Abstract
Aims
Although cytochromeP450(CYP)3A5 gene polymorphism affects personalized tacrolimus doses, there is no consensus as to whether CYP3A5 genotypes should be determined to adjust the doses. The aims were to compare the therapeutic ranges and clinical outcomes between the conventional and genotype-guided tacrolimus doses.Methods
This randomized controlled study compared 63 cases of the conventional tacrolimus dose group (0.1 mg/kg/day) with 62 cases of the genotype-guided doses group of 0.125, 0.1 and 0.08 mg/kg for CYP3A5*1/*1, *1/*3, and *3/*3 genotypes for the initial 3 days of kidney transplantation. After day 3, dose adjustment occurred in both groups to achieve therapeutic concentrations.Results
The genotype-guided group had an increased proportion of patients with tacrolimus concentrations in the therapeutic range at the steady state on day 3 (40.3 vs 23.8%, P = .048). A lower proportion of over-therapeutic concentration patients was noted in the genotype-guided group in the CYP3A5*3/*3 genotype (9.7 vs 27%, P = .013). Unexpectedly, more delayed graft functions (DGFs) were in the genotype-guided group (41.9 vs 22.2%, P = .018) especially in the CYP3A5*1/*1 participants who might have had an aggravated DGF by a longer ischaemic time and higher serum donor creatinine levels than in the control group. There were no significant differences of glomerular filtration rates or graft or patient survivals over a median 37-month follow-up period.Conclusions
Determination of the CYP3A5 genotype improved therapeutic range achievement. CYP3A5*1/*1 patients who have high risks of DGF should be closely monitored because of an increased risk of DGF and reduced glomerular filtration rate with high tacrolimus doses.Free full text

Therapeutic concentration achievement and allograft survival comparing usage of conventional tacrolimus doses and CYP3A5 genotype‐guided doses in renal transplantation patients
Abstract
Aims
Although cytochromeP450(CYP)3A5 gene polymorphism affects personalized tacrolimus doses, there is no consensus as to whether CYP3A5 genotypes should be determined to adjust the doses. The aims were to compare the therapeutic ranges and clinical outcomes between the conventional and genotype‐guided tacrolimus doses.
Methods
This randomized controlled study compared 63 cases of the conventional tacrolimus dose group (0.1 mg/kg/day) with 62 cases of the genotype‐guided doses group of 0.125, 0.1 and 0.08 mg/kg for CYP3A5*1/*1, *1/*3, and *3/*3 genotypes for the initial 3 days of kidney transplantation. After day 3, dose adjustment occurred in both groups to achieve therapeutic concentrations.
Results
The genotype‐guided group had an increased proportion of patients with tacrolimus concentrations in the therapeutic range at the steady state on day 3 (40.3 vs 23.8%, P = .048). A lower proportion of over‐therapeutic concentration patients was noted in the genotype‐guided group in the CYP3A5*3/*3 genotype (9.7 vs 27%, P = .013). Unexpectedly, more delayed graft functions (DGFs) were in the genotype‐guided group (41.9 vs 22.2%, P = .018) especially in the CYP3A5*1/*1 participants who might have had an aggravated DGF by a longer ischaemic time and higher serum donor creatinine levels than in the control group. There were no significant differences of glomerular filtration rates or graft or patient survivals over a median 37‐month follow‐up period.
Conclusions
Determination of the CYP3A5 genotype improved therapeutic range achievement. CYP3A5*1/*1 patients who have high risks of DGF should be closely monitored because of an increased risk of DGF and reduced glomerular filtration rate with high tacrolimus doses.
1. INTRODUCTION
Tacrolimus (TAC) is a major immunosuppressive drug for preventions of allograft rejections in kidney transplantation (KT) patients. Because of the highly variable pharmacokinetics and narrow therapeutic window of TAC, therapeutic drug monitoring is crucial. Adjustment of TAC dosage to maintain a trough concentration in a therapeutic range (TR) is also mandatory because a low drug concentration increases risk of rejection while a high concentration causes nephrotoxicity. A previous study found delayed graft function (DGF), the requirement for dialysis in the first week, was related to a higher TAC concentration at post‐KT day 4.1 TAC is metabolized to inactive forms by the cytochrome P450(CYP)3A5 enzyme which its gene CYP3A5 polymorphism affects personalized TAC doses.2, 3, 4, 5 CYP3A5 diplotypes, i.e., CYP3A5*1/*1, CYP3A5*1/*3 and CYP3A5*3/*3 determine the phenotype of TAC metabolism as extensive, intermediate and poor metabolizers.6 Individuals who express the CYP3A5*3/*3 allele, therefore, require lower TAC doses than CYP3A5*1/*1 and CYP3A5*1/*3 alleles to keep the desired TR.4, 7, 8, 9, 10, 11, 12, 13, 14, 15, 16 A previous study by the current authors demonstrated that the average dose of TAC for the induction phase in those who have CYP3A5*3/*3, CYP3A5*1/*3 and CYP3A5*1/*1 genotypes were 0.077, 0.097 and 0.142 mg/kg/day.17 Min et al. revealed that in KT patients receiving equal TAC doses, the CYP3A5 expressers i.e., CYP3A5*1/*1 and CYP3A5*1/*3 genotypes, had lower TAC concentrations and a higher incidence of acute cellular mediated rejection than the CYP3A5 non‐expressers (CYP3A5*3/*3).18 Prevalence of CYP3A5 genotypes is different in various ethnicities, for example, the Caucasian proportions of CYP3A5*1/*1, CYP3A5*1/*3 and CYP3A5*3/*3 were 1, 17 and 86%, respectively, while, in the Thai population, they were 13.75, 42.75 and 43.50%.4 Presently, there is no consensus as to whether the genotypes of CYP3A5 should predetermine TAC doses for guidance because little information has been reported regarding the benefit in clinical outcomes.6, 19 Additionally, a few studies reported contradictory results of genotype‐guided TAC dosing as being useful in an achievement of target therapeutic concentrations in the steady state.20, 21 Thervet et al.20 and Pallet et al.22 conducted randomized controlled trials (RCTs) in European centres comparing short‐ and long‐term clinical outcomes between the fixed TAC dosage group and the adjusted TAC doses according to the CYP3A5 genotype group in which the results demonstrated similar renal function and incidence of acute rejection and graft survival. Because of differences in CYP3A5 genotype prevalence among races and the fact that no RCT has been studied in Asian KT patients, the current authors performed this RCT study to compare the proportion of patients with their TAC concentrations in the TR and renal survivals between the conventional TAC dose and the genotype‐guided TAC dose groups.
2. METHODS
2.1. Study design and participants
A randomized controlled study comparing the conventional TAC doses with genotype‐guided TAC doses for the initial 3 days of KT was conducted at the Faculty of Medicine, Srinagarind Hospital, Khon Kaen University, over a 52‐month period from September 2013 to December 2017. The study protocol was approved by the Khon Kaen University Ethics Committee for Human Research, under the project number HE 561064 in accordance with the ethical principles of the Declaration of Helsinki, the Good Clinical Practice guidelines and was registered in the Clinical Trials Registry (Clinicaltrials.gov ID# NCT03173820).
End‐stage renal disease patients aged >18 years and on the waiting list for first‐time KT in the Srinagarind Hospital were eligible to enrol in this study. Exclusion criteria were patients with hypersensitivity to TAC, elevated AST and ALT ≥2 times the normal limits, or total bilirubin ≥1.5 mg/dL. Women patients who were either pregnant or breastfeeding were also excluded. All enrolled patients provided written informed consent and were analysed for CYP3A5 genotype alleles.
2.2. Study protocol and medication
Patients enrolled in this study and who received a cadaveric KT were randomly allocated in a 1:1 ratio into the control group with a fixed initial dose of TAC and the genotype‐guided group. The block of 4 randomization was computerized and the assignments were kept in sealed numbered envelopes. A staff nurse researcher enrolled study patients.
Although genotyping was performed in all study patients, physicians were aware only of the typing of genotype‐guided group findings before surgery (Day 0) to guide the initial dose. The gene expression of patients in the control group was blinded to the attending physicians. Depending on the time of transplantation, the initial dose of oral TAC (tacrolimus, Prograf, Fujisawas, GmbH, Munich, Germany) was given 2–6 hours before the surgery. The initial dose of TAC was 0.1 mg/kg/day in the control group, while those in the genotype‐guided group were 0.125 mg/kg for CYP3A5*1/*1, 0.1 mg/kg for CYP3A5*1/*3 and 0.08 mg/kg for CYP3A5*3/*3 genotypes. The second dose was halved of the loading dose and administered at the nearest 8.00 am or 8.00 pm time which was about 8–16 hours from the loading dose and then the same TAC doses were given every 12 hours at 8.00 am and 8.00 pm until day 3 of transplantation. After day 3, dose adjustment was allowed in both study groups based on the trough concentrations to achieve whole blood TR concentrations of 5–8 ng/mL for the first 4 weeks. From weeks 5 to 12 after transplantation, the TAC doses were adjusted to achieve the target concentrations around 5 ng/mL and from week 13 to week 24, the target concentrations were reduced to 3–5 ng/mL. The trough concentrations of TAC were monitored on days 1, 3, 4, 5, 7 and thereafter on every Monday, Wednesday and Friday until discharge from the hospital. TAC concentrations were also monitored at days 14, 28 and then every month or any other time if clinically indicated (Figure (Figure11).
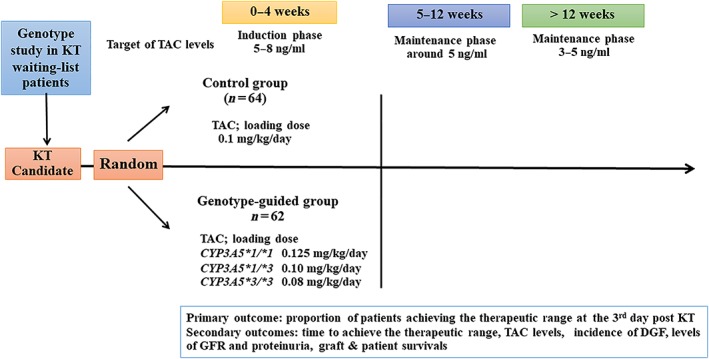
The algorithm of the study design included 126 volunteers who gave written consents. Sixty‐four and 62 volunteers were randomly assigned to the control and the genotype‐guided groups, by using a block of 4 randomization. Each group received different loading and first 3 days of tacrolimus (TAC) doses. One patient in the control group was withdrawn from the study because of the TAC discontinuation. DGF, delayed graft function; GFR, glomerular filtration rate; KT, kidney transplantation
Both study groups received the same administration and doses of immunosuppressive regimens except the initial and first 3 days doses of TAC as described above. For the details, induction therapy with intravenous methylprednisolone 1000 mg was given to all patients on the day of KT (day 0) and the designated induction drug was added; basiliximab 20 mg at days 0 and 4 if the recipients had a human leukocyte antigen‐DR (HLA‐DR) mismatch or more than 5 HLA mismatches or an ischaemic time > 20 hours or panel reactive antibody (PRA) 20–50%, rabbit anti‐human thymocyte immunoglobulin (thymoglobulin 1 mg/kg) if PRA >50%. Methylprednisolone was continued in reduced doses until day 3 and then oral prednisolone (60 mg/day) was started at day 4 and tapered to 5 mg/day within 3 months and this dose was continued if graft function was stable and there were no contraindications. The third combined immunosuppressive drug was mycophenolate mofetil at 1500 mg/day or mycophenolic acid 1080 mg/day. No participant received agents which had inducer or inhibitory effects on CYP3A5.
All participants in both groups were monitored serially for renal function after KT including blood urea nitrogen levels, serum creatinine levels, urinalyses and 24‐hour urine samples for creatinine and protein using the COBAS machine (Roche). Allograft function was assessed at 1‐week of renal transplantation and classified into 3 categories: (i) instant graft function where serum creatinine levels were ≤1.5 mg/dL; (ii) slow graft function where serum creatinine levels were >1.5 mg/dL but with no indications for requiring dialysis, and (iii) delayed graft function when dialysis was indicated in the first week.
2.3. Primary and secondary outcomes
The primary outcome was the proportion of patients whose TAC concentrations were in the TR of 5–8 ng/mL at the steady state on day 3 of KT. The secondary outcomes were the times to achieve the TR, trough concentrations of TAC measured at days 1, 3, 4, 5, 7, 14, 30 and then every month in the first year and every 2 months in the following years. Further monitoring was done for the incidence of DGF, levels of estimated glomerular filtration rate (eGRF) calculated from the Chronic Kidney Disease Epidemiology Collaboration creatinine equation,23 24‐hour urine samples for creatinine clearance and proteinuria at different time points. Graft and patient survival analyses were conducted to compare long‐term outcomes between both groups.
2.4. Genotype analysis and therapeutic drug monitoring
DNA was extracted from peripheral blood leucocytes (QIAamp DNA Blood Mini Kit). A genotyping of CY3A5 was analysed using real‐time polymerase chain reactions (real‐time PCR, PC Viia 7 system; Applied Biosystems, Life Technologies, Foster City, CA, USA) by sequence‐specific PCR primers and probes to identify single nucleotide polymorphisms. CY3A5*1 and CY3A5*3 were determined using Fluorescence Resonance Energy Transfer (TaqMan Assay Reagents for allelic discrimination; Applied Biosystems). Determinations of TAC concentrations from blood samples were performed using MEIA (IMx, Abbot Laboratories, Wiesbaden, Germany).
2.5. Statistical analyses
Sample sizes were calculated for the primary outcomes based on the a priori hypothesis that the higher percentages of patients achieving desired TR of TAC concentrations at day 3 in the genotype‐guided initial dose group were compared to the fixed conventional initial dose group (52.5 vs 30%). Therefore, the required sample size was at least 60 patients in each group to have a power of 80% with an α error of 5% to detect the differences between groups. Based on the assumption of a 5% loss of patients during the study period, a total of 126 patients were enrolled in this study.
All randomized patients were included in the intention‐to‐treat analysis. The analyses compared the genotype‐guided initial dose and the fixed conventional initial dose groups, both using the whole samples and subgroups according to their CYP3A5 genotypes. All data were assessed for normality of distribution and equality of variance. Continuous data were expressed as means ± standard deviation or median (interquartile range) if data were highly skewed. Two‐tailed Student's t‐tests and Mann–Whitney tests were used to compare the means and medians of the 2 groups. Categorical data were presented as percentages and compared by χ2 and Fisher's exact tests. A generalized linear model was used to analyse the relative risk (RR) for factors influencing the DGF. A generalized linear mixed model was used to evaluate the overall mean differences of eGFR. Comparisons of grafts and patient survivals between both groups using a 2‐sided log‐rank test were performed. Graft survival analysis censored the deaths with functioning grafts. The effects of CYP3A5 genotype‐guided doses on graft survival were presented as hazard ratios adjusted with other factors by Cox regression analysis. Two‐sided significance tests were used throughout and a P‐value <.05 was considered to represent a statistically significant difference. All analyses were performed with the STATA version 14.2 and the R program.
3. RESULTS
CYP3A5 genotypes of 879 end‐stage renal disease patients on the waiting list for KT in the Srinagarind Hospital were determined before starting this RCT, which included 139 (15.8%) of CYP3A5 *1/*1, 438 (49.8%) of CYP3A5*1/*3 and 302 (34.4%) of CYP3A5*3/*3 genotypes.
During the inclusion period, September 2013 to January 2016, there were 126 candidate cases for KT who participated in this study. The volunteers were randomly assigned to the control and the genotype‐guided groups, by using a block of 4 randomization. One patient in the control group was withdrawn from the study because he was changed from oral TAC to intravenous cyclosporine immediately after KT. Therefore, 63 and 62 cases of the control and genotype‐guided groups, respectively, were analysed. Their baseline characteristics are presented in Table 1 showing that age, sex, dialysis vintage, mode of dialysis, ABO blood groups, % PRA, HLA mismatch, distribution of CYP3A5 genotypes and ischaemic times were comparable in both groups. Table 2 shows the similar characteristics of deceased donors which may influence renal function after KT: age; sex; marginal and hypertensive donors; durations of shock, receipt of vasopressive drugs, oliguria and admission; and levels of serum creatinine, proteinuria, haematuria and pyuria before harvest.
Table 1
Characteristics of kidney transplantation recipients of the 2 study groups
Characteristics | Control group (n = 63) | Genotype‐guided group (n = 62) |
---|---|---|
Age, mean ± SD (y) | 40.68 ± 10.57 | 41.77 ± 9.84 |
Male sex, n (%) | 43 (68.3) | 37 (59.7) |
Underlying diseases, n (%) | ||
Hypertension | 26 (41.3) | 29 (46.8) |
Chronic glomerulonephritis | 6 (9.5) | 11 (17.7) |
Renal stone | 6 (9.5) | 3 (4.8) |
Diabetes mellitus | 4 (6.3) | 2 (3.2) |
Gout | 3 (4.8) | 1 (1.6) |
Polycystic kidney disease | 1 (1.6) | 1 (1.6) |
Unknown cause | 17 (27.0) | 15 (24.2) |
Mode of previous dialysis, n (%) | ||
Haemodialysis | 49 (77.8) | 39 (62.9) |
Peritoneal dialysis | 14 (22.2) | 23 (37.1) |
Duration of dialysis, mean ± SD (months) | 57.17 ± 38.59 | 62.05 ± 36.40 |
Genotype of CYP3A5, n (%) | ||
*1/*1 | 8 (12.7) | 8 (12.9) |
*1/*3 | 28 (44.4) | 31 (50.0) |
*3/*3 | 27 (42.9) | 23 (37.1) |
ABO blood group, % | ||
A/B/AB/O | 17.5/33.3/9.5/39.7 | 21.0/32.2/9.7/37.1 |
Panel reactive antibody, mean ± SD (%) | 8.52 ± 22.18 | 10.37 ± 25.74 |
Number of HLA mismatches, n (%) | ||
≥2 mismatches | 49 (77.8) | 41 (66.1) |
Number of HLA‐DR mismatches, % | ||
0/1/2 mismatches | 58.7/38.1/3.2 | 53.2/46.8/0 |
Ischaemic time, mean ± SD (h:min) | 16:45 ± 4:44 | 17:56 ± 4:90 |
Receiving IL2 antagonist, n (%) | 31 (49.2) | 38 (61.3) |
Receiving ATG, n (%) | 3 (4.8) | 1 (1.6) |
ATG, antithymocyte globulin; HLA, human leucocyte antigen; IL2, interleukin 2; SD; standard deviation.
Table 2
Characteristics of the deceased donors of the 2 study groups
Characteristics | Control group (n = 63) | Genotype‐guided group (n = 62) |
---|---|---|
Age, mean ± SD (y) | 36.41 ± 13.27 | 39.56 ± 14.05 |
Male sex, n (%) | 50 (79.4) | 48 (77.4) |
Body mass index, mean ± SD (kg/m 2 ) | 23.44 ± 3.47 | 23.31 ± 2.78 |
Causes of brain death, n (%) | ||
Trauma related events | 46 (73.0) | 42 (67.7) |
Nontrauma related events | 17 (27.0) | 20 (32.3) |
Marginal donor, n (%) | 12 (19.1) | 16 (25.8) |
Duration of admission, mean ± SD (h) | 60.45 ± 43.74 | 64.18 ± 56.95 |
Duration of shock, mean ± SD (h) | 6.85 ± 7.33 | 5.35 ± 6.66 |
Duration of oliguria, mean ± SD (h) | 1.68 ± 4.09 | 1.27 ± 4.76 |
Duration of norepinephrine administration, mean ± SD (h) | 15.10 ± 17.47 | 15.99 ± 17.90 |
Duration of dopamine administration, mean ± SD (h) | 21.25 ± 22.55 | 18.40 ± 20.26 |
Serum creatinine before harvest, mean ± SD (mg/dL) | 1.28 ± 0.70 | 1.26 ± 0.66 |
Present of arrest, n (%) | 4 (6.3) | 2 (3.2) |
Level of urine albumin before harvest, % | ||
0/trace/1+/2+/3+ | 62.3/11.5/21.3/4.9/0 | 57.6/18.6/17.0/5.1/1.7 |
Urine red blood cells, mean ± SD (cells/hpf) | 8.75 ± 17.47 | 13.85 ± 18.66 |
Urine white blood cells, mean ± SD (cells/hpf) | 3.21 ± 3.86 | 4.00 ± 5.00 |
hpf, high‐power field; SD; standard deviation.
3.1. Primary outcomes
There were significant differences in the proportion of the participants whose TAC concentrations were at the steady state (day 3) in the TR (5–8 ng/mL), subtherapeutic range (< 5 ng/mL) and over‐therapeutic range (> 8 ng/mL), P = .021. The control group had a lower proportion of TAC concentration achievement (23.8 vs 40.3%, P = .048) and a higher proportion of over‐therapeutic ranges (27 vs 9.7%, P = .013) as shown in Table 3.
Table 3
Tacrolimus level achievement at steady state of the 2 study groups
Control group (n = 63) | Genotype‐guided group (n = 62) | P value | |
---|---|---|---|
Tacrolimus level range, n (%) | .021 | ||
Therapeutic | 15 (23.8) | 25 (40.3) | .048 |
Subtherapeutic | 31 (49.2) | 31 (50.0) | .93 |
Over‐therapeutic | 17 (27.0) | 6 (9.7) | .013 |
3.2. Secondary outcomes
3.2.1. Means and medians of TAC trough concentrations at different time points (Tables S1, S2)
Significantly higher mean TAC concentrations were found in the control group on Day 1. In subgroup analysis categorized by genotypes, the CYP3A5*1/*1 of the genotype‐guided group had a significantly higher mean and median of TAC concentrations at day 3 than the control group. The mean and median of TAC concentrations of CYP3A5*3/*3 genotype in the genotype‐guided group were in the therapeutic range on days 1 and 3, while TAC concentrations in the control group were in the over‐therapeutic range. After day 3, physicians adjusted TAC dosages in all patients to keep concentrations in the TR, therefore, both groups had similar TAC concentrations.
Effects of different genotypes on TAC concentrations were demonstrated within each group; the CYP3A5*3/*3 genotype had significantly higher TAC concentrations compared with the others especially, in the first week post‐KT.
3.2.2. Time to achieve the therapeutic range of TAC concentration (5–8 ng/mL)
There was a trend of significant time differences between the CYP3A5 genotypes of both groups (P = .06). The median times and 95% confidence intervals (CI) of the control group classified as CYP3A5 genotypes, CYP3A5*1/*1, CYP3A5*1/*3 and CYP3A5*3/*3 were 10 (2–29), 2 (1–7) and 4 (3–5) days, while in the genotype‐guided group, the times to achieve the therapeutic ranges in CYP3A5*1/*1, CYP3A5*1/*3 and CYP3A5*3/*3 were 2 (1–12), 4 (2–7) and 3 (2–4) days, as shown in Figure 2.
3.2.3. Incidence of instant or good graft function, slow graft function and DGF
Instant or good graft function was defined as a condition with serum creatinine at day 7 of post‐KT that was ≤1.5 mg/d, while slow graft function (SGF) patients had serum creatinine levels at day 7 >1.5 mg/dL and there was no need for dialysis. DGF meant that the KT patients had to have dialysis within the first week of post‐KT. The incidences of instant or good graft function, SGF and DGF of both groups are shown in Table 4 in which the trend of significance is apparent (P = .058). Unexpectedly, DGF developed in the genotype‐guided group more than the control group (41.9 vs 22.2%, P = .018).
Table 4
Allograft function assessed at 1‐week of renal transplantation
Control group (n = 63) | Genotype‐guided group (n = 62) | P value | |
---|---|---|---|
Allograft function at day 7, n (%) | .058 | ||
Instant graft function | 24 (38.1) | 19 (30.7) | .38 |
Slow graft function | 25 (39.7) | 17 (27.4) | .15 |
Delay graft function | 14 (22.2) | 26 (41.9) | .018 |
All DGF and some SGF patients had allograft biopsies with 26 cases in the control group and 35 cases in the genotype‐guided group. The pathological findings are illustrated in Table S3 with no significant pathological differences of acute tubular necrosis, acute cellular mediated rejection and acute antibody‐mediated rejection (ABMR) between the groups.
Univariate analysis by a generalized linear model demonstrated the factors associated with DGF in the study groups (the genotype‐guided group vs control group, RR 1.89, 95% CI 1.09–3.26, P = .02) as an increase of donor age (for every 1 year older, RR 1.02, 95% CI 1.002–1.04, P = .03), a longer ischaemic time (>18 hours vs ≤ 8 hours, RR 2.61, 95% CI 1.51–4.49, P = .001), serum creatinine of donors before harvesting (>1 mg/dL vs ≤1 mg/dL, RR 2.85, 95% CI 1.43–5.67, P = .003) and the accumulated TAC doses on Days 3 and 7 of KT (Day 3; RR 1.08, 95% CI 1.04–1.12, P < .001, Day 7; OR 1.04, 95% CI 1.02–1.06, P < .001). Multivariate analysis also demonstrated that all of these variables were significantly associated with DGF, focusing on the genotype‐guided group that had a risk of DGF 1.71 times the control group (adjusted RR 1.71, 95% CI 1.03–2.84, P = .04) and increases of accumulated TAC doses that were risks of DGF exemplified by accumulated TAC doses at Day 3; adjusted RR 1.016, 95% CI 1.007–1.02, P < .001 and accumulated TAC doses at Day 7; adjusted RR 1.008, 95% CI 1.003–1.01, P < .001.
Subgroup analysis of CYP3A5 genotypes in both groups revealed that only the CYP3A5*1/*1 participants in the genotype‐guided group had significant differences of DGF compared with the control group (Table 5). When the other factors related with DGF in the CYP3A5*1/*1 patients were considered, the genotype‐guided group had longer ischaemic times (20.9 vs 14.2 hours, P < .001) and higher serum creatinine levels of donors (1.85 vs 1.22 mg/dL, P = .037). Because of low numbers of CYP3A5*1/*1 patients in both groups (8 cases in each group); however, the multivariate analysis to define the magnitude of effects of these higher ischaemic times and serum creatinine levels on DGF observed in the CYP3A5*1/*1 subgroup of the genotype‐guided group was unable to be performed.
Table 5
Occurrence of delayed graft function in the control and genotype‐guided groups classified by CYP3A5 genotypes
Control group (n = 63) | Genotype‐guided group (n = 62) | P value | |
---|---|---|---|
Occurrence of delay graft function, n (%) | |||
CYP3A5*1/*1 | 2 (25.0) | 6 (75.0) | .046 |
CYP3A5*1/*3 | 5 (17.9) | 11 (35.5) | .13 |
CYP3A5*3/*3 | 7 (25.9) | 9 (39.1) | .32 |
3.2.4. GFR at different time points after KT
Estimated GFRs evaluated by the CKD‐EPI formula of both groups are illustrated in Figure 3 in which the general linear mixed model demonstrated that there were no significant mean differences of eGFR at overall time points between the groups (mean differences −2.66, 95% CI −9.42 to 4.10, P = .44). In the subgroups of CYP3A5 genotypes, however, there were significantly lower eGFRs of CYP3A5*1/*1 in the genotype‐guided group compared with the control group (Figures S1, S2).
3.2.5. Graft and patient survival analyses
The mean and median follow‐up times of 125 participants were 36.2 ± 11.9 and 37.1 (27.6–46.8) months. Six patients—1 case in the control group and 5 cases in the genotype‐guided group—expired, 4 of whom had functioning grafts; and 7 different patients had graft failure during the follow‐up time, accounting for incident rates of death and graft failures of 1.33 and 1.99 per 1000 patient–months. Causes of death were 3 severe infections, 1 each of cryptococcal meningitis, cytomegaloviral pneumonia and vancomycin‐resistant enterococci infection, 2 cancers and 1 of cardiovascular disease. Nine graft failures were caused by 2 ABMRs, 2 interstitial fibrosis and tubular atrophy conditions, 1 combined cellular and ABMR, 1 severe vesicoureteral reflux,1 thrombotic microangiopathy, 1 cytomegalovirus vasculitis, and 1 BK nephropathy.
Log‐rank tests comparing the Kaplan–Meier graft and patient survival curves showed no significant differences between the control and genotype‐guided groups, i.e. P = .07 and .09 for graft survival and patient survival. Cox regression analyses of patient and graft survivals revealed the nonsignificant hazard ratio (HR) of the genotype‐guided group as shown in Figure 4, i.e. the unadjusted HR for patient survival was 5.18, 95% CI 0.60–44.3, P = .13 and unadjusted HR for graft survival was 3,78, 95% CI 0.78–18.2, P = .10. Factors associated with patient survival were marginal donors, urine proteinuria concentrations at months 2–4 after KT, presence of ABMR, severity of tubulointerstitial fibrosis of pre‐implanted allografts, and graft failure. Multivariate analysis by adjustment with the related factors showed the adjusted HR of the genotype‐guide group was 2.06 (95% CI 0.14–30.7, P = .60). Univariate analysis showed factors related to graft failures such as donor age, presence of DGF, GFR and proteinuria levels at months 4–24 after KT, and presence of ABMR. After adjustment of these related factors, the adjusted HR for graft survival of the genotype‐guide group was 2.67 (95% CI 0.23–31.4, P = .43).
4. DISCUSSION
The main result of this study was that the genotype‐guided TAC dose group increased the proportion of Thai KT patients with TAC concentrations in TR at the steady state. Additionally, the proportion of over‐therapeutic concentration patients was reduced in the genotype‐guided group especially in the CYP3A5*3/*3 genotype subgroup and there was a trend of increased time to achieve the TR in CYP3A5*1/*1 genotype. These findings were similar to the findings from a study in Caucasian KT patients by Thervet et al., in which CYP3A5*1/*1 and CYP3A5*3/*3 genotype proportions (4.2 and 78.8%) were about 2–3 times different from the present study (12.8 and 40.0%,).20 Therefore, adjustments of TAC doses based on CYP3A5 genotypes guidance improved achievements of target concentrations despite different races.
Although the genotype‐guided group achieved the TR more than the control group, about 50% of patients in both groups still had subtherapeutic tacrolimus concentrations on day 3. Both groups had similar genotype distributions in the subtherapeutic group, i.e., CYP3A5*1/*1, CYP3A5*1/*3 and CYP3A5*3/*3 were 25.8, 58.1, 16.1% in the control group and 22.6, 54.8, 22.6% in the genotype‐guided group (P = .81). In addition to genotypes of CYP3A5, multiple factors influenced the pharmacokinetics of TAC, including hepatic and renal functions, patient age, haematocrit and albumin concentrations, food intake, level of P‐glycoprotein expression, degree of inflammation, and change of drug volume distribution.24, 25 These factors should be taken into consideration for the possible reasons of subtherapeutic range.
Although a higher proportion of the genotype‐guided group achieved the TR, the overall renal outcomes including eGFR, renal pathologies and graft survivals were similar in both groups as found in the study by Thervet et al.20 These findings may suggest that other factors, not only the TAC concentrations that slightly fluctuate from the TR in a short time, may affect renal function. Further studies that evaluate direct effects of TAC on an immune system such as alteration of lymphocyte function or cytokines are warranted in an assessment of TAC effects rather than only monitoring TAC concentrations.
DGF and lower eGFRs were more frequent in the CYP3A5*1/*1 genotype subgroup of the genotype‐guided group, even though their TAC concentrations were not in the over‐therapeutic range. They received higher TAC doses compared with the control group and the multivariate analysis revealed the association of DGF with accumulated TAC doses at days 3 and 7. Therefore, physicians should be careful in increasing TAC dosage in patients who have the CYP3A5*1/*1 genotype to reach TR, especially if they had already received high TAC doses or had high risks of DGF such as receiving allografts with a prolonged ischaemic time, older donor ages and high levels of serum creatinine before harvest. An alternative approach is to either adjust the other immunosuppressive drugs so that high dose TAC could be avoided early in the time after transplantation or usage of other drugs, for example, diltiazem, that have interaction by decreasing TAC clearance resulting in the need for a lower TAC dosage. Additionally, recent studies demonstrated the distinct pharmacokinetics between extended‐release TAC and immediate‐release TAC formulas, therefore requiring different TAC doses in various CYP3A5 polymorphisms and ethnicities.26, 27, 28 Further RCTs are needed to confirm an effect of the extended‐release TAC formula on renal outcomes in different CYP3A5 genotypes.
A study of CYP3A5 genotypes of the 879 KT waiting list of Thai patients found the frequency of CYP3A5*3 was similar to the previous reports of Asian populations, for example, Vietnamese, Chinese, Japanese and Indian races. These frequencies, however, were lower than those in the Caucasian populations and higher than those from Africa. Previous studies revealed that the further away from the equator, the higher the prevalence of CYP3A5*3.29 Therefore, TAC doses required in various geographic areas might be different. This current study supports that pretransplant knowledge of CYP3A5 genotypes benefits in increasing achievement of TR. If patients had the CYP3A5*1/*1 genotype, they needed higher TAC doses. If, however, they had high risks of DGF, increasing the TAC dosage should be cautious. By contrast, TAC dose and cost can be reduced in patients with CYP3A5*3/*3 especially in races with a high frequency of CYP3A5*3/*3 such as Caucasians. Although the current Clinical Pharmacogenetics Implementation Consortium guidelines do not recommend testing for the CYP3A5 genotype in transplants, they provide recommendations on how to use CYP3A5 genotype information if it is known.6
The limitation of this study was that no protocol allograft biopsies were performed; hence, some subclinical pathologies such as possible rejection or acute tubular necrosis might not be revealed. Results of secondary outcomes—graft function, graft and patient survival—should be interpreted with caution because this study was mainly directed to study the primary outcome of the proportion of the study patients whose TAC concentrations were at the steady state. Due to the limited number of 8 cases of CYP3A5*1/*1 genotypes in each group and a higher risk of DGF in the genotype‐guided group, it was difficult to interpret an effect of the intervention in this subgroup. Finally, the CYP3A5 genotypes of donors, which might affect the renal outcomes, were not determined in this study.
In conclusion, adjustment of TAC dosage in the CYP3A5 genotypes improved the proportion of patients with TR achievement in the steady state. This benefit was shown in the CYP3A5*3/*3 patients who needed lower TAC doses. CYP3A5*1/*1 patients who had high risks of DGF and received high TAC doses had an increased risk of DGF and reduced GFR.
COMPETING INTERESTS
C.P. has been funded by the Astellas Pharma Europe Limited for another study that is not related to the current submitted article. The other authors of this manuscript have no competing interests to declare.
CONTRIBUTORS
S.A., C.P., K.K. and S.V. created the research idea, study design and data acquisition. S.A., C.P., K.K. and S.V. performed the study. S.A., C.L. and S.V. analysed and interpreted the data. S.A., S.V. and, C.L. wrote the manuscript.
Supporting information
Table S1.
Comparison of mean and median tacrolimus concentrations between the 2 study groups
Table S2. Mean and median tacrolimus concentrations categorized by the genotypes of CYP3A5 during first 30 days
Table S3. Pathology of allograft biopsies observed in the patients who had slow and delayed graft function in the control and genotype‐guided groups
Figure S1. Glomerular filtration rates across the follow‐up time period in the control and genotype‐guided groups classified as CYP3A5 genotypes
Figure S2. Glomerular filtration rates across the follow‐up time period in the genotype subgroups of the control and genotype‐guided groups; (A) genotype *1/*1 (B) genotype *1/*3 and (C) genotype *3/*3
ACKNOWLEDGEMENTS
We would like to acknowledge Prof. James A will for editing the manuscript via Publication Clinic KKU, Thailand.
The study was supported by funding from the National Science and Technology Development Agency (project number P‐12‐01486), Faculty of Medicine, Khon Kaen University and the Chronic Kidney Disease Prevention in the Northeast of Thailand (CKDNET) research funds.
Notes
Anutrakulchai S, Pongskul C, Kritmetapak K, Limwattananon C, Vannaprasaht S. Therapeutic concentration achievement and allograft survival comparing usage of conventional tacrolimus doses and CYP3A5 genotype‐guided doses in renal transplantation patients. Br J Clin Pharmacol. 2019;85:1964–1973. 10.1111/bcp.13980 [Europe PMC free article] [Abstract] [CrossRef] [Google Scholar]
The authors confirm that the PI for this paper is Sirirat Anutrakulchai and that she had direct clinical responsibility for patients.
REFERENCES
Articles from British Journal of Clinical Pharmacology are provided here courtesy of British Pharmacological Society
Full text links
Read article at publisher's site: https://doi.org/10.1111/bcp.13980
Read article for free, from open access legal sources, via Unpaywall:
https://bpspubs.onlinelibrary.wiley.com/doi/pdfdirect/10.1111/bcp.13980
Citations & impact
Impact metrics
Citations of article over time
Article citations
Early CYP3A5 Genotype-Based Adjustment of Tacrolimus Dosage Reduces Risk of De Novo Donor-Specific HLA Antibodies and Rejection among CYP3A5-Expressing Renal Transplant Patients.
Diagnostics (Basel), 14(19):2202, 02 Oct 2024
Cited by: 0 articles | PMID: 39410605 | PMCID: PMC11475898
Cost-effectiveness analysis of CYP3A5 genotype-guided tacrolimus dosing in solid organ transplantation using real-world data.
Pharmacogenomics J, 24(3):14, 15 May 2024
Cited by: 1 article | PMID: 38750044
A systematic review and meta-analysis recite the efficacy of Tacrolimus treatment in renal transplant patients in association with genetic variants of CYP3A5 gene.
Am J Clin Exp Urol, 11(4):275-292, 15 Aug 2023
Cited by: 3 articles | PMID: 37645617 | PMCID: PMC10461032
Review Free full text in Europe PMC
Higher number of tacrolimus dose adjustments in kidney transplant recipients who are extensive and intermediate CYP3A5 metabolizers.
Clin Transplant, 37(4):e14893, 11 Jan 2023
Cited by: 3 articles | PMID: 36571802 | PMCID: PMC10089949
Tacrolimus Concentration Is Effectively Predicted Using Combined Clinical and Genetic Factors in the Perioperative Period of Kidney Transplantation and Associated with Acute Rejection.
J Immunol Res, 2022:3129389, 09 Sep 2022
Cited by: 4 articles | PMID: 36118414 | PMCID: PMC9481373
Go to all (12) article citations
Data
Data behind the article
This data has been text mined from the article, or deposited into data resources.
BioStudies: supplemental material and supporting data
Clinical Trials
- (1 citation) ClinicalTrials.gov - NCT03173820
Similar Articles
To arrive at the top five similar articles we use a word-weighted algorithm to compare words from the Title and Abstract of each citation.
Impact of the CYP3A5 genotype on the distributions of dose-adjusted trough concentrations and incidence of rejection in Japanese renal transplant recipients receiving different tacrolimus formulations.
Clin Exp Nephrol, 21(5):787-796, 07 Mar 2017
Cited by: 4 articles | PMID: 28271256
The TOMATO Study (Tacrolimus Metabolization in Kidney Transplantation): Impact of the Concentration-Dose Ratio on Death-censored Graft Survival.
Transplantation, 104(6):1263-1271, 01 Jun 2020
Cited by: 28 articles | PMID: 31415035
Polymorphism of the CYP3A5 gene and its effect on tacrolimus blood level.
Exp Clin Transplant, 13 Suppl 1:197-200, 01 Apr 2015
Cited by: 13 articles | PMID: 25894154
Pharmacogenetic considerations for optimizing tacrolimus dosing in liver and kidney transplant patients.
World J Gastroenterol, 19(48):9156-9173, 01 Dec 2013
Cited by: 55 articles | PMID: 24409044 | PMCID: PMC3882390
Review Free full text in Europe PMC
Funding
Funders who supported this work.
Khon Kaen University (1)
Grant ID: Chronic Kidney Disease Prevention in the Northeast of Thailand
National Science and Technology Development Agency (1)
Grant ID: P‐12‐01486