Abstract
Free full text

50 years of spermatogenesis: Sertoli cells and their interactions with germ cells
Abstract
The complex morphology of the Sertoli cells and their interactions with germ cells has been a focus of investigators since they were first described by Enrico Sertoli. In the past 50 years, information on Sertoli cells has transcended morphology alone to become increasingly more focused on molecular questions. The goal of investigators has been to understand the role of the Sertoli cells in spermatogenesis and to apply that information to problems relating to male fertility. Sertoli cells are unique in that they are a nondividing cell population that is active for the reproductive lifetime of the animal and cyclically change morphology and gene expression. The numerous and distinctive junctional complexes and membrane specializations made by Sertoli cells provide a scaffold and environment for germ cell development. The increased focus of investigators on the molecular components and putative functions of testicular cells has resulted primarily from procedures that isolate specific cell types from the testicular milieu. Products of Sertoli cells that influence germ cell development and vice versa have been characterized from cultured cells and from the application of transgenic technologies. Germ cell transplantation has shown that the Sertoli cells respond to cues from germ cells with regard to developmental timing and has furthered a focus on spermatogenic stem cells and the stem cell niche. Very basic and universal features of spermatogenesis such as the cycle of the seminiferous epithelium and the spermatogenic wave are initiated by Sertoli cells and maintained by Sertoli-germ cell cooperation.
Introduction
In 1865 Enrico Sertoli published his original paper describing the cell that now bears his name and he speculated that this cell was “linked to the production of spermatozoa.” The content of the paper was wholly descriptive and discussed the cellular extensions and contacts with adjacent cells. More than 100 years later in 1967 when the journalBiology of Reproduction was born, there were approximately 35 papers about the Sertoli cells published. In the past 50 years,Biology of Reproduction has contributed in major ways to advancing our understanding of Sertoli cells with nearly 800 publications with the term “Sertoli” in the title or in the abstract. For this review, I have identified representative publications fromBiology of Reproduction that have made contributions to the field (Table 1). Note that I attempted to highlight approximately one to two publications per year and the choice was subject to my bias and to space limitations. I am sure many worthy contributions may have been left out of thisTable 1 and that is why I designated these publications as “representative.”
Table 1.
Representative publications inBiology of Reproduction relating to Sertoli cells and the interactions with germ cells (out of more than 800 total with Sertoli in title or abstract).
Morphology | ||
1969 | Roosen-Runge, E. C. | Comparative aspects of spermatogenesis [143] |
1970 | Dym, M. and Fawcett, D. W. | The blood–testis barrier in the rat and the physiological compartmentation of the seminiferous epithelium [144] |
1971 | Dym, M. and Fawcett, D. W. | Further observations on the numbers of spermatogonia, spermatocytes, and spermatids connected by intercellular bridges in the mammalian testis [145] |
1978 | Solari, A., and Fritz, I. | The ultrastructure of immature Sertoli cells. Maturation-like changes during culture and the maintenance of mitotic potentiality [146] |
1986 | Morales, C., Clermont, Y., Nadler, N. | Cyclic endocytic activity and kinetics of lysosomes in Sertoli cells of the rat: a morphometric analysis [147] |
1993 | deFranca, L., Ghosh, S., Ye, S., et al. | Surface and surface-to-volume relationships of the Sertoli cell during the cycle of the seminiferous epithelium in the rat [148] |
2015 | Lyon, K., Bosseboeuf, E., Vogl, A. | An alternative model of tubulobulbar complex internalization during junction remodeling in the seminiferous epithelium of the rat testis [149] |
2017 | Geyer, C. B. | A historical perspective on some "new" discoveries on spermatogenesis from the laboratory of Enrico Sertoli in 1878 [150] |
Cultured cells | ||
1971 | Steinberger, A. and Steinberger, E. | Replication pattern of Sertoli cells in maturing rat testis in vivo and in organ culture [151] |
1981 | Robinson, R. and Fritz, I. | Metabolism of glucose by Sertoli cells in culture [152] |
1982 | Mita, M. and Hall, P. | Metabolism of round spermatids from rats: lactate as the preferred substrate [153] |
2003 | Nagano, M., Ryu, B., Brinster, C., et al | Maintenance of mouse male germ line stem cells in vitro [154] |
2009 | DeGent, K., McKinnell, C., Williams, A., et al | Organotypic cultures of prepubertal mouse testes: a method to study androgen action in Sertoli cells while preserving their natural environment [155] |
Hormone response | ||
1976 | Means, A. R., Fakunding, J., Tindall D. | Follicle stimulating hormone regulation of protein kinase activity and protein synthesis in testis [156] |
1977 | Dym, M. and Raj, H. G. | Response of adult rat Sertoli cells and Leydig cells to depletion of luteinizing hormone and testosterone [157] |
1978 | Dorrington, J., Fritz, I., Armstrong, D. | Control of testicular estrogen biosynthesis [158] |
1978 | Kotite, N., Nayfeh, S., French, F. | FSH and androgen regulation of Sertoli cell function in the immature rat [159] |
1978 | Steinberger, A., Hintz, M., Heindel, J. | Changes in cyclic AMP responses to FSH in isolated rat Sertoli cells during sexual maturation [160] |
1989 | Russell, L. and Steinberger, A. | Sertoli cells in culture: views from the perspectives of an in vivoist and an in vitroist [161] |
1991 | Sanborn, B., Caston, L., Chang, L., et al | Regulation of androgen receptor mRNA in rat Sertoli and peritubular cells [162] |
1994 | O’Brien, D., Welch, J., Fulcher K., et al | Expression of mannose 6-phosphate receptor messenger ribonucleic acids in mouse spermatogenic and Sertoli cells [163] |
1994 | Cooke, P., Zhao, Y. Bunick, D. | Triiodothyronine inhibits proliferation and stimulates differentiation of cultured neonatal Sertoli cells: possible mechanism for increased adult testis weight and sperm production induced by neonatal goitrogen treatment [164] |
1996 | Meachem, S., McLachlan, R., et al | Neonatal exposure of rats to recombinant follicle stimulating hormone increases adult Sertoli and spermatogenic cell numbers [165] |
2011 | Toocheck, C., Clister, T., Shupe, J. et al | Mouse spermatogenesis requires classical and nonclassical testosterone signaling [166] |
Interactions with other cells | ||
1979 | Handel, M., and Eppig, J. | Sertoli cell differentiation in the testes of mice genetically deficient in germ cells [167] |
1980 | Tung, P and Fritz, I. | Interactions of Sertoli cells with myoid cells in vitro [168] |
1995 | Griswold, M. | Interactions between germ cells and Sertoli cells in the testis [169] |
2001 | Syed,V. and Hecht, N | Selective loss of Sertoli cell and germ cell function leads to a disruption in Sertoli cell–germ cell communication during aging in the Brown Norway rat [170] |
2011 | Oatley, M., Racicot, K., Oatley, J. | Sertoli cells dictate spermatogonial stem cell niches in the mouse testis [77] |
Gene expression | ||
1982 | Kissinger C., Skinner M., Griswold M. | Analysis of Sertoli cell-secreted proteins by two-dimensional gel electrophoresis [48] |
1982 | Skinner, M., and Griswold, M. | Secretion of testicular transferrin by cultured Sertoli cells is regulated by hormones and retinoids [171] |
1983 | Wright, W., Parvinen, M., Musto, N. et al | Identification of stage-specific proteins synthesized by rat seminiferous tubules [172] |
1984 | Sylvester, S., and Griswold, M. | A sulfated glycoprotein synthesized by Sertoli cells and by epididymal cells is a component of the sperm membrane [173] |
1986 | Borland, K., Muffly, K., Hall, P. | Production of components of extracellular matrix by cultured rat Sertoli cells [174] |
1986 | Morales, C., Clermont, Y., Nadler, N. | Cyclic endocytic activity and kinetics of lysosomes in Sertoli cells of the rat: a morphometric analysis [147] |
1986 | Wright, W., and Luzarraga, M. | Isolation of cyclic protein-2 from rat seminiferous tubule fluid and Sertoli cell culture medium [59] |
1987 | Morales, C., Hugly, S., Griswold, M. | Stage-dependent levels of specific mRNA transcripts in Sertoli cells [175] |
1990 | Zabludoff, S., Karzai, A. Wright, W. | Germ cell-Sertoli cell interactions: the effect of testicular maturation on the synthesis of cyclic protein-2 by rat Sertoli cells [176] |
2003 | Lui, W., Mruk, D., Lee, W., Cheng, C. | Sertoli cell tight junction dynamics: their regulation during spermatogenesis [62] |
2008 | Boyer, A., Hermo, L., Paquet, M., et al | Seminiferous tubule degeneration and infertility in mice with sustained activation of WNT/CTNNB1 signaling in Sertoli cells [177] |
2013 | Ortogero, N., Hennig, G., Langille, C., et al | Computer-assisted annotation of murine Sertoli cell small RNA transcriptome [178] |
2014 | Evans, E., Hogarth, C., Mitchell, D., et al | Riding the spermatogenic wave: profiling gene expression within neonatal germ and sertoli cells during a synchronized initial wave of spermatogenesis in mice [92] |
Vitamin A | ||
1990 | van Pelt, A., and de Rooij, D. | Synchronization of the seminiferous epithelium after vitamin A replacement in vitamin A-deficient mice [116] |
1995 | Tsuruta, J., and O’Brien, D. | Sertoli cell–spermatogenic cell interaction: the insulin-like growth factor-II/cation-independent mannose 6-phosphate receptor mediates changes in spermatogenic cell gene expression in mice [179] |
2016 | Agrimson, K., Onken, J., Mitchell, D., et al | Characterizing the spermatogonial response to retinoic acid during the onset of spermatogenesis and following synchronization in the neonatal mouse testis [130] |
Transplantation | ||
1998 | Franca, L., Ogawa, T. Avarbock, M., et al | Germ cell genotype controls cell cycle during spermatogenesis in the rat [75] |
1998 | Parreira, G., Ogawa, T., Avarbock, M., et al | Development of germ cell transplants in mice [180] |
2003 | Shinohara,T., Orwig, K., Avarbock, M., et al | Restoration of spermatogenesis in infertile mice by Sertoli cell transplantation [181] |
Gene knockouts | ||
2000 | Krishnamurthy, H., Danilovich, N., et al | Qualitative and quantitative decline in spermatogenesis of the follicle-stimulating hormone receptor knockout (FORKO) mouse [182] |
2007 | Sridharen, S., Simon, L., Meling, D., et al | Proliferation of adult Sertoli cells following conditional knockout of the Gap junctional protein GJA1 (connexin 43) in mice [183] |
2011 | Meng, J., Greenlee, A., Taub, C., Braun R. | Sertoli cell-specific deletion of the androgen receptor compromises testicular immune privilege in mice [184 ] |
2017 | Zhao, J., Zhao, J., Xu, G., Wang, Z., et al | Deletion of Spata2 by CRISPR/Cas9n causes increased inhibin alpha expression and attenuated fertility in male mice [138] |
The interest in understanding Sertoli cells and their interactions with germ cells has continued to grow worldwide with around 250 papers in 1990 and nearly 500 papers per year since 2012. The topics of the papers until and in the 1960s dealt almost entirely with the complex morphology of Sertoli cells in a variety of species. While the description of the morphology was greatly refined from that of the original Sertoli manuscript, there was very little insight into the biological function of these cells. The increased interest in the Sertoli cells and the morphology was generated by the availability and improved quality of electron microscopes.
Similar to the influence of the electron microscope on the interest in morphology, it was advances in a variety of technologies that fueled the increased interest in the Sertoli cells. Techniques such as primary cell culture, mRNA analysis by gene arrays and RNAseq, germ cell transplantation, flow cytometry, and targeted gene deletions have led to a greater understanding of the complexity of these cells over the last 50 years. Information documenting progress in our insight into the morphology and function of Sertoli cells have been documented in three books:The Sertoli Cell, Cache River Press, edited by Lonnie D. Russell and Michael D. Griswold in 1993 [1];Sertoli Cell Biology vol I, Elsevier, edited by Michael K. Skinner and Michael D. Griswold in 2005 [2]; andSertoli Cell Biology vol II, Elsevier, edited by Michael D. Griswold in 2015 [3]. In addition, a review that celebrated the 150th anniversary of the original description of the Sertoli cells was recently published and within it were listed both the significant milestones in research and major review articles on the Sertoli cell [4]. In this review, I will draw from those volumes and from additional important papers to highlight the major advances in the last 50 years and I will concentrate on basic science information derived from and applied to mammalian spermatogenesis. The important role of Sertoli cells in the formation of the testis in sex determination, spermatogenesis in nonmammals, and clinical issues will be reviewed by others.
Understanding the morphology
The Sertoli cells change structure during development and during the cycle of the seminiferous epithelium throughout their lifetime. They interact with basement membranes, and make and break junctions with each other and with multiple types of syncytial germ cells. They secrete fluids and proteins and they phagocytize unneeded parts of spermatids [4]. The Sertoli cells have proven to be among the most complicated and dynamic cell structures known in biology [4]. By 1967 many investigators had contributed to understanding the complex morphology of the Sertoli cells with focus on the changing ultrastructure during development and their cyclic interaction with germ cells [5–7]. In the mid-1960s and 1970s the field was dominated by the laboratories of Don Fawcett at Harvard University and Yves Clermont at McGill University. Among many contributions during this period, these investigators described the ultrastructure of junctions in the testis [7] and the relationships between germ cells and Sertoli cells within the stages of the cycle of the seminiferous epithelium in humans [8,9]. From these two leaders came many disciples who eventually continued the ultrastructure studies in their own laboratories. One of the most productive disciples was Lonnie Russell who was trained in the Clermont laboratory. Dr Russell was an evangelistic anatomist and he stayed true to his cause. He described the desmosome-like junctions between Sertoli and germ cells [10], the ectoplasmic specializations in Sertoli cells [11], and the tubulobulbar complexes formed between Sertoli cells and spermatids [12]. In three publications in theAmerican Journal of Anatomy, Lonnie Russell for the first time showed the three-dimensional form of Sertoli cells to everyone [13–15]. This breakthrough was accomplished based on observations of the serial sections of a single rat stage V Sertoli cell. A plastic model was built and a drawing based on the model completed the first depiction of a Sertoli cell in vivo. This drawing showed deep clefts where elongated spermatids were located, cup-like processes where round germ cells were held, and long thin apical processes between spermatids. The basal aspect of adjacent Sertoli cells was held by tight junctions in a pentagonal or hexagonal array. From this model and from observations using other approaches came morphometric data on many aspects of Sertoli–Sertoli interaction and Sertoli–germ cell interactions [13–16] (Figure 1)
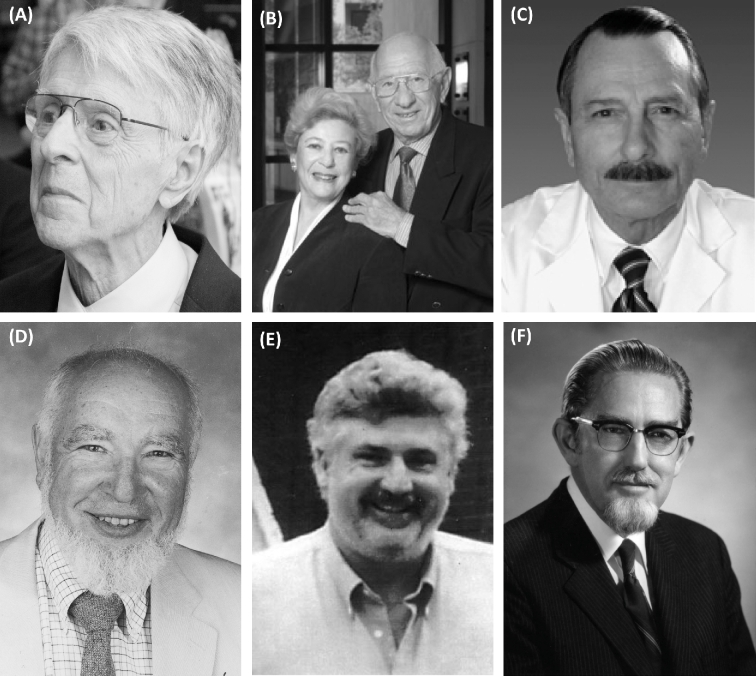
Innovators and pioneers in the studies relating to Sertoli cells and their interactions with germ cells. Yves Clermont (A) from McGill University defined the stages of the cycle of the seminiferous epithelium and cellular changes associated with it. Anna and Emil Steinberger (B) from Baylor College of Medicine pioneered in vitro studies on testis tissue and along with Irving Fritz (D) from the University of Toronto developed the methods for primary culture of Sertoli cells. Ralph Brinster (C) from the University of Pennsylvania reported the first successful transplantation of testicular stem cells. Lonnie Russell (E) did the first reconstruction of a Sertoli cell from serial microscopic sections and made major contributions regarding the microanatomy of Sertoli cells. Don Fawcett (F) from Harvard University who published extensively on male reproductive cellular anatomy.
The anatomy and ultrastructure data produced on Sertoli cells and germ cells via the transmission electron microscope from 1967 to present day are extensive and detailed. These data have reinforced the concept of the Sertoli cell as an extremely complex and dynamic cell. In addition, the knowledge of cellular anatomy and ultrastructure is a major resource for interpreting molecular-based procedures such as immunocytochemistry and in situ hybridization. In the 1990s the confocal microscope came into more general use for examination of testis morphology and for localization of specific antibodies labeled with fluorescent reporters. A clear example of how these technologies moved the field forward can be seen in studies of the blood–testis barrier (BTB). In 1967 Flickinger and Fawcett described in detail the structures of the occluding junctions between Sertoli cells as revealed in the transmission electron microscope [7]. Lonnie Russell expanded on what had ultimately been termed “Sertoli cell tight junctions” and postulated that the spermatocytes moved through the junctions by formation of new junctional complexes basal to the germ cells essentially forming an intermediate structure of spermatocytes with junctions above and beneath [17]. In the past 25 years many of the molecular components of the junctions have been identified (for a review, see [18]). Then, in 2012, Smith and Braun used antibodies to the proteins comprising the junctions and visualized with the confocal microscope how cysts of spermatocytes transit the BTB by forming a network of temporary compartments bounded by old and new tight junctions [19].
Isolating the Sertoli cells and germ cells
For the examination of morphology, the use of whole testis tissue was informative but for molecular and functional questions the isolation of individual cell types from the complex testicular tissue was necessary. The first practical isolation and culture of relatively pure rat Sertoli cells was published from several independent laboratories in 1975 [20–22]. The technique was relatively simple and involved stripping the interstitial cells from separated seminiferous tubules with trypsin. The peritubular myoid cells were then removed by collagenase digestion, and the tubule fragments containing Sertoli cells and germ cells were plated in culture dishes whereby the Sertoli cells attached and formed a monolayer and the germ cells were released into the medium [23]. These primary cultures of rodent Sertoli cells could be maintained in culture in minimal medium for days or weeks and were used to examine a number of properties that had been attributed to Sertoli cells in vivo [24]. The development of techniques for the primary culture of Sertoli cells was a major innovation and stimulated the interest of many physiologists, endocrinologists and cell biologists. The culture system was used to examine the effects of FSH, androgens and other hormones and growth factors and toxicants on the Sertoli cells [23]. Despite these many advances, the primary culture system has limitations. The techniques were optimal for Sertoli cells from prepubertal rodents and the application to the culture of cells from the adult was difficult (Figure 2).
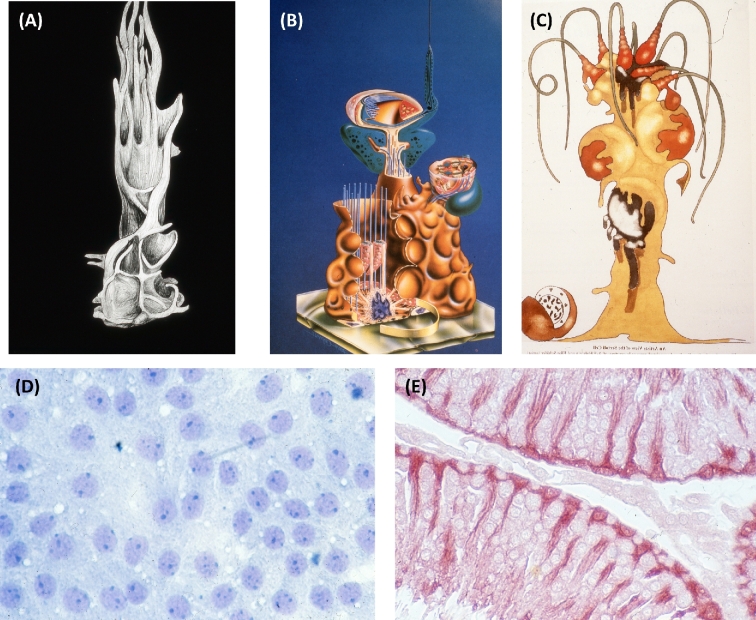
Representations of Sertoli cells from the archives of the author. (A) The artistic representation of a Sertoli cell from the model built by Lonnie Russell from serial sections in the electron microscope [13,14]. (B) Diagram illustrating the filaments and microtubules in mammalian Sertoli cells was on the cover of the first book on Sertoli cells [1]. (C) Artistic representation of Sertoli cells and associated germ cells by Holstein and Schafer and first printed inThe Sertoli Cell [1]. (D) Field of Sertoli cells in culture from testis of 20-day old rat showing epithelial nature of cells. (E) Cross section of rat seminiferous tubules stained by immunocytochemistry with antibody to clusterin highlighting Sertoli cells in the tissue.
The different developmental stages of germ cells were isolated by sedimentation at unit gravity [25–27]. The separation of these stages depended on the sedimentation of each germ cell type through a gradient of serum albumin and one limitation was the time required for the sedimentation to occur. This technique termed Staput was used for the isolation of sufficient germ cells to analyze the biochemistry or molecular biology and provided relatively pure populations of some stages [28]. A further refinement of the principles of Staput was the development of centrifugal elutriation that subjected cells to a fluid flow opposite to their sedimentation in a special chamber in a centrifuge. This method allowed for larger numbers of germ cells to be recovered and led to studies on protamines and transition proteins [29,30]. Magnetic-activated cell sorting where antibodies to cell surface proteins are coupled to magnetic beads has also been used to isolate spermatogonia from rodents and primates [31,32]. In addition, the development of transgenic mice that express fluorescent proteins in specific cell types has led to the isolation of both germ cells and Sertoli cells by fluorescent-activated cell sorting.
Products of Sertoli cells
One of the developments that followed the ability to maintain Sertoli cells in serum-free culture was the search for products of Sertoli cells that could be involved in their ability to sustain spermatogenesis. Initially, there was a great deal of interest in the androgen binding protein (ABP) that was found in the efferent duct fluid and epididymal fluid [33]. ABP binds androgens with very high affinity, can be readily assayed, and was proposed to be involved in the action of testosterone in the testis. When it was demonstrated that the production of ABP was via Sertoli cells under control of FSH both in vivo and in culture, the interest by investigators and funding agencies intensified [33–37]. Between 1975 and 1980 there were more than 100 papers published from the major laboratories in the field dealing with ABP. The secretion of ABP was used as a parameter of the functional state of Sertoli cells in culture [38]. Cultures could be treated with hormones, drugs, toxins, heat, germ cells, or peritubular cells, and the response of Sertoli cells was determined by the amount of ABP secreted into the culture medium [39]. There was published evidence that spermatogenic cells could internalize ABP from Sertoli cells [40] and that overexpression of ABP increased the number of primary spermatocytes [41]. Interest in ABP began to wane when it was shown that the genes for serum hormone binding globulin and ABP were identical and that ABP was widely expressed in other tissues [42]. In addition, it was later shown that the human Sertoli cells were unable to synthesize ABP but a similar transcript was made in human germ cells [43].
Because of the ease of culturing rat Sertoli cells in serum-free culture, the search for products of the Sertoli cells secreted into the culture medium continued. Using a bioassay, an FSH-inhibiting activity was found in the culture medium [44]. This finding eventually led to an entire field of study focused on inhibins, activins, and follistatins [45–47]. Attempts were made to characterize and identify the proteins secreted into the medium of the cultures. Radioactive precursors were added to the medium, and the proteins were separated by one- and two-dimensional electrophoresis and visualized by fluorography [48,49]. From these early experiments, it was shown that Sertoli cells secrete serum proteins such as transferrin and ceruloplasmin as well as two major glycoproteins first termed sulfated glycoproteins 1 and 2 (SGP-1 and SGP-2) [50–52]. It was later shown that SGP-1 was analogous to a protein that bound sphingolipids named prosaposin [53] and SGP-2 was renamed clusterin and was found in nearly every tissue in the body [54]. Over the last 25 years, a large number of the Sertoli cell gene products have been reported and they can be generally classified into several groups based on their known biochemical properties [55,56]. First, binding and transport proteins such as metal ion binding proteins and lipid binding proteins are made and secreted by Sertoli cells in high abundance [57]. It has been proposed that this is necessary in order to move essential materials around the tight junctions and make them available to the germ cells. Second, several proteases and protease inhibitors that may be essential in tissue remodeling and spermatid release are made and secreted into the culture medium. These include essential proteases such as cathepsin L (cyclic protein -2) [58–61]. Third, a number of products of Sertoli cells contribute to the basement membrane or to intercellular junctions [62]. A fourth category of the products of Sertoli cells are the hormones and growth factors that influence spermatogenesis directly or indirectly. Historically, this includes insulin-like growth factors, inhibin and activin, and Mullerian inhibiting substance [63]. Some secretions in this category directly influence germ cell development including glial cell-line-derived neurotrophic factor (GDNF) [64] and retinoic acid (RA) [65].
One of the first proposed functions of a secretion product from Sertoli cells involved the synthesis of transferrin and iron transport [57,66–68]. It was proposed that the Sertoli cells were part of an iron shuttle system that was designed to transport ferric ions around the tight junctions to supply germ cells in the adluminal compartment. This model was considered a classic example of the “nurse cell” function of Sertoli cells. This model proposed a mechanism for moving iron (Fe+3) through the BTB for use primarily in meiotic and mitotic cell divisions in spermatogonia and spermatocytes, and mitochondriogenesis in spermatids. Ferric ions are required for many cellular functions but must be transported bound to specific transport proteins such as transferrin. As more information became available about proteins involved in iron transport and storage and their localization in the testis, this model was revised to include protection of the testis from iron overload as a result of digestion of residual bodies [69]. It was proposed that there was an autonomous iron cycle within the seminiferous epithelium and that primary spermatocytes took up iron on transferrin made by Sertoli cells and the bulk of the iron was returned to the Sertoli cells via residual bodies. Excess iron could be secreted out the basal surface of the Sertoli cells bound to transferrin. The “nurse cell” function of Sertoli cells was to deliver iron to spermatocytes but also remove and/or recycle iron from residual bodies. This model suggests that Sertoli cells have a role in detoxifying the testis from the accumulation of excess iron. It has been suggested that this is still a “nurse cell” function that is equivalent to emptying the bedpans. It is likely that there are several such “recycling and waste management” functions in the role of the Sertoli cells [4,70].
Germ cell transplantation and Sertoli cell function
Perhaps the most innovative and surprising development in testis research over the past 50 years was the successful transplantation of germ cells from a mouse donor testis into the testis of a recipient mouse [71]. This technique was developed and characterized in the laboratory of Dr Ralph Brinster at the University of Pennsylvania. For the first time, it was demonstrated that germ cells can be transferred from one animal to another and between species. Stem cells could be frozen or cultured and successfully implanted into recipient testes [72]. The technique was also used experimentally in rodents to assay spermatogenic defects, correct genetic male infertility, and generate transgenic animals [73]. Besides having potential clinical applications, this spermatogonial transplantation stimulated research in spermatogonial stem cells because it serves as a functional assay for these cells. In addition, spermatogonial transplantation enabled the identification of factors and conditions that are basic to germ cell and Sertoli cell interactions [74]. By transplanting rat germ cells into mouse testes, it was shown that the germ cells controlled the timing of the cycle of the seminiferous epithelium [75]. The spermatogonial stem cell transplantation technique was used to examine whether murine germ cells required functional testosterone receptors to complete normal spermatogenesis [76]. Germ cells from testicular feminized mice that lacked testosterone receptors were transplanted into the seminiferous tubules of azoospermic mice expressing functional androgen receptors. When recipient testes were analyzed between 110 and 200 days following transplantation, multiple colonies of qualitatively normal donor-derived spermatogenesis were seen in each recipient testis, thereby establishing that Sertoli cells but not germ cells must have functional androgen receptors to complete spermatogenesis.
In tissues where stem cells are found, they occupy a niche where fate decisions are influenced by a growth factor milieu. In the testis, the spermatogenic stem cells (SSC) occupy a niche that can be investigated using the stem cells transplantation techniques. Oatley et al. used polythiouracil-induced transient hypothyroidism to increase the number of Sertoli cells in the murine testis and found that there was a threefold increase in both the number of stem cells and the number of niches in the treated animals [77]. The results of this experiment directly showed that the number of Sertoli cells determined the number of SSCs and the number of niches in the testis. Additional studies also showed that there was an age-related decline in the niches [78]. While the number and quality of stem cells is important for the maintenance of spermatogenesis, the presence of niches is also essential. These studies underscored the important relationship of germ cells and Sertoli cells in the maintenance of successful spermatogenesis.
Sertoli cell junctions and immune privilege
One area of interest since the early days of electron microscopy is characterization of the numerous junctions that Sertoli cells make with each other and with different germ cells. In the past several years, the biochemical components of many of these junctions have been characterized and have recently been reviewed [4,79]. The junctional complexes between Sertoli cells and germ cells likely provide communication between cells and are involved in the orderly movement of germ cells within the seminiferous epithelium. For example, the spermatids move basally and apically during various stages of the cycle of the seminiferous epithelium. One of the junctions that is unique to the testis is the BTB or Sertoli cell barrier (SCB). This is a physical barrier that forms about the time of the onset of meiosis and separates the spermatogonia in the basal aspect of the seminiferous epithelium from the spermatocytes and spermatids in the apical aspect. The function of the BTB/SCB has long been thought to be immunoprotective of the novel proteins expressed by spermatocytes and spermatids. These proteins are expressed after immunological self-tolerance has been established and could provoke both a humoral and cellular immune response [4]. While the BTB/SCB sequesters the spermatocytes and spermatids to prevent an immune response, it appears that there is much more to the story. For example, there is considerable evidence that Sertoli cells modulate the immune response by secreting immunomodulatory factors [80]. The direct evidence for this comes from studies where Sertoli cells survive transplantation across immunological barriers and in turn protect cells that are mixed with them such as pancreatic islet cells [4].
Evidence that the immune privilege status of the testis is more complicated than originally thought has recently come from the laboratory of Prof. Ken Tung at the University of Virginia [81]. A long-time pioneer in the study of testis immunology, Professor Tung and colleagues, has shown that while some germ cell antigens are sequestered, some move out of the tubule through the basal part of the Sertoli cells. These residual body- derived antigens maintained systemic tolerance as a result of the formation of Treg-dependent complexes. This result suggests a new paradigm of selective testis–antigen sequestration and tolerance, and it indicates that the immune protection for germ cell antigens in the testis depends on two processes: first, systemic tolerance involving antigen-specific Tregs and second, local mechanisms, including the BTB/SCB. The major elements of this new paradigm include the findings of Tregs as an important and essential peripheral tolerance mechanism, the immunogenicity of the residual bodies, and most importantly, the discovery of a new pathway of molecular transport from inside the seminiferous tubules into the testis interstitial space. These results are changing our ideas about how the testicular immune-privileged environment is maintained.
Gene expression in Sertoli cells and germ cells
Research into gene expression in testicular cells was stimulated by the hope that understanding the expressed mRNA would lead to functional insights. Both Sertoli cells and germ cells are very transcriptionally dynamic. The Sertoli cell gene expression changes during development, as a result of interaction with germ cells during the cycle of the seminiferous epithelium, and because of the action of growth factors and hormones. Likewise, the germ cell gene expression changes with every developmental stage during spermatogenesis. Much of the dynamic transcription in these cells is maintained throughout the reproductive lifetime of the organism. Initially, gene expression analysis in the testis involved a single gene approach with a targeted question. The techniques of PCR, northern blots, RNase protection, and in situ hybridization provided new information on a wide variety of specific Sertoli cell and germ cell transcripts. After 1998, the development of high-throughput approaches that could analyze thousands of genes in a single experiment using microarrays was available and within the past several years RNAseq has supplanted even this technology. One of the first applications of the array technology was the analysis of the action of FSH on transcripts from rat Sertoli cells in culture [82]. The goal of this study was to identify transcripts whose abundance was altered significantly as a result of FSH action. The Affymetrix GeneChips at that time had limited coverage of known genes but the results showed that the Sertoli cells in culture from 20 day old rats were very transcriptionally active and FSH ultimately altered the expression of hundreds of genes [83]. The results from this study showed both the benefit and the bane of global approaches to transcriptional analysis when hundreds of transcripts are altered. Often, so many transcripts are altered it is difficult to determine primary from secondary effects.
About this time there was a major switch in testis research from the rat to the mouse. The rat had been the rodent of choice because of the larger amount of material available but as transcriptome analysis became accessible and useful, many investigators chose to utilize the mouse because of the useful genetics and availability of mutants. For example, the hypogonadal (Hpg) mouse was used to examine the action of FSH and testosterone on the transcriptome of the testis of mice with no FSH or LH [84,85]. The major finding from these approaches was that the primary effect of FSH was a steady-state increase in the levels of many mRNAs in the testis of the hpg mice. In contrast, the primary action of testosterone in the Hpg mice was to decrease the abundance of many mRNAs in the testis suggesting that transcription of many mRNAs was repressed. Two studies investigated the changes in the transcriptome during postnatal development and the adult testis [86,87]. In one study, it was possible, given the data set profiles, to infer which transcripts represented germ cells or Sertoli cells [87]. In the other studies, the investigators used transcription profiles from cultured Sertoli cells along with the entire data set to identify 351 genes expressed only in the male germline at the time of the onset of meiosis [86]. Both studies concluded that there was an amazing number of genes expressed solely by the male germ cells late in development. More recently, the array technology was applied to mice with cell-specific gene deletions. Specifically, the Cre/Lox tools were used to generate mice where the gene for the androgen receptor was deleted in the Sertoli cells [88,89]. The approaches identified a number of genes that appeared to be androgen regulated and showed claudin 3 expression in Sertoli cells was androgen regulated and was a transient component of the BTB. The net result was the identification of a mechanism that Sertoli cells could regulate the permeability of the BTB via androgen action [89].
One of the issues in the studies on gene expression in whole testis tissue is that there is a myriad of cells that can contribute to the total transcript profile. One of the solutions is to isolate and purify specific cell types prior to analysis. Sertoli cells from prepubertal mice can be obtained by cell culture and germ cells can be isolated by unit gravity sedimentation as described above. In addition, both Sertoli cells and germ cells can be isolated by flow cytometry if they have been engineered to contain fluorescent or cell surface markers. Each of these techniques has limitations in that the testicular architecture is essentially destroyed and the cells are asked to survive in very artificial environments. Recently, a clever technique was developed that allows for transcription analysis in specific cell types without isolating the cells. The RiboTag mice utilize an in vivo ribosome tagging approach that allows for the isolation of polysomes from a single cell type within the testis [90]. A mouse with a cell-specific Cre-expressing transgene is crossed with the RiboTag mouse and this results in the placement of an immunoprecipitable antigen on the ribosomes of the target cell. The polysome-associated mRNA of the target cell can then be isolated from homogenized whole testis by immunoprecipitation and analyzed by arrays or RNAseq. This approach has been used to characterize the “translatome” individually in Sertoli cells, germ cells, and Leydig cells in vivo [90–92]. To identify AR-regulated genes, this technique was used to generate triple-mutant mice in which the Sertoli cells expressed the RiboTag but lacked androgen receptors. When the mRNA from the isolated polysomes was sequenced, hundreds of androgen regulated genes expressed solely in Sertoli cells were detected including many genes that had previously gone unreported [91]. The RiboTag approach is limited by the specificity and quality of the Cre-expressing strain of mice used in the genetic crosses but there appears to be many advantages in studies on the testis.
Another approach to examination of gene expression in Sertoli cells and germ cells has been to utilize either global or conditional gene inactivation or gene knockout (KO) approaches. In a recent review listing of Sertoli cell-specific gene KO mouse lines, it was surprising that only about 40 lines of mice had been generated [70]. These null mutations included enzymes involved in RA synthesis, hormone response, small RNA biogenesis, components of membrane junctions, and transcription factors. In that review of the KO studies done so far, it was concluded that the results strongly supported the notion that Sertoli cells participated in virtually every aspect of spermatogenesis including structural support, signals to germ cells for progression through mitotic, meiotic and haploid phases, and “waste management and recycling” of apoptotic cells and residual bodies [70]. Clearly, the advantage of the gene KO approach is that there is a potential to determine the function of genes if a phenotype develops as a result of the gene deletion.
Protein coding genes account for a small percentage of the genome, yet nearly all of the genome appears to be expressed as RNA [93,94]. This noncoding RNA (ncRNA) expression includes ribosomal RNA, transfer RNA, small nuclear and small nucleolar RNA essential for the ribosome and the spliceosome complex and potential “regulatory” ncRNAs including long ncRNAs, microRNAs (miRNA), small-interfering RNAs, and Piwi-interacting RNA (piRNA). In the testis, limited studies on the ncRNA have been done but it has been shown that the piRNA protects the germline from transposons [95]. In piRNA-deficient mice, retrotransposons are overexpressed and male germ cells are damaged from DNA damage and meiotic defects [96]. The presence of miRNAs in the testis and specifically in Sertoli cells and germ cells has recently been reviewed [97,98]. The biogenesis of miRNAs has been disrupted specifically in germ cells and in Sertoli cells, and several steps in spermatogenesis are disrupted. The targets of some germ cell miRNAs such as the let-7 family and the RA pathway have been proposed [97,99,100]. In one review, it is pointed out that while spermatogenesis is disrupted when biogenesis is knocked out in Sertoli cells it is not known which miRNAs function in which steps nor can a definitive function be assigned to a miRNA in Sertoli cells [97]. The presence of and the function of ncRNAs in the testis are topics that require more attention in future studies.
Sertoli cells, germ cells, and the cycle of the seminiferous epithelium
The recurring cellular associations in the testis were first termed the “spermatogenic cycle” in the late 1890s, and the current term “cycle of the seminiferous epithelium” was first used more than 50 years ago by LeBlond and Clermont in 1952 [101]. However, it was in 1972 that Clermont wrote an important review that defined the dynamics of the cycle and stated that for a given species, each step of spermatogenesis “has a constant duration; thus, germ cell differentiation unfolds as if regulated by a rigid time-scaled program” [102]. The cycle is similar in most mammals and while the timing may be species specific, the function is similar for all species to assure nonsynchronous and continuous spermatogenesis as opposed to synchronous and pulsatile release of sperm [24]. The constant recurring association of germ cell types in stages suggested that the sequence of developmental events occurring in spermatogonia, spermatocytes, and spermatids is coordinated with one another during spermatogenesis. There is also a spatial arrangement of the stages along the length of the tubule and this was described by Clermont as the “wave of the seminiferous epithelium.” Clermont defined the wave of the seminiferous epithelium as the distance between two successive, identical stages and stated that “the sequence of pictures along a wave is similar to the sequence of events taking place in one given area during a cycle of the seminiferous epithelium.” [101,102]. Both the “cycle” and the “wave” define the timing of the cycle, and understanding them is the key to understanding asynchronous spermatogenesis. The cycles from many different mammalian species have a number of commonalities. For example, the transition of the A undifferentiated spermatogonia to A1 differentiated spermatogonia, the onset of elongated spermatid development, and spermiation are linked to similar stages and four rounds of the cycle are required for the development of sperm from the onset of spermatogonial differentiation [24]. Clermont, in 1972, speculated that “some other factor(s) must be involved to account for the simultaneous entrance in spermatogenesis of spermatogonial stem cells”[102].
The morphology of the germ cells, especially the spermatids, is used to define the stages of the cycle. However, the morphology of the Sertoli cells also varies across the cycle. Virtually every component of the Sertoli cells including organelles, cytoskeletal components, lipid droplets, and endocytic vesicles changes dramatically across the stages of the cycle [103,104]. The first capability to examine molecular changes across the cycle came as a result of the use of antibodies and eventually in situ hybridization. The ability to experimentally examine biochemical and transcriptional changes in the stages of the cycle in the rat came about as a result of the pioneering work of M. Parvinen of the University of Turku. He utilized the variable light transmission through seminiferous tubules (termed transillumination) to manually dissect the stages of the cycle [105,106]. This technique stimulated the interest in the cycle of the seminiferous epithelium and was used to explore the cyclic functions in both Sertoli cells and germ cells in a large number of laboratories (for a review, see [106]). A salient example of the use of transillumination was the analysis by arrays of steady-state transcription in carefully dissected stages [107]. The results from the stage-dissected tubules were compared to data from isolated Sertoli cells, spermatogonia, early spermatocytes, pachytene spermatocytes, and round spermatids. In this manner, the 1286 array probe sets that varied more than fourfold across the cycle could be assigned to individual cell types. The same data were also used to compile a list of 58 genes that had 1000-fold higher expression in the testis when compared to 20 nonreproductive tissues [108].
An alternative to examining stage-specific transcription by transillumination and dissection was stage synchronization of the testis achieved by manipulating vitamin A levels. It has been known since 1925 that vitamin A is required for normal spermatogenesis (for recent reviews of this subject, see [109,110]). When retinol was added to cultured Sertoli cells, the steady-state level of a number of mRNAs was altered showing that the Sertoli cells can respond to retinoids. When retinol, the precursor to the biologically active RA, was deleted from the diet of rodents until spermatogenesis ceased and subsequently restored by an injection of retinol, it was found that the cycle of the seminiferous epithelium was synchronized throughout the testis [111]. Rather than the consecutive stages of the cycle along the seminiferous tubule resulting in asynchronous spermatogenesis, the tubules of the entire testis contained only one or two closely related stages at any point in time. The timing of the stages of the cycle in this synchronized spermatogenesis was similar to the timing in the asynchronous testis but there was no spermatogenic wave [112]. This was the first report that linked the cycle of the seminiferous epithelium with vitamin A and it was followed by additional studies on gene expression in rats with testes synchronized to various stages of the cycle [113–115] and to synchronization of mouse testes using vitamin A depletion and repletion [116].
In one report, it was suggested that most of the Sertoli cell products which change in relative concentrations during the cycle of the seminiferous epithelium appear to have a maximum in either stages VII or IX or in stages XIII to III. As a result, the investigators proposed that the Sertoli cells in the cycle could be described as having two different functional modes. In one mode (A) maximal levels of mRNA for a specific Sertoli cell product were found in stages VII-IX, and in the second mode (B) the maximal levels for differentially expressed genes were found in stages XIII to III. They showed that the distribution of the receptor mRNA and ABP mRNA could also be described in terms of these two modes of Sertoli cell function. Transferrin receptors, RA receptors, and ABP appear to fall into mode A, while FSH and androgen receptors were expressed in mode B. They proposed that most of the differential expression of genes by the Sertoli cells during the cycle fit into one of the dual modes than into an infinite number of operational modes [117]. This concept fit the known functional modes of the cycle with spermiation, onset of spermatogonial differentiation and meiosis, and tight junction dynamics in mode A and spermatogonial expansion in mode B.
Despite the usefulness of the original stage synchronization technique, it had a number of limitations. It required the maintenance of rats or mice on vitamin A-deficient diets for many weeks and at the end of this time the general health of the animals was poor and the extent of synchrony was often variable [118].
Recent progress in understanding the control of the cycle of the seminiferous epithelium has dramatically changed the landscape with regard to synchronous spermatogenesis. When gene expression from embryonic gonads and during spermatogenesis was examined, the expression of the Stra8 gene stood out. It was expressed only in females in the embryo and only in the males in the postnatal mice and expression correlated with the onset of meiosis [87,119]. Stra8 was shown to be inducible by RA, expressed in germ cells and required for the entrance of male and female germ cells into meiosis [120–124]. While the function of Stra8 is unknown, the presence of detectable Stra8 mRNA or protein was a specific and sensitive marker for the action of RA and the transition of A spermatogonia to A1 spermatogonia [24]. The findings that opened this field up dramatically was that a drug, WIN 18,446, previously shown to inhibit spermatogenesis in men and rabbits acted by inhibiting the aldehyde dehydrogenases that catalyzed the synthesis of retinal to RA [125,126] and it was directly shown that RA induced the transition of undifferentiated A spermatogonia to differentiating A1 spermatogonia by stimulating Stra8 and Kit gene expression [123,124]. Both a dietary vitamin A deficiency and a drug-induced deficiency resulted in a block of spermatogenesis at the transition of A spermatogonia to A1 spermatogonia.
When mice were fed WIN 18,446 from postnatal day 1 to 9, the result was an accumulation of A undifferentiated spermatogonia. At day 9, the mice were injected with RA and the testes were analyzed at intervals. Within 12 hours after the RA injection, there was a synchronous differentiation of A spermatogonia into A1 spermatogonia as determined by the expression of Stra8 mRNA and protein. The synchronous development of germ cells followed known developmental timing and was maintained into the adult testis where only a few closely related stages were present at any given time. This approach utilizing WIN 18,446 administration every 24 h resulted in the immediate inhibition of Aldh1a enzymes in the testis and since the half-life of RA in the testis was only 1.3 h there was a rapid testicular vitamin A deficiency [127]. Mice could be maintained on WIN 18,446 for days and the only apparent effect was the inhibition of the transition of A spermatogonia to A1 spermatogonia as measured by the lack of Stra8 induction [128]. Using WIN 18,446 to synchronize prepubertal mouse testes resulted in the capability to quickly generate adult mice with testes synchronized to a few stages of the cycle with relative ease. This approach allowed for the collection of sufficient amounts of testicular tissue to measure the RA levels across the cycle of the seminiferous epithelium for the first time. It was shown that there was a dramatic peak of RA levels associated with stages VIII-IX of the cycle coordinating exactly with the onset of spermatogonial differentiation [129]. Thus, the “some other factor” proposed by Clermont in 1972 that coordinates and drives spermatogenesis was shown to be pulses or gradients of RA that normally move along the tubule simultaneously pushing spermatogonia into differentiation, transitioning preleptotene spermatocytes across the BTB, and stimulating spermiation [24,129].
When the Win 18,446 protocol described above was used, the first wave of spermatogenesis was also synchronized so that at the end of the treatment there was an accumulation of A, Ap, and A aligned undifferentiated spermatogonia in the testis. When the block was released, normal spermatogonial differentiation could proceed in a synchronized fashion [130]. RiboTag mice were used with WIN 18,446/RA treatment were used to allow for the germ cell specific or Sertoli cell-specific isolation of polysomes from a total testis. The transcriptome of the Sertoli cells and of the A spermatogonia (from WIN 18,446 only treated animals) and of the various differentiating spermatogonial populations (from WIN 18,446 plus RA treated animals following different intervals of recovery) were then examined after collection of the germ cell-specific polysomes [92]. The combination of WIN 18,446 synchronization and the RiboTag mice provides a powerful approach to gene expression in the testis.
The role of RA in initiating the cycle of the seminiferous epithelium was explored using a transgenic mouse model that expressed beta-galactosidase under the control of a RA response element. Cells in 2- to 3-day old neonatal testes that had active RA signaling were visualized by beta-galactosidase activity [131]. The beta-galactosidase activity was found to be present in differentiating A1 spermatogonia, but the patches of activety were distributed nonuniformly along the tubule. This was interpreted to be representative of the initiation of the spermatogenic wave. When the neonatal testes were injected with exogenous RA, nearly all of the germ cells became positive for beta-galactosidase and when these mice became adults, spermatogenesis in the testis was synchronized [132,133]. So, the spermatogenic wave appeared to arise from patches or periodic spermatogonial differentiation along the tubule. Therefore, in neonatal mice or in mice made deficient in RA by diet or by WIN 18,446 where the most advanced cells are A undifferentiated spermatogonia, the spermatogenic wave can be eliminated by exogenous RA and the treated mice become adults with synchronous spermatogenesis.
Summary and remaining problems
We know much more about the Sertoli cells and their interactions with germ cells than we did 50 years ago or even 25 years ago. In the preface toThe Sertoli Cell in 1993, Lonnie Russell and I whimsically suggested that there were two categories of investigators working on spermatogenesis [1]. We categorized them as the “dogmatic religious Sertologists” who felt that Sertoli cells were required for everything and the “germ cell worshiping cult” who felt that germ cell development into functional gametes was completely autonomous. These two groupings were an attempt to underscore the lack of information about how the Sertoli cells and the germ cells interacted and which one, if either, was most influential in directing the process. After 1993, the ability to transplant spermatogonia in murine testes and establish long-term cultures of spermatogonia suggested that much of germ cell development was cell autonomous and offered encouragement to the “germ cell worshipping cult.” In addition, contributing to the disappointment of the “dogmatic religious Sertologists” a number of important genes had been genetically knocked out in Sertoli cells but still allowed germ cells to develop. Richard Sharpe in a review in 1994 suggested that the function of Sertoli cells was to allow the efficient but autonomous development of germ cells [134]. This idea was termed the “modified permissive view.” Consistent with this view is the in vitro culture of stem cells and spermatogonia which is well documented but the in vitro progression of germ cells through meiosis and the formation of spermatids has proven to be difficult to reproduce and inefficient, at best [135]. It appears that one of the barriers to spermatogenesis in culture is the essential requirement for Sertoli cells to provide both biochemical and structural elements. The “dogmatic religious Sertologists” would concede that much of germ cell development and the formation of functional gametes appears to be cell autonomous but Sertoli cells compartmentalize and provide regulatory discipline through paracrine signals. While we know how the Sertoli cell structure can compartmentalize germ cells, we know about only a few paracrine signals. GDNF is an example of a Sertoli cell signal that is required for germ cell replication and differentiation [64]. In addition, testosterone acting through Sertoli cells in ways that are not totally understood is essential for completion of meiosis and in vivo spermiogenesis [136]. Sertoli cells provide the RA that initiates the cycle of the seminiferous epithelium [137]. It is likely that these signaling events from Sertoli cell to germ cell and likely some that signal from germ cells to Sertoli cells are keys to understanding problems of human idiopathic infertility. They almost certainly contribute to the difficulty of generating gametes in vitro.
In reflecting on the progress, it is a reminder that technology drives the research approaches. Since 1967 basic research on spermatogenesis has been fueled by better microscopes, the development of primary cell culture, transgenic expertise, germ cell transplantation, flow cytometry, DNA arrays and RNAseq, and mass spectrometry. It is pretty safe to predict that the approaches will continue to rely on new technological innovations. One developing technology that will impact research on spermatogenesis will be the use of Crispr/Cas9 for genome editing. For example, genome editing has been used to globally inactivate the Spata2 gene that is normally highly expressed in Sertoli cells. The resulting phenotype showed a 40% decrease in testis size and sperm count with a 70% increase in inhibin alpha subunit mRNA [138]. A major advantage of this approach is that the use is not limited to rodents. Single cell gene expression analysis is also a developing technique that will have major impacts on understanding Sertoli and germ cell gene expression in cell-specific detail [139,140].
While technology drives the research approaches, it is often societal needs that drive research funding and as a result research topics. For example, the recent meta-analysis on the decline in sperm counts has generated much public interest [141]. While there have been many previous reports on declining sperm counts, this meta-regression analysis using a comprehensive approach reported a 50–60% decline in sperm counts between 1973 and 2011 among men in the developed world. The authors stated that, “Because of the significant public health implications of these results, research on the causes of this continuing decline is urgently needed.” Others have reported increased cases of testicular cancer and disorders of the male reproductive system and have associated these changes with environmental changes and the modern lifestyle. Endocrine disruptors released into the environment could be a cause [142]. A greater comprehension of the Sertoli cells and their interaction with germ cells will be necessary in order to understand the underlying mechanisms.
References
Articles from Biology of Reproduction are provided here courtesy of Oxford University Press
Full text links
Read article at publisher's site: https://doi.org/10.1093/biolre/ioy027
Read article for free, from open access legal sources, via Unpaywall:
https://academic.oup.com/biolreprod/article-pdf/99/1/87/25155051/ioy027.pdf
Citations & impact
Impact metrics
Citations of article over time
Alternative metrics
Smart citations by scite.ai
Explore citation contexts and check if this article has been
supported or disputed.
https://scite.ai/reports/10.1093/biolre/ioy027
Article citations
Short-Term Treatment of Melatonin Improves the Expression of Cell Adhesion Molecules in the Testis of the Mouse Cryptorchidism Model.
J Histochem Cytochem, 72(10):623-640, 20 Sep 2024
Cited by: 0 articles | PMID: 39301779
Temporal maturation of Sertoli cells during the establishment of the cycle of the seminiferous epithelium†.
Biol Reprod, 111(4):959-974, 01 Oct 2024
Cited by: 0 articles | PMID: 39077996
Preliminary Evidence of the Possible Roles of the Ferritinophagy-Iron Uptake Axis in Canine Testicular Cancer.
Animals (Basel), 14(17):2619, 09 Sep 2024
Cited by: 0 articles | PMID: 39272404 | PMCID: PMC11394645
Energy metabolism and spermatogenesis.
Heliyon, 10(19):e38591, 27 Sep 2024
Cited by: 0 articles | PMID: 39397940 | PMCID: PMC11470522
Review Free full text in Europe PMC
Therapeutic potential of Sertoli cells in vivo: alleviation of acute inflammation and improvement of sperm quality.
Stem Cell Res Ther, 15(1):282, 04 Sep 2024
Cited by: 0 articles | PMID: 39227878 | PMCID: PMC11373210
Go to all (87) article citations
Similar Articles
To arrive at the top five similar articles we use a word-weighted algorithm to compare words from the Title and Abstract of each citation.
The establishment of spermatogenesis in the seminiferous epithelium of the pubertal golden hamster (Mesocricetus auratus).
Adv Anat Embryol Cell Biol, 140:1-92, 01 Jan 1998
Cited by: 11 articles | PMID: 9586281
Riding the spermatogenic wave: profiling gene expression within neonatal germ and sertoli cells during a synchronized initial wave of spermatogenesis in mice.
Biol Reprod, 90(5):108, 09 Apr 2014
Cited by: 44 articles | PMID: 24719255 | PMCID: PMC4076369
The murine seminiferous epithelial cycle is pre-figured in the Sertoli cells of the embryonic testis.
Development, 129(3):635-647, 01 Feb 2002
Cited by: 31 articles | PMID: 11830565
Regulation of spermatogenesis in the microenvironment of the seminiferous epithelium: new insights and advances.
Mol Cell Endocrinol, 315(1-2):49-56, 12 Aug 2009
Cited by: 89 articles | PMID: 19682538 | PMCID: PMC3516447
Review Free full text in Europe PMC