788 (Figure 2D). However, performance on MoCA did not predict S&E scores in our cohort (r = 0.0737 [−0.1514, 0.2914], p = 0.5216). Only half of the PD cases in the lower quartile of S&E scores were also in the lower quartile of MoCA scores. Thus Ser(P)-1292 LRRK2 levels appear to predict several aspects of PD disease severity.
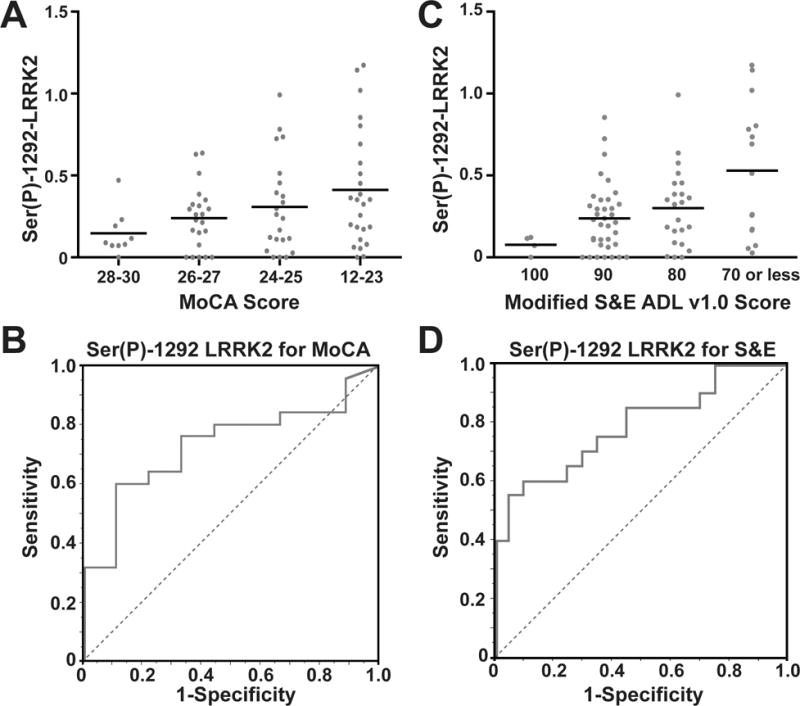
(A) Exosomal Ser(P)-1292 LRRK2 levels progressively increase with declining MoCA score in PD cases. Levels are significantly different across quartiles (p = 0.05, Kruskal-Wallis) (B) Receiver Operating Characteristic (ROC) Curve for Ser(P)-1292 LRRK2 prediction of the lower quartile of MoCA performance. Risk prediction is success is 0.731 with a sensitivity of 60% and specificity of 89% at the optimum threshold. (C) Ser(P)-1292 LRRK2 levels increase with declining S&E scores. Levels are significantly different across quartiles (p = 0.0061, Kruskal-Wallis). (D) ROC Curve analysis of Ser(P)-1292 LRRK2 levels distinguishing lower S&E quartile. Area under the ROC curve is 0.788 with a sensitivity of 60% and specificity of 90% at optimum threshold.
Discussion
This is the first study assessing Ser(P)-1292 LRRK2 levels in a large group of idiopathic PD cases and controls. To evaluate Ser(P)-1292 LRRK2 protein in idiopathic PD patients, we prioritized the collection of urine as a non-invasively derived biofluid in a typical medicated PD population. In a cross-sectional cohort of idiopathic PD patients enrolled in the Parkinson’s Disease Biomarker Program at the University of Alabama at Birmingham, we found exosome Ser(P)-1292 LRRK2 levels were significantly elevated in PD and evidence of correlation with multiple non-motor measures of PD including MoCA, MDS-UPDRS Part I and II, and Epworth SS, as well as global measures of successful daily living (Mod. S&E v1.0 and PDQ-39).
We did not detect significant associations between Ser(P)-1292 LRRK2 and motor impairment despite demonstration in larger studies that motor impairment scores do worsen over time in PD patients. In our cohort, patients were on medication at the time of clinical evaluation and sample donation, and our power to separate mild from moderate motor impairment was efficiently masked by dopaminergic therapies. For example, in this cohort of 79 PD cases, we had a poor correlation of MDS-UPDRS III scores and disease duration (r=−0.0216, p = 0.8521). Ser(P)-1292 LRRK2 levels may not successfully predict motor impairment in PD, or Ser(P)-1292 LRRK2 may be useful to predict motor impairment in non-medicated PD cases. Future studies are required to test these hypotheses.
Given a possible correlation between Ser(P)-1292 LRRK2 and MoCA performance, it would be of interest to determine if Ser(P)-1292 LRRK2 levels correlate with measures of symptom severity in patients diagnosed with Diffuse Lewy Body disease. Levels of exosome Ser(P)-1292 LRRK2 may generally predict cognitive impairment associated with α-synuclein. Or, Ser(P)-1292 LRRK2 levels may not be specific for predicting cognitive decline in α-synuclein linked disease. Specificity for Ser(P)-1292 LRRK2 as a biomarker for cognitive impairment in PD versus other causes of impairment like Alzheimer disease can be determined in future studies.
We found no evidence of association between LEDD levels and Ser(P)-1292 LRRK2 levels (or any other reported medication) that would alter the relationship between Ser(P)-1292 LRRK2 and non-motor measures of PD severity. Recent observations in G2019S-LRRK2 mutation carriers demonstrated that Ser(P)-1292 LRRK2 was relatively insensitive to medication because levels of Ser(P)-1292 were not different between medicated groups and non-medicated groups compared to G2019S-LRRK2 mutation carriers11. However, future studies to evaluate Ser(P)-1292 LRRK2 in de novo groups of PD patients that are not yet medicated as well as longitudinal series would be beneficial to better understand the relationship of Ser(P)-1292 LRRK2 and measures of disease progression and severity.
LRRK2 has been genetically linked to neurodegenerative disease, and we have recently demonstrated that the G2019S-LRRK2 mutation elevates urinary exosome Ser(P)-1292 LRRK2 levels ~5-fold above controls11. Here, we have identified some PD cases, as well as a few controls, that show comparable levels of Ser(P)-1292 LRRK2 as G2019S-LRRK2 mutation carriers with PD. Overall idiopathic PD with the highest levels of Ser(P)-1292 LRRK2 also demonstrated worse performance on clinical assessments of daily living impairment and cognitive performance.
The biological source of urinary Ser(P)-1292 LRRK2 in our exosome preparations has not been determined which is a limitation to interpretation of our results. LRRK2 is highly expressed in cells of the innate immune system and it has recently been demonstrated that inflammatory stimuli such as lipopolysaccharide can induce increased levels of LRRK2 autophosphorylation and exosomal release20. A possible explanation for increased Ser(P)-1292 LRRK2 levels in PD patients with poorer scores on assessments of cognitive performance and activities of daily living may be increased inflammation in the patients with aggressive progression of disease. Elevations in several cytokines have been observed in the CSF of PD patients as well as increased plasma C-reactive protein levels with correlations to PD severity21–24. Multivariate modeling for most of these inflammation markers also observed significant gender effects, potentially in line with observations from this study.
In PD, there are currently no validated biochemical biomarkers for independently assessing PD diagnosis or prognosis, or measuring disease severity25. In Alzheimer disease (AD), CSF levels of Aβ42, in concert with total tau and phosphorylated tau levels, may have value in identifying prodromal AD26. However, levels of CSF Aβ42 and tau stabilize when a patient has clinically manifested AD and thus fail to inform on disease progression. In PD, CSF levels of Aβ42, total tau, phospho-tau, and α-synuclein have been proposed to have prognostic and diagnostic value in early-stage PD27. However, each study reported major limitations for practical diagnostic or prognostic utility of α-synuclein, tau, or phospho-tau in PD28–32. Here, we observed that Ser(P)-1292 LRRK2 levels associated with several features of PD. As few viable biomarkers for PD exist, particularly for non-motor features33, Ser(P)-1292 LRRK2 may be a promising candidate for further exploration.
Acknowledgments
Urine samples were collected under funding via grant NIH U18 NS082132 as a component of the NINDS Parkinson’s Disease Biomarkers Program (PDBP) and banked by ABW and analyzed by KBF and ABR.
Funding for the study was provided by NIH U18 NS082132 and R01 NS064934 and T32GM008111.
We are grateful to Dr. Terje Dokland and Cynthia M. Rodenberg for assistance with the electron microscopy, which was carried out at the UAB Cryo-EM facility, Center for Structural Biology, University of Alabama at Birmingham.
All named authors had full access to all of the data in the study and take responsibility for the integrity of the data and the accuracy of the data analysis. KBF, RNA, DGS, and ABW contributed to experimental design and analysis of the data. KBF and NL performed statistical analysis. KBF and ABW wrote the manuscript. KBF performed the experiments. RGC was the study coordinator and ABR assisted in collecting biosamples and data curation.
Financial Disclosures
Dr. West has served as a consultant for or received honoraria from the Michael J. Fox Foundation for Parkinson Research, Pfizer Inc., Sanofi Inc., the Van Andel Institute, the National Institutes of Health, Ottawa University, Emory University, and Southern Research, in the last three years. Dr. West is an Investigator in studies funded by NIH U18 NS082132 R01 NS064934 P20 NS092530, by Pfizer Inc. to study LRRK2 kinase inhibitors owned by Pfizer and unrelated to this study, and by the Michael J. Fox Foundation for Parkinson Research, American Parkinson’s Disease Association, and the Alabama Drug Discovery Alliance.
Dr. Standaert is a member of the faculty of the University of Alabama at Birmingham and is supported by endowment and University funds. Dr. Standaert is an investigator in studies funded by Abbvie, the American Parkinson Disease Association, the Michael J. Fox Foundation for Parkinson Research, The Bachmann-Strauss Dytonia and Parkinson Foundation, the Dystonia Medical Research Foundation, Acerta Pharma, the MGH Collaborative Center for XDP, Ceregene, Inc., Quintiles, Inc., Alabama Department of Commerce, and NIH grants P01NS087997, P20NS087997, R25NS079188, R01NS064934, U18NS082132. He has a clinical practice and is compensated for these activities through the University of Alabama Health Services Foundation. In addition, in the last three years he has served as a consultant for or received honoraria from Auspex Inc., Teva Neurosciences, Serina Therapeutics, AstraZenica, Abbvie, the Michael J. Fox Foundation for Parkinson Research, Washington University, The Van Andel Institute, the National Institutes of Health, Boston University, The American Institute for Biological Sciences, Johns Hopkins University, the American Academy of Neurology, the Bachmann-Strauss Foundation, Banner Sun Health, Viropharma (now Shire), The Movement Disorder Society, the Kirchner Group, Georgia Regents University and he has received royalties for publications from McGraw Hill, Inc.
Dr. Alcalay has received consultation fees from Sanofi Inc. and Prophase Inc. unrelated to this study.
This study had no contributions from industry sponsors. Unrelated to this study, ABW received a research grant from Pfizer Inc, and RNA received consultation fees from Sanofi, Inc. and Prophase, Inc.
References
Full text links
Read article at publisher's site: https://doi.org/10.1002/mds.26686
Read article for free, from open access legal sources, via Unpaywall:
https://europepmc.org/articles/pmc5053851?pdf=render
Citations & impact
Impact metrics
Citations of article over time
Alternative metrics
Smart citations by scite.ai
Explore citation contexts and check if this article has been
supported or disputed.
https://scite.ai/reports/10.1002/mds.26686
Article citations
The potential of exosomal biomarkers: Revolutionizing Parkinson's disease: How do they influence pathogenesis, diagnosis, and therapeutic strategies?
AIMS Neurosci, 11(3):374-397, 23 Sep 2024
Cited by: 0 articles | PMID: 39431275 | PMCID: PMC11486621
Review Free full text in Europe PMC
Emerging Role of Extracellular Vesicles as Biomarkers in Neurodegenerative Diseases and Their Clinical and Therapeutic Potential in Central Nervous System Pathologies.
Int J Mol Sci, 25(18):10068, 19 Sep 2024
Cited by: 0 articles | PMID: 39337560 | PMCID: PMC11432603
Review Free full text in Europe PMC
Central nervous system-derived extracellular vesicles: the next generation of neural circulating biomarkers?
Transl Neurodegener, 13(1):32, 19 Jun 2024
Cited by: 1 article | PMID: 38898538 | PMCID: PMC11186231
Review Free full text in Europe PMC
Extracellular Vesicles: The Next Generation of Biomarkers and Treatment for Central Nervous System Diseases.
Int J Mol Sci, 25(13):7371, 05 Jul 2024
Cited by: 3 articles | PMID: 39000479
Review
Single molecule array measures of LRRK2 kinase activity in serum link Parkinson's disease severity to peripheral inflammation.
Mol Neurodegener, 19(1):47, 11 Jun 2024
Cited by: 2 articles | PMID: 38862989 | PMCID: PMC11167795
Go to all (104) article citations
Data
Data behind the article
This data has been text mined from the article, or deposited into data resources.
BioStudies: supplemental material and supporting data
Similar Articles
To arrive at the top five similar articles we use a word-weighted algorithm to compare words from the Title and Abstract of each citation.
Elevated LRRK2 autophosphorylation in brain-derived and peripheral exosomes in LRRK2 mutation carriers.
Acta Neuropathol Commun, 5(1):86, 22 Nov 2017
Cited by: 48 articles | PMID: 29166931 | PMCID: PMC5700679
Motor and nonmotor heterogeneity of LRRK2-related and idiopathic Parkinson's disease.
Mov Disord, 31(8):1192-1202, 19 Apr 2016
Cited by: 70 articles | PMID: 27091104
Serum Uric Acid in LRRK2 Related Parkinson's Disease: Longitudinal Data from the PPMI Study.
J Parkinsons Dis, 11(2):633-640, 01 Jan 2021
Cited by: 3 articles | PMID: 33682725
LRRK2 detection in human biofluids: potential use as a Parkinson's disease biomarker?
Biochem Soc Trans, 45(1):207-212, 01 Feb 2017
Cited by: 10 articles | PMID: 28202674
Review
Funding
Funders who supported this work.
NIGMS NIH HHS (1)
Grant ID: T32 GM008111
NIH (1)
Grant ID: U18 NS082132
NINDS NIH HHS (5)
Grant ID: U18 NS082132
Grant ID: P20 NS092530
Grant ID: R25 NS079188
Grant ID: R01 NS064934
Grant ID: P01 NS087997
National Institutes of Health (1)
Grant ID: U18 NS082132, R01 NS064934, and T32GM008111