Abstract
Background
Intestinal microbiota and their metabolites (e.g. short-chain fatty acids (SCFAs)) may influence nonalcoholic fatty liver disease (NAFLD).Objective
The objective of this article is to analyze gut bacterial diversity together with fecal SCFA concentrations and immunophenotyping of peripheral blood in histology-proven NAFLD patients.Methods
Thirty-two NAFLD patients (14 nonalcoholic fatty liver (NAFL), 18 nonalcoholic steatohepatitis (NASH)) and 27 healthy controls (HCs)) were included in this study. Bacterial communities in feces were profiled by 16S ribosomal RNA gene sequencing of the V3-V4 region. Fecal SCFA levels were analyzed by high-performance liquid chromatography. Fluorescence-activated cell sorting analysis was performed of peripheral blood mononuclear cells.Results
NASH patients were characterized by higher abundance of Fusobacteria and Fusobacteriaceae compared to NAFL and HCs. Conforming to our finding that NAFLD patients had higher fecal acetate and propionate levels, taxonomical differences of fecal bacteria were dominated by SCFA-producing bacteria. Higher fecal propionate and acetate levels were associated with lower resting regulatory T-cells (rTregs) (CD4+CD45RA+CD25++) as well as higher Th17/rTreg ratio in peripheral blood as immunological characteristics of NASH patients.Conclusions
NASH patients are characterized by a different gut microbiome composition with higher fecal SCFA levels and higher abundance of SCFA-producing bacteria in NAFLD. These changes are associated with immunological features of disease progression. Our data suggest an important role of the intestinal microbiome and immunomodulatory bacterial metabolites in human NAFLD.Free full text

Fecal SCFAs and SCFA-producing bacteria in gut microbiome of human NAFLD as a putative link to systemic T-cell activation and advanced disease
Associated Data
Abstract
Background
Intestinal microbiota and their metabolites (e.g. short-chain fatty acids (SCFAs)) may influence nonalcoholic fatty liver disease (NAFLD).
Objective
The objective of this article is to analyze gut bacterial diversity together with fecal SCFA concentrations and immunophenotyping of peripheral blood in histology-proven NAFLD patients.
Methods
Thirty-two NAFLD patients (14 nonalcoholic fatty liver (NAFL), 18 nonalcoholic steatohepatitis (NASH)) and 27 healthy controls (HCs)) were included in this study. Bacterial communities in feces were profiled by 16S ribosomal RNA gene sequencing of the V3–V4 region. Fecal SCFA levels were analyzed by high-performance liquid chromatography. Fluorescence-activated cell sorting analysis was performed of peripheral blood mononuclear cells.
Results
NASH patients were characterized by higher abundance of Fusobacteria and Fusobacteriaceae compared to NAFL and HCs. Conforming to our finding that NAFLD patients had higher fecal acetate and propionate levels, taxonomical differences of fecal bacteria were dominated by SCFA-producing bacteria. Higher fecal propionate and acetate levels were associated with lower resting regulatory T-cells (rTregs) (CD4+CD45RA+CD25++) as well as higher Th17/rTreg ratio in peripheral blood as immunological characteristics of NASH patients.
Conclusions
NASH patients are characterized by a different gut microbiome composition with higher fecal SCFA levels and higher abundance of SCFA-producing bacteria in NAFLD. These changes are associated with immunological features of disease progression. Our data suggest an important role of the intestinal microbiome and immunomodulatory bacterial metabolites in human NAFLD.
Key summary
Summarize the established knowledge of the subject:
Intestinal microbiota is one component of the “multiple hits” in the pathogenesis of nonalcoholic fatty liver disease (NAFLD).
Short-chain fatty acids (SCFAs) are the most abundant bacterial products derived from commensal bacterial fermentation of dietary fibers in intestines.
Fecal SCFAs have been proposed to influence the development of NAFLD, but their exact role in NAFLD pathogenesis remains unclear.
What are the significant and/or new findings of the study?
Intestinal microbiota was characterized by higher abundance of Fusobacteria and Fusobacteriaceae in nonalcoholic steatohepatitis (NASH) patients compared to nonalcoholic fatty liver (NAFL) and healthy controls.
Higher fecal SCFA concentrations and domination of SCFA-producing bacteria were observed in NAFLD patients.
SCFA concentrations were associated with immunological features of NAFLD disease progression.
Intestinal microbiota and fecal SCFA concentrations may be important targets for future personalized medicine in NAFLD patients.
Introduction
Nonalcoholic fatty liver disease (NAFLD) has growing clinical and socioeconomic impact. The pathogenesis of NAFLD is described by “multiple hits” on a nonalcoholic fatty liver (NAFL) that progress to nonalcoholic steatohepatitis (NASH). The intestinal microbiota is one of the “multiple hits” and is the focus of recent studies in NASH patients with different technical and methodical approaches leading to some inconsistent findings. In one study NASH patients showed a lower percentage of Bacteroidetes (Bacteroides/Prevotella) compared to NAFL patients and healthy controls (HCs),1 whereas in another study higher abundance of Bacteroides and lower abundance of Prevotella were observed in NASH patients.2 Loomba et al. detected higher abundance of Firmicutes in mild/moderate NAFLD and higher abundance of Proteobacteria in NAFLD patients with advanced fibrosis (no change for Bacteroidetes).3 Different mechanisms are proposed for the microbial contribution in NAFLD including regulation of gut barrier and inflammatory responses as well as bacterial metabolites, e.g. short-chain fatty acids (SCFAs).4
SCFAs such as acetate, propionate and butyrate are the most abundant bacterial products derived from commensal bacterial fermentation of dietary fibers in intestines; their role in obesity development is still controversial. On the one hand, SCFAs provide additional energy to the host and play a pivotal role in lipogenesis and gluconeogenesis, but on the other hand SCFAs increase satiety by activation of free fatty acid receptors and weight loss can thereby be sustained.5 In human studies fecal SCFA concentrations were higher in obese volunteers compared to lean individuals.6,7 In animal and in vitro studies, SCFAs affect proinflammatory cytokine production,8 promote conversion toward regulatory T-cells (Tregs) under specific conditions9 and enhance Foxp3 expression of colonic T-cells via G protein coupled receptor (GPCR) 43 activation,10 but also facilitate conversion of naïve T-cells into Th1 or Th17 cells depending on cytokine milieu and histone deacetylase inhibitor activity.11
In this study, we analyzed for the first time fecal SCFA concentrations together with gut microbiota composition in obese patients with histology-proven NASH and (NAFL) for a better understanding of functional changes of the fecal microbiota. Further integrative analysis of peripheral immune cell changes as characteristics of disease progression (NASH)12 were performed and interpreted together to gain insight into a potential interplay between intestinal bacteria composition, their metabolites and peripheral immune cells.
Methods
Patient characteristics
The study was approved by the local ethics committee (University of Würzburg: EK 96/12, 5 September 2012) and conforms to the ethical guidelines of the 1975 Declaration of Helsinki. After written informed consent was obtained, 32 NAFLD patients (14 NAFL, 18 NASH) and 27 HCs were enrolled in this prospective study. An overview of study participants is depicted in Figure 1. Study participants were all >18 years old. Twenty-four patients were morbidly obese and underwent bariatric surgery, and eight obese NAFLD patients were included without bariatric surgery, but with histologically diagnosed NAFLD. Significant alcohol consumption (women >20g/d and men >30
g/d) and other liver diseases such as chronic hepatitis B virus, hepatitis C virus infections as well as autoimmune liver diseases (hepatitis B surface antigen, anti-HCV, antinuclear antibodies) were excluded. Patients had no standardized diet before surgery. In general, a low-carbohydrate diet is recommended two weeks prior to surgery. Patients without bariatric surgery had no specific diet. Blood samples were taken at the day of inclusion while fecal samples were harvested immediately before bariatric surgery. Bariatric surgery followed up to 14 days after study inclusion. Patients had not received any antibiotic treatment the last six months prior to study inclusion. Biopsies were taken from subcapsular liver tissue during bariatric surgery or percutaneously from the right liver lobe in participants without bariatric surgery. All liver biopsies were analyzed by an experienced pathologist and classified using the Steatosis, Activity and Fibrosis (SAF) score.13 HCs were all individuals without evidence or history of liver or metabolic diseases. In HCs liver ultrasound and liver stiffness as determined by transient elastography measurement (FibroScan®) were normal. In HCs liver enzyme levels were measured and laboratory analysis showed no elevation for Glutamate oxalacetate tranasminase (GOT) and Glutamate pyruvate transaminase (GPT).
Fecal collection and analyses
Fecal samples were collected from study participants. The fecal samples were continuously cooled to a maximum of +4, subsequently snap frozen within 24 hours and finally stored at –80
° until further analysis.
Bacterial DNA extraction and 16S ribosomal RNA (rRNA) gene sequencing
Total genomic DNA from fecal pellets of study participants was extracted using the MoBio PowerSoil DNA Isolation kit (Dianova GmbH, Germany) with slight changes: Solution C1 and 20µl of proteinase K were added to fecal material and incubated for two hours at 50
to enhance the bacterial cell lysis. Bacterial communities were profiled by 16S rRNA gene amplicon sequencing. 16S rRNA gene variable region V4 was amplified using specific primers.14 Amplification was performed by Phusion® Hot Start Flex 2X Master Mix (New England BioLabs, Germany) in GeneAmp polymerase chain reaction (PCR) system 9700 (Applied Biosystems, USA). Quantitative normalization of PCR products was performed using the SequalPrep kit (Invitrogen GmbH, Germany) to pool equal amounts of amplicons per sample. Sequencing was performed by the Illumina MiSeq (2
×
300 base) sequencing kit (Illumina, USA) using Illumina-specific sequencing primers.
Analysis of sequences
Quality control of sequence reads was performed using the software mothur package.15 Forward and reverse reads (fastq) were assembled to contigs sequences and discarded if having any ambiguous base or more than eight homopolymers. Sequences were aligned against a mother-curated silva alignment database and screened to have alignment in the amplified specified V3–V4 region only. Chimeric sequences were detected with the Uchime algorithm and removed from subsequent analysis. Sequences were assigned taxonomically using mothur-formatted greengenes training sets and eliminated if classified as unknown, archaea, eukaryotes, chloroplast and mitochondria. Sequences were binned in to phylotypes using mothur.
SCFA analysis in fecal samples—high-performance liquid chromatography (HPLC)
Sample preparation and HPLC analysis of the fecal SCFA samples were carried out according to the method from De Baere et al.16 with minor modifications.
An SCFA extraction procedure was performed as previously described.17 Batches of 20 patient samples and eight external calibration points were measured per HPLC run. For data interpretation Chrom-Nav chromatography software was used (version 2.0, Jasco, de Meern, The Netherlands).
Immune cell analysis in peripheral blood
Lymphocyte separation medium (PAA) was used for the isolation of lymphocytes and peripheral blood mononuclear cells (PBMCs) ex vivo. Restimulation for cytokine detection as well as flow cytometry analysis were performed as previously described.12
Analysis of microbiome data and statistical analysis
Analyses of microbiome data were performed using the statistics software R (version 3.2.2) and the packages vegan_2.4-3 and metagenomeSeq (version 1.2.21).18 Samples with fewer than 500 sequences in total were discarded and only taxa that were detected in ≥10 samples have been included in subsequent analyses. Abundance counts have been normalized with the cumulative sum scaling approach as implemented in the metagenomeSeq package18 using default parameters. Principal component (PCA) and redundancy analysis (RDA) have been performed on the normalized log-transformed data as implemented in the vegan package. For the RDA study groups have been included as factorial covariates. Significance of covariates has been assessed based on 10,000 random permutations of the data. The correlation of the Th17/Treg ratio with the ordination configuration of the PCA has been calculated with the function “vectorfit” in the vegan package. Differential of taxon abundances were estimated using a zero-inflated log-normal regression model as implemented in the metagenomeSeq package. Normalized count mean, standard error (SE) and fold changes (logFC) are given on the log2 scale. Mean and median abundancy data are provided as supplemental material (Supplement Table 1(a) and (b)). In this study all p values of microbiome analysis have been corrected for multiple testing using the Benjamini-Hochberg method.
SPSS (19.0, SPSS Inc, Chicago, IL, USA) and Prism (GraphPad Software, La Jolla, CA, USA) were used for further analyses. Quantitative variables were expressed as median with first quartiles and were compared using the Mann-Whitney test. Spearman rho correlation coefficient was calculated. A p value <0.05 was considered statistically significant (*), p<
0.01
=
(**), p
<
0.001
=
(***). P values were adjusted for multiple testing unless expressively given as uncorrected p value (puncorr) to indicate significant trends.
Results
Baseline characteristics of the study cohort
In this study cohort 32 histology-proven NAFLD patients (14 NAFL and 18 NASH) and 27 HCs were included. Figure 1 depicts a flow diagram with the overview of study participants. NASH patients more often had metabolic syndrome including arterial hypertension, type II diabetes mellitus and dyslipidemia as compared to NAFL (Supplement Table 2). GPT and GOT were significantly elevated in NAFLD patients compared to HCs (GPT mean±
SD: 46.5
±
40.8
U/l (NAFL), 54.2
±
27.5
U/l (NASH), 18.2
±
8.5
U/l (HCs); GOT mean
±
SD: 33.9
±
19.4
U/l (NAFL), 50.0
±
28.4
U/l (NASH), 19.7
±
7.4
U/l (HCs)). Histological diagnosis for NASH was performed by an experienced pathologist according to SAF score.13 Twenty-two patients with NAFLD had F0/F1 fibrosis on liver biopsy and 10 of 18 NASH patients (55.6%) had significant fibrosis (F≥2). Twenty-seven HCs had no evidence of liver disease. These participants had a significantly lower body mass index and were younger compared to NAFLD patients as observed in previous human NAFLD studies.1
Changes in fecal microbiota in NAFLD patients and HCs
First, we analyzed α-diversity of the respective study groups. The Shannon diversity index showed a significantly reduced diversity in NAFL (p<
0.01) and NASH (p
<
0.01) patients compared to HCs (Figure 2(a)). At the phylum level, NASH patients had a significantly higher abundance of Fusobacteria compared to NAFL patients (p
=
0.022) and to HCs (puncorr
=
0.027) (Table 1). On the family level substantial and significant differences between the study groups were observed (Table 1, Figure 2(b)). NASH patients were characterized by a higher frequency of Fusobacteriaceae (puncorr
=
0.002) in comparison to NAFL patients and higher abundance of Fusobacteriaceae (p
=
0.009) and Prevotellaceae (puncorr
=
0.009) compared to HCs. NAFL patients had a higher frequency of Prevotellaceae (puncorr
=
0.034) in comparison to HCs. Further detailed results on phylum and family level are listed in Table 1.

(a) Reduced microbial diversity in NAFLD patients depicted by Shannon index. *p<
0.05. (b) Overview of microbial composition on family level in NAFL, NASH and HCs. HCs: healthy controls; NAFL: nonalcoholic fatty liver; NAFLD: nonalcoholic fatty liver disease; NASH: nonalcoholic steatohepatitis.
Table 1.
Differences on phylum and family levels.
Normalized count mean | SE | logFC | p uncorr | p corr | |||
---|---|---|---|---|---|---|---|
Phylum | |||||||
![]() | HCs | NASH | |||||
k__Bacteria_unclassified | 953 | 67 | 0.564 | –2.320 | 0.000 | 0.000 | |
Fusobacteria | 19 | 203 | 1.109 | 2.461 | 0.027 | 0.087 | |
Cyanobacteria | 1176 | 126 | 0.991 | –2.139 | 0.031 | 0.087 | |
Tenericutes | 783 | 44 | 0.832 | –1.756 | 0.035 | 0.087 | |
![]() | HCs | NAFL | |||||
k__Bacteria_unclassified | 953 | 28 | 0.657 | –2.251 | 0.001 | 0.006 | |
Verrucomicrobia | 4194 | 213 | 0.782 | –1.833 | 0.019 | 0.096 | |
Tenericutes | 783 | 3 | 1.301 | –2.749 | 0.035 | 0.115 | |
![]() | NAFL | NASH | |||||
Fusobacteria | 2 | 203 | 1.249 | 3.823 | 0.002 | 0.022 | |
Family | |||||||
![]() | HCs | NASH | |||||
Odoribacteraceae | 1133 | 200 | 0.300 | –1.208 | 0.000 | 0.002 | |
k__Bacteria_unclassified | 953 | 67 | 0.476 | –1.862 | 0.000 | 0.002 | |
Fusobacteriaceae | 19 | 203 | 1.043 | 3.609 | 0.001 | 0.009 | |
o__RF32_unclassified | 971 | 25 | 0.863 | –2.715 | 0.002 | 0.020 | |
Prevotellaceae | 6904 | 9177 | 0.955 | 2.494 | 0.009 | 0.087 | |
Shewanellaceae | 18 | 36 | 0.643 | 1.471 | 0.022 | 0.177 | |
Halomonadaceae | 43 | 77 | 0.479 | 0.994 | 0.038 | 0.261 | |
![]() | HCs | NAFL | |||||
k__Bacteria_unclassified | 953 | 28 | 0.555 | –1.495 | 0.007 | 0.157 | |
p__Firmicutes_unclassified | 4510 | 27 | 0.947 | –2.541 | 0.007 | 0.157 | |
Prevotellaceae | 6904 | 7696 | 1.085 | 2.298 | 0.034 | 0.490 | |
Odoribacteraceae | 1133 | 135 | 0.350 | –0.687 | 0.049 | 0.530 | |
![]() | NAFL | NASH | |||||
Fusobacteriaceae | 2 | 203 | 1.253 | 3.949 | 0.002 | 0.070 | |
Barnesiellaceae | 589 | 403 | 0.639 | –1.309 | 0.040 | 0.869 |
HCs: healthy controls; NAFL: nonalcoholic fatty liver; NASH: nonalcoholic steatohepatitis.
Normalized count mean, standard error (SE) and fold changes (logFC) are given on the log2 scale of gut microbiome phyla and families in NAFL, NASH and HCs. pcorr=
p value adjusted for multiple testing and puncorr
=
p value not adjusted for multiple testing. Only phyla and families are listed if puncorr
≤
0.05.
For the analysis on species level, 126 species were applied. Table 2 depicts detailed results, including all species with puncorr≤
0.05. On the species level, NASH patients were characterized by a significantly higher abundance of Fusobacterium (p
=
0.033) as well as of Prevotella, Eubacterium biforme and Enterobacteriacae_unclassified (puncorr
=
0.003, puncorr
=
0.016, puncorr
=
0.016) compared to NAFL patients. Further differences on species levels between NASH/NAFL and HCs are listed in Table 2.
Table 2.
Differences on species levels.
Species | Normalized count mean | SE | logFC | p uncorr | p corr | ||
---|---|---|---|---|---|---|---|
NASH vs. HCs | HCs | NASH | |||||
Acidaminococcus | 4 | 1473 | 1.280 | 5.788 | 0.000 | 0.000 | |
Lachnospira | 3556 | 539 | 0.353 | −1.582 | 0.000 | 0.000 | |
Odoribacter | 942 | 139 | 0.365 | −1.492 | 0.000 | 0.002 | |
Bacteria_unclassified | 953 | 67 | 0.494 | −1.761 | 0.000 | 0.008 | |
Megasphaera | 255 | 2442 | 1.076 | 3.817 | 0.000 | 0.008 | |
Fusobacterium | 19 | 203 | 1.048 | 3.713 | 0.000 | 0.008 | |
Ruminococcus.torques | 212 | 879 | 0.491 | 1.600 | 0.001 | 0.019 | |
Parabacteroides | 2283 | 200 | 0.496 | −1.546 | 0.002 | 0.027 | |
Eubacterium.biforme | 117 | 559 | 1.010 | 2.922 | 0.004 | 0.050 | |
Prevotella.copri | 4945 | 8450 | 0.998 | 2.801 | 0.005 | 0.054 | |
RF32_unclassified | 971 | 25 | 0.922 | −2.584 | 0.005 | 0.054 | |
Alistipes.finegoldii | 542 | 19 | 0.707 | −1.829 | 0.010 | 0.094 | |
Escherichia.coli | 3988 | 6990 | 0.699 | 1.638 | 0.019 | 0.172 | |
Enterobacteriaceae_unclassified | 117 | 784 | 0.662 | 1.496 | 0.024 | 0.188 | |
Shewanella.algae | 18 | 36 | 0.602 | 1.358 | 0.024 | 0.188 | |
Halomonas | 42 | 76 | 0.470 | 0.945 | 0.045 | 0.326 | |
Bifidobacterium.longum | 1732 | 579 | 0.505 | −0.979 | 0.053 | 0.354 | |
Enterococcus | 9 | 107 | 0.996 | 1.917 | 0.054 | 0.354 | |
NAFL vs HCs | HCs | NAFL | |||||
Prevotella.copri | 4945 | 7455 | 1.066 | 3.433 | 0.001 | 0.050 | |
Acidaminococcus | 4 | 1750 | 1.592 | 5.080 | 0.001 | 0.050 | |
Bacteria_unclassified | 953 | 28 | 0.551 | −1.707 | 0.002 | 0.050 | |
Firmicutes_unclassified | 4510 | 27 | 0.908 | −2.813 | 0.002 | 0.050 | |
Odoribacter | 942 | 89 | 0.361 | −1.104 | 0.002 | 0.050 | |
Lachnospira | 3556 | 307 | 0.419 | −1.258 | 0.003 | 0.050 | |
Rikenellaceae_unclassified | 2816 | 420 | 0.432 | −1.110 | 0.010 | 0.165 | |
Ruminococcus.torques | 212 | 391 | 0.555 | 1.300 | 0.019 | 0.270 | |
Dialister | 3103 | 4181 | 0.757 | 1.715 | 0.024 | 0.296 | |
Enterobacteriaceae_unclassified | 117 | 5 | 0.887 | −1.792 | 0.043 | 0.491 | |
Anaerotruncus | 161 | 16 | 0.521 | −1.031 | 0.048 | 0.491 | |
NASH vs NAFL | NAFL | NASH | |||||
Fusobacterium | 2 | 203 | 1.241 | 4.494 | 0.000 | 0.033 | |
Prevotella | 20 | 466 | 0.842 | 2.465 | 0.003 | 0.192 | |
Roseburia | 328 | 273 | 0.455 | −1.127 | 0.013 | 0.333 | |
Eubacterium.biforme | 20 | 559 | 1.036 | 2.492 | 0.016 | 0.333 | |
Enterobacteriaceae_unclassified | 5 | 784 | 1.287 | 3.091 | 0.016 | 0.333 | |
Parabacteroides | 744 | 200 | 0.551 | −1.308 | 0.018 | 0.333 | |
Dialister | 4181 | 1707 | 0.650 | −1.332 | 0.040 | 0.653 | |
F0/F1 vs![]() ![]() | F0/F1 | ≥F2 | |||||
Alistipes.onderdonkii | 285 | 31 | 0.778 | −2.566 | 0.001 | 0.092 | |
Clostridium.citroniae | 87 | 12 | 0.851 | −2.378 | 0.005 | 0.246 | |
Acidaminococcus | 219 | 1254 | 1.492 | 3.331 | 0.026 | 0.665 | |
Prevotella | 71 | 395 | 1.212 | 2.662 | 0.028 | 0.665 |
HCs: healthy controls; NAFL: nonalcoholic fatty liver; NASH: nonalcoholic steatohepatitis.
Normalized count mean, standard error (SE) and fold changes (logFC) are given on the log2 scale of gut microbiome species in NAFL, NASH and HCs as well as in advanced diseases (F0/F1 and ≥F2 fibrosis). pcorr=
p value adjusted for multiple testing and puncorr
=
p value not adjusted for multiple testing. Only species are listed if puncorr
≤
0.05.
ß-diversity analysis showed different profiles of gut microbiome data
PCA on operational taxonomic unit (OTU) level separates NAFLD patients (including NAFL and NASH patients) from HCs on the first axis as depicted in Figure 3(a). No significant difference was observed in the PCA on the OTU level between bariatric patients and obese patients without bariatric surgery to exclude preoperative diet as possible bias (Supplemental Figure 1). In our previous work NASH patients were successfully differentiated and characterized by increased Th17 (CD4+IL17+)/resting Treg (rTreg/CD4+CD45RA+CD25++) ratio in peripheral blood compared to NAFL patients.12 Here we observed a significant correlation (R2=
0.171, p value
<
0.01) of Th17/rTreg ratio, with the ordination configuration of the first two axes (Figure 3(a)). In Figure 3(b) PCA of microbiome data separate clearly the study cohort into high- and low-Th17/rTreg ratio subgroups (Th17/rTreg low
<
0.38, Th17/rTreg high
≥
0.38). There was thus a good correlation between the observed changes in the gut microbiome and peripheral T-cells. In a supervised ordination approach (RDA), NAFL, NASH and HCs could be successfully differentiated as well (Figure 3(c)). A permutation test based on 10,000 random permutations yields a highly significant effect (p
<
0.001) of the study group factor.

(a) Principal component analysis of gut microbiome data in NAFL, NASH and HCs with positive correlation to peripheral immune cell ratio Th17/rTreg. (b) Principal component analysis of gut microbiome data in patients with high- and low-Th17/rTreg ratio. (c) Redundancy analysis of gut microbiome data based on the study group (NAFL, NASH and HCs). HCs: healthy controls; NAFL: nonalcoholic fatty liver; NAFLD: nonalcoholic fatty liver disease; NASH: nonalcoholic steatohepatitis; rTreg: resting regulatory T-cell; Treg: regulatory T-cell.
Higher fecal SCFA concentrations as well as SCFA-producing bacteria in NAFLD patients
Acetate, propionate as well as butyrate concentrations were increased in feces of NAFLD patients. NAFL as well as NASH patients had significantly higher fecal concentrations of acetate and propionate compared to HCs. A trend toward higher fecal butyrate levels in NASH patients compared to HCs was observed (Figure 4(a)).
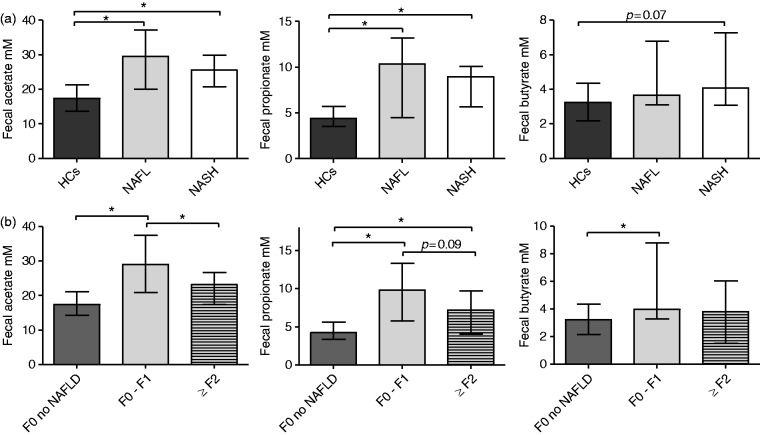
(a) Fecal acetate, propionate and butyrate concentrations in HCs, NAFL and NASH patients. *p<
0.05. Median
±
IQR; acetate: HCs 17.4
±
7.6
mM, NAFL 29.5
±
17.1
mM, NASH 25.6
±
9.2
mM; propionate: HCs 4.4
±
2.2
mM, NAFL 10.4
±
8.7
mM, NASH 9.0
±
4.5
mM; butyrate: HCs 3.2
±
2.2
mM, NAFL 3.6
±
3.7
mM, NASH 4.1
±
4.2
mM. (b) Fecal acetate, propionate and butyrate concentrations in F0 of NAFLD, NAFLD patients with no fibrosis (F0/F1) and moderate/advanced fibrosis (F≥2). *p
<
0.05. Median
±
IQR. HCs: healthy controls; IQR: interquartile range; NAFL: nonalcoholic fatty liver; NAFLD: nonalcoholic fatty liver disease; NASH: nonalcoholic steatohepatitis.
Bacterial communities were analyzed regarding their functional capacity of SCFA production based on previously published data.19–22 At the phylum and family levels, higher abundance of Fusobacteria and Fusobacteriaceae and higher frequency of Prevotellaceae were observed. Fusobacteriaceae as well as Prevotellaceae are bacterial families characterized by SCFA-producing capacity.19,20,22 Differences in species levels between NAFLD (NAFL/NASH) patients and HCs showed a significantly higher abundance of species such as Prevotella copri, Megashpaera, Fusobacterium, Ruminococcus torques and Eubacterium biforme (Table 2). Interestingly, these species are well described as SCFA-producing bacteria or belong to families with high capacity of SCFA production.19,20,22 Thus the observed differences in family and species level in gut microbiome analysis of NAFLD patients are functionally relevant and correlate well with higher fecal concentrations of acetate and propionate in these patients (Figure 4(a)).
Different microbial composition and SCFA production in patients with moderate/advanced fibrosis
Microbial composition and fecal SCFA concentrations were different in NAFLD patients with advanced disease. Patients with significant fibrosis had higher frequency of Acadaminococcus and Prevotella (puncorr=0.026, puncorr
=
0.028) (Table 2). No significant differences were observed in family level between F0/F1 patients and F≥2 patients (Supplemental Table 3).
NAFLD patients with significant fibrosis (F≥2 fibrosis) had reduced fecal acetate as well as a trend toward reduced propionate concentrations (Figure 4(b)). No significant differences were observed for butyrate between F0/F1 and F≥2 patients (Figure 4(b)). In a supervised PCA, F0/F1 patients were successfully separated from F≥2 patients (Figure 5).
Peripheral immune cell markers in NASH patients are associated with fecal SCFA levels
As a unique feature of this study, data of fecal SCFA concentrations were analyzed together with peripheral immune cell changes as depicted in Figure 6. A significant negative correlation between rTregs in peripheral blood and fecal propionate as well as acetate levels was observed. Peripheral Th17/rTreg ratio showed a positive correlation for fecal acetate and propionate concentrations (Figure 6).

As previously described, NASH patients were characterized by an increased Th17/rTreg ratio in peripheral blood as well as in hepatic tissue compared to NAFL patients.12 These immune cell changes correlated to fecal SCFA concentrations in this study. Correlations between fecal propionate, acetate and peripheral rTregs (propionate: Spearman rho –0.33, p<
0.05; acetate: Spearman rho –0.34, p
<
0.05) as well as Th17/rTreg ratio (propionate: Spearman rho 0.36, p
<
0.01; acetate: Spearman rho 0.34, p
<
0.05). Spearman rho correlation coefficient is depicted in each graph. NAFL: nonalcoholic fatty liver; NASH: nonalcoholic steatohepatitis; rTreg: resting regulatory T-cell; SCFA: short-chain fatty acid.
Discussion
In this study, gut microbiome was analyzed in 32 NAFLD patients and 27 HCs together with fecal SCFA as functional bacterial metabolites. Differences in microbial composition were rigorously corrected for multiple testing. In our study, NASH patients could be differentiated from NAFL patients based on their intestinal microbiome by higher abundance of Fusobacteria (phylum), Fusobacteriaceae (family), Fusobacterium (species) as well as Prevotella, Eubacterium biforme and Enterobacteriacae_unclassified (Tables 1 and and22).
In past years, different studies showed partly controversial results about changes in the intestinal microbiome of NAFLD patients.1,2,23 These differences could be explained by the complexity of obesity in the context of metabolic syndrome as well as the heterogeneity of methodological data assessment and data interpretation of published studies (including inconsistent correction for multiple testing).24
An increase in the phylum Fusobacterium and/or of some species in this phylum has recently been described in Asian obese patients.25 Fusobacterium is associated with a higher expression and activation of inflammatory signals and has been identified as an important pathogen in patients with acute and chronic periodontitis.26,27 As a functional link between microbiome and active metabolites, higher prevalence of Fusobacterium and correlation to SCFA concentrations in root canals was observed in subgingival biofilm samples from periodontal pockets of type 2 diabetic patients.27 Recent studies indicated an interrelationship between periodontitis and systemic diseases (e.g. cardiovascular disease, type 2 diabetes and NAFLD) through bacterial translocation, bacteremia and increase in circulating inflammatory mediators.28,29 Thus, the higher abundance of Fusobacteria in gut microbiota in our study and the impact of Fusobacteriaceae in the context of metabolic diseases could be attributed to direct or indirect activation of inflammatory signals as well as to production of bacterial metabolites such as SCFAs.
Following recent research fecal SCFA concentrations were analyzed and NAFLD patients were characterized by significantly higher fecal acetate and propionate in our study (Figure 4(a)). Rahat-Rozenbloom et al. observed higher fecal SCFA concentrations in overweight and obese individuals with no difference in rectal SCFA absorption or dietary changes.6 Increased abundance of Fusobacteria, Fusobacteriaceae as well as Prevotellaceae and the above-described changes in species level in NAFLD patients in our study are well in line with higher fecal SCFA concentrations due to predominance of SCFA-producing families and bacteria.19,20,22 In a metagenomic analysis Loomba et al. revealed a different abundance of SCFA production proteins in NAFLD patients with advanced and mild/moderate fibrosis.3 The observed higher predicted abundance of acetate-producing enzymes in the microbiota of mild/moderate NAFLD patients matches higher fecal acetate concentrations in our study population (F0–F1) vs≥
F2 (Figure 4(b)).
Despite increasing evidence of significant changes in various inflammatory diseases, the particular functional role of SCFAs in NAFLD remains to be defined. In light of increased fecal SCFAs in this study, NAFLD patients were characterized by a remarkable positive correlation between fecal propionate/acetate concentrations and peripheral Th17/rTreg ratio as well as a negative correlation to peripheral rTregs as two immunological features of progressive disease (Figure 6). Even though many studies support the idea that SCFAs induce an anti-inflammatory profile of T-cells, there is also evidence that SCFAs induce pro-inflammatory T-cells (e.g. Th1 and Th17) under specific conditions and depending on disease model.11,30
The mechanistic role of SCFAs in the context of obesity and metabolic diseases in humans remains open since intestinal immune cell analysis was not possible in our study given the limitations of a human study of NAFLD and has never been performed elsewhere. Furthermore, in this study a limited number of NAFLD patients were analyzed because of a complex and simultaneous analysis of ex vivo PBMCs, liver histology and analysis of fecal samples. Finally, it is important to note that the present cohort includes a combination of patients undergoing bariatric surgery as well as those without bariatric surgery.
This study explored for the first-time the link between gut microbial changes in NAFLD patients and higher SCFA concentrations in feces as well as the significant correlation to peripheral immune cells as a marker of disease progression in NAFLD. Our data suggest that higher prevalence of SCFA-producing bacteria in feces of NAFLD patients could contribute to disease progression by maintaining low-grade inflammatory processes with influence on circulating immune cells and impact on peripheral target organs such as the liver. The present study further contributes to elucidate the still controversial role of SCFAs in the context of obesity in humans and targets the field for future studies of intestinal immune cell activation to better understand the final impact of fecal SCFAs in humans. Furthermore, increased permeability in the gut-liver axis as well as other bacterial metabolites (e.g. endotoxins, bacterial ethanol) and fecal bile acids are important targets influencing disease progression in NAFLD and should be the focus of future studies together with fecal SCFAs.31,32
Supplemental Material
Supplemental material for Fecal SCFAs and SCFA-producing bacteria in gut microbiome of human NAFLD as a putative link to systemic T-cell activation and advanced disease by Monika Rau, Ateequr Rehman, Marcus Dittrich, Albert K. Groen, Heike M. Hermanns, Florian Seyfried, Niklas Beyersdorf, Thomas Dandekar, Philip Rosenstiel and Andreas Geier in United European Gastroenterology Journal
Acknowledgments
Authors' contributions are as follows: Conception and design: M. Rau and A. Geier. Data collection: M. Rau and A. Geier. Sample management and processing: M. Rau, A. Rehman, A.K. Groen, H.M. Hermanns, F. Seyfried, N. Beyersdorf and P. Rosenstiel. Bioinformatic and statistical analyses: M. Dittrich, T. Dandekar and M. Rau. Data interpretation: M. Rau, M. Dittrich, T. Dandekar and A. Geier. Drafting of the article: M. Rau and A. Geier. Review and approval of the paper: all authors.
Declaration of conflicting interests
None declared.
Funding
This work was supported by the Interdisciplinary Center for Clinical Research (IZKF) Würzburg. Grant number: Z-3/28.
Ethics approval
This study was approved by the local ethics committee (University of Würzburg: EK 96/12, 5 September 2012) and conforms to the ethical guidelines of the 1975 Declaration of Helsinki.
Informed consent
Written informed consent was obtained from all participants.
References
Articles from United European Gastroenterology Journal are provided here courtesy of Wiley
Full text links
Read article at publisher's site: https://doi.org/10.1177/2050640618804444
Read article for free, from open access legal sources, via Unpaywall:
https://onlinelibrary.wiley.com/doi/pdfdirect/10.1177/2050640618804444
Citations & impact
Impact metrics
Citations of article over time
Alternative metrics
Smart citations by scite.ai
Explore citation contexts and check if this article has been
supported or disputed.
https://scite.ai/reports/10.1177/2050640618804444
Article citations
Effect of Mixed Probiotics on Alleviating H1N1 Influenza Infection and Regulating Gut Microbiota.
Foods, 13(19):3079, 27 Sep 2024
Cited by: 0 articles | PMID: 39410114 | PMCID: PMC11475989
A Comparison of the Cecal Microbiota between the Infection and Recovery Periods in Chickens with Different Susceptibilities to Eimeria tenella.
Animals (Basel), 14(18):2709, 18 Sep 2024
Cited by: 0 articles | PMID: 39335298 | PMCID: PMC11428751
The role of intestinal microbiota in physiologic and body compositional changes that accompany CLA-mediated weight loss in obese mice.
Mol Metab, 89:102029, 16 Sep 2024
Cited by: 0 articles | PMID: 39293564 | PMCID: PMC11447304
Soybean oil-based HFD induces gut dysbiosis that leads to steatosis, hepatic inflammation and insulin resistance in mice.
Front Microbiol, 15:1407258, 06 Aug 2024
Cited by: 0 articles | PMID: 39165573 | PMCID: PMC11334085
Gut microbiota, circulating inflammatory proteins and sepsis: a bi-directional Mendelian randomization study.
Front Cell Infect Microbiol, 14:1398756, 08 Aug 2024
Cited by: 0 articles | PMID: 39176264 | PMCID: PMC11338885
Go to all (144) article citations
Data
Data behind the article
This data has been text mined from the article, or deposited into data resources.
BioStudies: supplemental material and supporting data
Similar Articles
To arrive at the top five similar articles we use a word-weighted algorithm to compare words from the Title and Abstract of each citation.
Progression from Nonalcoholic Fatty Liver to Nonalcoholic Steatohepatitis Is Marked by a Higher Frequency of Th17 Cells in the Liver and an Increased Th17/Resting Regulatory T Cell Ratio in Peripheral Blood and in the Liver.
J Immunol, 196(1):97-105, 30 Nov 2015
Cited by: 158 articles | PMID: 26621860
Gut Microbiota Dysbiosis in Patients with Biopsy-Proven Nonalcoholic Fatty Liver Disease: A Cross-Sectional Study in Taiwan.
Nutrients, 12(3):E820, 19 Mar 2020
Cited by: 47 articles | PMID: 32204538 | PMCID: PMC7146257
Role of intestinal flora in the development of nonalcoholic fatty liver disease in children.
Microbiol Spectr, 12(2):e0100623, 08 Jan 2024
Cited by: 0 articles | PMID: 38189294 | PMCID: PMC10846053
Review article: can bugs be drugs? The potential of probiotics and prebiotics as treatment for non-alcoholic fatty liver disease.
Aliment Pharmacol Ther, 50(6):628-639, 02 Aug 2019
Cited by: 29 articles | PMID: 31373710
Review
Funding
Funders who supported this work.