Abstract
Background
In patients who have had type 1 diabetes for 5 years, current recommendations regarding screening for diabetic retinopathy include annual dilated retinal examinations to detect proliferative retinopathy or clinically significant macular edema, both of which require timely intervention to preserve vision. During 30 years of the Diabetes Control and Complications Trial (DCCT) and its longitudinal follow-up Epidemiology of Diabetes Interventions and Complications (EDIC) study, retinal photography was performed at intervals of 6 months to 4 years.Methods
We used retinal photographs from the DCCT/EDIC study to develop a rational screening frequency for retinopathy. Markov modeling was used to determine the likelihood of progression to proliferative diabetic retinopathy or clinically significant macular edema in patients with various initial retinopathy levels (no retinopathy or mild, moderate, or severe nonproliferative diabetic retinopathy). The models included recognized risk factors for progression of retinopathy.Results
Overall, the probability of progression to proliferative diabetic retinopathy or clinically significant macular edema was limited to approximately 5% between retinal screening examinations at 4 years among patients who had no retinopathy, 3 years among those with mild retinopathy, 6 months among those with moderate retinopathy, and 3 months among those with severe nonproliferative diabetic retinopathy. The risk of progression was also closely related to mean glycated hemoglobin levels. The risk of progression from no retinopathy to proliferative diabetic retinopathy or clinically significant macular edema was 1.0% over 5 years among patients with a glycated hemoglobin level of 6%, as compared with 4.3% over 3 years among patients with a glycated hemoglobin level of 10%. Over a 20-year period, the frequency of eye examinations was 58% lower with our practical, evidence-based schedule than with routine annual examinations, which resulted in substantial cost savings.Conclusions
Our model for establishing an individualized schedule for retinopathy screening on the basis of the patient's current state of retinopathy and glycated hemoglobin level reduced the frequency of eye examinations without delaying the diagnosis of clinically significant disease. (Funded by the National Institute of Diabetes and Digestive and Kidney Diseases and others; DCCT/EDIC ClinicalTrials.gov numbers, NCT00360893 and NCT00360815 .).Free full text

Frequency of Evidence-Based Screening for Retinopathy in Type 1 Diabetes
Abstract
BACKGROUND
In patients who have had type 1 diabetes for 5 years, current recommendations regarding screening for diabetic retinopathy include annual dilated retinal examinations to detect proliferative retinopathy or clinically significant macular edema, both of which require timely intervention to preserve vision. During 30 years of the Diabetes Control and Complications Trial (DCCT) and its longitudinal follow-up Epidemiology of Diabetes Interventions and Complications (EDIC) study, retinal photography was performed at intervals of 6 months to 4 years.
METHODS
We used retinal photographs from the DCCT/EDIC study to develop a rational screening frequency for retinopathy. Markov modeling was used to determine the likelihood of progression to proliferative diabetic retinopathy or clinically significant macular edema in patients with various initial retinopathy levels (no retinopathy or mild, moderate, or severe nonproliferative diabetic retinopathy). The models included recognized risk factors for progression of retinopathy.
RESULTS
Overall, the probability of progression to proliferative diabetic retinopathy or clinically significant macular edema was limited to approximately 5% between retinal screening examinations at 4 years among patients who had no retinopathy, 3 years among those with mild retinopathy, 6 months among those with moderate retinopathy, and 3 months among those with severe nonproliferative diabetic retinopathy. The risk of progression was also closely related to mean glycated hemoglobin levels. The risk of progression from no retinopathy to proliferative diabetic retinopathy or clinically significant macular edema was 1.0% over 5 years among patients with a glycated hemoglobin level of 6%, as compared with 4.3% over 3 years among patients with a glycated hemoglobin level of 10%. Over a 20-year period, the frequency of eye examinations was 58% lower with our practical, evidence-based schedule than with routine annual examinations, which resulted in substantial cost savings.
CONCLUSIONS
Our model for establishing an individualized schedule for retinopathy screening on the basis of the patient’s current state of retinopathy and glycated hemoglobin level reduced the frequency of eye examinations without delaying the diagnosis of clinically significant disease.
Diabetic retinopathy is the most common cause of blindness in adults in the United States.1 Fortunately, the risk of the development and progression of retinopathy can be reduced substantially by modern-day intensive glycemic management.2–5 Moreover, if clinically significant macular edema or vision-threatening proliferative diabetic retinopathy develops, timely intervention with laser photocoagulation or with intraocular glucocorticoids or anti–vascular endothelial growth factor (VEGF) agents can substantially reduce loss of vision.6–9 Thus, the goal of retinopathy screening is the timely detection of retinopathy that would, without intervention, cause vision loss.
In patients with type 1 diabetes, annual screening for retinopathy starting 3 to 5 years after diagnosis has long been recommended,10–12 largely on the basis of now-outdated epidemiologic studies. However, the long-term benefits of intensive therapy on the clinical course of retinopathy are now well established,13 and recommendations for screening are being reevaluated.
The Diabetes Control and Complications Trial (DCCT), which was conducted from 1983 through 1993, and the follow-up Epidemiology of Diabetes Interventions and Complications (EDIC) study (which has been ongoing since 1994) have followed a cohort of patients with type 1 diabetes with regularly scheduled fundus photography for more than 30 years.13 Nearly complete follow-up with strong adherence to the fundus photography schedule and systematic assessment of risk factors for retinopathy have provided the opportunity to formulate rational, data-driven strategies to screen for retinopathy.
Our goal was to establish an evidence-based fundus photography screening schedule that would efficiently detect potentially vision-threatening changes. In addition, we evaluated individualization of the screening frequency on the basis of the effects of established risk factors.
METHODS
The DCCT and EDIC studies were designed by their respective research groups. The methods of both studies have been described previously.2,3,14 The data were collected at the Biostatistics Center of George Washington University. The second and last authors performed the statistical analyses. The first, second, and last authors wrote the first draft of the manuscript, and the members of the writing committee provided additional text and revisions. The members of the writing committee vouch for the accuracy and completeness of the data and analyses and for the fidelity of the study to the protocols (available with the full text of this article at NEJM.org). The DCCT/EDIC Research Group made the decision to submit the manuscript for publication.
The institutional review boards at each participating center approved both study protocols. All the patients provided written informed consent.
PATIENTS
From 1983 through 1989, the DCCT enrolled 1441 patients with type 1 diabetes who were 13 to 39 years of age. The primary prevention cohort (726 patients) had diabetes for 1 to 5 years and no retinopathy detected by means of stereoscopic fundus photography at baseline. The secondary intervention cohort (715 patients) had diabetes for 1 to 15 years and very mild to moderate nonproliferative diabetic retinopathy.
After the DCCT ended in 1993, a total of 1375 patients (95% of the cohort) joined the observational EDIC follow-up study. Here, we present data on fundus photography obtained from 1983 through 2012, with a maximum of 28.7 years of follow-up (mean, 23.5 years), including 95% of the survivors.
INTERVENTIONS
During the DCCT, 711 patients were randomly assigned to receive intensive therapy aimed at lowering glycemia as close to the nondiabetic range as safely possible, and 730 patients were randomly assigned to conventional therapy aimed at preventing symptoms of hyperglycemia and hypoglycemia with no specific glucose targets.2 At the end of the trial, after an average of 6.5 years of treatment, all the patients received instruction regarding intensive therapy and were referred to their health care providers for diabetes care. The EDIC follow-up study evaluated patients annually.
RETINOPATHY AND VISUAL ACUITY
Standardized stereoscopic seven-field fundus photographs were obtained every 6 months during the DCCT and every fourth year during the EDIC study (see the Supplementary Appendix, available at NEJM.org). In addition, photographs were obtained in the complete cohort during years 4 and 10 of the EDIC study.
Photographs were graded centrally with the use of the final Early Treatment Diabetic Retinopathy Study (ETDRS) grading scale, which assigns a combined grade to each patient that is based on the severity of retinopathy in both eyes.15 Graders were unaware of the treatment assignments and glycated hemoglobin levels of the patients.
BIOMEDICAL EVALUATIONS
Blood pressure and glycated hemoglobin levels were measured quarterly during the DCCT and annually thereafter.14,16 The albumin excretion rate and plasma lipid concentrations were measured yearly during the DCCT and in alternate years thereafter. Time-dependent covariates included the time-weighted mean glycated hemoglobin level, weighted by 0.25 for quarterly DCCT values and by 1 for annual EDIC values, current smoking status, hypertension and hyperlipidemia status, current age, duration of diabetes, and body-mass index (BMI).
STATISTICAL ANALYSIS
A longitudinal Markov model,17 which allowed for uneven visit intervals among patients, was used to estimate the cumulative incidence of transitions among five mutually exclusive retinopathy states that were based on ETDRS grades. State 1 corresponded to no retinopathy; state 2, mild nonproliferative diabetic retinopathy, including microaneurysms only; state 3, moderate nonproliferative diabetic retinopathy; and state 4, severe nonproliferative diabetic retinopathy. State 5 corresponded to any of the following: proliferative diabetic retinopathy, clinically significant macular edema, or previous self-reported treatment with panretinal or focal photocoagulation, intraocular glucocorticoids, or anti-VEGF agents. We were principally concerned with determining a retinopathy screening schedule that would limit the risk of progression from lesser states to state 5 during the interval up to the next scheduled visit, at which point progression could be detected by retinal examination and interventions could be recommended to preserve vision.
Among 23,961 retinopathy examinations over various intervals (see Section 2 in the Supplementary Appendix), 14.5% showed worsening from the level at the previous visit, 7.8% showed improvement, and 77.7% showed no change (see Table S1 in the Supplementary Appendix). Only 2.2% of all transitions were two or more steps (worsening or improvement) from the state at the previous visit. Thus, the model only allowed for worsening from states 1 to 2, 2 to 3, 3 to 4, 3 to 5, and 4 to 5 and for improvement from states 2 to 1, 3 to 2, and 4 to 3.
The maximum duration of diabetes was 43 years, with a mean of 29.3 years, at the final retinal assessment. Exponential event-time regression models for each transition provided estimated probabilities for each retinopathy state at a point in time, transition probabilities between states over an interval of time, cumulative-incidence functions over time, and hazard ratios for covariate effects on the risks of transitions. An individualized screening schedule was determined on the basis of each patient’s current retinal status and mean glycated hemoglobin level (the major risk factors identified), so that the risk of the development of state 5 retinopathy before the next examination was low (e.g., approximately 5%).
Two measures were used to assess effects on cost (see the Supplementary Appendix). Undetected time was the elapsed time from the onset of state 5 retinopathy to the next examination at which it would be detected and treated. The number of prior visits was the number of negative examinations (at states 1 through 4) before state 5 retinopathy was detected. More frequent examinations were associated with a shorter period before the detection of state 5 retinopathy but a greater number of negative examinations.
Computations were performed with the use of the msm package of R software.18 The Model Fit Assessment in the Supplementary Appendix provides sensitivity analyses showing the validity of the core Markov model. A companion statistical article describes the computation of the cost measures used here.19
RESULTS
MARKOV INCIDENCE MODELS
On the basis of the probabilities of transitions from state to state (Tables S2 and S3 in the Supplementary Appendix), we computed the probabilities of transitions from lower levels of retinopathy (states 1 through 4) to state 5 retinopathy with screening intervals of 1 month, 2 months, 3 months, 6 months, 9 months, and 1 to 5 years (Table 1). Figure 1 shows the corresponding cumulative-incidence functions of transitions from states 1 through 4 to state 5. On average, patients remained in state 2 for 4.1 years (the longest period) before transitioning to a different state, and they remained in state 4 for 0.39 years (the shortest period).
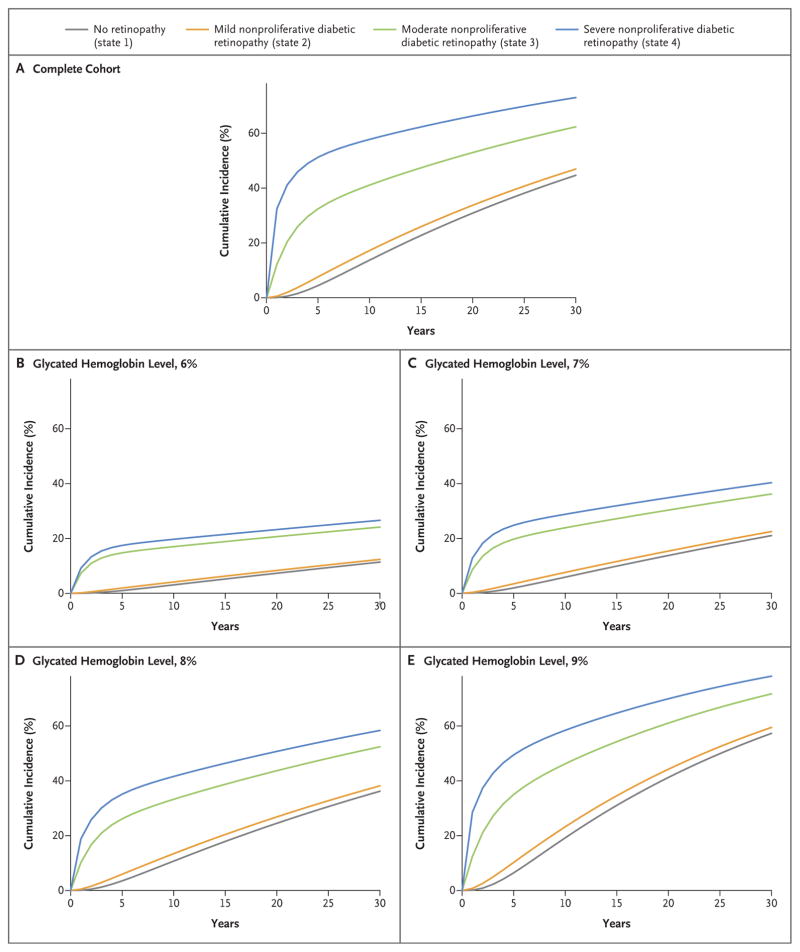
Cumulative Incidence of State 5 Retinopathy (Proliferative Diabetic Retinopathy or Clinically Significant Macular Edema).
Table 1
Probability over a Given Follow-Up Interval of Progression from Lower Levels of Retinopathy (States 1 through 4) to State 5 Retinopathy (Proliferative Diabetic Retinopathy or Clinically Significant Macular Edema), Stratified According to the Initial State.*
Interval of Follow-Up | State 1 to State 5 | State 2 to State 5 | State 3 to State 5 | State 4 to State 5 |
---|---|---|---|---|
percent (95% CI) | ||||
1 mo | 0 | 0 | 1.1 (0–1.3) | 5.7 (3.6–8.8) |
| ||||
2 mo | 0 | 0.02 (0.016–0.021) | 2.3 (2.0–2.6) | 10.4 (6.5–16.0) |
| ||||
3 mo | 0 | 0.04 (0.036–0.047) | 3.4 (3.1–3.8) | 14.4 (9.4–22.0) |
| ||||
6 mo | 0.01 (0.01–0.02) | 0.16 (0.14–0.18) | 6.6 (6.0–7.3) | 23.0 (15.8–32.7) |
| ||||
9 mo | 0.04 (0.04–0.05) | 0.35 (0.31–0.39) | 9.6 (8.8–10.5) | 28.6 (20.9–38.4) |
| ||||
1 yr | 0.10 (0.09–0.11) | 0.59 (0.53–0.67) | 12.3 (11.3–13.5) | 32.5 (23.8–44.2) |
| ||||
2 yr | 0.59 (0.53–0.67) | 2.0 (1.8–2.2) | 20.5 (18.9–22.3) | 41.2 (32.6–50.6) |
| ||||
3 yr | 1.6 (1.4–1.7) | 3.7 (3.4–4.1) | 25.9 (23.9–28.2) | 45.9 (38.2–55.7) |
| ||||
4 yr | 2.9 (2.6–3.2) | 5.7 (5.2–6.2) | 29.7 (27.6–32.2) | 49.0 (42.0–58.0) |
| ||||
5 yr | 4.5 (4.1–5.0) | 7.6 (7.0–8.4) | 32.5 (30.2–35.3) | 51.3 (44.6–60.8) |
These cumulative-incidence functions provided the estimated probabilities of progression from intermediate states 1 through 4 to state 5 retinopathy over increasing screening intervals. Thus, an interval could be chosen with the goal of limiting this probability to approximately 5%. For example, patients in state 1 had only a 2.9% chance of progression to state 5 during the next 4 years (Table 1), and those in state 2 had only a 3.7% chance of doing so by 3 years. The probabilities of progression to state 5 were 6.6% over 6 months in patients with state 3 and 14.4% over 3 months in patients with state 4. In patients with state 4 retinopathy, monitoring that was more frequent than quarterly would be necessary to reduce this probability to below 14.4%. This suggests that a practical schedule could use screening intervals of 4 years, 3 years, 6 months, and 3 months for the four retinopathy states, respectively.
COVARIATE EFFECTS AND PATIENT-SPECIFIC RISKS
Higher mean glycated hemoglobin values were associated with a significantly increased risk of worsening retinopathy and a lower chance of improving retinopathy (Table 2). For example, each increase of 1 percentage point in the mean glycated hemoglobin level was associated with a 15.4% higher risk of progression from state 1 to state 2 (hazard ratio, 1.15) and a 12.4% lower chance of regression from state 2 to state 1. Figure 1 shows the cumulative incidence of state 5 retinopathy stratified according to mean glycated hemoglobin levels.
Table 2
Risk of Transition to a More or Less Severe State of Retinopathy as a Function of a Unit (1 Percentage Point) Increase in the Weighted Mean Glycated Hemoglobin Level.*
Transition | Hazard Ratio for Transition (95% CI) |
---|---|
Worsening retinopathy | |
State 1 to state 2: no retinopathy to mild nonproliferative diabetic retinopathy | 1.15 (1.12–1.19) |
State 2 to state 3: mild nonproliferative diabetic retinopathy to moderate nonproliferative diabetic retinopathy | 1.38 (1.32–1.44) |
State 3 to state 4: moderate nonproliferative diabetic retinopathy to severe nonproliferative diabetic retinopathy | 1.47 (1.18–1.82) |
State 3 to state 5: moderate nonproliferative diabetic retinopathy to proliferative diabetic retinopathy or clinically significant macular edema | 1.10 (0.97–1.25) |
State 4 to state 5: severe nonproliferative diabetic retinopathy to proliferative diabetic retinopathy or clinically significant macular edema | 1.60 (1.15–2.23) |
Improving retinopathy | |
State 2 to state 1: mild nonproliferative diabetic retinopathy to no diabetic retinopathy | 0.88 (0.83–0.92) |
State 3 to state 2: moderate nonproliferative diabetic retinopathy to mild nonproliferative diabetic retinopathy | 0.79 (0.73–0.85) |
State 4 to state 3: severe nonproliferative diabetic retinopathy to moderate nonproliferative diabetic retinopathy | 0.77 (0.55–1.08) |
Age, sex, duration of diabetes, current smoking status, BMI, hypertension, hyperlipidemia, and treatment group had some significant unadjusted associations with possible transitions (Table S4 in the Supplementary Appendix). Table S5 in the Supplementary Appendix shows the cumulative-incidence functions within covariate subgroups. However, only the glycated hemoglobin level had a substantive effect (Table 2).
Table 3 shows the potential screening schedule and the resulting cumulative probabilities of the development of state 5 retinopathy within subgroups of patients according to the cumulative-incidence functions within subgroups shown in Table S5 in the Supplementary Appendix. For example, in patients with a mean glycated hemoglobin value of 6%, the probability of progression from state 1 to state 5 retinopathy was 1.0% within 5 years, the probability of progression from state 2 was 1.9% within 5 years, the probability of progression from state 3 was 4.3% in 6 months, and the probability of progression from state 4 was 3.3% in 3 months. For patients with a glycated hemoglobin level of 6%, this translates into scheduled examinations after 5 years for those with state 1 retinopathy, 5 years for those with state 2, 6 months for those with state 3, and 3 months for those with state 4. Conversely, in patients with a mean glycated hemoglobin level of 10%, scheduled screening at 3 years, 2 years, 3 months, and 1 month for states 1 through 4, respectively, might be used with probabilities of state 5 retinopathy of 4.3%, 4.5%, 4.0%, and 7.8%, respectively.
Table 3
Screening Schedule and the Corresponding Probability of Progression from Lower Levels of Retinopathy (States 1 through 4) to State 5 Retinopathy (Proliferative Diabetic Retinopathy or Clinically Significant Macular Edema).*
Covariate | State 1 to State 5 | State 2 to State 5 | State 3 to State 5 | State 4 to State 5 | ||||
---|---|---|---|---|---|---|---|---|
Screening Interval | Probability | Screening Interval | Probability | Screening Interval | Probability | Screening Interval | Probability | |
percent | percent | percent | percent | |||||
Overall | 4 yr | 2.9 | 3 yr | 3.7 | 6 mo | 6.6 | 3 mo | 14.4 |
| ||||||||
Glycated hemoglobin level (%) | ||||||||
| ||||||||
![]() | ||||||||
| ||||||||
![]() ![]() | 5 yr | 1.0 | 5 yr | 1.9 | 6 mo | 4.3 | 3 mo | 3.3 |
| ||||||||
![]() ![]() | 5 yr | 3.5 | 4 yr | 4.4 | 3 mo | 3.0 | 1 mo | 3.1 |
| ||||||||
![]() ![]() | 3 yr | 4.3 | 2 yr | 4.5 | 3 mo | 4.0 | 1 mo | 7.8 |
| ||||||||
![]() | ||||||||
| ||||||||
![]() ![]() | 5 yr | 1.2 | 5 yr | 2.3 | 6 mo | 3.9 | 3 mo | 2.5 |
| ||||||||
![]() ![]() | 5 yr | 3.4 | 4 yr | 4.4 | 3 mo | 2.9 | 1 mo | 4.5 |
| ||||||||
![]() ![]() | 3 yr | 3.5 | 2 yr | 3.9 | 3 mo | 4.2 | 1 mo | 7.9 |
| ||||||||
Sex | ||||||||
| ||||||||
![]() | 4 yr | 2.9 | 3 yr | 3.8 | 3 mo | 3.1 | 1 mo | 5.7 |
| ||||||||
![]() | 4 yr | 2.9 | 3 yr | 3.7 | 3 mo | 3.8 | 1 mo | 5.5 |
| ||||||||
Treatment group | ||||||||
| ||||||||
![]() | 4 yr | 3.8 | 3 yr | 4.7 | 3 mo | 3.1 | 1 mo | 6.3 |
| ||||||||
![]() | 3 yr | 4.3 | 2 yr | 4.5 | 3 mo | 2.7 | 1 mo | 9.2 |
| ||||||||
Age (yr) | ||||||||
| ||||||||
![]() | 5 yr | 4.9 | 3 yr | 3.9 | 3 mo | 3.2 | 1 mo | 3.9 |
| ||||||||
![]() | 5 yr | 3.2 | 4 yr | 5.2 | 3 mo | 3.6 | 1 mo | 6.3 |
| ||||||||
![]() | 5 yr | 1.3 | 4 yr | 3.2 | 3 mo | 3.6 | 1 mo | 6.3 |
| ||||||||
Duration of diabetes (yr) | ||||||||
| ||||||||
![]() | 5 yr | 4.3 | 4 yr | 5.0 | 2 mo | 3.6 | 1 mo | 12.0 |
| ||||||||
![]() | 5 yr | 4.7 | 3 yr | 4.4 | 3 mo | 4.5 | 1 mo | 8.8 |
| ||||||||
![]() | 5 yr | 3.0 | 3 yr | 3.6 | 3 mo | 3.2 | 1 mo | 4.7 |
| ||||||||
![]() | 5 yr | 1.4 | 5 yr | 5.0 | 6 mo | 4.2 | 2 mo | 4.4 |
| ||||||||
Hypertension† | ||||||||
| ||||||||
![]() | 5 yr | 2.6 | 3 yr | 3.5 | 3 mo | 3.4 | 1 mo | 2.8 |
| ||||||||
![]() | 5 yr | 4.5 | 3 yr | 3.7 | 3 mo | 3.4 | 1 mo | 6.2 |
| ||||||||
Hyperlipidemia‡ | ||||||||
| ||||||||
![]() | 5 yr | 4.7 | 3 yr | 4.3 | 3 mo | 3.8 | 1 mo | 5.6 |
| ||||||||
![]() | 5 yr | 4.2 | 3 yr | 3.5 | 3 mo | 3.3 | 1 mo | 5.2 |
Models using current glycated hemoglobin values (Table S4 in the Supplementary Appendix) yielded hazard ratios for transition that were similar to those of the updated mean glycated hemoglobin level (Table 2), with nearly identical recommended visit schedules (Table 3). Although many covariates had significant effects on the risks of transitions (Table S4 in the Supplementary Appendix), the resulting proposed screening schedules within subgroups were similar, such as for men versus women and intensive versus conventional diabetes treatment. Separate mean glycated hemoglobin models within each treatment group showed that the cumulative-incidence functions at various glycated hemoglobin levels were similar in the conventional and intensive-treatment groups.
COST IMPLICATIONS
With the practical, evidence-based schedule (at 4 years, 3 years, 6 months, and 3 months), the risk of progression to state 5 from states 1 and 2 was less than 5%, but it was greater than 5% from states 3 and 4. The average time that state 5 retinopathy might go undetected was 0.42 years (Table 4); thus, less than 6 months would pass between the development of state 5 retinopathy and detection of it at the next examination. In comparison, fixed annual visits, regardless of retinopathy status, allowed for the nonlinear cumulative-incidence functions and yielded an average time of undetected state 5 retinopathy of 0.61 years. Thus, on average, the time during which state 5 retinopathy would go undetected was 2.3 months shorter with the practical, evidence-based schedule than with yearly screening. This improvement is based on more frequent visits in the high-risk retinopathy groups — specifically, the 6-month schedule for patients with state 3 retinopathy and the 3-month schedule for patients with state 4 retinopathy.
Table 4
Effect of Screening Schedules on Delay in the Detection of State 5 Retinopathy.
Schedule | Screening Interval | Average Delay in Detection of State 5 Retinopathy | Average Visits before Detection of State 5 Retinopathy during 20-Yr Period | |||
---|---|---|---|---|---|---|
State 1 | State 2 | State 3 | State 4 | |||
yr | no. | |||||
Practical* | 4 yr | 3 yr | 6 mo | 3 mo | 0.42 | 7.7 |
| ||||||
Annual (fixed) | 1 yr | 1 yr | 1 yr | 1 yr | 0.61 | 18.4 |
| ||||||
Precise (to the month)† | 5 yr 3 mo | 3 yr 7 mo | 4 mo | 1 mo | 0.81 | 7.4 |
Over a 20-year period, annual screening resulted in an average of 18.4 examinations up to the time of detection of state 5 retinopathy (or the end of the period) versus only 7.7 examinations with the practical, evidence-based 4-year, 3-year, 6-month, and 3-month schedule, for an average decrease of 10.7 (58% fewer) retinal examinations per patient (Table 4). Since digital photography costs approximately $200 and approximately 1 million patients have type 1 diabetes in the United States, the cost savings for eye screening with our data-driven approach, given the distribution of patients among the four states in the population, would be approximately $1 billion over 20 years, a 43.4% reduction as compared with routine annual screening (see the Supplementary Appendix).
Table 4 also shows a 5 year–3 month, 3 year– 7 month, 4-month, and 1-month screening schedule to the nearest month that would provide approximately a 5% chance of progression to state 5 retinopathy in patients in all four intermediate retinopathy states. The principal difference from the practical 4-year, 3-year, 6-month, and 3-month schedule is the longer screening interval for state 1. As a result, the undetected time is higher (0.81 years), but the number of visits (7.4) is slightly lower than with the practical schedule.
DISCUSSION
We developed Markov models on the basis of approximately 24,000 ophthalmologic assessments with seven-field fundus photography that were performed at intervals of 6 months to 48 months during almost 30 years of follow-up of our type 1 diabetes cohort. These models provide smoothed estimates of the cumulative incidence of progression of retinopathy from relatively benign levels (e.g., no retinopathy [state 1] or mild retinopathy [state 2]) that do not cause vision loss to more pernicious levels (proliferative diabetic retinopathy or clinically significant macular edema [state 5]) that can threaten sight and require timely detection and treatment. The models were used to formulate an evidence-based retinal screening schedule that was principally based on controlling the probabilities of progression to state 5 from lesser states to within an acceptable limit, such as 5%.
Although retinal examinations were performed every 6 months during the DCCT and every 4 years during the EDIC study, these models still allowed us to assess the properties of more frequent retinal examinations from the smooth estimated cumulative-incidence curves of the possible transitions. In aggregate, these models provide reliable estimates of the probability of progression of retinopathy over shorter periods, such as 3 months, with the use of the increment in risk over two points in time along a curve.
Progression from states 1 or 2 to state 5 retinopathy was unlikely over a period of 4 or more years, but progression was highly likely over shorter periods in patients with moderate (state 3) or severe (state 4) nonproliferative diabetic retinopathy. Our data suggest that a practical, evidence-based schedule for time to the next examination would be 4 years, 3 years, 6 months, and 3 months for patients with states 1 through 4, respectively, for which the corresponding cumulative incidence of progression to state 5 retinopathy would be 2.9%, 3.7%, 6.6%, and 14.4%.
Not surprisingly, within each retinopathy category, increasing glycated hemoglobin levels were associated with higher risks20 requiring more frequent screening schedules. Nonglycemic factors had only small additive effects beyond the glycated hemoglobin level.
Other investigators have described patient-specific recommendations for retinopathy screening, 21 including some using multistate Markov models22–24 and others comparing the cost-effectiveness of biannual and annual screening versus no screening.25,26 Our final models used extensive empirical data to determine an efficient personalized visit schedule that was based only on the current retinal disease state and glycated hemoglobin level. We also determined the length of time that severe, potentially vision-threatening retinopathy might remain undetected (i.e., the time between the onset of state 5 retinopathy and the next examination at which it is detected). The personalized schedules have the potential to reduce both the undetected time and the number of negative examinations at substantially lower cost. A Web application (https://extappsbscgwu.edu/shinypub/edic/retinopathy/) provides the cumulative incidence of state 5 retinopathy for a patient on the basis of the current level of retinopathy and the average glycated hemoglobin level (see the Supplementary Appendix). It can be used by health care providers to determine the recommended time until the next eye examination.
Scheduling retinopathy screenings at fixed intervals (e.g., annually) may be easier to implement than individualized scheduling on the basis of retinal status. However, we suspect that automated scheduling systems in common use by ophthalmologists could be used to cope with this potential barrier.
A limitation of our study is its reliance on seven-field fundus photography, rather than dilated or undilated ophthalmoscopy or other examinations. However, objective fundus photography is widely considered to be the standard-of-care method.27 Moreover, we speculate that increased use of photography and telemedicine may actually improve adherence to screening28 and lower the cost, even though the cost of photography is currently higher than that of ophthalmoscopy.
Additional sensitivity analyses show that the underlying model provides an adequate description of the DCCT experience, but it was not possible to fit more complex models that might apply. The model has not been validated in an independent cohort and may not be applicable to other populations.
Our analyses used the updated weighted mean glycated hemoglobin level to tailor the screening schedule on the basis of the previous DCCT/EDIC results showing that the mean glycated hemoglobin level had a strong association with the risk of progression of retinopathy.2,3 However, in practice, the physician is more likely to know the current glycated hemoglobin level than the historical mean glycated hemoglobin level. Additional analyses showed that the screening recommendations and probabilities of progression are virtually the same using the current or mean glycated hemoglobin values. If an additional glycated hemoglobin value is obtained between retinopathy examinations, then conservatively, the time of the next examination could be chosen as the earliest between the previously scheduled screening date and the date obtained using the more recent glycated hemoglobin value.
Our calculations apply to the distribution of retinopathy levels in our study cohort over the past 30 years but not necessarily in the general type 1 diabetes population for which such data are absent. However, the widespread adoption of intensive therapy has probably shifted retinopathy to less severe states29 for which an individualized schedule has the greatest cost savings relative to annual examinations.
We have previously found that pregnancy results in a period of increased maternal risk of worsening retinopathy that lasts for approximately 1 year after birth.30 These periods of increased risk account for less than 2% of our total study experience. Accordingly, we have elected to include the 180 women who had at least one pregnancy in these analyses. Finally, validation studies involving patients with type 2 diabetes should be performed before our findings in type 1 diabetes are generalized.
In conclusion, on the basis of nearly 30 years of retinopathy assessments, we developed Markov transition models to estimate the probabilities of progression from preclinical levels of retinopathy to proliferative diabetic retinopathy or clinically significant macular edema that require treatment to preserve vision. A practical, evidence-based, individualized screening schedule would provide a shorter time during which proliferative retinopathy or macular edema would go undetected and would require substantially fewer examinations than the currently recommended annual screening.
Acknowledgments
Supported by cooperative agreement grants (1982–1993, 2012–2017) and contracts (1982–2012) with the Division of Diabetes, Endocrinology, and Metabolic Diseases of the National Institute of Diabetes and Digestive and Kidney Diseases (current grant numbers, U01 DK094176 and U01 DK094157), and by the National Eye Institute, the National Institute of Neurological Disorders and Stroke, the General Clinical Research Center Program (1993–2007), and the Clinical and Translational Science Center Program (2006–present). Free or discounted supplies or equipment were contributed by Abbott Diabetes Care, Animas, Becton Dickinson, Diabetes Care by Bayer, Eli Lilly, Extend Nutrition, Insulet, LifeScan, Medtronic Diabetes, Nipro Home Diagnostics, Nova Diabetes Care, Omron, Perrigo Diabetes Care, Roche Diabetes Care, and Sanofi Aventis.
We thank our participant volunteers, whose dedication to improving the health of people with diabetes has made DCCT/EDIC possible.
Funded by the National Institute of Diabetes and Digestive and Kidney Diseases and others; DCCT/EDIC ClinicalTrials.gov numbers, NCT00360893 and NCT00360815.
Footnotes
Dr. Hainsworth reports holding stock in Katalyst Surgical. No other potential conflict of interest relevant to this article was reported.
Disclosure forms provided by the authors are available with the full text of this article at NEJM.org.
References
Full text links
Read article at publisher's site: https://doi.org/10.1056/nejmoa1612836
Read article for free, from open access legal sources, via Unpaywall:
https://europepmc.org/articles/pmc5557280?pdf=render
Citations & impact
Impact metrics
Article citations
2023 Clinical Practice Guidelines for Diabetes Management in Korea: Full Version Recommendation of the Korean Diabetes Association.
Diabetes Metab J, 48(4):546-708, 26 Jul 2024
Cited by: 2 articles | PMID: 39091005 | PMCID: PMC11307112
Automated Machine Learning for Predicting Diabetic Retinopathy Progression From Ultra-Widefield Retinal Images.
JAMA Ophthalmol, 142(3):171-177, 01 Mar 2024
Cited by: 5 articles | PMID: 38329765
12. Retinopathy, Neuropathy, and Foot Care: Standards of Care in Diabetes-2024.
Diabetes Care, 47(suppl 1):S231-S243, 01 Jan 2024
Cited by: 20 articles | PMID: 38078577
Review
14. Children and Adolescents: Standards of Care in Diabetes-2024.
Diabetes Care, 47(suppl 1):S258-S281, 01 Jan 2024
Cited by: 14 articles | PMID: 38078582
Review
Diabetes-Related Microvascular Complications in Primary Health Care Settings in the West Bank, Palestine.
J Clin Med, 12(21):6719, 24 Oct 2023
Cited by: 4 articles | PMID: 37959185 | PMCID: PMC10649955
Go to all (57) article citations
Data
Data behind the article
This data has been text mined from the article, or deposited into data resources.
BioStudies: supplemental material and supporting data
Clinical Trials (2)
- (2 citations) ClinicalTrials.gov - NCT00360893
- (2 citations) ClinicalTrials.gov - NCT00360815
Similar Articles
To arrive at the top five similar articles we use a word-weighted algorithm to compare words from the Title and Abstract of each citation.
Effects of Prior Intensive Insulin Therapy and Risk Factors on Patient-Reported Visual Function Outcomes in the Diabetes Control and Complications Trial/Epidemiology of Diabetes Interventions and Complications (DCCT/EDIC) Cohort.
JAMA Ophthalmol, 134(2):137-145, 01 Feb 2016
Cited by: 20 articles | PMID: 26584339 | PMCID: PMC4825807
Prolonged effect of intensive therapy on the risk of retinopathy complications in patients with type 1 diabetes mellitus: 10 years after the Diabetes Control and Complications Trial.
Arch Ophthalmol, 126(12):1707-1715, 01 Dec 2008
Cited by: 170 articles | PMID: 19064853 | PMCID: PMC2663518
Refractive Error and Retinopathy Outcomes in Type 1 Diabetes: The Diabetes Control and Complications Trial/Epidemiology of Diabetes Interventions and Complications Study.
Ophthalmology, 128(4):554-560, 14 Sep 2020
Cited by: 4 articles | PMID: 32941962 | PMCID: PMC7956062
Epidemiology of Ocular Functions and Diseases in Persons With Diabetes
National Institute of Diabetes and Digestive and Kidney Diseases (US), Bethesda (MD), 03 Mar 2021
Cited by: 0 articles | PMID: 33651534
ReviewBooks & documents Free full text in Europe PMC
Funding
Funders who supported this work.
NCATS NIH HHS (1)
Grant ID: UL1 TR001863
NIDDK NIH HHS (4)
Grant ID: P30 DK036836
Grant ID: U01 DK094176
Grant ID: U01 DK094157
Grant ID: P30 DK017047