Abstract
Free full text

Replication of Human Noroviruses in Stem Cell-Derived Human Enteroids
Abstract
The major barrier to research and development of effective interventions for human noroviruses (HuNoVs) has been the lack of a robust and reproducible in vitro cultivation system. HuNoVs are the leading cause of gastroenteritis worldwide. We report successful cultivation of multiple HuNoV strains in enterocytes in stem cell-derived, nontransformed human intestinal enteroid monolayer cultures. Bile, a critical factor of the intestinal milieu, is required for strain-dependent HuNoV replication. Lack of appropriate histoblood group antigen expression in intestinal cells restricts virus replication, and infectivity is abrogated by inactivation (e.g., irradiation, heating) and serum neutralization. This culture system recapitulates the human intestinal epithelium, permits human host-pathogen studies of previously noncultivatable pathogens, and allows the assessment of methods to prevent and treat HuNoV infections.
Introduction
Human noroviruses (HuNoVs) are the most common cause of epidemic and sporadic cases of acute gastroenteritis worldwide, and the leading cause of food-borne gastroenteritis (1–3). Following the introduction of rotavirus vaccines, HuNoVs have become the predominant gastrointestinal pathogen within pediatric populations in developed countries (4). HuNoVs are highly contagious, with rapid person-to-person transmission directly through the fecal–oral route and indirectly from contact with contaminated fomites, or consumption of contaminated food or water. In addition to causing morbidity and mortality in young children, immunocompromised patients, and the elderly, norovirus disease causes significant economic burden as a result of health care costs and loss of productivity (5, 6). HuNoVs have resisted significant efforts to establish in vitro cultivation methods for over 40 years. Previous reports of possible cultivation systems have not been reproduced or support limited replication of a single strain (7–10). Insight into the pathophysiology of HuNoV infections has been elucidated primarily from studies using healthy adult volunteers. The lack of a reproducible culture system for HuNoVs has remained the major barrier to achieving a full mechanistic understanding of their replication, stability, antigenic complexity and evolution. An in vitro cultivation system is critical to define HuNoV-host interactions that underlie the high virus infectivity and explosive illness they cause, to determine how to prevent transmission, and to treat infections and illness.
Ex vivo human intestinal enteroid cultures support HuNoV replication
Attempts to culture HuNoVs in transformed intestinal epithelial cells and in primary human immune cells have been unsuccessful (8, 11, 12). We hypothesized that a novel culture system pioneered by the Clevers group in the Netherlands that generates human intestinal enteroids (HIEs) from stem cells isolated from intestinal crypts in human intestinal tissues (13, 14) and recapitulates the natural intestinal epithelium should support HuNoV growth. These multicellular, differentiated HIEs are nontransformed, physiologically active cultures that respond to agonists and contain multiple intestinal epithelial cell types (enterocytes, goblet, enteroendocrine, and Paneth cells) whether grown as three dimensional or monolayer cultures (Fig. S1) (13–16). To evaluate whether these novel cultures support replication of the previously noncultivatable HuNoVs, monolayers of HIEs were inoculated with GII.4 HuNoVs, which cause the majority of pandemic and outbreak infections worldwide (1). Jejunal monolayer cultures were readily infected by stool filtrates of multiple GII.4 variants (2006a, 2006b-1–3, 2009, and 2012-1, -2; Table S1). At 96 hours post-infection (hpi), 1.5–2.5 log10 increases in genome equivalents of viral progeny were identified by RT-qPCR in comparison to the amount of genomic RNA detected at 1 hpi after removal of the virus inoculum and two washes of the monolayers to remove unattached virus (Fig. 1A, fold changes indicated above the bars for each variant). All inocula used to infect enteroid cultures were fecal filtrates, suggesting that bacteria were not required as co-factors for infection in contrast to previous reports of HuNoV cultivation in BJAB and Raji B cell lines (9, 10). Lipopolysaccharide (LPS) in stool filtrates did not promote replication, as there was no reduction in HuNoV replication in samples treated with polymyxin B that reduced LPS levels from 4.84 to 0.63 endotoxin units (12) (Fig. S2A).

Replication of GII.4 variants in human intestinal enteroids. Jejunal HIE monolayers were inoculated with (A) 9×105 genome equivalents of the indicated HuNoV GII.4 stool filtrates. RNA was extracted from cells and medium, and viral genome equivalents quantified by RT-qPCR. RNA at 1 hpi was collected after removal of virus inoculum and washing of cells twice to remove any unattached viruses. Each data bar represents the mean of three wells of inoculated HIEs. Error bars denote standard deviation. Each experiment was performed two or more times, with three technical replicates in each experiment. Panels (B-E) and (F) represent monolayers inoculated with 9×107 and 9×105 genome equivalents of GII.4/2012-1, respectively. (B) Expression of VP1 was detected in enterocytes (villin, red) in formalin-fixed, paraffin-embedded enteroid monolayer sections using antibody against GII.4/2012 VLPs (green). DAPI detects nuclei (blue). Scale bar = 25 µm. (C) Flow cytometry quantitation and immunofluorescent detection of infected cells. Scale bar = 100 µm. (D) Electron micrograph of HuNoV particles from the supernatant of infected HIEs. Scale bar = 50 nm. Inset: small particle. Scale bar = 25 nm. (E) Western blot detecting polyprotein processing and VP1 expression. Asterisk marks a non-specific band. (F) Kinetics of HuNoV yield at the indicated time points. (G) Passaging of GII.4/2009 HuNoV in jejunal HIEs. (F, G) Viral genome equivalents quantified by RT-qPCR as indicated for panel A.
We next evaluated the growth characteristics of HuNoV infection by assessing cytopathic effect (CPE), antigen detection, and the kinetics of infection. Cytopathic changes such as cell rounding, destruction of the monolayer and an increase in number of dead cells as assessed by trypan blue staining were observed in GII.4-inoculated cultures. CPE was observed for GII.4 variants tested (2012-1, -2 and 2006b-3, see Table S1 for strain details; GII.4/2012-1 results shown in Fig. S2B, left panel). CPE was not reduced by inoculation with polymyxin B-treated samples (Fig. S2B), and CPE was not observed in cultures inoculated with gamma-irradiated stool filtrate (Fig. S2B, right panels), which abrogated viral replication. Viral replication was demonstrated by detecting the major viral capsid protein (VP1), with nonstructural proteins, [RNA-dependent RNA polymerase (Pol) and NTPase], or double-stranded RNA (dsRNA, an intermediate in HuNoV RNA replication) in infected cells by confocal microscopy (Fig. 1B and Fig. S3A–C). Immunofluorescent analysis for VP1 revealed 35–45% of cells in the HIE monolayer were infected, which was confirmed by flow cytometry where 41% of cells were VP1-positive (Fig. 1C). HIE cultures contain multiple cell types (stem cells, Paneth cells, goblet, enteroendocrine and enterocyte cells) and only the enterocytes were infected. Detection of villin, an enterocyte marker, in VP1-positive cells showed that HuNoVs infected and replicated in enterocytes (Fig. 1B). Productive infection was confirmed by transmission electron microscopic visualization of virus particles with typical morphology (17) in the supernatant of infected HIEs (Fig. 1D). Two particle sizes were detected (Fig. 1D), with particles of the expected size (31.6 +/− 3.3 nm) and some smaller particles (Fig. 1D inset, 18.5 +/− 3.7 nm). Both particle sizes have been observed previously in stools of children infected with HuNoVs and in preparations of recombinant virus-like particles (VLPs) (18). By Western blot analysis, nonstructural polyprotein synthesis and processing (as evidenced by the detection of several VPg-containing polyprotein processing intermediates) and capsid protein (VP1) production were first detected at 12 hpi in infected cells but not at 1 or 6 hpi or in mock-infected cells (Fig. 1E). Consistent with the production of virus particles (Fig. 1D), VP1 was detected in the culture supernatant by 24 hpi (Fig. 1E). Replication was confirmed by the growth kinetics of GII.4/2012-1 in jejunal HIE monolayer cultures, which showed a time-dependent increase in genome equivalents between 1 and 24 hpi after which a plateau was reached (Fig. 1F). Because polyprotein processing, RNA replication, and synthesis of subgenomic RNA are required for VP1 and particle production (19), these findings demonstrate that an entire HuNoV replication cycle occurs in infected HIEs by 24 hpi. GII.4/2009 and GII.4/2012-1 HuNoV could also be passaged in jejunal HIEs with optimized conditions (Fig. 1G, GII.4/2009 shown, see below for conditions). Cells expressing VP1 and VPg were observed during infection with passaged virus and particles of both sizes (Fig. S4B) were seen. Together, these results indicate that GII.4 HuNoV-infection of HIEs results in a productive and complete virus replication cycle and this system can be used to define cellular processes HuNoVs co-opt to replicate and induce pathogenesis.
Replication of some HuNoV strains requires the presence of bile
Noroviruses are genetically diverse. Most HuNoVs are classified into two genogroups (GI and GII), which are further subdivided into 9 GI genotypes and 20 GII genotypes. We next tested whether monolayer cultures of jejunal HIEs could support replication of other HuNoV strains (GI.1, GII.3, GII.17). Initially, no replication of these viruses was observed. Consequently, several components of the intestinal milieu were assessed for their ability to promote replication of these HuNoVs in HIE monolayers. Addition of proteases (trypsin, pancreatin) required for the replication of another gastrointestinal virus, human rotavirus, failed to enhance HuNoV replication. In contrast, viral replication, as demonstrated by RT-qPCR and immunofluorescence analyses, was observed in HIEs pretreated with nontoxic levels of human bile and inoculated with stool filtrates of GII.3, GII.17 and GI.1 HuNoVs (Fig. 2A and B, and Fig. S5). Replication occurred in a bile dose-dependent manner, with concentrations of human bile greater than or equal to 0.5% being required for GII.3 replication (Fig. 2A). Bile from different sources such as human, sow and commercially available bovine and porcine, all promoted GII.3 replication without CPE (Fig. S6). Assessment of the kinetics of GII.3 infection demonstrated that, similar to GII.4 strains, virus yields increased between 1 and 24 hpi (Fig. 2C). The addition of bile to GII.4 HuNoV-inoculated cultures was not required, but it enhanced virus replication (Fig. S7). Enhancement was observed when human (Fig. S7A), piglet (Fig. S7B), porcine (Fig. S7C) and sow (Fig. S7D) bile were evaluated. These results indicate there are strain-specific differences in the requirement for factors in the intestinal milieu such as bile to support or enhance HuNoV replication. Of note, even with the addition of bile, the increases in yields for GII.3 as well as for GII.17 and GI.1 virus strains were lower than that observed for the GII.4 variants. In 12 independent experiments performed in triplicate on jejunal HIEs, the mean fold increase of GII.3 genome equivalents ranged from 10–173 fold with an average of a 48 fold increase as compared to 34–6730 fold increases (average 670 fold) for GII.4 HuNoVs. Evaluation of additional intestinal components or further optimization of conditions may be required to achieve higher levels of replication for GII.3 and other HuNoVs. To date, we have successfully obtained replication of two HuNoV genogroups comprising four genotypes of virus (including four GII.4 variants, and GII.3, GII.17 and GI.1 strains) in human jejunal enteroid monolayers (table S1).
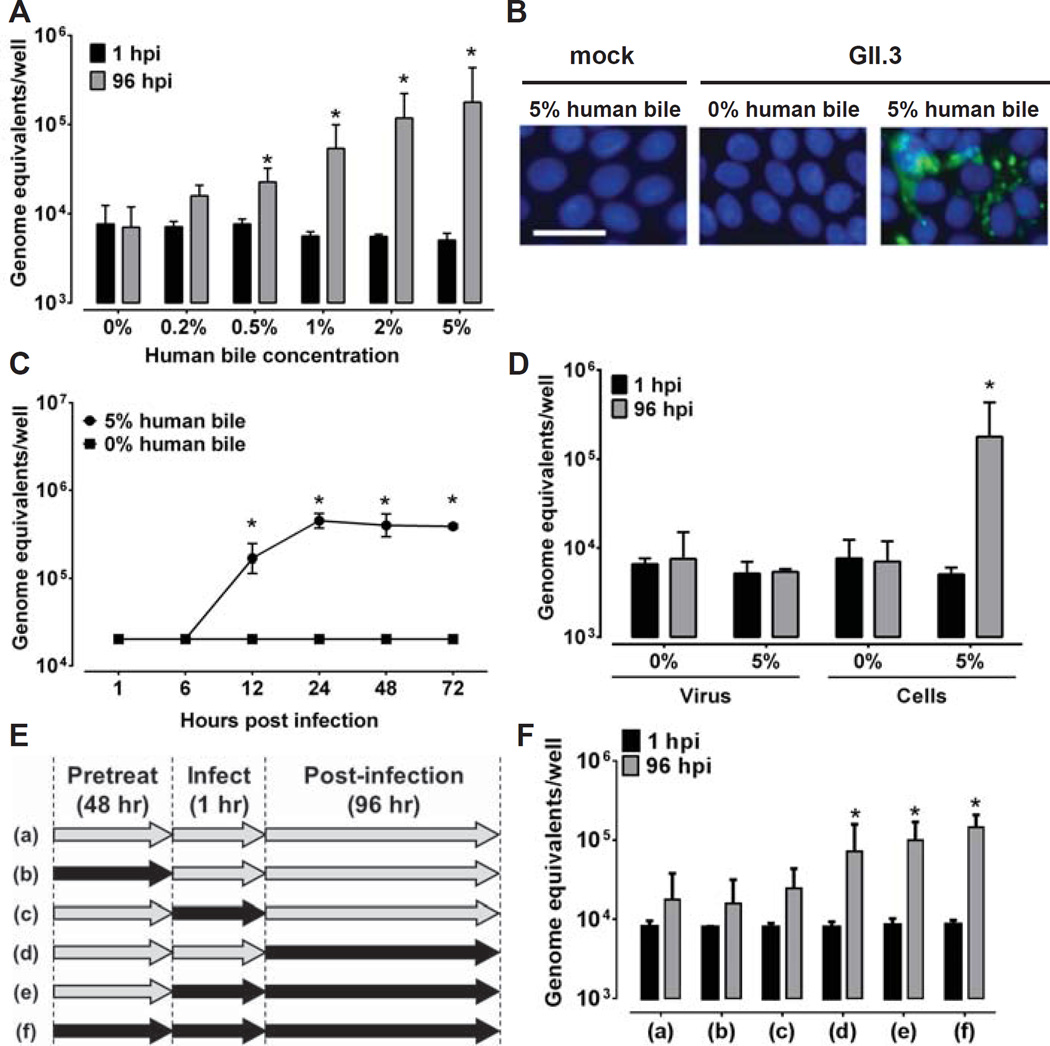
Bile is required for GII.3 HuNoV replication and affects the cells. Jejunal HIE monolayers were pretreated (A-C) with the indicated concentrations of human bile for 2 days and then inoculated with GII.3 stool filtrate [(A, C) 4.3×105 or (B) 4.3×107 genome equivalents] and incubated with the same bile concentrations as used for pretreatment (see supplementary methods). (B) VP1 was detected in methanol-fixed monolayers at 24 hpi using guinea pig anti-GII.3 VLP antiserum (green) and DAPI to detect nuclei (blue). Scale bar = 25 µm. (D) To determine if the effect of bile was on the virus or the cells, the virus was either not treated or treated with 5% human bile for 1 hour at 37°C, and then diluted to decrease the bile concentration to 0.025% prior to infection of HIE monolayers not pretreated with bile. Alternatively, cells were either not treated or treated with 5% human bile for 2 days prior to and during infection. Inoculations were performed with 4.3×105 genome equivalents. (E) Schematic showing with black arrows when bile was added to HIEs for the experiment shown in (F). For A, C, D and F, genome equivalents were determined as indicated in Fig. 1. Error bars denote standard deviation. *, P< 0.05 comparing genome equivalents to 1 hpi.
We next evaluated whether the requirement of bile for GII.3 HuNoV infection and replication was due to a bile effect on the HIE cells or the virus. Stool filtrate was pretreated with 5% bile or PBS for 1 hour and then diluted in PBS (final concentration of 0.025% in the bile-treated sample) prior to inoculating HIE cultures not treated with bile. HIEs also were either not treated or treated with 5% bile for 48 hours prior to and during infection. The pre-treatment of cells with bile was carried out for a longer duration as compared to pre-treatment of the virus with bile because bile has multiple known effects on cellular functions, including acting as a detergent, increasing digestion and absorption of fat, and regulating metabolic and inflammatory processes by activating various signaling pathways (20). An increase in genome equivalents was observed only when HIEs, not the virus, was treated with bile (Fig. 2D), indicating that the bile effect is on the cells and not the virus. Further assessment of bile treatment revealed that addition of bile to cultures during or after virus adsorption but not prior to adsorption is required for GII.3 replication (Fig. 2E and F). Testing of heat- or trypsin-treated bile for GII.3 infections in HIEs found no effect on replication (Fig. S8), indicating that the active factor is not proteinaceous. The successful cultivation of GII.3 HuNoV required both novel HIE cultures and supplementary bile as the addition of bile to transformed epithelial cell lines including Huh7, Vero, HEK293FT and undifferentiated or differentiated Caco-2 BBe cells did not promote HuNoV replication (Fig. S9). These results indicate HIEs and bile together mimic the human replication niche of HuNoVs.
The sensitivity of the HIE culture system to support HuNoV replication was evaluated by determining the lower limit of virus required for successful infection. For this, the infectious dose 50% (ID50) for GII.4 and GII.3 HuNoVs was calculated by the Reed-Muench method (21). The ID50 for GII.4/2012-1 and GII.3 HuNoVs were ~1,200 and ~2.0×104 genome equivalents/well, respectively, assessed at 7 days post-infection (dpi, geometric mean of two experiments, representative experiments shown in Fig. S10A and B). These results indicate that this replication system is amenable to determining the infectivity of low levels of virus, as has been observed in emesis, contaminated food and in fecal samples after recovery from illness (8, 22, 23). This replication system also will allow evaluation of whether virus, currently detected in a variety of samples using molecular methods, is infectious and poses a potential risk to human health.
HuNoVs replicate in enterocytes in cultures from different segments of the small intestine
The site of replication of HuNoVs in immunocompetent individuals is unknown, although histologic alterations have been observed in biopsies from volunteers infected with Norwalk virus (24) and antigen has been detected in duodenal, jejunal and to a lesser extent in ileal enterocytes from gnotobiotic pigs infected with a GII.4 HuNoV (25). We therefore evaluated whether HuNoVs infect cells derived from different regions of the small intestine by inoculating HIE cultures made from biopsies obtained from different intestinal segments that retain segment-specific properties (26). GII.4 and GII.3 HuNoVs replicated in enteroids derived from duodenal, jejunal and ileal intestinal segments (Fig. 3). Replication varied by strain and intestinal segment, with GII.4 variants showing 11–1535 fold increases between 1 and 96 hpi in the three segments and GII.3 virus showing 3–51 fold increases. We investigated whether HuNoV replicated in cell types other than enterocytes. HuNoV antigen was not detected in goblet (n=200 cells) or enteroendocrine cells (n=50 cells) assessed in duodenal, jejunal and ileal HIEs. Together, the growth of HuNoVs in HIEs from all segments of the small intestine detected by RNA replication and confocal staining indicates enterocytes (Fig. 1B) are the primary target for infection and replication.

GII.4 variants and GII.3 HuNoVs replicate in HIEs generated from different intestinal segments. Duodenal (D1), jejunal (J2), and ileal (IL16) HIEs were treated with 1% sow bile for the GII.4 variants or 5% human bile for GII.3 for 48 hours, and then inoculated with the indicated HuNoVs (GII.4/2006b-2, GII.4/2009, GII.4/2012-1: 9×105; GII.4/2006b-3: 5.5×105; GII.3: 4.3×105 genome equivalents) and cultured in the presence of bile. Genome equivalents were determined as indicated in Fig. 1. Error bars denote standard deviation.
Secretor status of HIEs affects strain-specific HuNoV replication
HuNoV infection is dependent on expression of genetically-determined histoblood group antigens (HBGAs), and genetic resistance to some HuNoV genotypes has been documented in challenge and outbreak studies (27). The presence of a functional fucosyltransferase 2 (FUT2, secretor positive genotype) enzyme, which transfers fucose to HBGA precursors in gastrointestinal cells in secretor positive persons, correlates with susceptibility to infection with most GII.4 HuNoVs. We generated HIEs from secretor positive and negative persons to determine whether these cultures recapitulate genotype-specific patterns of HuNoV susceptibility (14). All secretor positive jejunal HIEs supported productive replication of GII.4 variants (44–1270 fold) and GII.3 HuNoVs (10–173 fold) based on increases in genome equivalents between 1 and 96 hpi (Fig. 4A). In contrast, GII.4 strains did not infect HIEs generated from secretor negative individuals when assayed at 96 hpi or 6 dpi (Fig. 4B and C, respectively). However, GII.3 virus infected 2 of 3 secretor negative HIEs, one of which was positive only after 6 days in culture (Fig. 4B and C, respectively). These results mirror epidemiologic data wherein GII.3, but not GII.4 HuNoVs, can infect some secretor negative individuals (27) and indicate that HuNoV infection of HIEs is a biologically relevant system that mimics infections in genetically-defined individuals. Further analysis of genetically-defined HIEs will aid in determining additional host susceptibility factors to infection.

Replication of GII.4 strains but not GII.3 depends on HIE secretor status. (A) Secretor positive jejunal (J2, J3, J6 and J11) or (B) secretor negative jejunal (J4, J8 and J10) HIEs were inoculated with the indicated GII.4 or GII.3 HuNoVs (with the same amounts of genome equivalents as indicated in Fig. 3) in the presence of bile (1% sow bile for GII.4 variants or 5% human bile for GII.3) for 96 hours. At 96 hpi, GII.4 strains replicate in secretor positive HIEs but not secretor negative lines, while GII.3 replicates in all secretor positive HIEs and one secretor negative line (J8). (C) At 6 dpi, the GII.3 virus shows replication in an additional secretor negative HIE (J4) while no growth of GII.4/2012-1 virus is seen. A secretor positive J2 HIE is included as control to show replication of GII.4 at 6 dpi. (A–C) Genome equivalents were determined as indicated in Fig. 1. Error bars denote standard deviation.
HuNoV culture in HIEs allows evaluation of virus neutralization and inactivation
Functional antibodies in serum that block the binding of HuNoV VLPs to HBGAs correlate with protection against clinical gastroenteritis in volunteer challenge and vaccination studies (28–30). HBGA-blocking assays examine the ability of human serum to block the interaction of HuNoV VLPs with H type 1 and H type 3 glycans and porcine gastric mucin (31, 32), and the antibody titer that results in 50% blocking (BT50) has been used as a surrogate for virus neutralization (28). We used the HIE cultivation system to directly measure virus neutralization. Neutralizing antibody titers (the reciprocal antibody dilution present in serum able to reduce virus yields by 50% compared to virus incubated in media alone) were compared to HBGA-blocking titers. Serum samples from two individuals, one with a high BT50 and another with a low BT50 against GII.4 VLPs, were tested (Table 1). Both samples had low BT50s against GII.3 VLPs. The neutralization titers were higher than the BT50 values (Table 1 and Fig. S11A–D) and virus-induced CPE was neutralized (Fig. S11E), suggesting that virus neutralization is a more sensitive assay than the HBGA blocking assay and, besides HBGA-blocking epitopes, additional neutralization epitopes may exist on norovirus particles.
Table 1
Comparison of BT50 and 50% neutralization titers.
GII.3 | GII.4 | |||
---|---|---|---|---|
Serum | BT501 | 50% neutralization2 | BT501 | 50% neutralization2 |
1 | 187 | 990 | 671 | 105,000 |
2 | 70 | 835 | 53 | 214 |
The previous inability to cultivate HuNoVs has hampered the development of strategies to control and prevent HuNoV infection, and determination of the effectiveness of existing methods to inactivate virus to prevent transmission in various settings, including in food or on contaminated surfaces. The persistence of HuNoVs in the environment, high transmissibility and the problem of chronic infection of immunocompromised individuals document a need for antiviral treatment and prophylaxis of norovirus infections. To determine if the HIE infection model is suitable for testing virus inactivation, we evaluated GII.3 and GII.4 HuNoV inactivation by gamma irradiation and heat treatment. No growth was observed following gamma irradiation of either GII.4 or GII.3 HuNoVs (Fig. 5A). Compared to incubation of GII.4 or GII.3 viruses at room temperature for 60 minutes, both viruses were inactivated by heating at 60°C for as little as 15 min; no increase in yield was detected from 1 to 72 hpi (Fig. 5B and C). These studies indicate HuNoV infection of HIEs will allow evaluation of new methods to inactivate HuNoVs and to measure the effectiveness of disinfectants and sanitizers, including characterization of the efficacy of both traditional and novel control measures.
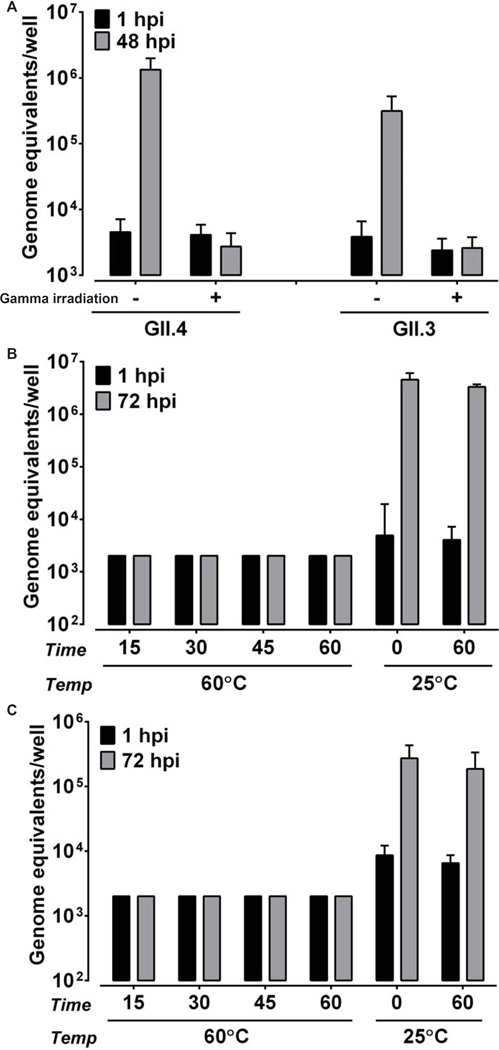
Inactivation of GII.4 and GII.3 HuNoV infectivity by gamma irradiation and heat treatment. (A) GII.4/2012-1 and GII.3 HuNoVs were gamma irradiated or incubated at room temperature overnight. (B) GII.4/2012-1 or (C) GII.3 (9×105 and 4.3×105 genome equivalents, respectively) were heat-inactivated at 60°C for the indicated time points or incubated at room temperature for 0 and 60 minutes. Jejunal HIEs were inoculated with each sample. Genome equivalents were determined as indicated in Fig. 1. Error bars denote standard deviation.
Implications of in vitro replication of HuNoV for understanding its biology
HuNoV was visualized by Albert Kapikian in 1972 (17), but conditions to grow these viruses in vitro have remained an unsolved mystery for more than 40 years. We demonstrate the successful development of a robust in vitro replication system for multiple HuNoV strains and provide insights into HuNoV strain-specific growth requirements. HuNoV replication in HIEs is robust based on achieving several log10 increases in replication of multiple variants of the epidemiologically predominant GII.4 HuNoVs in HIE lines from different small intestinal segments from multiple immunocompetent individuals; replication was documented by expression of structural and nonstructural proteins, production and release of virus particles into culture supernatants, and successful passaging of virus. The cultivation system described in this study has biologic relevance with replication of different strains being consistent with known host restriction based on HBGA expression. Variability in replication between different HIE cultures is observed; specific cultures (secretor positive) are highly susceptible to HuNoV strains and other cultures (secretor negative) show variable resistance to infection with specific HuNoV strains. These results mirror infectivity patterns seen in epidemiological studies. Future work with additional strains is needed to understand the basis for this variability particularly for infection of different secretor negative lines with GII.3 viruses.
HuNoVs replicate in enterocytes from different intestinal segments in HIEs. In addition, factors present in the intestinal milieu, such as bile, enhance or are required for replication to occur. Bile obtained from various mammalian sources (human, sow, piglet, porcine and bovine) mediate this effect, although there is variability between bile sources and virus strains. These results are consistent with previous data from intestinal biopises from HuNoV-infected immunocompromised transplant patients and studies in large animals (gnotobiotic pigs and newborn calves) infected orally with HuNoV GII.4 or bovine norovirus GIII.1 strains, respectively, where enterocytes in different segments of the small intestine are clearly infected and express viral antigen (25, 33, 34). Filtered stool was used as inoculum in the present study and bacterial LPS was not required for infectivity. These results differ from reports of cultivation of a single strain of HuNoV in B cells where unfiltered inocula and commensal bacteria are required as cofactors (9, 10), and of HuNoV replication in other animal models following non-oral routes of inoculation, including intraperitoneal injection of immunodeficient mice (35) or intravenous injection of chimpanzees (36); in those models, virus was not detected in intestinal epithelium but in cells in the lamina propria with some expressing DC-SIGN (chimpanzees) or with a macrophage-like morphology (mice). Replication of HuNoVs in enterocytes in HIEs supports the observation that another site(s) of primary replication besides B cells must exist because HuNoVs can infect B-cell deficient patients (37, 38). Our results are reminiscent of initial conditions that required the addition of intestinal contents from gnotobiotic piglets to successfully culture a porcine enteric calicivirus (PEC), a member of the Sapovirus genus of the Caliciviridae family, using primary porcine kidney cells (39). Species-specific bile enhanced primary replication in that system and subsequent studies showed that bile acids and a continuous porcine kidney cell line can support PEC replication (40). Additional studies are needed to determine the active components of bile needed to support HuNoV replication in HIEs. The active component(s) in bile and mechanism(s) of action required for HuNoV cultivation in HIEs remain to be fully characterized but initial characterization demonstrates that it is not a protein. The establishment of this new cultivation system will facilitate applications in many different realms of public health importance such as food safety, development of new diagnostics, vaccines and therapeutics, and advance research on HuNoV evolution, immunity and pathogenesis.
Acknowledgments
This work was supported in part by Public Health Service grant PO1 AI 057788 (to M.K.E.) and Agriculture and Food Research Initiative Competitive Grant 2011-68003-30395 from the USDA National Institute of Food and Agriculture. We acknowledge the assistance of the Study Design and Clinical Research Core and Cellular and Molecular Morphology Core of the Texas Medical Center Digestive Diseases Center and the Cytometry and Cell Sorting Core, which are supported in part by Public Health Service grants P30 DK-56338, P30 CA-125123 (to C.K. Osborne), NIAID AI036211 and the Dan L. Duncan Cancer Center at Baylor College of Medicine. We thank Drs. BVV Prasad, Timothy Palzkill, Susan Marriott and Mark Donowitz for critical reading of the manuscript.
Enteroids are available with a MTA. Baylor College of Medicine has filed a patent application related to this work.
Footnotes
SEC, RLA and MKE supervised the project. KE, KM, JRB, UK, VRT, LQ, BK, X-LZ, and FN designed and performed experiments and analyzed data. SEC, RLA, SR, SEB, and MKE designed experiments and analyzed data. ARO, DB and DYG provided reagents and conceptual advice. MKE, SEC, and KE wrote the initial draft of the manuscript. All authors provided critical review and final approval of the manuscript.
All relevant data are within this paper and Supplementary information files.
The authors declare no conflicts of interest.
Supplementary Materials:
Materials and methods
Fig. S1–S11
Table S1
References and Notes
Full text links
Read article at publisher's site: https://doi.org/10.1126/science.aaf5211
Read article for free, from open access legal sources, via Unpaywall:
https://science.sciencemag.org/content/sci/353/6306/1387.full.pdf
Citations & impact
Impact metrics
Article citations
Ultraviolet (UV-C) Light Systems for the Inactivation of Feline Calicivirus and Tulane Virus in Model Fluid Foods.
Food Environ Virol, 16(4):506-515, 10 Oct 2024
Cited by: 0 articles | PMID: 39384722
Development and characterization of segment-specific enteroids from the pig small intestine in Matrigel and transwell inserts: insights into susceptibility to porcine epidemic diarrhea Virus.
Front Immunol, 15:1451154, 17 Sep 2024
Cited by: 0 articles | PMID: 39355235 | PMCID: PMC11442308
Bivalent norovirus mRNA vaccine elicits cellular and humoral responses protecting human enteroids from GII.4 infection.
NPJ Vaccines, 9(1):182, 01 Oct 2024
Cited by: 0 articles | PMID: 39353926 | PMCID: PMC11445234
Organoids: development and applications in disease models, drug discovery, precision medicine, and regenerative medicine.
MedComm (2020), 5(10):e735, 21 Sep 2024
Cited by: 0 articles | PMID: 39309690 | PMCID: PMC11416091
Review Free full text in Europe PMC
Effect of chorioamnionitis on postnatal growth in very preterm infants: a population-based study in Japan.
Arch Gynecol Obstet, 01 Oct 2024
Cited by: 0 articles | PMID: 39354115
Go to all (728) article citations
Other citations
Data
Data behind the article
This data has been text mined from the article, or deposited into data resources.
BioStudies: supplemental material and supporting data
Similar Articles
To arrive at the top five similar articles we use a word-weighted algorithm to compare words from the Title and Abstract of each citation.
Human Norovirus Cultivation in Nontransformed Stem Cell-Derived Human Intestinal Enteroid Cultures: Success and Challenges.
Viruses, 11(7):E638, 11 Jul 2019
Cited by: 55 articles | PMID: 31336765 | PMCID: PMC6669637
Review Free full text in Europe PMC
New Insights and Enhanced Human Norovirus Cultivation in Human Intestinal Enteroids.
mSphere, 6(1):e01136-20, 27 Jan 2021
Cited by: 63 articles | PMID: 33504663 | PMCID: PMC7885322
Bile acid-sensitive human norovirus strains are susceptible to sphingosine-1-phosphate receptor 2 inhibition.
J Virol, 98(7):e0202023, 17 Jun 2024
Cited by: 1 article | PMID: 38884472
Antiviral Activity of Olanexidine-Containing Hand Rub against Human Noroviruses.
mBio, 13(2):e0284821, 17 Mar 2022
Cited by: 9 articles | PMID: 35297675 | PMCID: PMC9040745
Funding
Funders who supported this work.
Agriculture and Food Research Initiative (1)
Grant ID: 2011-68003-30395
Dan L. Duncan Cancer Center at Baylor College of Medicine
NCI NIH HHS (1)
Grant ID: P30 CA125123
NIAID NIH HHS (3)
Grant ID: P30 AI036211
Grant ID: U19 AI116497
Grant ID: P01 AI057788
NIDDK NIH HHS (1)
Grant ID: P30 DK056338
National Institute of Allergy and Infectious Diseases (1)
Grant ID: AI036211
Public Health Service (3)
Grant ID: P30 DK-56338
Grant ID: PO1 AI 057788
Grant ID: P30 CA-125123