Abstract
Free full text

Utility-value intervention with parents increases students’ STEM preparation and career pursuit
Significance
The need for students trained in science, technology, engineering, and mathematics (STEM) jobs is growing rapidly in the United States, yet students do not enroll in the necessary courses to prepare for STEM careers. In a randomized controlled trial, parents in the utility–value intervention group received materials detailing the importance of STEM for their adolescents in high school. The intervention increased mathematics and science ACT scores and course-taking in high school. This greater high-school STEM preparation was associated, 5 y later, with increased STEM career pursuit. These findings suggest that educational policies should promote the personal relevance of high-school mathematics and science courses and involve parents in helping to promote STEM preparation and career pursuit.
Abstract
During high school, developing competence in science, technology, engineering, and mathematics (STEM) is critically important as preparation to pursue STEM careers, yet students in the United States lag behind other countries, ranking 35th in mathematics and 27th in science achievement internationally. Given the importance of STEM careers as drivers of modern economies, this deficiency in preparation for STEM careers threatens the United States’ continued economic progress. In the present study, we evaluated the long-term effects of a theory-based intervention designed to help parents convey the importance of mathematics and science courses to their high-school–aged children. A prior report on this intervention showed that it promoted STEM course-taking in high school; in the current follow-up study, we found that the intervention improved mathematics and science standardized test scores on a college preparatory examination (ACT) for adolescents by 12 percentile points. Greater high-school STEM preparation (STEM course-taking and ACT scores) was associated with increased STEM career pursuit (i.e., STEM career interest, the number of college STEM courses, and students’ attitudes toward STEM) 5 y after the intervention. These results suggest that the intervention can affect STEM career pursuit indirectly by increasing high-school STEM preparation. This finding underscores the importance of targeting high-school STEM preparation to increase STEM career pursuit. Overall, these findings demonstrate that a motivational intervention with parents can have important effects on STEM preparation in high school, as well as downstream effects on STEM career pursuit 5 y later.
A science, technology, engineering, and mathematics (STEM) “pipeline problem” exists in the United States, where STEM careers are growing rapidly, yet too few students are prepared to take advantage of them (1). STEM occupations are estimated to grow by 17% between 2009 and 2018, compared with 9.8% for non-STEM occupations, and it is expected that the majority (92%) of all STEM jobs will require some postsecondary education (2, 3). Furthermore, in 2008 only 4% of bachelor degrees in the United States were earned in engineering, compared with 31% in other countries, such as China (4, 5). Increasing the number of individuals qualified for and actually pursuing STEM careers can help the United States stay competitive with other advancing countries and improve the global economy through increased innovation and technology (6, 7). Therefore, it is vital to develop a population of college graduates with STEM degrees to meet these ever-increasing demands.
To increase the pool of STEM workers, we need to increase the number of students with the necessary knowledge and skills needed for STEM careers. Currently, students in the United States lack mathematical and scientific skills when compared with students in other nations. In fact, international test score results show that 15-y-olds in the United States rank 35th in mathematics literacy and 27th in science literacy among participating nations (4, 5). Similar deficits in students’ STEM competence are revealed by examining students’ college preparatory examination scores. Specifically, in a recent study of ACT test-takers, only 44% met the college readiness level for mathematics and 36% for science (8).
One way to develop students’ mathematics and science skills is to increase enrollment in STEM courses in high school. Research supports the link between increased exposure to STEM topics in classes and higher STEM achievement (9). However, students are allowed to opt out of elective STEM courses in high school, and as a result, many students are not exposed to the advanced mathematics or science classes necessary to attain postsecondary STEM degrees. For example, in 2009 only 17.8% of high-school students enrolled in physics and 21.0% enrolled in calculus or precalculus (10).
The importance of high-school STEM preparation can be seen when examining students’ STEM career pursuit after high school and in college. Research shows that advanced high-school mathematics (e.g., algebra II, calculus) and science (e.g., physics, chemistry) courses are crucial predictors of STEM major enrollment in college (1, 11). Furthermore, in college there is a high degree of attrition from STEM majors. For example, only 62% of students between 2003 and 2009 who entered 4-y colleges as STEM majors graduated with a bachelor degree from a STEM domain, and insufficient STEM preparation in high school plays a role in this lack of persistence toward STEM careers (1, 2, 12). Thus, not only is STEM preparation in high school lacking, but it also affects entry into STEM majors in college, persistence toward those majors, and ultimately entry into STEM careers.
Although many factors contribute to the lower levels of STEM preparation and career pursuit, research in psychology shows that this is, in part, a motivational problem (13). Expectancy-value theory posits that individuals make achievement-related choices, such as how hard they will study for a test or the types of courses in which they enroll, based on (i) their expectations for success and (ii) their subjective task value (i.e., the importance of a task) (13). Expectancy-value theory defines different types of subjective task value: intrinsic value (a task is important because it is enjoyable), attainment value (a task is important because it is related to your identity), and utility value (a task is important because it is relevant or useful for a current or future goal). Researchers have recently focused on increasing students’ perceived utility value with interventions because it is viewed as malleable to outside forces. Furthermore, correlational and longitudinal research has shown that utility value is a significant predictor of mathematics and science course-taking and STEM major enrollment (1, 14, 15). Thus, intervening to increase students’ perceived utility value in STEM domains is one promising direction for increasing students’ high-school STEM preparation as well as STEM career pursuit.
Much of the intervention research has focused on student-centered interventions designed to increase students’ utility value in a topic or task, either by communicating utility value directly to students (i.e., telling them why it is useful) or asking students to generate their own ideas about why STEM topics are useful (i.e., asking them to write about why it is personally useful) (16, 17). Results suggest that these student-centered interventions can improve students’ motivation and performance in STEM domains, but the positive effects of these interventions often depend on individual differences. For example, explaining the utility of a novel math technique improved performance and interest for students who were already highly interested or confident in their mathematics ability (18, 19). Conversely, asking students to write about the utility of STEM topics in their lives proved to be most effective for students who lacked confidence (20) or who were members of underrepresented groups in colleges (21). For example, in a randomized controlled trial in high-school science classes, less confident students who were asked to write about the utility of the course showed increased interest in science and higher science grades than less-confident students in the control group (20). Although these studies suggest that utility-value interventions have positive effects for particular subgroups, utility-value interventions that combine direct communication of utility value with a self-generated component show a more universal positive effect on students’ motivation and performance (22). Furthermore, larger-scale randomized controlled trials have confirmed that the effects found in initial studies can improve high-school and college students’ motivation at scale and with representative groups of students (21, 23). Overall, findings from these student-centered intervention studies demonstrate consistent positive causal effects of utility value on motivation, interest, and academic performance (16, 17, 24).
Even though student-centered interventions have been shown to be successful, schools do not always have the class time, the teacher training time, or the resources available to implement these interventions in classrooms. One alternative and potentially powerful route for increasing students’ perception of the relevance of STEM topics is by engaging their parents, given that parents play a critical role in shaping their students’ motivational beliefs (25, 26). Past research shows that parents’ motivational beliefs, such as the importance they place on mathematics and science for their child, predict their children’s motivational beliefs and educational outcomes in those subject areas (27). Parents are hypothesized to affect their children’s attitudes through multiple mechanisms, including parents’ behaviors (28, 29) and the quality of parents’ conversations with their children (30). These correlational findings suggest that parents can play an important role in communicating utility-value beliefs to their children and in increasing their children’s STEM motivation and career pursuit. Therefore, we tested whether an intervention designed to help parents communicate the utility of mathematics and science to their children would improve students’ STEM motivation and achievement.
Current Study
There is a long history of so-called two-generation interventions, which are defined as interventions that involve both parents and children (31). Consistent with this tradition of two-generation interventions, Harackiewicz et al. (32) tested an intervention that encouraged parents to communicate the utility value of STEM topics to their high-school–aged children. Parents were randomly assigned to the intervention or control condition, and the intervention materials (i.e., two brochures and a website) were distributed to parents when their children were in the 10th and 11th grades of high school. Intervention materials provided information about the utility and relevance of mathematics and science for high-school students and encouragement to discuss this information with their child. In addition, parents were given advice and recommendations about how to most effectively communicate this information to their children. For example, parents were advised to personalize the information to their children’s interests and goals by helping their children generate their own connections with STEM topics and supporting these connections with information and examples from the intervention materials. Parents in the control group did not receive any additional materials. Students could only be affected by the intervention through interactions with their parents because none of the intervention materials were ever directed to students. Harackiewicz et al. (32) found that this intervention increased students’ STEM course-taking in the 11th and 12th grades of high school by approximately one semester of additional STEM course-taking on average. Moreover, Rozek et al. (33) showed that those effects were mediated by changes in parents’ and students’ attitudes about STEM topics.
The current study extended the original study in two important ways: first, we investigated if this parental intervention affected students’ mathematics and science achievement in high school; second, we conducted a 5-y follow-up when the participants were 20-y-old to examine students’ STEM career aspirations, college STEM course-taking, likelihood of choosing a STEM major in college, and perceptions of STEM value. To examine academic achievement as an additional measure of students’ high-school STEM preparation, we collected and analyzed students’ college preparatory standardized test scores (i.e., ACT scores) from their high-school records. Scores on this test reflect knowledge accrued from mathematics and science courses taken in high school, and we hypothesized that scores would be higher in the intervention condition, given that those students enrolled in more STEM courses. Furthermore, we tracked participants 5 y after the initial intervention (age 20) to assess long-term intervention effects on posthigh-school STEM career pursuit outcomes. At this time, we asked students about the type of career they desired, how many STEM courses they had taken in college, their current plans for their college major, and about their STEM attitudes.
The goal of the current study was to examine the effects of the intervention on an additional high-school STEM preparation variable (i.e., ACT scores), as well as downstream effects on posthigh-school STEM career pursuit. First, we hypothesized that this parent-centered utility-value intervention would increase students’ mathematics and science ACT scores, in addition to the already documented effects on 11th and 12th grade mathematics and science course-taking. Second, we predicted that these effects on high-school outcomes would better prepare students for STEM careers. Thus, we predicted that the intervention would have long-term positive effects on posthigh-school STEM career pursuit (i.e., college STEM course-taking, the likelihood of choosing a STEM major, self-reported STEM value, and whether students aspired to a STEM career 5 y after the initial intervention) to the extent that the intervention improved students’ high-school STEM preparation. Although the intervention could also directly affect posthigh-school outcomes, research on the lasting effects of psychological interventions suggests that they are maintained by initiating a chain of positive recursive processes (34). That is, the intervention may change some outcome (behavior or psychological process) in the short term, and that outcome then has an effect on later outcomes. We tested our hypothesis by examining the indirect effect of the intervention on STEM career pursuit variables via high-school STEM preparation variables. In all, this study is unique in terms of being able to test both the short-term and long-term effects of a cost-effective parent-centered intervention on critical STEM preparation and career pursuit outcomes.
Results
Statistics and Correlations.
Descriptive statistics and zero-order correlations are reported in Table S1.
Table S1.
Zero-order correlations and descriptive statistics for major study variables
Variable | 1 | 2 | 3 | 4 | 5 | 6 | 7 | 8 |
1. Parental education | — | |||||||
2. 11th and 12th grade mathematics and science course-taking (semesters) | 0.22** | — | ||||||
3. Mathematics and science ACT score | 0.38*** | 0.30** | — | |||||
4. Perceived STEM value | 0.00 | 0.23** | 0.20* | — | ||||
5. College STEM course-taking (semesters) | 0.29* | 0.43*** | 0.39** | 0.49*** | — | |||
6. STEM career aspirations | 0.06 | 0.34*** | 0.20 | 0.60*** | 0.47*** | — | ||
7. College STEM major | 0.11 | 0.30** | 0.15 | 0.55*** | 0.48*** | 0.56*** | — | |
8. Perceived parental STEM support | 0.13 | 0.12 | 0.31** | 0.53*** | 0.28** | 0.34*** | 0.30*** | — |
Mean | 15.42 | 7.85 | 24.55 | 5.50 | 5.86 | 61.70 | — | 5.93 |
SD | 1.92 | 2.57 | 4.39 | 1.50 | 3.87 | 20.90 | — | 1.01 |
Structural Equation Model.
We used structural equation modeling to examine the relationships between variables over time during and after high school (Fig. 1 for the theoretical model). There were seven base predictors for our model, which were used to predict all high-school and posthigh-school outcomes: the intervention (+1 for intervention group and −1 for control group), students’ gender (+1 for male and −1 for female), level of parental education (z-scored), and all resulting two-way interactions as well as the three-way interaction between the intervention, students’ gender, and parental education.
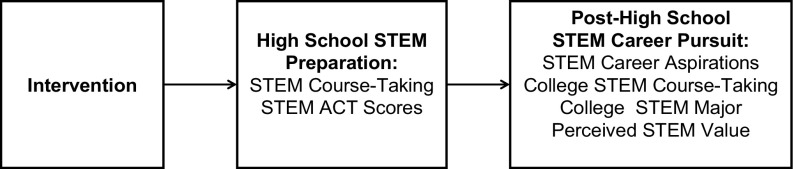
Theoretical model of how the intervention should have long-term effects on STEM career pursuit through increased STEM preparation in high school.
We hypothesized that increased high-school STEM preparation would have positive long-term effects on posthigh-school STEM career pursuit; we therefore allowed mathematics and science ACT scores and mathematics and science course-taking in 11th and 12th grade to predict the posthigh-school outcomes, along with the base predictors. All variables measured at the same time point (e.g., high school) were allowed to correlate. To assess the long-term effect of the intervention on posthigh-school STEM career pursuit, we examined the total indirect effect of the intervention on posthigh-school outcomes through high-school variables (35). Missing data were accounted for by using full information maximum-likelihood methods (36). The model was saturated, which does not allow for a meaningful test of model fit. For additional information on the model and measures, SI Materials and Methods (Table S2 summarizes the significant direct and indirect effects).
Table S2.
Summary of significant parameter estimates for direct and indirect effects for the model
Parameter | z | β |
Direct effects | ||
![]() | ||
![]() ![]() | 2.22* | 0.16 |
![]() ![]() | 3.13** | 0.23 |
![]() | ||
![]() ![]() | 2.46* | 0.19 |
![]() ![]() | 4.92*** | 0.42 |
![]() | ||
![]() ![]() | 2.29* | 0.22 |
![]() | ||
![]() ![]() | 3.77*** | 0.34 |
![]() ![]() | 2.36* | 0.26 |
![]() | ||
![]() ![]() | 3.13** | 0.30 |
![]() | ||
![]() ![]() | 2.82** | 0.29 |
Indirect effects | ||
![]() | 2.54* | — |
![]() | 2.15* | — |
![]() | 2.19* | — |
Direct intervention effects on high-school STEM preparation.
The intervention had a significant effect on mathematics and science ACT score (z = 2.46, β = 0.19, P < 0.05) (Fig. 2A) and, as previously reported (32), 11th and 12th grade STEM course-taking (z = 2.22, β = 0.16, P < 0.05). Specifically, the intervention increased mathematics and science ACT scores by about 12 percentile points (Fig. 2B) and 11th and 12th grade STEM course-taking by nearly one semester (0.81 semesters). Because students were enrolling in 11th and 12th grade STEM courses and taking the ACT during the same time period, these variables were allowed to correlate in the model, but we cannot determine if increased course-taking led to higher ACT scores or vice-versa.
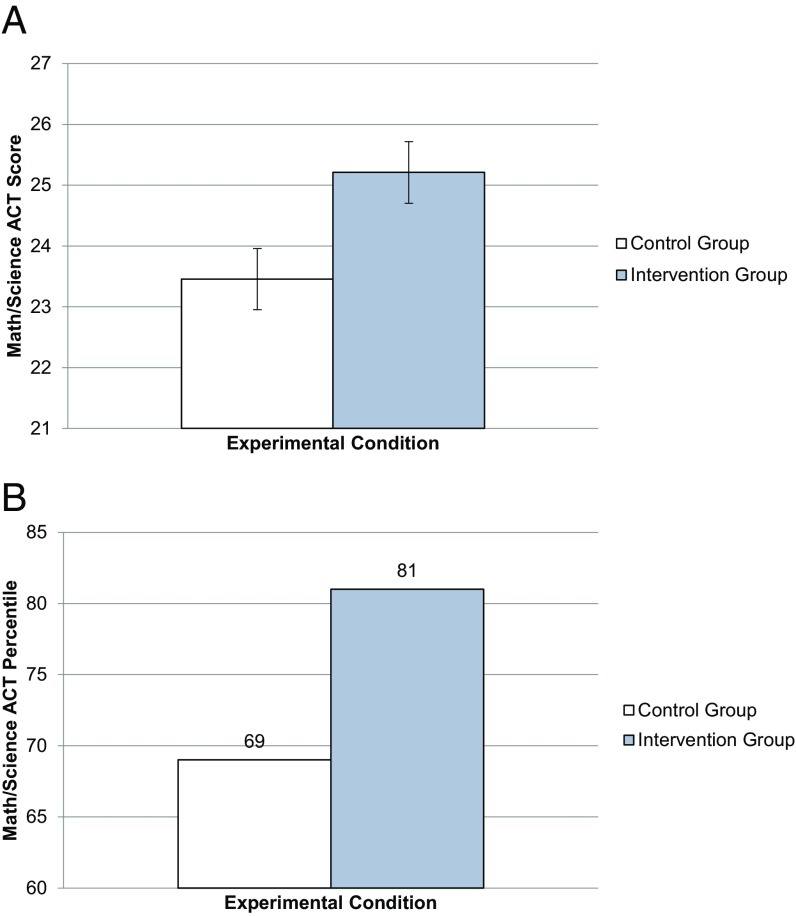
Effects of the intervention on ACT scores. The intervention significantly improved mathematics and science ACT scores (A), translating to a 12 percentile point increase (B). Effects are significant, even when controlling for students’ performance on state high-school standardized mathematics and science tests given before the intervention. Error bars represent ±1 SE of the mean.
Ancillary ACT score analysis.
Supplemental analyses were also conducted to examine if there was a difference on preintervention performance and if controlling for preintervention performance changed the intervention effect on mathematics and science ACT score (Table S3). Accordingly, we collected and examined high-school state standardized mathematics and science test scores for students from before the intervention [10th grade Wisconsin Knowledge and Concepts Examination (WKCE) scores] and found no significant difference between intervention and control groups on students’ preintervention test scores (z = −0.29, β = −0.03, P = 0.77). Moreover, even when controlling for these preintervention test scores, the positive effect of the intervention on mathematics and science ACT score was significant (z = 3.61, β = 0.20, P < 0.001).
Table S3.
Unstandardized βs and SEs for regressions on ACT scores
Predictor | Math and science ACT | Math and science ACT |
Intervention | 0.88* (0.36) | 0.92*** (0.25) |
Parental education | 1.70*** (0.40) | 0.53 (0.30) |
Gender | 0.13 (0.36) | −0.17 (0.26) |
Intervention × Gender | 0.27 (0.36) | 0.05 (0.26) |
Intervention × Parental education | −0.40 (0.40) | −0.01 (0.29) |
Parental education × Gender | 0.35 (0.40) | −0.04 (0.29) |
Intervention × Parental education × Gender | −0.58 (0.40) | 0.02 (0.29) |
Preintervention test scores | 0.10*** (0.01) |
Direct intervention effects on posthigh-school STEM career pursuit.
We estimated a secondary model that was identical to the main structural equation model, except that high-school STEM course-taking and ACT scores were not included. This approach allowed us to assess whether the intervention directly affected posthigh-school outcomes. There were no significant direct intervention effects on posthigh-school STEM career pursuit variables (Ps > 0.36) (Table S4).
Table S4.
Unstandardized betas and SEs for direct effects on posthigh-school outcomes in a structural equation model without high-school STEM preparation variables
Predictor | College STEM course-taking | Student’s’ STEM value | College STEM major | STEM career aspirations |
Intervention | 0.02 (0.35) | −0.02 (0.13) | 0.00 (0.05) | 1.70 (1.90) |
Parental education | 1.12** (0.36) | 0.00 (0.14) | 0.05 (0.05) | 1.11 (1.99) |
Gender | −0.11 (0.35) | 0.10 (0.13) | 0.00 (0.05) | 0.14 (1.90) |
Intervention × Gender | −0.16 (0.35) | −0.04 (0.13) | 0.02 (0.05) | 0.06 (1.90) |
Intervention × Parental education | 0.23 (0.36) | −0.01 (0.14) | 0.00 (0.05) | 0.80 (1.99) |
Parental education × Gender | 0.59 (0.36) | 0.13 (0.14) | 0.00 (0.05) | 0.27 (1.99) |
Intervention × Parental education × Gender | 0.16 (0.36) | 0.06 (0.14) | −0.04 (0.05) | −0.59 (1.99) |
Associations between high-school STEM preparation and posthigh-school STEM career pursuit.
Next, we tested whether high-school STEM preparation variables were predictors of posthigh-school STEM career pursuit outcomes. Mathematics and science ACT score was a significant predictor of college STEM course-taking (z = 2.36, β = 0.26, P = 0.02), such that students with higher mathematics and science ACT scores enrolled in more college STEM classes. High-school mathematics and science course-taking was a significant predictor of students’ college STEM course-taking (z = 3.77, β = 0.34, P < 0.01), the likelihood that students’ desired a STEM major (z = 2.82, β = 0.29, P < 0.01), perceived STEM value (z = 2.29, β = 0.22, P = 0.02), and STEM career aspirations (z = 3.13, β = 0.30, P < 0.01). Thus, mathematics and science course-taking in high school was a significant predictor of all posthigh-school outcomes, such that students who enrolled in more high-school mathematics and science courses also enrolled in more college mathematics and science courses, were more likely to desire a STEM major, valued STEM topics more, and were more likely to aspire to a STEM career. Overall, these results suggest that high-school STEM preparation is implicated in posthigh-school STEM career pursuit. In this model, none of the base predictors showed significant direct effects on college outcomes, indicating that the intervention may affect posthigh-school outcomes indirectly.
Indirect effects of the intervention on posthigh-school STEM career pursuit through high-school STEM preparation.
Finally, we tested for indirect effects of the intervention (37, 38). We hypothesized that the intervention would increase posthigh-school STEM career pursuit to the degree that it increased high-school STEM preparation (i.e., mathematics and science ACT scores and 11th and 12th grade mathematics and science course-taking). We tested this hypothesis by examining the total indirect effect on each of the posthigh-school outcomes through the two high-school STEM preparation variables. That is, we examined how intervention-induced changes in high-school STEM preparation were associated with increased STEM career pursuit after high school. Results showed that there was a significant indirect effect of the intervention on all but one of the posthigh-school outcomes. Specifically, there were significant indirect effects of the intervention on college STEM course-taking (z = 2.54, P = 0.01), STEM career aspirations (z = 2.19, P = 0.03), and perceived STEM value (z = 2.15, P = 0.03). The total indirect effect on the likelihood of desiring a STEM major was not significant (z = 1.67, P = 0.09). These results suggest that the intervention can influence posthigh-school STEM career pursuit indirectly, through intervention effects on high-school STEM preparation outcomes (Fig. 3 for a summary path model of these effects). Because the link between high-school STEM preparation and posthigh-school STEM career pursuit variables is correlational, we cannot infer a causal effect of the intervention on these outcomes, although the longitudinal design strengthens the evidence. Rather, we believe that our findings highlight a pathway through which interventions may work by starting a positive recursive process in which success in high school begets success after high school in the STEM domain.
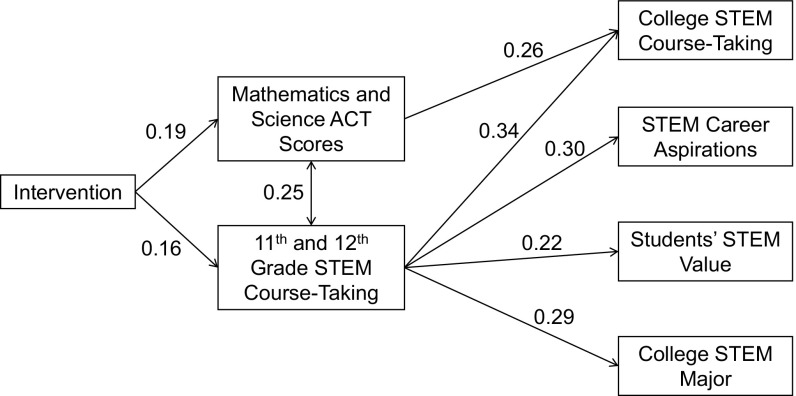
Primary empirical path model. The effect of the intervention on high-school outcomes (i.e., mathematics and science ACT scores and course-taking) as well as outcomes measured 5 y after the intervention, including college STEM course-taking, STEM career aspirations, STEM motivation, and college STEM major. The intervention affected 5-y outcomes through mathematics and science ACT scores and 11th/12th grade STEM course-taking. The only indirect effect that is not significant is from the intervention to college STEM major (z = 1.67, P = 0.09). All paths between subsequent variables were tested, but only significant paths are shown. Numbers represent standardized β-weights.
Discussion
Given the need to promote preparation and career pursuit in STEM domains in the United States (1–3), the goal of this project was to develop and test an intervention that engaged parents to encourage their children to take STEM courses in high school. We randomly assigned families to an intervention or control group, and intervention group parents received materials about the relevance of mathematics and science for their children and encouragement to discuss the relevance of these topics with their children during high school. Previous reports on this intervention found that it increased mathematics and science course-taking in high school (32). The current study followed up on those findings by examining the effect of the intervention on standardized test scores in high school as well as the long-term effects of increased high-school STEM preparation on STEM career pursuit after high school. We found that the intervention improved performance on ACT mathematics and science test scores by 12 percentile points. Consistent with a recursive process model whereby an initial improvement spurs on later positive outcomes (34), greater STEM preparation in high school was associated with increased STEM career pursuit after high school (i.e., STEM course-taking in college, STEM career aspirations, and perceived STEM value).
These results suggest that students’ motivation is one key factor that can be leveraged to enhance STEM competence and career pursuit. Recent research has shown that precise, theory-driven social-psychological interventions can have powerful and long-lasting effects on educational outcomes when applied directly with students in schools (16, 17, 39). This study contributes to that body of knowledge by showing that parents are an additional resource that can be engaged to promote important student outcomes. These results are also consistent with expectancy-value theory, which predicts that increasing the personal relevance of topics will increase motivation and achievement (13) and points to the important role of parents in helping children to develop their motivational beliefs (25–27). Prior research on parents’ utility-value beliefs and students’ outcomes is correlational, so this project shows that parents can play a causal role in improving students’ motivation by helping their children to find the utility and relevance in what they are learning.
The current study underscores the value of parents as communicators of STEM information to their children. Although parental involvement declines from elementary school to high school, it is still associated with academic success during the high-school years (40) and when it is the right type of involvement, such as emphasizing the personal relevance and importance of course material (41). However, little research has examined the efficacy of interventions with parents or focused on investigating if there is a positive effect of providing parents with resources to help promote optimal types of parental involvement. The current study does just this by providing parents with information and strategies to spur effective communication with their adolescents about STEM topics.
Interventions directed at parents, in comparison with those directed at students, have many advantages. First, the intervention can change parents’ behavior over the long term, so that pro-STEM messages are reinforced over time (e.g., with multiple conversations between parents and children). In contrast, student-centered classroom interventions are limited by the number of times that they are implemented in the classroom (17). Second, a parent-centered intervention can lead to a more customized implementation (e.g., by allowing parents to decide how to best use intervention materials with their child) and allow for one-on-one conversations, whereas teacher-led interventions often require less individualized communication, given the logistics of the classroom. For example, one parent might know that their child reacts well to direct conversations, whereas another parent might know that their child does not learn well from direct conversations and prefers to explore information on their own. Third, these interventions can capitalize on parents’ experiences and history with their children to facilitate personalizing the relevance of STEM topics by making connections to their children’s specific interests. Although these are strengths of parent-centered interventions, this does not guarantee that all parents will effectively communicate the information to their children. Moreover, other types of interventions (e.g., student-centered interventions) show great promise as well (17), and the most effective method to improve students’ educational outcomes likely involves careful intervention at multiple levels with students (e.g., parents, teachers, peers) to leverage all available resources.
Finally, although we found that this intervention was equally effective for both high and low socioeconomic status (SES) families in our sample, we note that the intervention effect on ACT performance was equivalent to about 50% of the SES gap on mathematics and science test score performance. Given the low enrollment rates of low SES groups in STEM courses (e.g., only 11.3% of low SES students enroll in precalculus or calculus compared with 39.6% of high SES students) (10) and general achievement gaps related to SES (42), parent-centered utility-value interventions might be one way to increase STEM course enrollment for underrepresented groups and reduce persistent achievement gaps. In addition, because STEM jobs are projected to grow in the future and often pay well (2, 3), interventions that increase representation of low SES groups in STEM fields might help to reduce persistent unemployment and income inequality gaps as well.
Although the current study showed initial evidence to support this type of parent-centered intervention in helping to address STEM pipeline problems, there are several limitations to this study that should be addressed in future research. First, the sample in this study was recruited in Wisconsin and may not be representative of the overall population in the United States. Other studies have shown that utility-value interventions show similar effects for individuals from diverse racial and SES groups (20, 21), and two-generation interventions have been shown to be effective even in very low SES populations (43), but future studies are needed to test this type of parent-centered utility-value intervention in more diverse populations to show that the effects generalize to various racial and SES groups. Second, the families in this study had been part of an ongoing longitudinal study for many years, which might have increased the level of engagement that parents had with intervention materials. This means that parents in the current study might have been more likely to use intervention materials than a newly recruited group of parents would be because the parents in this study were accustomed to participating in study activities. Future studies should take this into account and ensure that parents engage with intervention materials at high enough rates to see similar gains in students’ outcomes. Third, although this study involved 181 families and 543 total participants, including parents and children in all of the families, future studies should examine these effects in larger samples to demonstrate the scalability of these results. One possibility would involve working directly with school districts to engage parents in these activities with their children.
Finally, although we found a direct effect of the intervention on two indicators of STEM preparation (ACT scores and STEM course-taking), we did not find direct effects on posthigh-school STEM career pursuit. Instead, we found an indirect pathway: the intervention improved high-school STEM preparation, which predicted subsequent STEM career pursuit. One limitation of such indirect effects is that they are correlational; thus, a third variable could be responsible for this lasting effect instead of the intervention. Nevertheless, an indirect effect is consistent with our contention that the intervention had lasting effects on important STEM outcomes. An indirect effects analysis is powerful because it moves further down the causal chain by testing the more proximal effect of an initial mechanism (e.g., high-school STEM preparation) on the distal outcome (e.g., STEM career pursuit) (34, 37, 38, 44).
In conclusion, based on the findings from the current and previous utility-value intervention studies (16, 39), there is mounting evidence that promoting the relevance of STEM topics for students is an effective method for increasing students’ STEM achievement, career pursuit, and motivation. In addition, these interventions are cost-effective to implement; for example, the parent-centered utility value intervention was comprised of two informational brochures and access to a website. However, recent recommendations from the US Congress and the Department of Education make no mention of this psychological perspective as a way to grow STEM education and alleviate the “pipeline problem” (45). Instead, recommendations center on increasing the budget for STEM classes and resources from kindergarten through 12th grade. Although increasing STEM-education funding will likely increase overall STEM preparation, the body of research on STEM motivation in general—and on utility-value interventions in particular—highlights the importance of targeting students’ psychological beliefs about STEM topics as one pathway to promote further STEM engagement. Overall, findings from the current study emphasize the value of taking a psychological approach to the present STEM pipeline problem.
Materials and Methods
Participants.
The participating families were originally recruited from the state of Wisconsin in 1990–1991 when mothers were pregnant with their children as part of a longitudinal study with various aims over the years (SI Materials and Methods for additional information). The current sample included 181 families (543 participants including parents and children) with students who attended 108 different high schools, mostly remaining in the state of Wisconsin. Participating students and parents provided written informed assent and consent approved by the Institutional Review Board at the University of Wisconsin–Madison. There were 100 students (47% female) in the control group, and 81 students (48% female) in the intervention group, and the racial breakdown of participants reflected the population of Wisconsin in general (90% White) (46).
Procedure.
The current study involved parents and students for a time period of 5 y, starting when the students were in 10th grade in high school. Variables of interest included students’ 10th grade standardized state test scores (WKCE scores), students’ STEM course-taking during 11th and 12th grade of high school, students’ mathematics and science ACT scores, and students’ survey responses 5 y after the beginning of 10th grade. The posthigh-school survey assessed four self-reported outcome measures: STEM career aspirations, college STEM course-taking, desired college major, and perceived STEM value. Families were randomly assigned to the intervention or control group, and intervention group parents were sent intervention materials during 10th and 11th grade, whereas control group parents were sent no materials. Specifically, parents in the intervention group received a brochure in the fall of 10th grade, another brochure in January of 11th grade, and access to a website in January of 11th grade. The brochures and websites gave information about the utility of various STEM topics for adolescents, encouragement to communicate this information, and advice about how parents might most effectively communicate this information to their children. A previous report showed that 86% of the parents (either mother, father, or both) reported remembering and using the intervention materials, and 75% of the adolescents confirmed exposure to the intervention materials from their parents, demonstrating a high degree of overall engagement with the intervention (32). SI Materials and Methods for further details on intervention materials and measures.
SI Materials and Methods
Participants.
The families who participated in this randomized controlled trial (32) have also participated in a larger, longitudinal study since 1990–1991 (Wisconsin Study of Families and Work; for recruitment details, see ref. 47). Median household income at the time of recruitment was $50,000; in 1991, the median income of married couple families in the United States with the wife in the paid labor force was $48,169 (48). Therefore, our sample matches the national figure well. Average years of parents’ education was 15.42 y (SD = 1.92) on a scale where 12 y was equivalent to high-school graduation or GED completion. The racial breakdown of the students reflected the state in which the sample was recruited: 90% were White (not of Hispanic origin), 2% were African American, 1% were Native American, and 7% were biracial or multiracial (46).
The original sample for the current study had 188 families in 108 different high schools, but seven students and their parents were dropped from analyses because we did not receive a high-school transcript for them. This lack of transcript occurred for a variety of reasons, including a lack of consent and homeschooling (32). Collection of the transcript did not vary by condition or gender.
Intervention Materials.
The intervention consisted of sending intervention group parents two brochures in the mail and also giving them access to a password-protected website. The first set of intervention materials consisted of a single brochure entitled, “Making Connections: Helping Your Teen Find Value in School,” sent to both parents when the students were in 10th grade. Because prior research had shown that many parents did not know why common topics in mathematics and science could be useful or relevant (30), this brochure contained information and examples about the usefulness of STEM topics in their child’s life (e.g., calculating sales prices when shopping, mathematics courses in high school as necessary for a variety of college majors). It also encouraged parents to discuss these topics with their child and provided them with strategies and advice about how to communicate this information most effectively with their child. The advice centered on involving children in generating personally relevant examples.
A second set of intervention materials was sent in 11th grade to both parents individually and included a second brochure entitled, “Making Connections: Helping Your Teen with the Choices Ahead,” as well as access to a password-protected website entitled “Choices Ahead.” This brochure gave additional examples of how STEM topics were relevant in everyday life and also included a greater focus on how STEM topics were important for a variety of careers and majors in college. There were also additional tips and encouragement given to parents about the ways in which they could communicate this information effectively to their child. More specifically, there were three overarching sections in this brochure: (i) information on specific occupations in mathematics, physics, biology, and chemistry, as well as how individuals in these fields used mathematics and science in their everyday activities; (ii) separate sections on the relevance of STEM topics for everyday activities, adult life more generally, and college and career planning in particular; and (iii) information on ways to overcome challenges in communicating this information to the child (e.g., parents were asked to first find connections with mathematics and science in their own lives and then help their child discover examples specific to their lives).
The website contained extensive information related to the relevance of mathematics and science for college and career planning. It also offered parents the opportunity to send specific links from the website to their students via email. The information on the website was broken up into four sections. The precollege planning section included specific pages for both the parent and student, and each page provided links and information on websites where students could find more career and college planning information (e.g., a link to a website that focused on finding the right college, a link to a website that allowed one to search a database of potential careers). The next section included information on the preparation needed to obtain a career in biology, chemistry, physics, and mathematics, with more clickable links specific to those fields. The third section provided parents and students with career information on four career domains in particular: biology and chemistry, healthcare (medicine, nursing, and veterinary), physics and engineering, and mathematics and architecture. Finally, the last section included more information on mathematics and science in one’s everyday life as well as testimonials from students at University of Wisconsin–Madison detailing how their mathematics and science preparation in high school has been important for their college trajectories (e.g., “Although I did not particularly care for math or science in high school, once I got to college, I was so glad that I opted to take advanced level courses in each. I didn’t realize at the time how much a strong background in math and science would help me with the required courses for my major, like zoology and statistics. Math and science classes weren’t the most interesting to me, but they very well may have been the most useful, and I probably would have struggled through many college courses had I decided only to take the minimum level of math and science required for graduation.”).
Measures.
Student’s’ transcripts were collected after graduation, as previously reported (32), and we also collected students’ WKCE and ACT scores from school records for the current study. Another set of measures was collected through a survey given during the summer 2 y after students’ senior years of high school. The proportion of missing data for the variables described below did not significantly differ by experimental condition.
11th and 12th grade STEM courses-taking.
High-school transcripts were collected (control: male n = 53, female n = 47; intervention: male n = 42, female n = 39) and coded for number of semester of mathematics and science taken in 11th and 12th grade (mean = 7.85; SD = 2.57; response rate: 100%).
Mathematics and science ACT score.
ACT scores were collected through official high-school records from the students, and the mathematics and science ACT scores were averaged to create a composite mathematics and science ACT score measure (mean = 24.55; SD = 4.39; response rate: 71%).
College STEM course-taking.
Mathematics and science college course-taking was assessed by asking students 2 y after their senior year of high school how many science and mathematics courses they had taken so far in college. A composite measure of college STEM course-taking was created by summing the two variables (mean = 5.86; SD = 3.87; response rate: 68%).
STEM career aspirations.
Two years after students’ senior year of high school, students responded to an open-ended question asking what career they envisioned themselves having at age 30. Responses to this open-ended question were matched to careers using the O*NET OnLine career coding system, which is sponsored by the US Department of Labor, Employment, and Training. The O*NET OnLine website (www.onetcenter.org/overview.html) offers scores, referred to as knowledge values, for mathematics (mean = 55.25; SD = 18.37), physics (mean = 23.00; SD = 21.52), chemistry (mean = 25.33; SD = 22.16), biology (mean = 31.97; SD = 29.93), and engineering and technology (mean = 27.72; SD = 24.23) on a scale from 0 to 100 for each career. A higher score on a knowledge value indicates that a higher level of knowledge in that topic is needed for that career. For example, a career as a civil engineer would have higher scores on the five knowledge values compared with a career as a lawyer. Once careers were matched to a career on the O*NET online website, the highest of five knowledge values was used for each student to create the STEM career aspirations measure (mean = 61.70; SD = 20.90; response rate: 65%).
College STEM major.
Two years after students’ senior year of high school, students were also asked to report their major using an open-ended question. The majors were coded as a STEM major or a non-STEM major using two independent observers, and disagreements were resolved by an independent third coder (40.17% STEM majors; response rate: 65%).
Perceived STEM value.
Students’ STEM value (M = 5.50; SD = 1.50) was assessed 2 y after students’ senior year of high school with three items measured on a 1 (strongly disagree) to 7 (strongly agree) scale that asked about the students’ perceptions of the importance of mathematics and science in general, as well as in their future career and classes (“Math and science are important for my future”; “I will use math and science in my job or career”; “I want to take more math and science classes in the future.” α = 0.91; response rate: 73%).
Perceived parental STEM support.
Perceived parental STEM support (mean = 5.93; SD = 1.01) 2 y after students’ senior year of high school was assessed using three items measured on a 1 (strongly disagree) and 7 (strongly agree) scale that asked about how much students’ parents valued and encouraged mathematics and science topics and courses (“My parents think math and science are important for my life”; “My parents encouraged me to take math and science courses”; “My parents have encouraged me to do well in my math and science courses.” α =0.84; response rate: 73%). This variable was used to assess if students in our intervention group viewed their parents as being more supportive of their STEM career pursuit than students in the control condition. Because this was not a primary student variable of interest, we only reported analyses on this variable in SI Results.
Parents’ education level.
Both parents reported on the number of years of education they received. Twelve years was equivalent to a high-school graduate, 16 y was equivalent to a 4-y college graduate, and greater than 16 y indicates some amount of graduate school. Mothers (mean = 15.42; SD = 2.10) and fathers (mean = 15.42; SD = 2.41) reported generally similar levels of education on average. A variable of parents’ average years of education was created by averaging the two variables (mean = 15.42; SD = 1.92; response rate: 100%).
Preintervention standardized test scores.
We collected Wisconsin 10th grade state mathematics and science standardized test scores (WKCE scores) from students’ high-school records to have a preintervention measure of students’ performance. The test was given in the fall of 10th grade, before the intervention. Students who attended private schools or out-of-state schools did not take Wisconsin state tests. A composite of students’ WKCE mathematics and science test scores (mean = 533.74; SD = 36.10; response rate: 60%) was created by averaging across their mathematics and science subscale scores. As reported in the main text, there was not a significant difference between intervention and control group students on WKCE mathematics and science test scores.
SI Results
Variance Explained in Main Structural Equation Model.
The main model of interest in the paper (all predictors included and allowed to predict all subsequent variables in the model) accounted for 9.1% of the variance for 11th and 12th grade mathematics and science course-taking, 25.0% of mathematics and science ACT score, 9.0% of students’ college STEM value, 31.9% of college STEM course-taking, 13.5% of STEM career aspirations, and 11.3% of college STEM major choice.
Regression Analyses on ACT Scores.
We conducted two additional regressions to address concerns about randomization. In the first regression, we tested whether the intervention affected mathematics and science ACT score, as we do in the main model in the paper, which involved regressing mathematics and science ACT score on the seven base predictors (intervention, students’ gender, parents’ education level, and the interactions between those variables). In the next regression, we ran the same model on the same dependent variable, but we included students’ preintervention mathematics and science test score measure in the analysis, which controlled for preintervention competence. When controlling for prior performance, there was a significant effect of the intervention on mathematics and science ACT score (z = 3.61, β = 0.20, P < 0.001), consistent with the analysis without controlling for prior performance (z = 2.45, β = 0.19, P < 0.05). Full results from these two regressions are reported in Table S3. From these analyses, the intervention has a consistent and significant positive effect on students’ mathematics and science ACT scores.
Supplemental Structural Equation Model, Including Perceived Parental STEM Support.
For this analysis, we estimated an identical model to the model described in the main text, except that we also included perceived parental STEM support as a posthigh-school outcome along with perceived STEM value, college STEM course-taking, STEM career aspirations, and college STEM major. The purpose of this analysis was to examine whether the intervention had a long-term effect on students’ perceptions of the amount of encouragement and support they had from their parents to pursue STEM careers, even years after the intervention. Brief, theory-based interventions are hypothesized to work via recursive processes, whereby a change in attitude helps to change behavior, which in turn supports the initial change in attitude (34). Here, we are able to test for part of this recursive process by examining the long-term effect of the intervention on students’ perceptions of how supportive and encouraging their parents are about STEM. Given the important roles that parents’ perceived attitudes have in students’ motivation (27, 33), it is useful to know if this parent-delivered intervention affected students’ long-term perceptions of their parents’ attitudes in addition to their own STEM attitudes and behaviors. Overall, although this was not a primary dependent variable of interest, it does speak to how a parent-centered intervention might have lasting effects on how students feel supported by their parents in STEM domains long after the initial interactions that were encouraged by the intervention.
As with the primary model, this model is saturated, so there are not interpretable indices of model fit. All significant effects from the model reported in the main text remained significant in this supplemental model. New tests showed that mathematics and science ACT score was a significant predictor of perceived parental STEM support (z = 3.47, β = 0.38, P < 0.001), such that students with higher mathematics and science ACT scores reported greater levels of perceived parental STEM support. Additionally, there was a significant indirect effect of the intervention on perceived parental STEM support through high-school STEM course-taking and ACT scores (z = 2.13, P < 0.05). In all, the model explained 16.4% of the variance in perceived parental STEM support. Thus, this finding supports the recursive process theory of psychological interventions by demonstrating that the intervention affected perceptions of parental STEM support 5 y after the intervention through effects on high-school STEM course-taking and ACT scores.
Acknowledgments
We thank Wisconsin Study of Families and Work research assistants for their help in conducting this research; Sian Beilock for helpful comments; members of our advisory board (Jacque Eccles, Adam Gamoran, Jo Handelsman, Jenefer Husman, Dominic Johann-Berkel, Ann Renninger, and Judith Smetana) for their guidance; and the families of the Wisconsin Study of Families and Work project for their participation over the years. This research was supported by National Science Foundation Grant Division of Research On Learning 0814750; and by the Institute for Education Sciences, US Department of Education, through Award R305B090009 and Grant 144-NL14 to the University of Wisconsin–Madison and through Award R305B140042 to Northwestern University. The opinions expressed are those of the authors and do not represent views of the National Science Foundation or the US Department of Education.
Footnotes
The authors declare no conflict of interest.
This article is a PNAS Direct Submission.
This article contains supporting information online at www.pnas.org/lookup/suppl/10.1073/pnas.1607386114/-/DCSupplemental.
References
Articles from Proceedings of the National Academy of Sciences of the United States of America are provided here courtesy of National Academy of Sciences
Full text links
Read article at publisher's site: https://doi.org/10.1073/pnas.1607386114
Read article for free, from open access legal sources, via Unpaywall:
https://www.pnas.org/content/pnas/114/5/909.full.pdf
Citations & impact
Impact metrics
Article citations
Editorial: Informal STEM learning at home and in community spaces.
Front Psychol, 15:1383075, 13 Mar 2024
Cited by: 0 articles | PMID: 38544519 | PMCID: PMC10965790
Operationalizing competency-based assessment: Contextualizing for cultural and gender divides.
MedEdPublish (2016), 13:210, 11 Oct 2023
Cited by: 0 articles | PMID: 37842229 | PMCID: PMC10576182
Sensitivity to intrinsic rewards is domain general and related to mental health.
Nat Ment Health, 1(9):679-691, 06 Sep 2023
Cited by: 1 article | PMID: 38665692 | PMCID: PMC11041740
Field-specific cultural capital and persistence in college majors.
Soc Sci Res, 103:102654, 24 Oct 2021
Cited by: 2 articles | PMID: 35183311 | PMCID: PMC8861445
Science Interest, Utility, Self-Efficacy, Identity, and Science Achievement Among High School Students: An Application of SEM Tree.
Front Psychol, 12:634120, 09 Sep 2021
Cited by: 1 article | PMID: 34566743 | PMCID: PMC8458621
Go to all (19) article citations
Similar Articles
To arrive at the top five similar articles we use a word-weighted algorithm to compare words from the Title and Abstract of each citation.
Helping parents to motivate adolescents in mathematics and science: an experimental test of a utility-value intervention.
Psychol Sci, 23(8):899-906, 03 Jul 2012
Cited by: 47 articles | PMID: 22760887
Effect of providing gender equality information on students' motivations to choose STEM.
PLoS One, 16(6):e0252710, 23 Jun 2021
Cited by: 0 articles | PMID: 34161349 | PMCID: PMC8221466
Pathways to college and STEM careers: enhancing the high school experience.
New Dir Youth Dev, 2013(140):9-29, 01 Jan 2013
Cited by: 6 articles | PMID: 24474256 | PMCID: PMC4135439
How Cardiac Anesthesiology Can Help "STEM" the Tide of Under-representation of Minorities in Science and Medicine.
J Cardiothorac Vasc Anesth, 32(2):631-635, 20 Jun 2017
Cited by: 0 articles | PMID: 29366746
Review
Funding
Funders who supported this work.
DoED | Institute of Education Sciences (2)
Grant ID: R305B140042
Grant ID: R305B090009
National Science Foundation (1)
Grant ID: 0814750