Abstract
Introduction
Determination of the target-binding fraction (TBF) of radiopharmaceuticals using cell-based assays is prone to inconsistencies arising from several intrinsic and extrinsic factors. Here, we report a cell-free quantitative method of analysis to determine the TBF of radioligands.Methods
Magnetic beads functionalized with Ni-NTA or streptavidin were incubated with 1 μg of histidine-tagged or biotinylated antigen of choice for 15 min, followed by incubating 1 ng of the radioligand for 30 min. The beads, supernatant and wash fractions were measured for radioactivity on a gamma counter. The TBF was determined by quantifying the percentage of activity associated with the magnetic beads.Results
The described method works robustly with a variety of radioisotopes and class of molecules used as radioligands. The entire assay can be completed within 2 h.Conclusion
The described method yields results in a rapid and reliable manner whilst improving and extending the scope of previously described bead-based radioimmunoassays.Advances in knowledge
Using a bead-based radioligand binding assay overcomes the limitations of traditional cell-based assays. The described method is applicable to antibody as well as non-antibody based radioligands and is independent of the effect of target antigen density on cells, the choice of radioisotope used for synthesis of the radioligand and the temperature at which the assay is performed.Implications for patient care
The bead-based radioligand binding assay is significantly easier to perform and is ideally suited for adoption by the radiopharmacy as a quality control method of analysis to fulfill the criteria for release of radiopharmaceuticals in the clinic. The use of this assay is likely to ensure a more reliable validation of radiopharmaceutical quality and result in fewer failed doses, which could ultimately translate to an efficient release of radiopharmaceuticals for administration to patients in the clinic.Free full text

A Rapid Bead-Based Radioligand Binding Assay for the Determination of Target-Binding Fraction and Quality Control of Radiopharmaceuticals
Abstract
Introduction:
Determination of the target-binding fraction (TBF) of radiopharmaceuticals using cell-based assays is prone to inconsistencies arising from several intrinsic and extrinsic factors. Here, we report a cell-free quantitative method of analysis to determine the TBF of radioligands.
Methods:
Magnetic beads functionalized with Ni-NTA or streptavidin were incubated with 1 μg of histidine-tagged or biotinylated antigen of choice for 15 min, followed by incubating 1 ng of the radioligand for 15 mins. The beads, supernatant and wash fractions were measured for radioactivity on a gamma counter. The TBF was determined by quantifying the percentage of activity associated with the magnetic beads.
Results:
The described method works robustly with a variety of radioisotopes and class of molecules used as radioligands. The entire assay can be completed within 2 hours.
Conclusion:
The described method yields results in a rapid and reliable manner whilst improving and extending the scope of previously described bead-based radioimmunoassays.
Advances in Knowledge:
Using a bead-based radioligand binding assay overcomes the limitations of traditional cell-based assays. The described method is applicable to antibody as well as non-antibody based radioligands and is independent of the effect of target antigen density on cells, the choice of radioisotope used for synthesis of the radioligand and the temperature at which the assay is performed.
Implications for Patient Care:
The bead-based radioligand binding assay is significantly easier to perform and is ideally suited for adoption by the radiopharmacy as a quality control method of analysis to fulfill the criteria for release of radiopharmaceuticals in the clinic. The use of this assay is likely to ensure a more reliable validation of radiopharmaceutical quality and result in fewer failed doses, which could ultimately translate to an efficient release of radiopharmaceuticals for administration to patients in the clinic.
INTRODUCTION
Quantification of the target-binding fraction (TBF) of radioligands including radiolabeled antibodies, peptides and small molecules is a critical quality attribute and prerequisite for the release and use of radiopharmaceuticals in the preclinical as well as clinical setting. TBF is the fraction of the antibody which retains its binding to the cognate target despite undergoing modifications including bioconjugation and radiolabeling. This parameter is commonly referred to as “immunorcactivity” or “immunorcactivc fraction ” (IRF) by researchers and radiopharmacists in the field of nuclear medicine. However, given the immunologic connotation of this term to describe an exogenous agent’s ability to evoke an immune response in a host, the usage of “immunorcactivity” to describe a feature of radiopharmaceuticals might be a misnomer. Furthermore, the term “immunorcactivity” is seldom used in the context of non-antibody-based synthetic radiopharmaceuticals such as PSMA- or somatostatin receptor-targeting peptide analogs and the evaluation of their target-binding ability. Thus, we propose the use of TBF as a more broadly applicable term instead of immunoreactivity.
For more than 3 decades, the TBF of antibody-based radiopharmaceuticals has been evaluated using cell-based assays, most of which are adaptations and modifications of the extensively cited method of Lindmo et al. [1] Arguably, cell-based radioligand binding assays can provide useful information about TBF; however, they can be cumbersome to perform and are often plagued by inconsistencies attributed to several intrinsic and extrinsic factors. A major intrinsic factor that contributes to the variability in results comes from the cells themselves. Specifically, variability in expression of the target protein due to intercellular heterogeneity, expression of multiple target isoforms, target shedding, and genomic and epigenetic instability are factors that can affect the robustness and reproducibility of these assays. Assay reproducibility can be negatively impacted by inter-laboratory differences in the source and passaging history of established cell lines. Furthermore, cell-based assays based on the method of Lindmo are not ideal for the assessment of radioligands that bind to antigens having inherently low levels of expression and abundance on the cell surface.[2, 3] Additionally, the vast majority of cell-based assays used for the evaluation of antibody-based radiopharmaceuticals do not block or account for Fc-mediated binding of antibodies to cells, which can contribute to high background binding.
Next, extrinsic factors such as the number of data points included in the linear regression analysis of the double inverse plot in a Lindmo assay dramatically affects the slope of the curve, its intercept on the ordinate and the associated correlation coefficient factor, resulting in reduced precision and reliability of repeat measurements.[2] Cell-based assays and the results thereof are also subject to variations arising from the radioisotope (residualizing versus non-residualizing) used for labeling the antibody and the temperature used for incubating the radioligand with cells. This is most applicable to cases wherein the Lindmo method has been modified to perform incubation of radioligand(s) with cells at room temperature or 37 °C instead of 4 °C. Cellular internalization of the target-bound radioligand impacts the slope of the double-inverse plot in a Lindmo assay, and this effect may be highly pronounced in the case of antibodies labeled with residualizing radiometals. On the other hand, radioiodinated antibodies that bind to internalizing targets are metabolized within lysosomes to release monoiodotyrosines and/or radioiodide, which are effluxed by the target cells.[4] In contrast, the TBF obtained from the bead-based radioligand binding assay is independent of the dynamics of cellular internalization, temperature and choice of radioisotope used.
In the early 1990s, Rhodes and coworkers reported a solid phase method of analysis using affinity thin layer chromatography to evaluate the immunoreactive fraction of radiolabeled antibodies.[5, 6] In 2001, Zalutsky developed and reported a bead-based solid-phase radioimmunoassay to evaluate immunoreactivity.[7] Surprisingly, however, a search of the wider literature revealed a very limited use of these platforms beyond the reports of Rhodes, Zalutsky and colleagues. In contrast to their infrequent use in the scientific literature, biotechnology companies have adopted bead-based binding assays for the routine evaluation of the immunoreactivity of antibody-based radioimmunoconjugates. However, there is a lack of published methods available to the wider community including scientists and radiopharmacists who are engaged in the preclinical and clinical development of radiopharmaceuticals.
To overcome the limitations of cell-based assays whilst extending the scope for the use of a cell-free method of analysis, we report a rapid bead-based radioimmunoassay that can be easily adopted and executed by scientists and trainees in preclinical research laboratories as well as technicians in the clinical radiopharmacy.
MATERIALS AND METHODS
Our method replaces cells with commercially available 1-micron diameter magnetic beads functionalized with Ni-NTA (Nickel-Nitrilotriacetic acid; HisPur™ Ni-NTA magnetic beads; 88831; Thermo Scientific) or streptavidin (65601; DynaBeads™ MyOne™ Streptavidin Tl; Invitrogen™). The assay design comprises 3 arms, and each arm analyzes samples in triplicates. The first arm serves as a control to account for non-specific binding (NSB) of the radioligand to beads lacking the target antigen, the second arm assesses radioligand binding to antigen-coated beads, and the third arm validates the specificity of radioligand binding to the cognate antigen in the presence of an excess of unlabeled ligand.
To demonstrate the scope and utility of our method, the following target antigen (Ag) and antibody (Ab) or small molecule (SM) combinations were assayed – Histidine (His)-tagged DLL3 (Ag) with [89Zr]Zr-DFO-SC16.56 (Ab) and [177Lu]Lu-CHX-A”-DTPA-SC16.56 (Ab); His-tagged B7-H3 (Ag) with [131I]I-Omburtamab (Ab); His-tagged versus biotinylated Her2 (Ag) with [89Zr]Zr-DFO-Trastuzumab (Ab); polyvalent biotinylated sialyl-LewisA antigen (Ag) with [89Zr]Zr-DFO-5B1 (Ab); His-tagged PSMA (Ag) with [177Lu]Lu-PSMA-617 (SM).
His-tagged DLL3 protein and the SC16.56 antibody were kindly provided by AbbVie Stemcentrx; polyvalent biotinylated sLea-PAA (01-044) was purchased from Glycotech, and the 5B1 antibody was kindly provided by MabVax Therapeutics Holdings Inc.; biotinylated human Her2 protein (HE2-H822R-200μg) was purchased from Acro Biosystems, and both Trastuzumab and Pertuzumab were purchased from the pharmacy at MSKCC; recombinant human His-tagged B7-H3 protein (1949-B3-50) was purchased from R&D Systems, and Omburtamab was kindly provided by Y-mabs Therapeutics; recombinant human Her2 Fc-chimera His-tagged protein (1129-ER-050) and recombinant human His-tagged PSMA/FOLH1 protein (4234-ZN-010) were purchased from R&D Systems; PSMA-617 (9933) was obtained from ABX.
An overview of the method is shown in Fig 1. Briefly, each sample was prepared by aliquoting 20 μL of the magnetic bead slurry into a 1.5 mL lo-bind microcentrifuge tube (13-698-794; Fisher Scientific). The beads were washed by adding 380 μL of phosphate-buffered saline containing 0.05% Tween-20 (PBS-T), and the tubes were vortexed for 5 seconds followed by a brief spin in a minicentrifuge prior to placing the tubes on a magnetic rack (12321D; DynaMag™−2; ThermoFisher Scientific) for 30-45 sec to isolate the magnetic beads. The antigen was resuspended as per manufacturer instructions to achieve a concentration of a 0.1 mg/mL. The washed beads were resuspended in 390 μL of PBS-T and the beads in all tubes except the control arm were incubated with 1 μg (10 μL) of His-tagged or biotinylated antigen for 15 min on a rotating mixer at room temperature. Subsequently, the beads were washed once with 400 μL of PBS-T before adding 1 ng of the radioligand resuspended in 1% BSA-PBS or PBS-T. The radioligand was incubated with antigen-coated beads for 30 min on a rotating mixer at room temperature. A large excess (1-5 μg) of the unlabeled ligand was added a few seconds prior to adding 1 ng of the radioligand to antigen-coated beads in the blocking arm. Thereafter, the beads were isolated using a magnet, and the supernatant containing unbound radioligand was aspirated with a pipette and collected in separate tubes. To remove non-specifically-bound radioligand, the beads were washed twice with 400 μL of PBS-T. Finally, the beads, supernatant and washes were measured for radioactivity on a gamma counter. The radioligand TBF was determined from the percentage of total activity bound to antigen-coated magnetic beads minus the percentage of non-specific binding in the control arm.
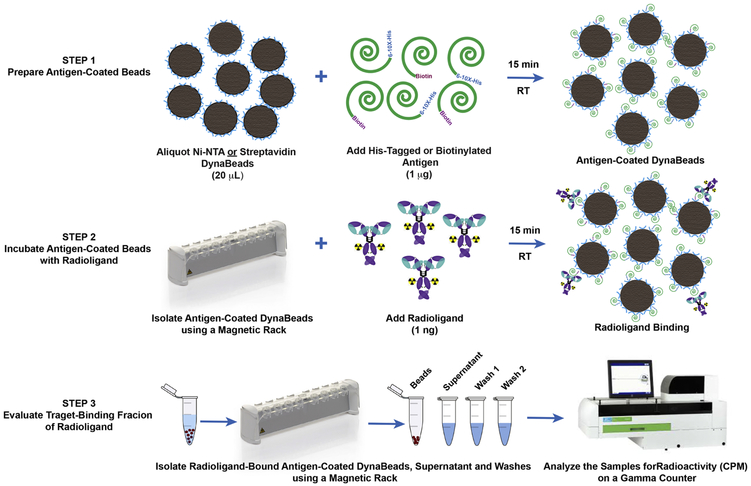
The first step of the assay involves preparation of the antigen-coated magnetic beads. The second step allows the radioligand to bind with the target antigen on the beads. The final step isolates the beads from the supernatant and washes to quantify the target-binding fraction of the radioligand.
Statistical Analysis:
All data presented are expressed as means ± SD unless stated in the figure legend. Where applicable, statistical differences were analyzed by unpaired, two-tailed, Student’s t-test using GraphPad Prism 7 software. The two-sided F-test of equality of variances was calculated using the R statistical computing environment. Comparisons with p-values <0.05 were considered statistically significant.
RESULTS AND DISCUSSION
Unlike traditional cell- and bead-based assays, which evaluate immunoreactive fraction from radioligand binding under conditions of infinite antigen excess using a range of antigen concentrations and mathematical analysis of Scatchard plots and linear extrapolation, our assay is extremely easy to set up, execute and analyze results thereof. Regardless of the nature of the target antigen or the radioligand, the current method uses a fixed quantity of magnetic beads coated with a fixed amount of target antigen (1 μg), which is allowed to bind with a fixed amount (1 ng) of the radioligand.
To highlight the practical advantage(s) for using our method of analysis over established cell-based assays for the quality control of radiopharmaceuticals, we present five different scenarios in this brief communication. First, we present a case wherein the Lindmo assay does not qualify as a method of analysis due to the low level of expression and abundance of the target antigen – DLL3 (Delta-like protein 3) on SCLC (small cell lung cancer) cells. In a previous report, we replaced the Lindmo assay with a standard radioligand binding assay to evaluate the TBF of [89Zr]Zr-DFO-SC16.56 with DLL3-expressing H82 cells. [8] The low expression of DLL3 (10-14 × 103 copies per cell) necessitated the use of 50 million H82 cells per tube to provide sufficient antigen excess, amounting to 450 million cells per assay that were used to evaluate the TBF of [89Zr]Zr-DFO-SC16.56 versus 10 million BT474 cells per tube/90 million cells per assay required to assess the TBF of [89Zr]Zr-DFO-Trastuzumab to HER2, which is expressed in the order of 1-2 × 106 copies per BT474 breast cancer cell.
Importantly, performing the cell-based radioligand binding assay at the end of synthesis (EOS) and 24 h after radiosynthesis of [89Zr]Zr-DFO-SC16.56 would mandate having greater than 1 billion H82 cells in culture at a given time for use in 2 rounds of the cell-based assay done within 24 hours of each other. Despite the extensive effort, time and resources spent in cell culture, the cell-based assay did not yield TBF values > 80% at EOS across n=4 trials done within a span of 4 weeks (Fig. 2A). Surprisingly the TBF of [89Zr]Zr-DFO-SC16.56 was low at EOS and high at 24 h after radiosynthesis in trials 3 and 4. In sum, the cell-based radioligand binding assay yielded suboptimal, inconsistent and unreliable results when billions of H82 cells, which grow as floating spheres, were sub-cultured to execute the assays. Plausibly, the need to use an exceedingly high number of cells to simulate conditions of antigen excess for a target with inherently low cell surface abundance may have led to suboptimal culture conditions or inadvertently allowed a subpopulation of antigen-negative cells to outgrow the antigen-positive cells – leading to the inconsistent results.
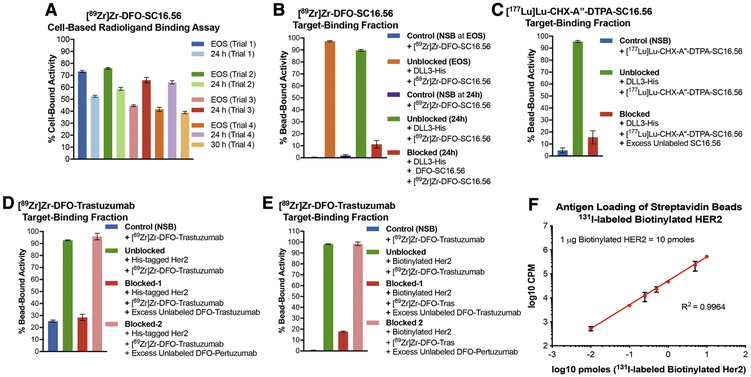
A) Inconsistent results obtained from cell-based assays to determine the target-binding fraction (TBF) of [89Zr]Zr-DFO-SC16.56 (molar activity = 14 MBq/nmol) at end of synthesis (EOS) and 24 h after storage of the sample at −80 °C in 4 consecutive experimental trials done over a period of 4 weeks; B) Demonstration of high TBF for [89Zr]Zr-DFO-SC16.56 using Ni-NTA beads coated with His-tagged DLL3 and minimum non-specific binding (NSB) to naked Ni-NTA beads at (EOS) and 24 h later; C) High TBF demonstrated by [177Lu]Lu-CHX-A”-DTPA-SC16.56 (molar activity = 22.4 MBq/nmol) – a radioimmunotherapeutic variant of the DLL3-targeting antibody. Specificity of binding to antigen-coated beads for both the DLL3-targeting radioligands was validated in the presence of a 3500-fold excess of unlabeled SC16.56 immunoconjugates; D) Evaluation of the TBF of [89Zr]Zr-DFO-Trastuzumab (molar activity = 14 MBq/nmol) using Ni-NTA beads coated with His-tagged Her2 protein showed ~25% NSB of the radioligand to naked Ni-NTA beads; E) Using streptavidin beads coated with biotinylated Her2 to evaluate the TBF of [89Zr]Zr-DFO-Trastuzumab eliminated the NSB and demonstrated high specific binding, which could be blocked by excess unlabeled DFO-Trastuzumab, but not DFO-Pertuzumab; F) Using 131I-labeled biotinylated Her2 to determine the degree of loading of the streptavidin beads demonstrated linearity with increasing concentration of the antigen over at least 4 orders of magnitude. The error bars on each data point represent 95% CI. The plot validates that the bead-based assay is being operated in the pre-saturation concentration range when 1 μg (10 pmoles) of biotinylated Her2 was used with 20 μL (200 μg) of streptavidin beads.
Conversely, switching to the bead-based assay showed minimal non-specific binding of [89Zr]Zr-DFO-SC16.56 whilst yielding > 90% TBF at EOS. Despite a marginal drop in the TBF upon storage of the radioimmunoconjugate for 24 h after radiosynthesis, the TBF was high and specific, considering the minimal non-specific uptake and efficient blockade of [89Zr]Zr-DFO-SC16.56 binding achieved from coincubation of the radioligand with a 2500 – 3000-fold (2.5 – 3.0 μg) excess of the unlabeled DFO-SC16.56 immunoconjugate (Fig. 2B). To circumvent the potential limitation of cell-based assays being prone to cell-death and loss of structural integrity of the antibody carrying a therapeutic radionuclide, we tested a 177Lu-labeled variant of the DLL3-targeting SC16.56 antibody in the bead-based assay set up. The bead-based assay yielded high TBF for [177Lu]Lu-CHX-A”-DTPA-SC16.56 at EOS and showed efficient blockade in the presence of excess unlabeled ligand, both of which were comparable to its 89Zr-labeled diagnostic counterpart (Fig. 2C).
In contrast to the condition of low target abundance described above, the second case describes 89Zr-labeled Trastuzumab – a clinically relevant immunoPET tracer that binds to Her2, which has a high abundance on the surface of several cancer cells.[9] Specifically, this case highlights “non-specific binding” (NSB) – an important criterion that should be considered while adopting the bead-based radioimmunoassay as a quality control method of analysis for radiopharmaceuticals. Performing the bead-based assay using Ni-NTA beads coated with His-tagged Her2 yielded > 90% TBF for [89Zr]Zr-DFO-Trastuzumab. Furthermore, the binding of [89Zr]Zr-DFO-Trastuzumab to Her2 could be blocked in the presence of a 1000-1500 fold (1 – 1.5 μg) excess unlabeled DFO-Trastuzumab but was agnostic to the presence of the same excess of DFO-Pertuzumab, which binds to a different domain of Her2.[10] However, the assay displayed a high degree (~25%) of NSB of [89Zr]Zr-DFO-Trastuzumab to Ni-NTA beads that were not coated with the target antigen. To overcome this limitation, we modified our protocol by replacing Ni-NTA beads with streptavidin beads and His-tagged Her2 with biotinylated Her2. Execution of the assay with the modified protocol yielded more favorable results including minimum NSB, high TBF, and radioligand binding that could still be blocked in the presence of an excess of DFO-Trastuzumab but was insensitive to the presence of DFO-Pertuzumab. Finally, the loading of streptavidin beads with biotinylated antigen was assessed by performing a titration of 131I-labeled-Her2 (0.01, 0.1, 0.25, 0.5, 1, 5 and 10 pmoles) using 20 μL of beads (1 mg/mL) (Fig. 1F). Despite loading slightly above the prescribed theoretical limit of 8 pmoles of biotinylated per 20 μg of the streptavidin beads, the linearity of the plot suggested that our bead-based assays were performed within the pre-saturation concentration range of the target antigen(s).
Moving on, the third case highlights the poor performance of cell-based assays for the assessment of the TBF of radioiodinated antibodies. Cellular catabolism of internalized radioiodinated antibodies releases monoiodotyrosines, which can yield unreliable and misleading results in cell-based assays, leading to the portrayal of low immunoreactivity for this class of radiopharmaceuticals.[11-14] Fig. 3A exemplifies this by summarizing a Lindmo assay-based evaluation of [131I]I-Omburtamab – a radiotherapeutic agent that binds to B7-H3 (CD276) expressed on the surface of metastatic central nervous system neuroblastoma cells including pediatric brain tumors.[15-17] Using the cell-based assay to analyze [131I]I-Omburtamab yielded low %IRF (~ 72%) at EOS, which progressively decreased to ~ 50% at 76 h after radiosynthesis. On the other hand, testing the same batch of [131I]I-Omburtamab with the bead-based assay (Fig. 3B) demonstrated ~ 90% TBF at EOS, with a slight drop in TBF at 76 h plausibly due to 131I-induced radiolysis of the sample upon storage. The specificity of radioligand binding to His-tagged B7-H3 protein was demonstrated by substituting [131I]I-Omburtamab with [89Zr]Zr-DFO-Pertuzumab, which yielded low NSB. Case in point, using a cell-based assay to assess the TBF of radioiodinated antibodies may lead to failed patient doses if acceptance criterion for quality control and release of the radiopharmaceutical agent are not fulfilled. Having multiple such “failed doses” is not only a drain on resources, but also a somewhat unjustified exposure of the technicians to high doses of ionizing radiation during the process of radiosynthesis and performing quality control in the radiopharmacy.
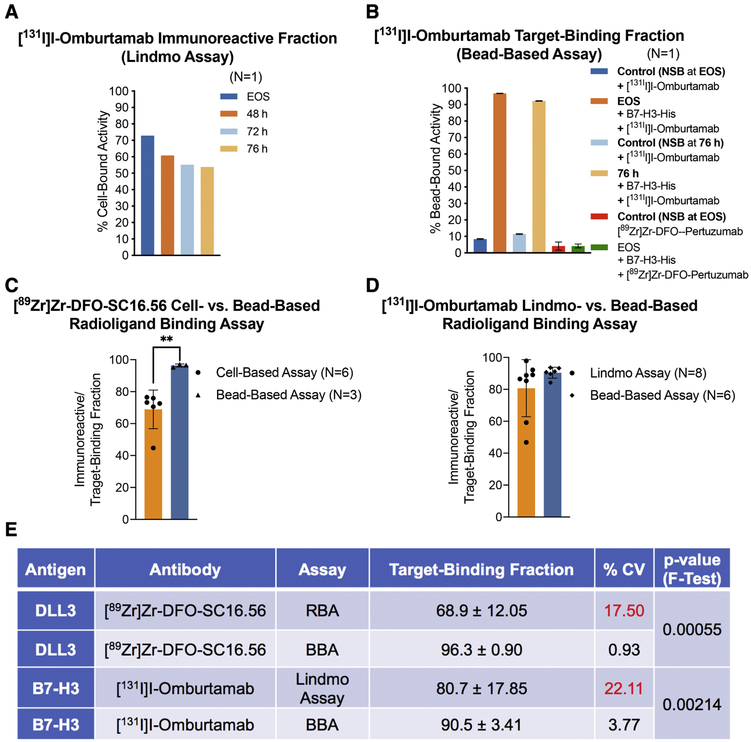
A) Poor and progressively decreasing immunoreactive fractions obtained from Lindmo assay of [131I]I-Omburtamab (molar activity = 392 MBq/nmol) with B7-H3-expressing LAN-1 cells at EOS, 48 h, 72 h and 76 h after radiosynthesis (radioligand samples/aliquots stored at −80 °C; B) High TBFs obtained for the same preparation of [131I]I-Omburtamab with His-tagged B7-H3 in a bead-based assay performed at EOS and 76 h after storage of the sample at −80 °C; C-D) Bar graphs showing a comparative analysis of the means from multiple experiments to evaluate the performance of cell-based radioligand binding assay (RBA) versus bead-based assay (BBA); E) Summarized statistics for the two methods of analyses showing superior intermediate precision obtained from use of BBA (%CV <5; F-test of equality of variances p-value <0.005).
A comparison of the performance of the two assays for analyzing [89Zr]Zr-DFO-SC16.56/DLL3 and [131I]I-Omburtamab/B7-H3 in multiple experiments summarized in figs. 3C--3E3E revealed that the cell-based radioligand binding assay had a wider range arising from an occasionally failed dose (low immunoreactive fraction); whereas the bead-based assay consistently yielded high (>90 %) TBFs. A comparison of the coefficient of variation (fig. 3E) derived from using the two assays confirms a significantly better intermediate precision (same assay performed on different days) yielded by the bead-based assay (BBA < 4%) compared to the cell-based radioligand binding assay (RBA/Lindmo assay >10%) (p=0.00055 and 0.00214 for [89Zr]Zr-DFO-SC16.56/DLL3 and [131I]I-Omburtamab/B7-H3, respectively, by F-test of equal variances). The BBA also yielded excellent and consistent results in both the radioligand antibody/antigen systems when transferred from the development lab to the radiopharmacy for routine validation runs, thus indicating high reproducibility.
The fourth case represents target antigens that are shed from the surface of cells. Mucins and carbohydrate antigens fall under this category and may yield low or variable immunoreactivity based on how much antigen is present on the surface of the cell during the course of performing the assay. Antigen shedding can limit the vast majority of the applied radioactivity to the supernatant fractions in a cell-based assay and may misrepresent the target-binding fraction of the radioligand. To demonstrate the utility of the bead-based assay for this class of antigens, we evaluated the binding of [89Zr]Zr-DFO-5B1 to biotinylated polyvalent sialyl-LewisA antigen or carbohydrate antigen CA19.9 – a serum biomarker for pancreatic cancer.[18, 19] The bead-based assay yielded ≥ 90% TBF for [89Zr]Zr-DFO-5B1 and demonstrated minimal NSB. Availability of the bead-based radioligand binding assay could serve as a more robust and reliable quality control method of analysis for the evaluation and release of this clinically relevant immunoPET tracer. Notably, challenging [89Zr]Zr-DFO-5B1 with a 1000-fold excess of the unlabeled DFO-5B1 immunoconjugate was unable to impact the degree of radioligand binding (Fig. 4A). This might be attributed to the polyvalency of the target antigen, which warrants the need to use a higher excess of the unlabeled ligand to reduce/block the bead-bound radioactivity.
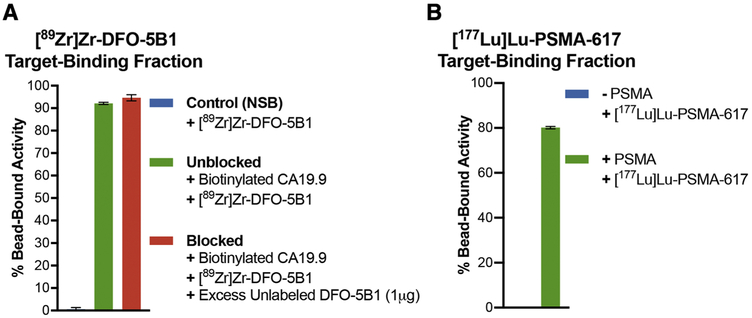
A) High TBF of [89Zr]Zr-DFO-5B1 (molar activity =13 MBq/nmol) with polyvalent biotinylated CA19.9 antigen; C) Demonstration of the utility of the bead-based assay with small molecule radioligand – [177Lu]Lu-PSMA-617 (molar activity = 2.6 MBq/nmol) showing high (~80%) TBF and minimum NSB at 24 h post radiosynthesis.
Finally, as a proof of concept, we tested the scope of the bead-based assay to evaluate the TBF of small molecule radioligands. Performing the assay with 0.5 μg of His-tagged PSMA as the target antigen coated on Ni-NTA functionalized magnetic beads demonstrated a high TBF of [177Lu]Lu-PSMA-617 at 24 h after radiosynthesis with minimum NSB of the radioligand to beads without PSMA (Fig. 4B).
Before we conclude, it is important to issue four disclaimers. First, one advantage of the traditional radioligand binding assays including the Lindmo assay is the inherent capability to evaluate the KD (affinity constant) of the radioligand and Bmax (maximum number of target antigen/receptor) per cell. Whilst the bead-based assay is amenable to modifications that would allow the evaluation of the KD of radioligands, the simplified bead-based assay as described here only assesses the TBF. Secondly, unlike easily accessible conformational epitopes on single-pass membrane proteins such as DLL3, multi-pass transmembrane proteins such as G-protein coupled receptors (GPCRs), which rely on cell membrane topology to successfully bind antibody ligands may not be suitable for evaluation in a bead-based assay. Thirdly, the development of preclinical animal models should continue to rely on cell-based assays to inform and validate the choice of cell lines to be used for in vivo experiments and to assess the number of surface exposed targets per cell. Therefore, the bead-based assay should be considered a complementary method of analysis in addition to cell-based radioligand binding assays, flow cytometry or surface plasmon resonance during the preclinical development of a radiopharmaceutical. Finally, the bead-based assay may be ideally suited for use in the clinical radiopharmacy, where KD and Bmax are not typical release criteria and the TBF is used as a primary measure of radiopharmaceutical quality.
CONCLUSION
Herein, we report a simple and easy to set up, execute and interpret cell-free method of analysis for the assessment of target-binding fraction of radioligands. The bead-based assay is broadly applicable in the preclinical and clinical settings and is amenable to testing a wide variety of radioligands which bind to different classes of target antigens. Specifically, the development and reporting of this method will benefit the clinical radiopharmacy, which currently lacks a method of analysis that can yield results in a rapid and reproducible manner to serve as a quality control for the release of radiopharmaceuticals in downstream applications including validation of novel radiopharmaceuticals and subsequent dosing of patients.
ACKNOWLEDGEMENTS
The authors thank AbbVie Stemcentrx for providing the SC16.56 antibody, MabVax Therapeutics Holdings Inc., for providing the 5B1 antibody, and Y-mabs Theraputics for providing the Omburtamab antibody.
Financial Support: The authors gratefully acknowledge the Radiochemistry and Molecular Imaging Probe core, which was supported in part by NIH grant P30 CA08748. This work is supported in part by NIH grants R01 CA213448-01 (JTP and JSL), U01 CA213359-01 (JTP) and P01CA190174. The authors thank the Mr. William H. and Mrs. Alice Goodwin, the Commonwealth Foundation for Cancer Research, and the Center for Experimental Therapeutics of Memorial Sloan Kettering Cancer Center. SKS acknowledges the postdoctoral fellowship from the Tow Foundation.
Footnotes
FINANCIAL DISCLOSURE
JSL has received research funding from MabVax Therapeutics Holdings, Inc. There are no other potential conflicts of interest.
Publisher's Disclaimer: This is a PDF file of an unedited manuscript that has been accepted for publication. As a service to our customers we are providing this early version of the manuscript. The manuscript will undergo copyediting, typesetting, and review of the resulting proof before it is published in its final citable form. Please note that during the production process errors may be discovered which could affect the content, and all legal disclaimers that apply to the journal pertain.
REFERENCES
Full text links
Read article at publisher's site: https://doi.org/10.1016/j.nucmedbio.2019.04.005
Read article for free, from open access legal sources, via Unpaywall:
http://manuscript.elsevier.com/S0969805119301076/pdf/S0969805119301076.pdf
Citations & impact
Impact metrics
Article citations
Water solubility and folate receptor affinity-driven plasma membrane-targeted carbon dots for cancer cell imaging.
RSC Adv, 14(47):34816-34822, 31 Oct 2024
Cited by: 0 articles | PMID: 39483381 | PMCID: PMC11526347
Optimizing the Therapeutic Index of sdAb-Based Radiopharmaceuticals Using Pretargeting.
J Nucl Med, 65(10):1564-1570, 01 Oct 2024
Cited by: 0 articles | PMID: 39266288 | PMCID: PMC11448608
Theranostic GPA33-Pretargeted Radioimmunotherapy of Human Colorectal Carcinoma with a Bivalent <sup>177</sup>Lu-Labeled Radiohapten.
J Nucl Med, 65(10):1611-1618, 01 Oct 2024
Cited by: 0 articles | PMID: 39168519
CEACAM5-Targeted Immuno-PET in Androgen Receptor-Negative Prostate Cancer.
J Nucl Med, 65(7):1043-1050, 01 Jul 2024
Cited by: 0 articles | PMID: 38782457
Cadherin-17 as a target for the immunoPET of adenocarcinoma.
Eur J Nucl Med Mol Imaging, 51(9):2547-2557, 16 Apr 2024
Cited by: 0 articles | PMID: 38625402 | PMCID: PMC11223962
Go to all (36) article citations
Similar Articles
To arrive at the top five similar articles we use a word-weighted algorithm to compare words from the Title and Abstract of each citation.
Erratum: Preparation of Poly(pentafluorophenyl acrylate) Functionalized SiO2 Beads for Protein Purification.
J Vis Exp, (146), 30 Apr 2019
Cited by: 0 articles | PMID: 31038480
Peptide protein binding assay using ImageFlashPlates or Imaging Beads.
Comb Chem High Throughput Screen, 7(8):763-770, 01 Dec 2004
Cited by: 3 articles | PMID: 15578938
More advantages in detecting bone and soft tissue metastases from prostate cancer using 18F-PSMA PET/CT.
Hell J Nucl Med, 22(1):6-9, 01 Jan 2019
Cited by: 33 articles | PMID: 30843003
In vitro receptor binding assays: general methods and considerations.
Q J Nucl Med Mol Imaging, 52(3):245-253, 13 May 2008
Cited by: 25 articles | PMID: 18475249
Review
Funding
Funders who supported this work.
NCI NIH HHS (5)
Grant ID: P01 CA190174
Grant ID: P30 CA008748
Grant ID: U01 CA213359
Grant ID: R01 CA172546
Grant ID: R01 CA213448