Abstract
Importance
Among cognitively normal individuals, elevated brain amyloid (defined by cerebrospinal fluid assays or positron emission tomography regional summaries) can be related to risk for later Alzheimer-related cognitive decline.Objective
To characterize and quantify the risk for Alzheimer-related cognitive decline among cognitively normal individuals with elevated brain amyloid.Design, setting, and participants
Exploratory analyses were conducted with longitudinal cognitive and biomarker data from 445 cognitively normal individuals in the United States and Canada. Participants were observed from August 23, 2005, to June 7, 2016, for a median of 3.1 years (interquartile range, 2.0-4.2 years; maximum follow-up, 10.3 years) as part of the Alzheimer's Disease Neuroimaging Initiative (ADNI).Exposures
Individuals were classified at baseline as having normal (n = 243) or elevated (n = 202) brain amyloid using positron emission tomography amyloid imaging or a cerebrospinal fluid assay of amyloid β.Main outcomes and measures
Outcomes included scores on the Preclinical Alzheimer Cognitive Composite (PACC; a sum of 4 baseline standardized z scores, which decreases with worse performance), Mini-Mental State Examination (MMSE; 0 [worst] to 30 [best] points), Clinical Dementia Rating Sum of Boxes (CDR-Sum of Boxes; 0 [best] to 18 [worst] points), and Logical Memory Delayed Recall (0 [worst] to 25 [best] story units).Results
Among the 445 participants (243 with normal amyloid, 202 with elevated amyloid), mean (SD) age was 74.0 (5.9) years, mean education was 16.4 (2.7) years, and 52% were women. The mean score for PACC at baseline was 0.00 (2.60); for MMSE, 29.0 (1.2); for CDR-Sum of Boxes, 0.04 (0.14); and for Logical Memory Delayed Recall, 13.1 (3.3). Compared with the group with normal amyloid, those with elevated amyloid had worse mean scores at 4 years on the PACC (mean difference, 1.51 points [95% CI, 0.94-2.10]; P < .001), MMSE (mean difference, 0.56 points [95% CI, 0.32-0.80]; P < .001), and CDR-Sum of Boxes (mean difference, 0.23 points [95% CI, 0.08-0.38]; P = .002). For Logical Memory Delayed Recall, between-group score was not statistically significant at 4 years (mean difference, 0.73 story units [95% CI, -0.02 to 1.48]; P = .056).Conclusions and relevance
Exploratory analyses of a cognitively normal cohort followed up for a median of 3.1 years suggest that elevation in baseline brain amyloid level, compared with normal brain amyloid level, was associated with higher likelihood of cognitive decline, although the findings are of uncertain clinical significance. Further research is needed to assess the clinical importance of these differences and measure longer-term associations.Free full text

Association Between Elevated Brain Amyloid and Subsequent Cognitive Decline Among Cognitively Normal Persons
Abstract
IMPORTANCE
Among cognitively normal individuals, elevated brain amyloid (defined by cerebrospinal fluid assays or positron emission tomography regional summaries) can be related to risk for later Alzheimer-related cognitive decline.
OBJECTIVE
To characterize and quantify the risk for Alzheimer-related cognitive decline among cognitively normal individuals with elevated brain amyloid.
DESIGN, SETTING, AND PARTICIPANTS
Exploratory analyses were conducted with longitudinal cognitive and biomarker data from 445 cognitively normal individuals in the United States and Canada. Participants were observed from August 23, 2005, to June 7, 2016, for a median of 3.1 years (interquartile range, 2.0–4.2 years; maximum follow-up, 10.3 years) as part of the Alzheimer’s Disease Neuroimaging Initiative (ADNI).
EXPOSURES
Individuals were classified at baseline as having normal (n = 243) or elevated (n = 202) brain amyloid using positron emission tomography amyloid imaging or a cerebrospinal fluid assay of amyloid β.
MAIN OUTCOMES AND MEASURES
Outcomes included scores on the Preclinical Alzheimer Cognitive Composite (PACC; a sum of 4 baseline standardized Z scores, which decreases with worse performance), Mini-Mental State Examination (MMSE; 0 [worst] to 30 [best] points), Clinical Dementia Rating Sum of Boxes (CDR–Sum of Boxes; 0 [best] to 18 [worst] points), and Logical Memory Delayed Recall (0 [worst] to 25 [best] story units).
RESULTS
Among the 445 participants (243 with normal amyloid, 202 with elevated amyloid), mean (SD) age was 74.0 (5.9) years, mean education was 16.4 (2.7) years, and 52% were women. The mean score for PACC at baseline was 0.00 (2.60); for MMSE, 29.0 (1.2); for CDR–Sum of Boxes, 0.04 (0.14); and for Logical Memory Delayed Recall, 13.1 (3.3). Compared with the group with normal amyloid, those with elevated amyloid had worse mean scores at 4 years on the PACC (mean difference, 1.51 points [95% CI, 0.94–2.10]; P < .001), MMSE (mean difference, 0.56 points [95% CI, 0.32–0.80]; P < .001), and CDR–Sum of Boxes (mean difference, 0.23 points [95% CI, 0.08–0.38]; P = .002). For Logical Memory Delayed Recall, between-group score was not statistically significant at 4 years (mean difference, 0.73 story units [95% CI, −0.02 to 1.48]; P = .056).
CONCLUSIONS AND RELEVANCE
Exploratory analyses of a cognitively normal cohort followed up for a median of 3.1 years suggest that elevation in baseline brain amyloid level, compared with normal brain amyloid level, was associated with higher likelihood of cognitive decline, although the findings are of uncertain clinical significance. Further research is needed to assess the clinical importance of these differences and measure longer-term associations.
Clinical diagnosis of Alzheimer disease (AD) has required the syndrome of dementia with impairment of multiple cognitive domains sufficient to interfere with performance of daily activities. Dementia is preceded by the syndrome of mild cognitive impairment (MCI) with symptoms and objective evidence of cognitive impairment but with preservation of function. Biomarkers of AD pathology, particularly amyloid positron emission tomography (PET) or cerebrospinal fluid (CSF) evidence of brain amyloid, allow specific identification of individuals with MCI likely to progress to AD dementia. MCI accompanied by such biomarker abnormalities is now considered to be prodromal AD.1
Accumulating evidence suggests that the neurobiology of AD precedes symptoms by years.2 Approximately one-third of individuals with clinically normal cognition, who are at least 65 to 70 years of age, may have biomarker evidence of elevated brain amyloid3—an assertion consistent with 2 large postmortem studies.4,5 This has been termed the preclinical stage of AD.3 Studies indicate that elevated amyloid is associated with risk of cognitive worsening; the risk of progression to MCI over 5 to 6 years ranges from 11% to 56% with higher risk associated with biomarker evidence of neurodegeneration, such as hippocampal atrophy or CSF tau, at baseline.6–8
The concept of preclinical AD has important implications for the understanding of aging and AD and for drug development. Preclinical AD trials have been launched based on the hypothesis that disease-modifying interventions will be most effective when initiated early.9 However, a major question remains: is preclinical AD part of AD, with inevitable progression to symptomatic disease? The purpose of this investigation was to characterize the cognitive changes among participants of the Alzheimer’s Disease Neuroimaging Initiative (ADNI)10 with normal cognition or subjective memory concerns and to compare individuals with vs without elevated brain amyloid at baseline.
Methods
The ADNI study has observed individuals diagnosed as cognitively normal or with varying degrees of cognitive impairment since 2005.10 The ADNI battery includes serial evaluations through neuroimaging, CSF, and other biomarkers, and through clinical and neuropsychological assessments. For the present analysis, the subset of participants with normal cognition or a subjective memory concern with CSF β-amyloid peptide (Aβ42) measurement or amyloid PET imaging were analyzed. Amyloid PET imaging was conducted with 2 different tracers: Pittsburgh Compound B (PiB) and florbetapir. Individuals were classified as having elevated brain amyloid based on high amyloid PET standardized uptake value ratio (SUVR)11 or low CSF Aβ42. Amyloid PET SUVRs are a summary of uptake in the frontal, cingulate, temporal, and parietal regions relative to the whole cerebellum.
All participants included in the analysis had baseline Mini-Mental State Examination (MMSE)12 scores of 24 to 30 (range, 0 [worst] to 30 [best]) and Clinical Dementia Rating (CDR)13 Global and Memory Box scores of 0 (range for each, 0 [best] to 3 [worst]). Logical Memory Delayed Recall14 scores are based on years of education and were required to be at least 9 for 16 years of education, at least 5 for 8 to 15 years of education, and at least 3 for 0 to 7 years of education (range, 0 [worst] to 25 [best] story units). Participants were allowed to have subjective memory concerns as self-reported or reported by a study partner or clinician. Participants returned for follow-up assessment at 6 months, 1 year, and every year thereafter. Race and ethnicity were self-reported using fixed categories as required by the National Institutes of Health. Data were collected at 83 ADNI sites in the United States and Canada from August 23, 2005, to June 7, 2016. ADNI was approved by the institutional review boards of all participating institutions. Written informed consent was obtained from all participants at each site.
Exposure
Only individuals with at least 1 follow-up evaluation and at least 1 observation of florbetapir amyloid PET, PiB amyloid PET, or CSF Aβ42 were included in this analysis. Baseline values of florbetapir SUVR, PiB SUVR, and ventricular volume were imputed as necessary for individuals with follow-up, but no baseline observation, using a linear mixed-effects model. The models included individual-specific random intercepts and slopes, and fixed effects for time, age, apolipoprotein E (APOE) ε4 carriage, and Preclinical Alzheimer Cognitive Composite (PACC)15 at baseline. PiB SUVR values were then transformed to florbetapir using the formula y = 0.15 + 0.67x.11 Elevated amyloid at baseline was defined by florbetapir SUVR greater than 1.1 (a threshold derived from discrimination of probable Alzheimer cases from younger healthy control participants in an independent data set11) or CSF Aβ42 less than 192 pg/mL (a threshold derived from discrimination of autopsy-confirmed Alzheimer cases in an independent data set16). Participants were classified as having elevated amyloid if they met either threshold. Otherwise, they were classified as having normal amyloid.
Outcomes
Continuous response variables included PACC (a sum of 4 baseline standardized z scores [decreases with Delayed Recall.14 Bio-markers included FreeSurfer ventricular and hippocampal volumes17; CSF tau, phospho-tau (pTau), Aβ42, fluorodeoxy-glucose (FDG)-PET, and florbetapir PET.16 FDG-PET was used to measure glucose metabolism in the brain while florbetapir PET and PiB PET were used to measure brain amyloid accumulation. The version of the PACC used in this analysis was modified from the original because of specific assessments used in ADNI. In particular, the modified PACC is a baseline standardized z score composite of the Alzheimer Disease Assessment Scale—Cognitive Subscale Delayed Word Recall, Logical Memory Delayed Recall, MMSE, and (log-transformed) Trail-Making Test B Time to Completion.18 Binary response variables included CDR–Global scores greater than 0, any increase in CDR–Sum of Boxes, progression to early MCI, and progression to late MCI. The distinction between early MCI and late MCI was based solely on the severity of episodic memory impairment as measured by the Logical Memory II paragraph recall test. Early MCI and late MCI were defined as having CDR–Global of greater than 0 and Logical Memory scores below the education-adjusted thresholds described in eTable 1 in the Supplement.19
Statistical Methods
The characteristics of the elevated amyloid and normal amyloid groups were summarized, and differences were tested by 2-sample t test or Pearson χ2 test. Progression by amyloid group among ADNI participants with normal cognition was analyzed using linear and generalized linear mixed-effects models.20,21 The primary analysis treated time as continuous. Quadratic terms for time were added if supported by Akaike Information Criterion (AIC),22 an objective model selection tool. Additional models were included that were identical to the primary models except that time was treated as categorical. These categorical time models made no assumptions about the shape of temporal trends, and therefore were useful for assessing the shape assumptions imposed by the continuous time models. Age, which is known to be associated with amyloid burden, was included as a covariate in all models. Additional covariates were selected by AIC among APOEε4 carriage, sex, family history of dementia, education, and ventricular volume at baseline. Baseline cognitive measures were not included as covariates because they were instead modeled as response variables. For linear models, the correlation structure was selected by AIC from random intercept, random slope, compound symmetric, and unstructured options.
The overall amyloid group effect was tested using likelihood ratio tests that compared the full model to a reduced model with no parameters for amyloid group. Additional sensitivity analyses included logistic mixed-effects models of potentially binomial outcomes (MMSE, CDR–Sum of Boxes, and Logical Memory Delayed Recall). Logistic mixed-effects models were also fit to repeated binary response variables. Because fixed effects from logistic mixed effects are conditional (on random-effects estimates), the parametric bootstrap was used to derive marginal estimates of event rates over time and P values. Marginal estimates are more appropriate for interpretation at the population level. Logistic models included random intercepts only.
Because fewer data were available at the later study visits, point estimates at year 4 were emphasized. The primary analysis approach, mixed-effect models fit by maximum likelihood, was robust to data missing at random (ie, missingness that was dependent only on other data included in the model). A multiple imputation approach was also applied that was also robust to the same missing at random assumption, but it could typically admit more auxiliary variables, making the missing at random assumption more plausible. To assess the sensitivity of the results to departures from missing at random, imputed values for participants with elevated amyloid were shifted closer to those with normal amyloid by varying degrees (range, 50%–100% in 10% increments).23 The minimum factor for which the amyloid group difference at year 10 was no longer significant at the .05 level was reported. This analysis provides an assessment of how robust the conclusions are to potential bias induced by attrition.
Participants could continue in the study regardless of the initiation of any medication for dementia, but no individuals in this cohort with normal cognition or subjective memory concerns were taking such medication at baseline. No adjustments were made to control type I error; therefore, all analyses should be considered exploratory. All tests were 2-sided and P values smaller than .05 were considered statistically significant. Models were fit and plotted using R (version 3.2.2, https://www.R-project.org/).
Results
Key variables by amyloid group are reported in the Table. Participants were classified as having normal amyloid (n = 243 [55%]) and elevated amyloid (n = 202 [45%]; 95% CI, 41% to 50%). The 2 groups had similar percentages of individuals with subjective memory concerns (22% in the normal amyloid group and 25% in the elevated amyloid group) with a difference of 3% (95% CI, −5% to 10%; P = .53). The elevated amyloid group was older (74.7 vs 73.4 years; P = .02), less educated (16.1 vs 16.7 years; P = .02), had lower PACC scores (−0.38 vs 0.32; P = .02), and a greater proportion of individuals with at least 1 APOEε4 allele (42% vs 16%; difference, 26% [95% CI, 17% to 34%]; P < .001). Missing baseline amyloid PET SUVRs were linearly interpolated from follow-up florbetapir PET SUVRs (55 in the normal amyloid group vs 36 in the elevated amyloid group) or PiB PET SUVRs (7 in the normal amyloid group vs 12 in the elevated amyloid group). eFigures 1 and 2 in the Supplement illustrate these imputations, based on the models summarized in eTables 2 and 3 in the Supplement.
Table
Participant Characteristics By Brain Amyloid Groupa
Normal Brain Amyloid (n = 243) | Elevated Brain Amyloid (n = 202)b | Overall (N = 445) | P Valuec | |
---|---|---|---|---|
Baseline Characteristics | ||||
Age, mean (SD), y | 73.4 (5.8) | 74.7 (6.0) | 74.0 (5.9) | .02 |
Women | 115 (47) | 118 (58) | 233 (52) | .02 |
Education, mean (SD), y | 16.7 (2.7) | 16.1 (2.6) | 16.4 (2.7) | .02 |
Family history of dementia | 88 (36) | 87 (43) | 175 (39) | .14 |
Ethnicity | ||||
![]() | 233 (96) | 193 (96) | 426 (96) | .75 |
![]() | 9 (4) | 7 (3) | 16 (4) | |
![]() | 1 (0.4) | 2 (1) | 3 (1) | |
Race | ||||
![]() | 0 | 1 (0.5) | 1 (0.2) | .39 |
![]() | 4 (2) | 2 (1) | 6 (1) | |
![]() | 15 (6) | 14 (7) | 29 (7) | |
![]() | 223 (92) | 181 (90) | 404 (91) | |
![]() | 1 (0.4) | 4 (2) | 5 (1) | |
Subjective memory concern | 54 (22) | 50 (25) | 104 (23) | .53 |
≥1 APOEz4 allele | 40 (16) | 85 (42) | 125 (28) | <.001 |
PACC (z score composite), mean (SD)d | 0.32 (2.53) | −0.38 (2.64) | 0.00 (2.60) | .02 |
MMSE, mean (SD)d | 29.1 (1.2) | 29.0 (1.1) | 29.0 (1.2) | .99 |
Logical Memory Delayed Recall, mean (SD)d | 13.4 (3.2) | 12.9 (3.3) | 13.1 (3.3) | .08 |
ADAS13, mean (SD)d | 9.0 (4.3) | 9.5 (4.4) | 9.2 (4.3) | .28 |
CDR-Sum of Boxesd | ||||
![]() | 227 (93) | 186 (92) | 413 (93) | .51 |
![]() | 16 (7) | 15 (7) | 31 (7) | |
![]() | 0 | 1 (0.5) | 1 (0.2) | |
Ventricular volume, mean (SD), % ICV | 2.1 (1.1) | 2.3 (1.1) | 2.2 (1.1) | .07 |
CSF tau, mean (SD), pg/mLe | 59.4 (22.5) | 76.8 (38.4) | 67.8 (32.3) | <.001 |
CSF pTau, mean (SD), pg/mLe | 26.5 (11.8) | 38.1 (22.3) | 32.1 (18.6) | <.001 |
CSF Aβ42 <192 pg/mLe | 0/194 | 155/178 (87) | 155/372 (42) | |
CSF Aβ42, mean (SD), pg/mLe | 238.9 (26.6) | 157.5 (36.8) | 200.0 (51.7) | |
Amyloid PETSUVR, >1.1e,f | 0/208 | 138/177 (78) | 138/385 (36) | |
Amyloid PET SUVR, mean (SD)e,f | 1.0 (0.06) | 1.2 (0.19) | 1.11 (0.18) | |
Elevated brain amyloid by PET and CSFe | 0/159 | 91/153 (59) | 91/312 (29) | |
Follow-up Characteristics | ||||
Follow-up, mean (SD), y | 4.2 (2.8) | 3.7 (2.6) | 3.9 (2.7) | .05 |
Last known status | ||||
![]() | 158 (65) | 121 (60) | 279 (63) | .34 |
![]() | 82 (34) | 80 (40) | 162 (36) | |
![]() | 3 (1) | 1 (0.5) | 4 (1) | |
Progression to dementia | 7 (3) | 13 (6) | 20 (4) | .01 |
Initiated antidementia medicationg | 9 (4) | 20 (10) | 29 (7) | .01 |
Abbreviations: Aβ42, β-amyloid; ADAS13, Alzheimer Disease Assessment Scale 13-item cognitive subscale; APOEε4, apolipoprotein E; CDR, Clinical Dementia Rating; CSF, cerebrospinal fluid; ICV, intracranial volume; MMSE, Mini-Mental State Examination; PACC, Preclinical Alzheimer Cognitive Composite; pTau, phospho-tau; PiB, Pittsburgh Compound B; PET, positron emission tomography; SUVR, standard uptake value ratio.
Baseline ventricular volume imputations are depicted in eFigure 3 in the Supplement, based on the model summarized in eTable 4 in the Supplement. eTable 5 in the Supplement provides summary statistics of the follow-up time for observations of each outcome, eTable 6 in the Supplement summarizes the number of individuals lost to follow-up by month, and eTable 7 in the Supplement summarizes the baseline characteristics by study status at last follow-up (still active vs lost to follow-up or deceased). Overall, 279 of the 445 participants (63%) were reported to be still active in the study and the remainder were lost to follow-up (n = 162) or deceased (n = 4).
Figure 1 shows modeled mean profiles of the continuous outcomes by amyloid group controlling for covariates selected by AIC treating time as categorical and continuous. The likelihood ratio test for the overall amyloid group effect was significant for PACC (continuous time model
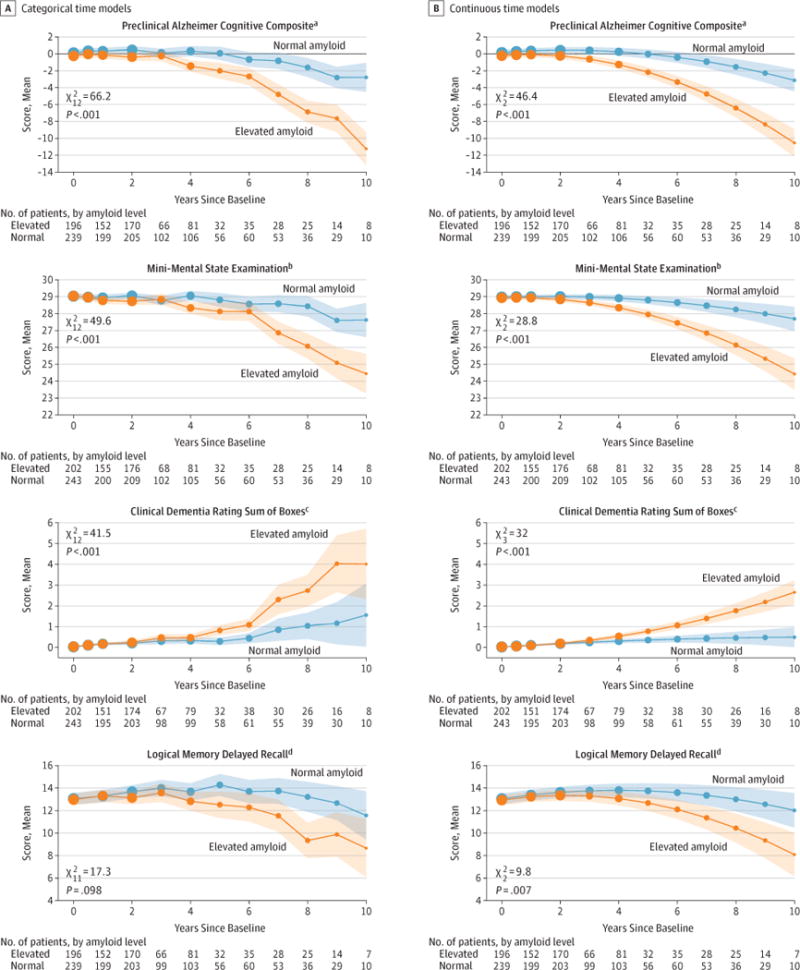
Linearmixed-effectsmodelswerecontrolledforageandotherbaselinecovariatesand are conditional on covariates as follows: mean age (74 years), mean education (16.4 years), men, and APOEε4=0.5 (range, 0–1 APOEε4 alleles) (Supplement). Likelihood ratio tests comparing models (elevated amyloid group vs normal) were used for χ2 statistics and P values. Shaded regions indicate 95% CIs. Dot sizes are proportional to the number of observations. Continuous time models include a quadratic term.
aSelected covariates were sex, ventricular volume, family history, and education (sum of 4 baseline standardized Z scores that decrease with worse performance).
bSelected covariates were sex and education (score range, 0 [worst] to 30 [best]).
cNo additional covariates were selected (score range, 0 [best] to 18 [worst]).
dSelected covariates were APOEε4, sex, ventricular volume, and education (score range, 0 [worst] to 25 [best] story units).
The group with elevated amyloid had worse mean scores at 4 years on the PACC, MMSE, and CDR–Sum of Boxes. The mean PACC was 0.16 (95% CI, −0.16 to 0.49) at baseline and was 0.25 (95% CI, −0.22 to 0.72) at 4 years for normal amyloid and −0.23 (95% CI, −0.54 to 0.08) at baseline and −1.27 (95% CI, −1.74 to −0.78) at 4 years for elevated amyloid (mean difference, 1.51 points [95% CI, 0.94 to 2.08]; P < .001). The MMSE was 29.0 (95% CI, 28.9 to 29.1) at baseline and 28.9 (95% CI, 28.7 to 29.1) at 4 years for normal amyloid and 28.9 (95% CI, 28.8 to 29.1) at baseline and 28.3 (95% CI, 28.1 to 28.6) at 4 years for elevated amyloid (mean difference, 0.56 points [95% CI, 0.32 to 0.80]; P < .001). The CDR–Sum of Boxes was 0.03 (95% CI, 0.01 to 0.05) at baseline and 0.31 (95% CI, 0.21 to 0.41) at 4 years for normal amyloid and 0.04 (95% CI, 0.01 to 0.06) at baseline and 0.54 (95% CI, 0.43 to 0.66) at 4 years for elevated amyloid (mean difference, 0.23 points [95% CI, 0.08 to 0.38]; P = .002). Logical Memory Delayed Recall was 13.1 (95% CI, 12.6 to 13.5) at baseline and 13.8 (95% CI, 13.2 to 14.4) at 4 years for normal amyloid and 12.9 (95% CI, 12.5 to 13.4) at baseline and 13.1 (95% CI, 12.5 to 13.7) at 4 years for elevated amyloid (mean difference, 0.73 story units [95% CI, −0.02 to 1.48]; P = .056). Based on the limited data at 10 years, the mean amyloid group difference was projected to increase for the PACC (estimated at 7.40 points [95% CI, 5.30 to 9.50]; P < .001), MMSE (estimated at 3.27 points [95% CI, 2.10 to 4.44]; P < .001), and CDR–Sum of Boxes (estimated at 2.16 points [95% CI, 1.40 to 2.91]; P < .001).
The supplemental material includes other sensitivity analyses including profiles from models excluding individuals with imputed amyloid status (eFigures 4 and 5 in the Supplement), models excluding individuals with subjective memory concerns (eFigures 6 and 7 in the Supplement), and models controlling for age only (eFigures 8 and 9 in the Supplement). eFigure 10 in the Supplement shows a spaghetti plot of the raw PACC observations, and eFigure 11 in the Supplement shows profiles from logistic mixed-effects models of MMSE, CDR–Sum of Boxes, and Logical Memory Delayed Recall. Results were similar across these supplemental analyses.
Figure 2 shows the modeled proportion of progression events over time by amyloid group with covariates selected by AIC. The likelihood ratio test for the overall amyloid group association was significant at P < .001 level for all 4 progression events when time was treated as continuous. With the categorical time models, the overall amyloid group association was significant at P < .001 level for CDR–Global and early MCI and was significant at the P = .01 level for CDR–Sum of Boxes and late MCI progression. eTables 12 through 15 in the Supplement summarize the covariates selected by AIC for these models. At 4 years, 32.2% of individuals with elevated amyloid had developed symptoms consistent with the prodromal stage of AD, as indicated by a CDR–Global score of 0.5; in comparison to 15.3% of individuals with normal amyloid (difference, 16.9% [95% CI, 7.5% to 26.0%]; P < .001). Based on the limited data at 10 years, 88.2% of individuals with elevated amyloid were estimated to have progressed based on the CDR–Global assessment compared with only 29.4% of individuals with normal amyloid (difference, 58.8% [95% CI, 40.3 to 78.2%]; P < .001). At 4 years, a greater proportion of individuals with elevated amyloid (14.5%) vs normal amyloid (6.9%) also progressed on CDR–Sum of Boxes assessment (difference, 7.6% [95% CI, 1.6% to 12.7%]; P = .003) to early MCI (normal amyloid, 3.0%; elevated amyloid, 9.2%; difference, 6.2% [95% CI, 2.2% to 9.8%]; P < .001) and to late MCI (normal amyloid, 1.6%; elevated amyloid, 3.9%; difference, 2.3% [95% CI, 0.079% to 5.0%]; P = .02).
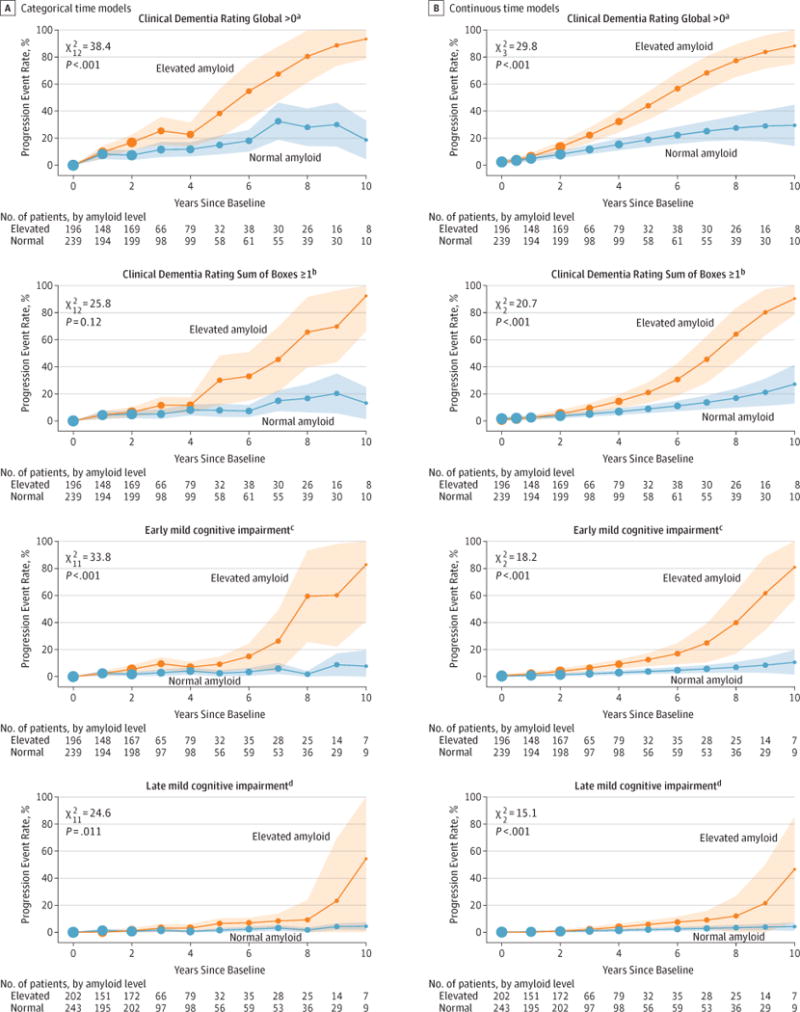
See Figure 1 for explanation of statistical components.
aSelected covariates were education, Preclinical Alzheimer Cognitive Composite (PACC), and ventricular volume. Model includes a quadratic effect.
bSelected covariates were PACC and ventricular volume.
cThe selected covariate was PACC.
dThe selected covariate was education.
Ventricular volume was selected as a predictor variable in models of PACC (−0.21 points per %ICV [95% CI −0.41 to −0.01]; P = .04), Logical Memory Delayed Recall (−0.25 story units per %ICV [95% CI, −0.55 to 0.04]; P = .09), CDR–Global progression (odds ratio [OR] = 1.8 [95% CI, 1.2 to 2.5]; P = .002), CDR–Sum of Boxes progression (OR = 2.0 [95% CI, 1.3 to 3.1]; P = .002), and early MCI progression (OR = 2.0 [95% CI, 1.1 to 3.7]; P = .03). Family history was only selected as a covariate in models of the PACC, but the association was not statistically significant.
Mean biomarker profiles are depicted in Figures 3 and and4.4. All the biomarker profiles considered were significantly separated (P < .001). Among individuals with elevated amyloid, those with at least 1 APOEε4 allele showed greater cognitive decline on the modified PACC than those with no ε4 allele (eFigure 12 in the Supplement; likelihood ratio, P < .001). A greater proportion of individuals with elevated amyloid initiated antidementia medication use (eFigure 13 in the Supplement, likelihood ratio P = .04).
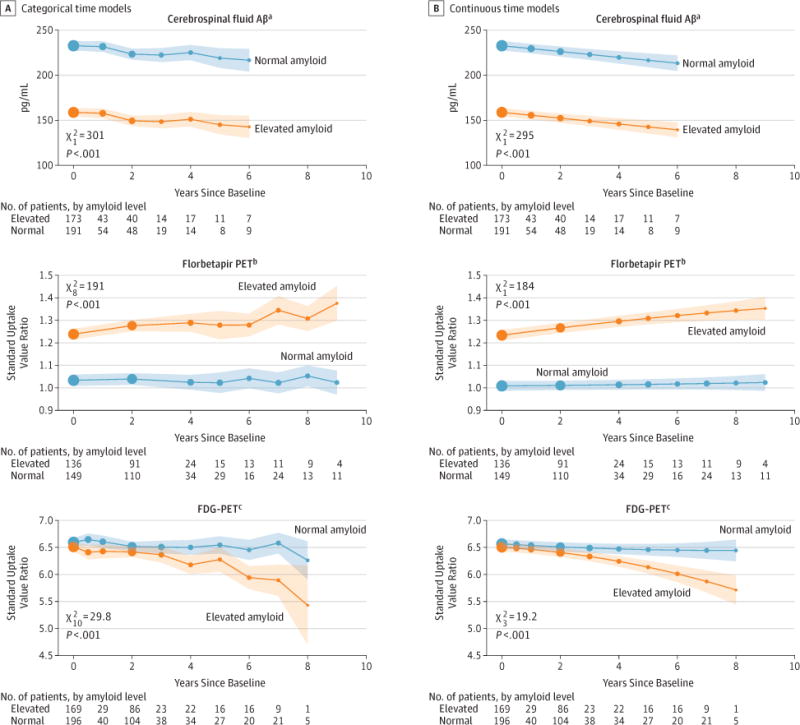
Profiles are from linear mixed-effects models controlling for age and other baseline covariates selected by Akaike Information Criterion. Profiles are conditional on covariates as follows: mean age (74 years) and ventricular volume (2.2% ICV), men, APOEε4=0.5 (APOEε4=0 indicates 0 alleles; APOEε4=1 indicates ≥1 allele). Shaded regions indicate 95% CIs. Dot sizes are proportional to the number of observations. Abbreviations: FDG, fluorodeoxyglucose; PET, positron emission tomography.
aSelected covariates were APOEε4 and ventricular volume.
bSelected covariates were APOEε4 and sex, and this model did not include longitudinal Pittsburgh Compound B observations.
cSelected covariates were APOEε4 and sex.
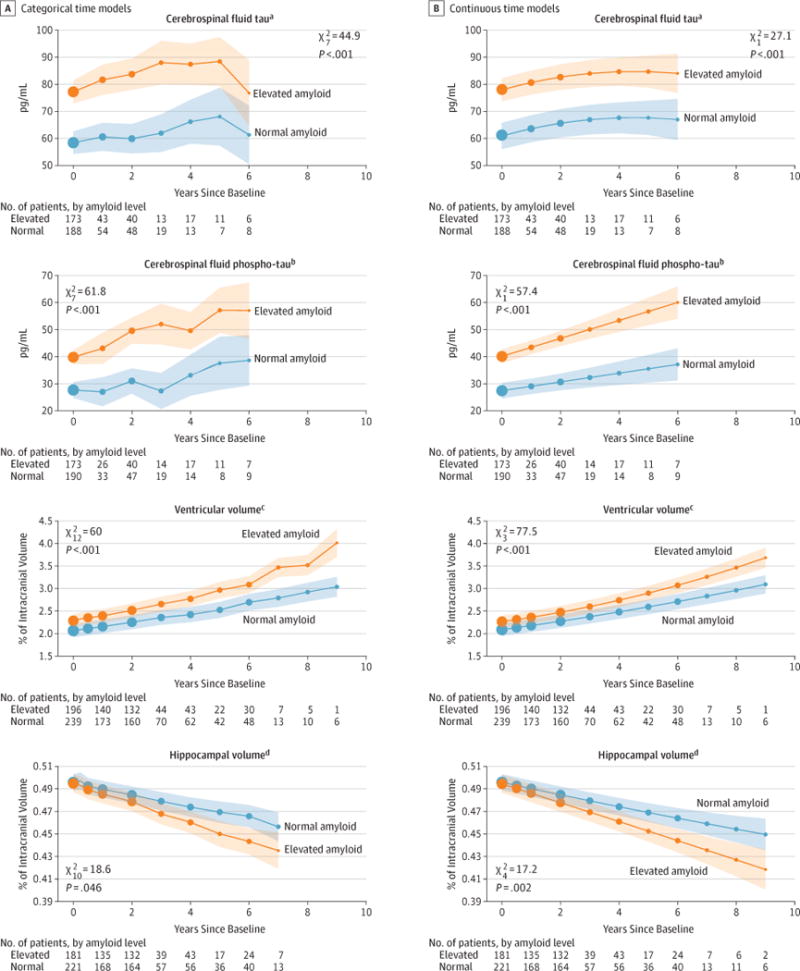
Profiles are from linear mixed-effects models controlling for age and other baseline covariates selected by Akaike Information Criterion. P values are from likelihood ratio tests comparing models (elevated amyloid group vs normal). Shaded regions indicate 95% CIs. Dot sizes are proportional to the number of observations.
aSelected covariates were APOEε4 and ventricular volume.
bSelected covariates were APOEε4, education, and ventricular volume.
cSelected covariates were APOEε4, sex, and education.
dSelected covariates were sex and education.
The multiple imputation sensitivity analysis found that the estimated differences at year 10 in continuous measures were significant (P < .05) after shifting the imputed values for the elevated amyloid group 40% of the way toward the normal amyloid group (CDR–Sum of Boxes was robust up to a 50% shift). Differences in the estimated rate of progression at year 10 maintained significance (P < .05) after a 30% shift of CDR–Global, an 80% shift of CDR–Sum of Boxes, and an 80% shift of CDR–Global and Logical Memory Delayed Recall for early MCI progression, and a 70% shift of CDR–Global and Logical Memory Delayed Recall for late MCI progression.
Discussion
In this natural history biomarker study of cognitively normal individuals, a larger proportion of the individuals with elevated brain amyloid at baseline developed cognitive symptoms compared with the individuals without elevated brain amyloid at baseline. Dichotomizing participants into elevated vs normal amyloid groups separated those with progressive cognitive decline from those without cognitive decline. Even though the interpretation was influenced by the small percentage of participants observed for 10 years, this suggests that preclinical AD, defined as clinically normal individuals with elevated brain amyloid,3 may represent the presymptomatic stage of AD. Additional follow-up of the ADNI cohort will be important to confirm these observations. Although this work did not establish a causal role of elevated amyloid in subsequent decline, these results supported other findings (eg, genetic data24,25) pointing to the critical role of amyloid in the neurobiology of AD.
Analyses included a modified version of the PACC, a cognitive composite designed as an outcome measure for preclinical AD trials,15 as well as the MMSE and Logical Memory tests, which are components of the PACC. Each of these measures showed little or no decline over 10 years among individuals without elevated brain amyloid, in contrast to decline evident 3 to 4 years from baseline among those with elevated amyloid.
Biomarker data presented a more complex picture. Measurement of CSF tau, pTau, and Aβ42 showed marked differences between groups at baseline, indicating association with brain amyloid elevation (determined in some by CSF Aβ42 reduction). Longitudinal change in these biomarkers was similar across groups, suggesting that they are sensitive to elevated amyloid but do not reflect cognitive and clinical decline once amyloidosis is established. Brain atrophy, as reflected by enlargement of ventricular size, showed change in participants with normal amyloid but greater change in participants with elevated amyloid. This trend is consistent with brain atrophy with normal aging that is aggravated in the presence of amyloid.
In the clinical context, assessment of brain amyloid status by lumbar puncture or amyloid PET scan in clinically normal older individuals supports a diagnosis of preclinical AD3 with attendant prognostic information. Accurate estimates of sensitivity, specificity, and predictive value regarding the dementia stage of AD will require continued follow-up. No effective treatments are yet available for preclinical AD; there may be some value to early diagnosis for family education and planning. Therapeutic trials are now underway to assess whether the prognosis of preclinical AD can be altered by interventions targeting amyloid.
The modified PACC assessment captures performance on tasks of episodic memory, orientation, and executive function—the prominent domains of AD-related cognitive dysfunction. The longitudinal curves suggested that decline on the PACC was restricted to those with evidence of elevated brain amyloid; the participants with normal amyloid showed minimal change over 10 years. This finding strengthens the link between elevated amyloid and the primary manifestations of AD. Individuals with normal amyloid remain free of AD-pattern impairment for many years.
The longitudinal CDR curves demonstrated that those participants with elevated amyloid were more likely to develop early symptoms consistent with prodromal AD within 6 years. This suggests that elevated brain amyloid may indicate a pre-symptomatic stage of disease rather than a risk factor for disease; AD is a gradually progressive disorder that can be identified at the asymptomatic phase using biomarkers of amyloid. Treatment of preclinical AD with antiamyloid or other interventions, if shown to be effective, would represent management of disease rather than risk reduction. This view is important in the consideration of risk and benefit inherent in weighing therapeutic options from a regulatory perspective and for an individual. Accurate characterization of the potential progression of earliest symptoms may inform discussion of the utility of screening people for amyloid abnormalities, with or without effective therapeutic options.
APOE genotype is the most important genetic risk factor for sporadic AD, and it exerts effects on amyloid accumulation as well as on amyloid-independent mechanisms.26 In the preclinical AD population in ADNI, the presence of an APOEε4 allele was associated with substantially increased cognitive decline. This suggests a strong association of APOE genotype, in addition to the association of amyloid, at this early stage of disease as has been reported previously.27
Regulatory bodies have moved toward supporting early intervention trials in preclinical AD,28 but uncertainty remains about ascribing clinical meaningfulness to slowing of progression at an asymptomatic stage. These analyses of long-term follow-up of untreated individuals with elevated brain amyloid can inform consideration of risk and benefit for putative disease-modifying interventions.
Estimates of the prevalence of AD are generally based on assessment of dementia; recent data suggest that there are 5 to 6 million cases of AD in the United States and more than 30 million worldwide.29 Preclinical and prodromal AD represent predementia stages of the illness. If these stages, which together last longer than the dementia stage, are included in prevalence estimates, the numbers of affected individuals are more than doubled.
Limitations
This study has several limitations. First, the use of antidementia medications during follow-up was infrequent but greater in the group with elevated amyloid. This may have slowed the progression of cognitive decline in some patients and mildly reduced the between-group difference in rate of decline. Second, group differences and changes on continuous measures are of uncertain clinical importance. Third, the limited number of observations at the latest time points and high rate of loss to follow-up in this study raise concerns that conclusions made about these latest time points might be unsupported extrapolations of earlier trends. However, when models imposed no assumptions about the shape of the mean trajectories, conclusions were similar. These categorical time models confirm sustained group separation on the PACC occurring as early as 4 years when 81 observations in the elevated amyloid group and 106 observations in the normal amyloid group were observed. With models that impose more structure on the trajectories (continuous time models in Figure 1), group separation at the P = .003 level on the PACC occurred as early as 2 years (170 in the elevated amyloid group and 205 in the normal amyloid group). Although median follow-up was only 3.1 years, 25% of participants were observed beyond 4 years; at 8 years, there were 25 observations in the elevated amyloid group and 36 observations in the normal amyloid group. The multiple imputation analysis suggests that the conclusions regarding differences at year 10 were robust to substantial departures from the missing at random assumption used by the primary analysis models.
Fourth, randomized trials would be necessary to assess whether interventions based on these study findings affect the course of the disease. Fifth, tau PET imaging was not conducted, and there was limited collection of CSF tau. Only 83% of participants had lumbar punctures at baseline, which limited its utility in this analysis. However, ventricular volume, a robust measure of neurodegeneration, was selected as a covariate in several models. The amyloid group effect persisted when ventricular volume was included, suggesting an association between elevated brain amyloid and cognitive decline that was independent of neurodegeneration. Sixth, models did not include a covariate for baseline cognition. Instead, baseline cognition was modeled as an outcome variable so that the degree of separation at baseline is evident in the figures. Seventh, because analyses were not specified prior to beginning data collection in 2005 and because of the large number of comparisons and potential for type I error, all analyses should be considered as exploratory.
Conclusions
Exploratory analyses of a cognitively normal cohort followed up for a median of 3.1 years suggest that elevation in baseline brain amyloid level, compared with normal brain amyloid level, was associated with higher likelihood of cognitive decline, although the findings are of uncertain clinical significance. Further research is needed to assess the clinical importance of these differences and measure longer-term associations.
Acknowledgments
Dr Donohoe reports receipt of grants from the National Institutes of Health (NIH), the Alzheimer’s Association, the Michael J. Fox Foundation, and the W. Garfield Weston Foundation during the conduct of the study; and receipt of personal fees for scientific advisory board participation from Neurotrack and Eli Lilly outside the submitted work. Dr Sperling reports receipt of grants from NIH, Harvard Neurodiscovery Center, Fidelity Neurosciences, and the Alzheimer’s Association during the conduct of the study; personal fees from Lilly/Avid and Janssen Immunotherapy for site coinvestigator services; and personal fees from Bracket, Genentech, Sanofi, Biogen, Roche, Abbvie, Lundbeck, Otsuka, and Merck outside the submitted work for consultancy services. Dr Petersen reports receipt of grants from NIH during the conduct of the study; and personal fees from Hoffman-La Roche, Merck, Genentech, Biogen, and Eli Lilly outside the submitted work for consultancy services. Dr Weiner reports receipt of grants and personal fees from the Alzheimer’s Association; grants from NIH/National Institute on Aging (NIA), the US Department of Defense (DOD), the California Department of Public Health, Veterans Administration, US Army Medical Research Acquisition Activity, the Alzheimer’s Drug Discovery Foundation, Siemens, The Rosenberg Alzheimer’s Project, The Ray and Dagmar Dolby Family Fund, Connie and Kevin Shanahan, Global Alzheimer’s Platform Foundation, the Patient-Centered Outcomes Research Institute, General Electric, Monell Chemical Senses Center, Biogen Idec, The Stroke Foundation, Johnson & Johnson, Cogstate, and The Drew Foundation; and personal fees from Pfizer, Alzheon, Eli Lilly, Dolby Ventures, Janssen, Accera Pharma, the Alzheimer’s Drug Discovery Foundation, Avid Radiopharmaceuticals, Araclon, Merck, The Scienomics Group, AVOS Consulting, INC Research, Bioclinica, Howard University, Guidepoint, and Genentech outside the submitted work. Dr Aisen reports receipt of grants and personal fees from Eli Lilly; grants from Janssen, NIA, Foundation-NIH, the Alzheimer’s Association; and personal fees from NeuroPhage, Merck, Roche, Amgen, Abbvie, Pfizer, Novartis, Janssen, Lundbeck, Biogen, iPerian, Probiodrug, Anavex, Cohbar, Cytox, aTyr, and Avanir outside the submitted work. No other disclosures were reported.
Funding/Support: This work was supported by Biomarkers Across Neurodegenerative Disease (BAND-14-338179) grant from the Alzheimer’s Association, Michael J. Fox Foundation, Weston Brain Institute, ADNI, NIH (U01 AG024904), DOD (W81XWH-12-2-0012), NIA, the National Institute of Biomedical Imaging and Bioengineering, AbbVie, Alzheimer’s Drug Discovery Foundation, Araclon Biotech, BioClinica, Biogen, Bristol-Myers Squibb, CereSpir, Eisai, Elan Pharmaceuticals, Eli Lilly, EuroImmun, F. Hoffmann-La Roche, Genentech, Fujirebio, GE Healthcare, IXICO, Janssen Alzheimer Immunotherapy Research & Development, Johnson & Johnson Pharmaceutical Research & Development, Lumosity, Lundbeck, Merck, Meso Scale Diagnostics, NeuroRx Research, Neurotrack Technologies, Novartis Pharmaceuticals, Pfizer, Piramal Imaging, Servier, Takeda, Transition Therapeutics, and the Canadian Institutes of Health Research.
Glossary
CDR | Clinical Dementia Rating |
CSF | cerebrospinal fluid |
MCI | mild cognitive impairment |
MMSE | Mini-Mental State Examination |
PACC | Preclinical Alzheimer Cognitive Composite |
PET | positron emission tomography |
Footnotes
Group Information: A complete listing of the Alzheimer’s Disease Neuroimaging Initiative Investigators can be found at: http://adni.loni.usc.edu/wp-content/uploads/how_to_apply/ADNI_Acknowledgement_Listpdf.
Author Contributions: Dr Donohue and Mr Sun had full access to all of the data in the study and take responsibility for the integrity of the data and the accuracy of the data analysis.
Concept and design: Donohue, Sperling, Aisen.Acquisition, analysis, or interpretation of data: Donohue, Petersen, Sun, Weiner, Aisen.
Drafting of the manuscript: Donohue, Petersen, Sun, Aisen.
Critical revision of the manuscript for important intellectual content: Donohue, Sperling, Petersen, Weiner, Aisen.
Statistical analysis: Donohue, Sun.
Obtained funding: Donohue, Aisen.
Administrative, technical, or material support: Petersen, Aisen.
Supervision: Donohue, Sperling, Petersen, Aisen.
Conflict of Interest Disclosures: All authors have completed and submitted the ICMJE Form for Disclosure of Potential Conflicts of Interest.
The Alzheimer’s Disease Neuroimaging Initiative: Data used in the preparation of this article were obtained from the Alzheimer’s Disease Neuroimaging Initiative (ADNI) database (http://adni.loni.usc.edu). As such, the investigators within the ADNI contributed to the design and implementation of ADNI and/or provided data but did not participate in analysis or writing of this report. A complete listing of ADNI investigators can be found at: http://adni.loni.usc.edu/wp-content/uploads/how_to_apply/ADNI_Acknowledgement_List.pdf.
Additional Contributions: The authors are grateful to the ADNI study volunteers and their families.
Role of the Funder/Sponsor: [Name] had no role in the design and conduct of the study; collection, management, analysis, and interpretation of the data; preparation, review, or approval of the manuscript; and decision to submit the manuscript for publication.
Contributor Information
Michael C. Donohue, Alzheimer’s Therapeutic Research Institute, Department of Neurology, University of Southern California, San Diego.
Reisa A. Sperling, Center for Alzheimer Research and Treatment, Brigham and Women’s Hospital, Harvard Medical School, Boston, Massachusetts. Department of Neurology, Brigham and Women’s Hospital, Harvard Medical School, Boston, Massachusetts.
Ronald Petersen, Massachusetts General Hospital, Boston (Sperling); Department of Neurology, Mayo Clinic, Rochester, Minnesota.
Chung-Kai Sun, Alzheimer’s Therapeutic Research Institute, Department of Neurology, University of Southern California, San Diego.
Michael W. Weiner, Center for Imaging of Neurodegenerative Diseases, University of California-San Francisco. San Francisco VA Medical Center, San Francisco, California.
Paul S. Aisen, Alzheimer’s Therapeutic Research Institute, Department of Neurology, University of Southern California, San Diego.
References
Full text links
Read article at publisher's site: https://doi.org/10.1001/jama.2017.6669
Read article for free, from open access legal sources, via Unpaywall:
https://jamanetwork.com/journals/jama/articlepdf/2631529/jama_donohue_2017_oi_170051.pdf
Citations & impact
Impact metrics
Article citations
Blood Biomarkers to Detect Alzheimer Disease in Primary Care and Secondary Care.
JAMA, 332(15):1245-1257, 01 Oct 2024
Cited by: 6 articles | PMID: 39068545
Lewy body pathology exacerbates brain hypometabolism and cognitive decline in Alzheimer's disease.
Nat Commun, 15(1):8061, 14 Sep 2024
Cited by: 1 article | PMID: 39277604 | PMCID: PMC11401923
Screening for early Alzheimer's disease: enhancing diagnosis with linguistic features and biomarkers.
Front Aging Neurosci, 16:1451326, 23 Sep 2024
Cited by: 0 articles | PMID: 39376506 | PMCID: PMC11456453
Cognitive modeling of the Mnemonic Similarity Task as a digital biomarker for Alzheimer's disease.
Alzheimers Dement, 20(10):6935-6947, 06 Sep 2024
Cited by: 4 articles | PMID: 39239893 | PMCID: PMC11485396
Tau Positron Emission Tomography for Predicting Dementia in Individuals With Mild Cognitive Impairment.
JAMA Neurol, 81(8):845-856, 01 Aug 2024
Cited by: 0 articles | PMID: 38857029
Go to all (228) article citations
Data
Data behind the article
This data has been text mined from the article, or deposited into data resources.
BioStudies: supplemental material and supporting data
Similar Articles
To arrive at the top five similar articles we use a word-weighted algorithm to compare words from the Title and Abstract of each citation.
Cognitive and neuroimaging features and brain β-amyloidosis in individuals at risk of Alzheimer's disease (INSIGHT-preAD): a longitudinal observational study.
Lancet Neurol, 17(4):335-346, 27 Feb 2018
Cited by: 98 articles | PMID: 29500152
Amyloid and Tau Prediction of Cognitive and Functional Decline in Unimpaired Older Individuals: Longitudinal Data from the A4 and LEARN Studies.
J Prev Alzheimers Dis, 11(4):802-813, 01 Jan 2024
Cited by: 0 articles | PMID: 39044488 | PMCID: PMC11266444
Alzheimer's Disease Biomarkers and Future Decline in Cognitive Normal Older Adults.
J Alzheimers Dis, 60(4):1451-1459, 01 Jan 2017
Cited by: 38 articles | PMID: 29036824 | PMCID: PMC5773095
High-clearance anti-amyloid immunotherapies in Alzheimer's disease. Part 1: Meta-analysis and review of efficacy and safety data, and medico-economical aspects.
Rev Neurol (Paris), 178(10):1011-1030, 29 Sep 2022
Cited by: 27 articles | PMID: 36184326
Review
Funding
Funders who supported this work.
NIA NIH HHS (4)
Grant ID: U01 AG024904
Grant ID: U19 AG010483
Grant ID: R01 AG049750
Grant ID: K24 AG035007