Abstract
Free full text

Biogenic selenium nanoparticles synthesized by Lactobacillus casei ATCC 393 alleviate intestinal epithelial barrier dysfunction caused by oxidative stress via Nrf2 signaling-mediated mitochondrial pathway
Abstract
Background: Selenium (Se) can exert antioxidative activity and prevent the body from experiencing oxidative injury. Biogenic Se nanoparticles (SeNPs) synthesized by probiotics possess relatively strong chemical stability, high bioavailability, and low toxicity, this makes them potential Se supplements. Previously, we demonstrated that SeNPs synthesized by Lactobacillus casei ATCC 393 can alleviate hydrogen peroxide (H2O2)-induced human and porcine intestinal epithelial cells' oxidative damage. However, the antioxidant mechanism remains unclear.
Methods: The possible antioxidant mechanism and protective effect of SeNPs on intestinal epithelial permeability and mitochondrial function were evaluated by establishing an H2O2-induced oxidative damage model of human colon mucosal epithelial cells (NCM460) and conducting Nrf2 inhibitor interference experiments. Mitochondrial membrane potential (MMP), mitochondrial DNA content, adenosine triphosphate (ATP), ROS, and protein expression levels of Nrf2-related genes were determined. Mitochondrial ultrastructure was visualized by transmission electron microscopy.
Results: An amount of 4 μg Se/mL of SeNPs synthesized by L. casei ATCC 393 alleviated increase of ROS, reduced ATP and MMP, and maintained intestinal epithelial permeability in NCM460 cells challenged by H2O2. In addition, SeNPs improved the protein levels of Nrf2, HO-1, and NQO-1. Moreover, SeNPs attenuated the damage of mitochondrial ultrastructure caused by oxidative stress. Nrf2 inhibitor (ML385) abolished the regulatory effect of SeNPs on intracellular ROS production.
Conclusion: Data suggest that biogenic SeNPs synthesized by L. casei ATCC 393 can protect the intestinal epithelial barrier function against oxidative damage by alleviating ROS-mediated mitochondrial dysfunction via Nrf2 signaling pathway. Biogenic SeNPs are an attractive candidate for potential Se supplement agent in preventing oxidative stress-related intestinal disease by targeting mitochondria.
Introduction
Nanotechnology is a vigorous research and innovation area which affects our daily lives in many ways. Nanoparticles have abundant applications in biomedicine, agriculture, and the environment etc, due to their unique physical and chemical properties.1–4 In recent years, biological synthesis of nanoparticles using biological entities such as microorganisms, algae, and plants have been regarded as “green processes” due to its rapidity, cost-effectiveness, and eco-friendly nature compared with traditional physical and chemical methods.5,6 Moreover, various non-metallic, metallic including metal oxide nanoparticles synthesized via biological technique exhibit various biological activities.7–10 Among the non-metallic nanoparticles, selenium nanoparticles (SeNPs) have attracted extensive attention due to their exclusive physicochemical properties including chemical stability, biocompatibility, and low toxicity. Biogenic SeNPs have wide applicability in the field of nanomedicine as antimicrobial, antioxidant, and anticancer agents.6,11 Furthermore, SeNPs are considered to be a potential and novel nutritional supplement for Se.12
The intestinal epithelial barrier function is the key component of intestines to prevent the invasion of pathogenic antigen. In order to maintain intestinal homeostasis, the intestinal epithelial barrier must be tightly regulated. If intestinal barrier dysfunction is not effectively controlled, it will result in inflammatory bowel disease (IBD). Mitochondria, as the core of cell viability and function, control many physiological metabolic processes.13,14 Mitochondria are crucial for the regulation of intestinal epithelial barrier function. Mitochondrial dysfunction could cause abnormal intestinal barrier function. Therefore, mitochondria may be a potential target to treat IBD.15 The “openness” of the mitochondrial permeability transition pore could cause the overproduction of ROS and release of Cytc,16 which could result in intestinal mucosal injury.17,18
The intestines are more susceptible to oxidative stress. Therefore, it is urgent to explore safe and effective antioxidants to alleviate intestinal disorders caused by oxidative stress. SeNPs are a highly effective antioxidant. SeNPs exert antioxidative effects in a variety of ways, including scavenging free radicals, activating antioxidative selenase activity such as GPx, and enhancing the activity of other key enzymes in the body’s antioxidant defense system.19 In previous studies, we found that biogenic SeNPs synthesized by Lactobacillus casei ATCC 393 had the ability to protect intestinal epithelium cells (IPEC-J2 and NCM460 cells) from oxidative injury.20 However, the potential antioxidative mechanism remains unclear.
To determine whether biogenic SeNPs synthesized by L. casei ATCC 393 could alleviate oxidative stress-induced intestinal epithelial barrier dysfunction via Nrf2 signaling-mediated mitochondrial pathway, we established the oxidative damage model of human colon mucosal epithelial cells (NCM 460) induced by hydrogen peroxide (H2O2) and conducted an Nrf2 inhibitor interference experiment to detect intestinal epithelial permeability, the mitochondrial membrane potential (MMP), mitochondrial DNA (mtDNA) content, adenosine triphosphate (ATP) and ROS production, protein expression levels of Nrf2, NQO-1, and HO-1. The ultrastructure of mitochondria was visualized by transmission electron microscopy (TEM).
Materials and methods
Microorganism and culture conditions
L. casei ATCC 393 strain was kept in our laboratory. deMan, Rogosa and Sharpe (MRS) broth was purchased from Sigma-Aldrich Co. (St Louis, MO, USA). L. casei ATCC 393 used for biosynthesis of SeNPs was cultivated with MRS at 37°C without shaking.
Reagents
Sodium selenite, fluorescein isothiocyanate-dextran (FITC-dextran) with average molecular weight 4,000 and H2O2 were purchased from Sigma-Aldrich Co.. Nrf2 inhibitor (ML385) was purchased from MedChemExpress (Shanghai, People's Republic of China). All reagents for cell culture were purchased from Life Technologies (Thermo Fisher Scientific, Waltham, MA, USA). Mito-Tracker Green Kit, ATP Assay Kit, MMP Assay Kit, ROS detection Kit, and Cell Counting Kit-8 (CCK-8) were purchased from Beyotime Biotechnology (Shanghai, People's Republic of China). RIPA lysis buffer and bicinchoninic acid (BCA) protein assay kit were purchased from Solarbio Life Sciences Biotech Co. (Beijing, People's Republic of China). Nrf2, NQO-1, and HO-1 primary antibodies and corresponding secondary antibody were purchased from Abcam Biotechnology (Cambridge, MA, USA). Genomic DNA extraction Kit was purchased from OMEGA Bio-Tek (Norcross, GA, USA). ChamQTM SYBR® qPCR Master Mix Kit was purchased from Vazyme (NanJing, People's Republic of China).
Cell culture
NCM460 cells were purchased from Cell Resource Center, Shanghai Institute, Chinese Academy of Sciences (Shanghai, People's Republic of China). NCM460 cells were cultured in DMEM/high glucose medium with 10% FBS and 1% antibiotic mixture (100 U/mL of penicillin and 100 μg/mL streptomycin) at 37°C under a humidified atmosphere containing 5% CO2.
Preparation of SeNPs
SeNPs were prepared by the method established in our laboratory.20
Effect of SeNPs on cell viability of NCM460 exposed to H2O2
NCM460 cells (1×103 cells per well) were seeded into 96-well plates (Corning Incorporated, Corning, NY, USA) overnight to allow cells' attachment. Cells from SeNPs groups were cultivated with 4 μg Se/mL of SeNPs for 12 hours based on our previous study.20 Other groups were given an equal volume of FBS-free medium. Then, cells from H2O2-model and SeNPs protective groups were exposed to 500 μM H2O2 for 6 hours. Cell viability was evaluated with CCK-8 according to the manufacturer’s instructions.
Intestinal epithelial ATP levels
An amount of 2×105 NCM460 cells were seeded into 6-well plates (Corning Incorporated). After overnight cultivation, cells from SeNPs groups were cultivated with 4 μg Se/mL of SeNPs for 12 hours. Other groups were administered an equal volume of FBS-free medium. After that, cells from H2O2-model and SeNPs protective groups were exposed to 500 μM H2O2 for 6 hours. After treatment, intracellular ATP was measured with ATP Assay Kit according to the corresponding protocol using GloMax®-96 Microplate Luminometer (Bio-Rad Laboratories Inc., Hercules, CA, USA).
Mito-tracker green staining
Cell treatment methods were the same as described previously. Mito-Tracker Green is a mitochondria green fluorescent probe that can be used for mitochondrial-specific fluorescent staining of living cells. After treatment, cells were cultivated with 100 nM Mito-Tracker Green for 30 minutes at 37°C. After washing three times with serum-free medium, the cellular mitochondria were visualized by fluorescence microscope (Leica Microsystems, Wetzlar, Germany).
MMP (
ψm) assay
MMP of experimental cells was detected by MMP Assay Kit with JC-1 staining. Cell treatment methods were the same as described previously. After that, cells were washed three times with phosphatic buffer solution (PBS) and stained with JC-1 for 20 minutes. Then, cells were washed three times with JC-1 buffer after JC-1 solution was discarded. Finally, images were captured with a Laser Scanning Confocal Microscope (Leica sp5, Leica Microsystems). Green monomeric JC-1 and red aggregated JC-1 were detected at an emission wavelength of 530 nm and 590 nm, respectively. Moreover, MMP was also detected by Multi-function Microplate Reader (Bio-Rad Laboratories Inc.) with JC-1 staining in the same manner as described previously.
ROS detection and quantification
Cell treatment methods were the same as described previously. After treatment, intracellular ROS were measured by ROS detection Kit. Briefly, cells were incubated with 10 μM 2ʹ,7ʹ-dichlorodihydrofluorescein diacetate (DCFH-DA) for 20 minutes. Then, cells were washed three times with FBS-free medium to remove DCFH-DA. The levels of intracellular ROS were determined by FACSCalibur Flow Cytometry (BD Biosciences, San Jose, CA, USA) at an excitation wavelength of 480 nm and emission wavelength of 515 nm. In addition, intracellular ROS were observed by Fluorescence Microscope (Leica Microsystems).
Measurement of intestinal epithelial mtDNA copy number
mtDNA copy number was determined according to a previously reported method with slight modification.21 Primer sequences for real-time PCR assay were as follows: mt D-Loop sense 5ʹ- GATCGTACATAGCACATATCATGTC-3ʹ, anti-sense 5ʹ-GGTCCTGAAGTAAGAACCAGATG-3ʹ; β-actin sense 5ʹ- CCCCTCCTCTCTTGCCTCTC-3ʹ, anti-sense 5ʹ- AAAAGTCCTAGGAAAATGGCAGAAG-3ʹ.21 Firstly, total DNA was extracted from NCM460 cells by genomic DNA extraction Kit. Real-time PCR was performed with a ChamQTM SYBR® qPCR Master Mix Kit. The PCR amplification system had 20 µL reaction volume consisting of 1 µL DNA, 10 µL 2×ChamQ SYBR qPCR Master Mix, 0.5 µL each of sense and anti-sense primers, and 8 µL ddH2O. Then, PCR reaction was performed on CFX96 TouchTM Real-Time PCR Detection System (Bio-Rad Laboratories Inc.) according to the following conditions: 95°C for 10 seconds, 50 cycles including 95°C for 10 seconds, 55°C for 30 seconds, and 72°C for 30 seconds. The relative mtDNA copy number was analyzed by 2−Ct method.
Intestinal epithelial permeability analysis
An amount of 1×105 NCM460 cells per well were seeded into 24-well transwell plates with a pore size of 0.4 μm (Corning Incorporated). Cell treatment methods were the same as detailed previously. After treatment, transendothelial electrical resistance (TER) was determined by Millicell Electrical Resistance System (Millipore, Billerica, MA, USA). Moreover, effects of SeNPs on the paracellular passage of FITC-dextran (4 kDa) in NCM460 exposed to H2O2 were evaluated. Briefly, after treatment, 100 μL of FITC-dextran (2.2 μg/mL) was added to the upper chamber of transwells and co-incubated for 2 hours. After that, 100 μL of culture medium at the apical side and basolateral side were collected, respectively. Concentration of FITC-dextran in medium was detected by Multi-function Microplate Reader (Bio-Rad Laboratories Inc.) at an excitation wavelength of 490 nm and an emission wavelength of 520 nm.
Ultrastructure of mitochondria observed by TEM
An amount of 5×105 NCM460 cells were seeded into a 25 cm2 flask and cultivated until they reached 80%~90% confluence. Then, cells were exposed to H2O2 for 6 hours after treatment with 4 μg Se/mL of SeNPs for 12 hours. After that, cells were collected and washed three times with PBS, then fixed with 2.5% glutaraldehyde overnight at 4°C. After samples were pretreated according the standard procedures including staining, dehydration, and embedding, and slicing ultra-thin sections, the ultrastructure of mitochondria was observed on a Techni 20 transmission electron microscope.
Expression levels of Nrf2 signaling-associated proteins analyzed by Western blot
Cell treatment methods were the same as described previously. After treatment, cells were washed three times with PBS. Total protein was extracted by homogenizing cells with 200 µL RIPA lysis buffer. Protein concentrations were measured by the BCA protein Assay Kit. Samples containing approximately 40 μg protein were boiled with 5× loading buffer at 95°C for 5 minutes and separated by 10% sodium dodecyl sulfate polyacrylamide gel electrophoresis, and then transferred to polyvinylidene difluoride membrane (Millipore, Massachusetts, USA). After being blocked with 5% skim milk blocking buffer for 2 hours at room temperature, the membranes were incubated with the primary antibodies for Nrf2, HO-1, NQO-1, and β-actin at 4°C overnight. Subsequently, the membranes were washed three times with Tris buffered saline with Tween 20, and then incubated with secondary antibodies for 1 hour at room temperature. After that, the immunoreactive bands were visualized by Clarity Western ECL substrate Kit (BioRad Laboratories Inc.). The gray values of the bands were analyzed by the built-in software.
Effect of Nrf2 inhibitor on cell viability and ROS production
Nrf2 inhibitor (ML385) was dissolved in 100% DMSO to prepare a stock solution, and then diluted with DMEM/high glucose medium containing 0.5% DMSO.22 Firstly, NCM460 cells were exposed to different concentrations of ML385 (0.2, 0.5, 1, 2, 5, 10, 15 μM) for 12 hours. After that, cell viability was determined by CCK-8. Moreover, NCM460 cells were cultivated with 4 µg/mL of SeNPs and/or 5 µM ML385 for 12 hours, followed by treatment with 500 μM H2O2 for 6 hours. Then, the effect of ML385 on the protein expression levels of Nrf2 were evaluated by Western blot analysis. In addition, intracellular ROS levels in each experimental group were measured by the ROS detection Kit with DCFH-DA staining.
Statistical analysis
All experimental data were statistically analyzed by SPSS v.19.0 statistical software (IBM Corporation, Armonk, NY, USA) and are shown as mean ± standard error of mean (SEM). All assays were performed in at least three independent experiments. The statistical significance was calculated by one-way ANOVA or Student’s t-test. Differences were considered to be significant at P<0.05.
Results
Establishment of H2O2-induced cell injury model
Results showed that treatment with 500 μM H2O2 for 12 hours significantly affected the viability of NCM460 cells with inhibitory ratio of 56% (Figure 1A). Compared to the H2O2 model group, pretreatment with 4 μg Se/mL of SeNPs significant alleviated the toxic effects of 500 μM H2O2 on NCM460 cells. However, exposure to 500 μM H2O2 for 6 hours did not exhibit an inhibitory effect on the growth of NCM460 cells (Figure 1B). Therefore, exposure to 500 μM H2O2 for 6 hours at 37°C was chosen as the conditions to induce NCM460 cells' oxidative damage in the following experiments.
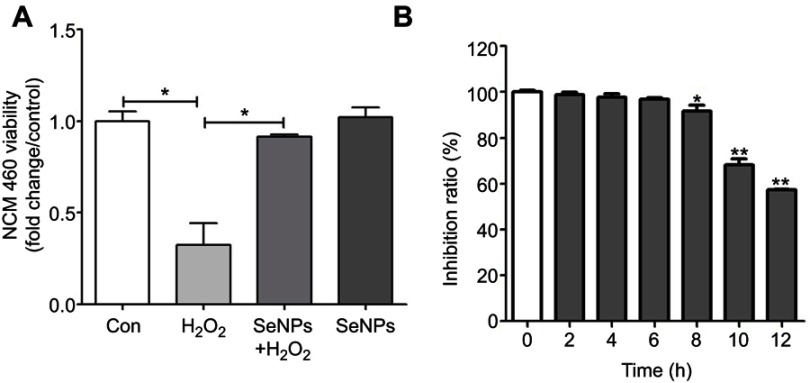
The viability of NCM460 cells treated with H2O2 and/or SeNPs. (A) NCM460 cells treated with 4 μg Se/mL of SeNPs for 12 hours before being challenged by 500 μM H2O2 for 6 hours. (B) NCM460 cells treated with 500 μM H2O2 for 2, 4, 6, 8, 10 or 12 hours. Cell viability was determined by CCK-8. All data were presented as mean ± SEM of three separate experiments. *P<0.05, **P<0.01.
Abbreviations: H2O2, hydrogen peroxide; SeNPs, selenium nanoparticles; CCK-8, Cell Counting Kit-8; SEM, standard error of mean.
SeNPs alleviates H2O2-induced intestinal epithelial barrier dysfunction
As shown in Figure 2A, treatment with SeNPs before exposure to H2O2 did not affect the TER of cells. However, H2O2 obviously caused a reduction in TER after NCM460 cells were exposed to H2O2 for 6 hours, which suggests that H2O2-induced oxidative stress destroyed the intestinal epithelial barrier function and the models were successfully established. Pretreatment with SeNPs significantly attenuated the reduction of TER caused by H2O2. Moreover, compared with the normal control, FITC-dextran fluxes across NCM460 cells in H2O2-model group increased 1.49-fold. In contrast, pretreatment with SeNPs significantly alleviated the H2O2-induced increase of FITC-dextran permeation (Figure 2B).
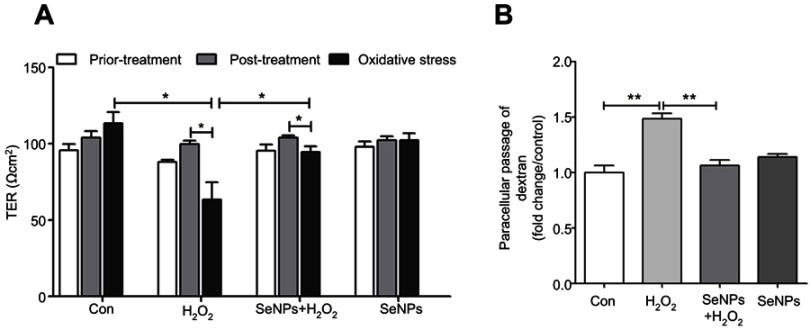
Effect of SeNPs on intestinal epithelial permeability. (A) TER after different treatment in NCM460 cells. (B) Effect of SeNPs on FITC-dextran fluxes across NCM460 cells exposed to H2O2. All data were presented as mean ± SEM of three separate experiments. *P<0.05, **P<0.01.
Abbreviations: Con, control; SeNPs, selenium nanoparticles; TER, transepithelial electrical resistance; FITC, fluorescein isothiocyanate; H2O2,hydrogen peroxide; SEM, standard error of mean.
SeNPs inhibits H2O2-induced ROS production
Effect of SeNPs on redox state of NCM460 cells challenged by oxidative stress was evaluated by detecting intracellular ROS production. DCFH can be oxidized to DCF by ROS, which stimulates the green fluorescence and indicates ROS levels. Results showed that H2O2 caused the increase of ROS production when compared with control group. However, pretreatment with SeNPs obviously alleviated the increase of ROS caused by H2O2 (Figure 3A–C).
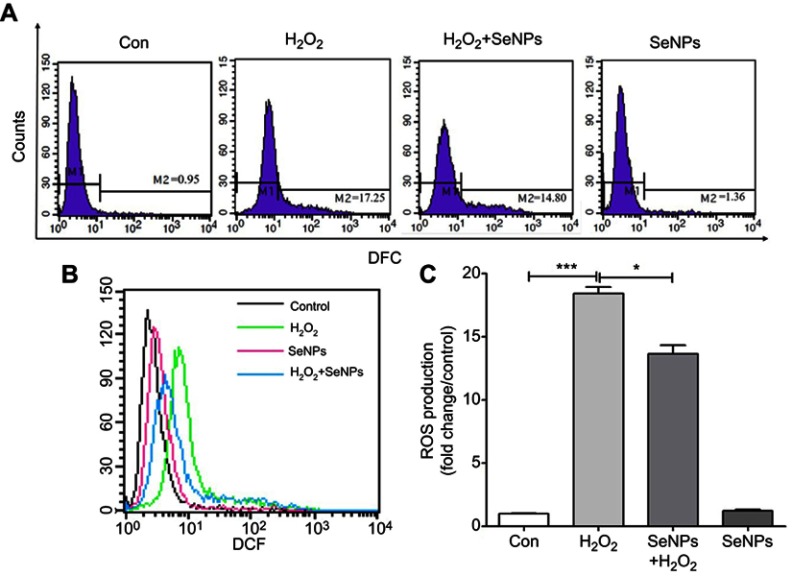
SeNPs inhibit H2O2-induced ROS production. (A) Intracellular ROS were detected by flow cytometry with DCFH-DA staining. (B) Comparative analysis of ROS production. (C) Quantitative analysis of the cellular ROS levels (fold change/control). All data were presented as mean ± S.E.M of three separate experiments. *P<0.05, ***P<0.001.
Abbreviations: Con, control; DFC, 2',7'-dichlorofluorescein; SeNPs, selenium nanoparticles; H2O2, hydrogen peroxide; DCFH-DA, 2ʹ,7ʹ-dichlorodihydrofluorescein diacetate; SEM, standard error of mean.
SeNPs attenuates H2O2-caused mitochondrial dysfunction
Intracellular ATP levels were determined after treatment with H2O2 and/or SeNPs. Results showed that exposure to H2O2 significantly reduced intracellular ATP levels when compared with the normal control group, which indicated that H2O2 resulted in mitochondrial dysfunction. Pretreatment with SeNPs could alleviate the above-mentioned phenomenon (Figure 4A). As shown in Figure 4B, mtDNA copy number of the H2O2-model group decreased rapidly. However, mtDNA copy number in SeNPs pretreatment group was higher than that in H2O2-model group. Moreover, pretreatment with SeNPs effectively inhibited decrease of mitochondria number caused by H2O2 (Figure 4C and andDD).
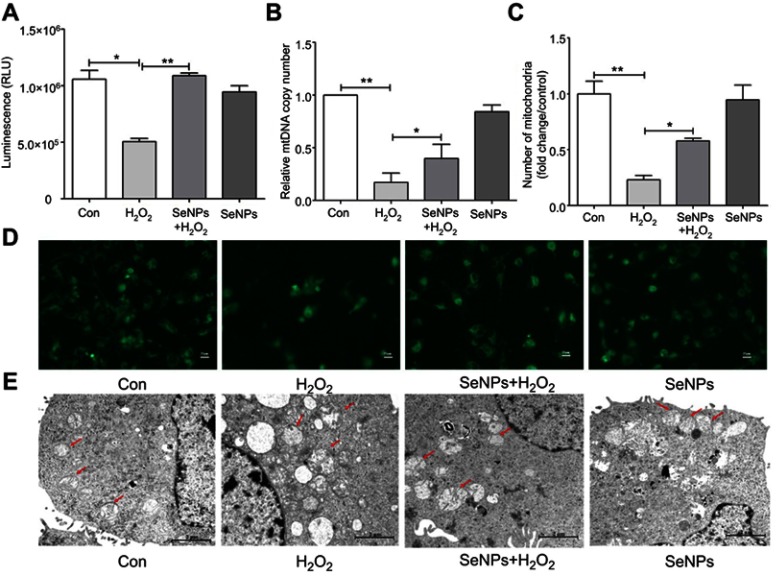
Protective effect of SeNPs on H2O2-induced mitochondrial damage of NCM460 cells. (A) The levels of ATP were measured by ATP assay Kit. (B) Mitochondrial DNA copy number was analyzed by real-time PCR. (C) Quantitative analysis of the cellular mitochondria (fold change/control). (D) Cellular mitochondria were observed by fluorescence microscope with Mito-Tracker Green staining. (E) Mitochondrial ultrastructure of NCM460 cells was observed by TEM. All data were presented as mean ± SEM of three separate experiments. *P<0.05, **P<0.01.
Abbreviations: Con, control; SeNPs, selenium nanoparticles; H2O2, hydrogen peroxide; ATP, adenosine triphosphate; TEM, transmission electron microscopy; SEM, standard error of mean.
Ultrastructure of mitochondria observed by TEM
Compared with the normal control, the ultrastructure of mitochondria in H2O2-induced oxidative damage model group exhibited apparent change: vacuoles were found; the swell was obvious; the mitochondrial cristae were vague; the density of matrix was low; minority of mitochondrial membrane was not intact. However, pretreatment with SeNPs alleviated H2O2-induced destruction of mitochondria. In control and SeNPs protective groups, the mitochondria had a column or mesh shape; the mitochondrial cristae were clear; the density of matrix was normal; the mitochondrial membrane was intact (Figure 4E).
SeNPs weakens the depolarization of MMP of intestinal epithelial cells
In order to explore how SeNPs protect mitochondria against H2O2-induced oxidative damage, firstly, levels of MMP in each experimental group were determined using JC-1. Compared with the normal control, MMP in H2O2-induced oxidative damage model group was significantly decreased. However, pretreatment with SeNPs significantly weakened the decrease of MMP caused by H2O2 (Figure 5A and andBB).

Effect of SeNPs on mitochondrial membrane potential (MMP) of NCM460 cells exposed to H2O2. (A) MMP was detected by an MMP assay Kit with JC-1 staining. MMP was visualized under the confocal microscope. (B) MMP was also measured by Multi-function Microplate Reader. All data were presented as mean ± SEM of three separate experiments. **P<0.01.
Abbreviations: Con, control; SeNPs, selenium nanoparticles; H2O2, hydrogen peroxide; SEM, standard error of mean.
Effect of SeNPs on Nrf2 activation and its downstream genes
In the current study, we investigated the relationship of the protective effect of SeNPs on intestinal barrier dysfunction caused by oxidative stress with Nrf2 signaling pathway due to the crucial role of Nfr2 in redox homeostasis. Results showed that SeNPs activated Nrf2 signaling and significantly improved HO-1 and NQO-1 protein levels compared with the H2O2 model group (Figure 6A and andBB).

Effect of SeNPs on Nrf2 activation and expression levels of its downstream genes. (A) The protein levels of Nrf2, NQO-1, and HO-1 were detected by Western blot analysis. (B) Quantitative analysis of the protein expression levels of Nrf2, NQO-1, and HO-1. (C) The viability of NCM460 cells treated with Nrf2 inhibitor (ML385) at 0.2, 0.5, 1.5, 2, 4, 6 or 8 µM. (D) Cells were treated with 4 µg/mL of SeNPs and/or 5 µM ML385 for 12 hours, followed by treatment with 500 μM H2O2 for 6 hours. The expression of Nrf2 was determined using Western blot analysis. (E) ML385 abolished the regulatory effect of SeNPs on ROS production. Cells were treated with 4 µg/mL of SeNPs and/or 5 µM ML385 for 12 hours, followed by treatment with 500 μM H2O2 for 6 hours. ROS production was visualized by fluorescence microscopy with DCFH-DA staining. All data were presented as mean ± S.E.M of three separate experiments. *P<0.05, **P<0.01.
Abbreviations: Con, control; SeNPs, selenium nanoparticles; H2O2, hydrogen peroxide; DCFH-DA, 2ʹ,7ʹ-dichlorodihydrofluorescein diacetate; SEM, standard error of mean.
Nrf2 inhibitor abolishes the antioxidant effects of SeNPs
Results showed that 5 μM ML385 as Nrf2 inhibitor did not affect cell viability (Figure 6C). However, the expression levels of Nrf2 were significantly downregulated in NCM460 cells treated with 5 μM ML385. Therefore, 5 μM was chosen as the concentration of ML385 interference experiment. SeNPs pretreatment activated Nrf2 signaling compared with the normal and oxidative stress groups (Figure 6D). Moreover, inhibition of Nrf2-signaling pathway abolished the regulatory effect of SeNPs on ROS overproduction caused by H2O2 (Figure 6E).
Discussion
Oxidative stress contributes to many pathological conditions and diseases.23 ROS, as highly reactive molecules, can damage cell structures and alter cell functions. Mitochondria are the key organelles for energy metabolism and ROS production.24 The plasticity of mitochondrial structure and function is a crucial feature for maintaining cellular homeostasis. Mitochondrial structure damage can affect mitochondrial function, and result in ROS accumulation and activation of inflammatory bodies, which may eventually lead to intestinal inflammation. IBD patients and experimental colitis mouse model exhibited mitochondrial stress and mitochondrial dysfunction in their intestinal epithelial cells.25 Previous studies indicated that increase of paracellular permeability was observed in dinitrophenol-induced uncouplers of oxidative phosphorylation of intestinal epithelial cell model, characterized by lower TER.26,27 mtDNA encodes respiratory chain complexes Ⅰ, Ⅲ, Ⅳ, and Ⅴ. In addition, mitochondria possess the ability to modulate crucial cellular functions including ROS production and stress signal transduction.28,29 In the current study, we found that H2O2-induced oxidative stress leaded to the drastic production of ROS, the depletion of ATP, and the reduction of MMP and mtDNA in normal human colon mucosal epithelial cells. These results indicated that H2O2 caused mitochondrial damage and dysfunction. Conversely, pretreatment with SeNPs alleviated these adverse effects. Moreover, SeNPs ameliorated H2O2-induced increase in FITC-dextran fluxes across NCM460 cells and H2O2-induced decrease of TER. These results indicate that SeNPs synthesized by L. casei ATCC 393 exhibit therapeutic potential against oxidative stress and preserve intestinal epithelial cell survival, mitochondrial function, and further maintain intestinal epithelial barrier integrity.
Antioxidant supplementation can effectively regulate the redox state of the body and maintain cellular homeostasis. Se is a much more potent antioxidant than vitamins E, C, and A.30 Se compounds protect mtDNA as well as telomere length and function.31 However, traditional Se supplements usually have relatively low bioavailability and high toxicity. Therefore, it is urgent to explore an innovative system for transforming carriers of Se.11 The effect of nanoscale Se on alleviating oxidative stress is well-known. Moreover, there is no significant medical side effect as a therapeutic agent.32,33 Therefore, Nano-Se has the potential to be applied in biomedical fields. The advantage of SeNPs in a zero oxidation state is that they possess low toxicity, high bioavailability and biocompatibility compared to other oxidation states.34 Various microorganisms such as Bacillus lichenifrrmis,35 Lactobacillus acidophillus,36 and Zooglea ramigera37 are able to convert toxic Se+IV and (or) Se+VI into nontoxic Se with the form of SeNPs. We have confirmed that probiotics L. casei ATCC 393 could synthesize SeNPs. Moreover, biogenic SeNPs by L. casei ATCC 393 possess strong antioxidant activity and low cytotoxicity compared with selenomethionine and sodium selenite, and can effectively protect human and porcine intestinal epithelial cells against H2O2- or Diquat-induced injury.20 Currently, biogenic SeNPs by L. casei ATCC 393 ameliorated H2O2-induced mithochondrial dysfunction in normal human colon mucosal epithelial cells. Nanoparticles usually pass through the intestinal epithelium in paracellular or transcellular pathway.38 Intestinal epithelial cells possess the ability to transfer mineral elements NPs. Endocytosis is an important start of transcellular transport. Internalization of NPs is a process of energy-dependence. The particle size of SeNPs is closely related to their biological activities.39 The absorption of NPs with a size of 100 nm in gastrointestinal tract is 15–250 times higher than that of large NPs.40 The size of biogenic SeNPs applied in the study was 50~80 nm.20
Exposure to acute or chronic oxidative stress can lead to cell damage, and impairs the normal physiological function of various organs, resulting in a variety of diseases. However, cells can protect themselves from serious damage by activating systems that scavenge free radicals to withstand oxidative stress. Nrf2-Keap1 is an important scavenger system-related signaling pathway that mediates a protective effect on cells challenged by oxidative stress.41,42 Nrf2 protects gastrointestinal cells from oxidative stress and subsequent inflammation through several mechanisms: 1) up-regulation of antioxidant and xenobiotic enzymes; 2) amelioration of inflammation by downregulation of NFκB; 3) stimulation of ABC transporters and MRP2.43 In homeostatic conditions, Nrf2 is not functionally activated, and exits in the form of Nrf2-Keap1 complex. However, when cells are subjected to oxidative stress, Keap1 is degraded from Nrf2-Keap1 complex, and then Nrf2 is released and transferred to the nucleus, and then combines with antioxidant response element, thereby regulating the expression of antioxidant-related genes and finally exerting its antioxidant defense function. Biogenic SeNPs protect cells against oxidative stress via activation of Nrf2 signaling, and up-regulation of antioxidant enzymes.44 Our results are consistent with the previously mentioned study. Up-regulation of Nrf2 and its downstream target genes was observed in cells treated with SeNPs under H2O2-induced oxidative stress. Those results demonstrated that Nrf2-mediated signaling pathway was closely related to cellular ROS production and the regulatory effect of biogenic SeNPs on intestinal epithelial barrier dysfunction caused by oxidative stress.
Conclusion
In summary, biogenic SeNPs synthesized by L. casei ATCC 393 exhibited considerably strong antioxidative activity to alleviate oxidative stress-caused intestinal epithelial barrier dysfunction. Moreover, as summarized in Figure 7, the potential mechanism of antioxidant properties may be associated with the regulatory effect of SeNPs on mitochondrial function via Nrf2-signaling pathway. These results suggest that SeNPs synthesized by probiotics are alternative supplements of Se for prevention and treatment of oxidative stress-related intestinal disorders. Moreover, the present study enlightens us that mitochondria-targeting SeNPs may be applied to regulate intestinal barrier dysfunction so as to avoid intestinal diseases in humans challenged by oxidative stress.
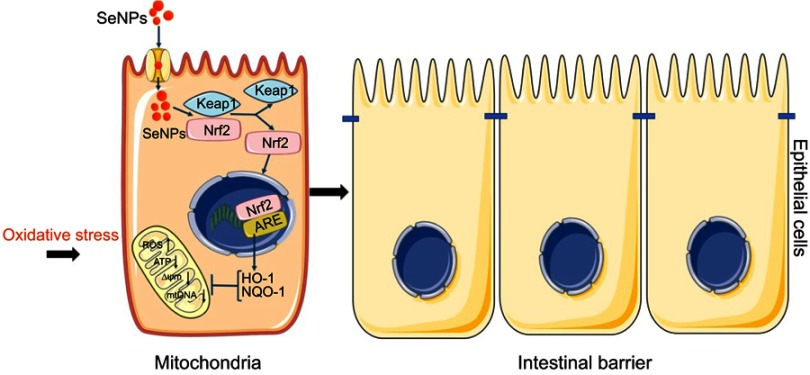
Schematic representation of the proposed mechanism by which biogenic SeNPs synthesized by Lactobacillus casei ATCC 393 protect intestinal epithelial barrier function against oxidative damage. After SeNPs are transported into intestinal epithelial cells, Nrf2 is separated from the Keap1-Nrf2 complex and moved into the nucleus, where it binds to ARE. Transcriptional activation of antioxidant enzymes HO-1 and NQO-1 occurs with the activation of ARE, which function together to inhibit ROS production, weaken the depolarization of MMP, and enhance mitochondrial DNA copy number. In summary, SeNPs exhibit the protective effect on intestinal epithelial barrier function against oxidative damage by alleviating ROS-mediated mitochondrial dysfunction via Nrf2 signaling pathway under oxidative stress.
Abbreviations: SeNPs, selenium nanoparticles; ARE, antioxidant response element; MMP, mitochondrial membrane potential.
Acknowledgments
This work was supported by the National Natural Science Foundation of China (no 31672435;), Seed Foundation of Innovation and Creation for Graduate Students in Northwestern Polytechnical University (no ZZ2018046;), and the Key Research and Development Program of Shaanxi Province (no 2018NY-102).
Author contributions
All authors contributed to data analysis, drafting or revising the article, gave final approval of the version to be published, and agree to be accountable for all aspects of the work.
Disclosure
The authors report no conflicts of interest in this work.
References
Articles from International Journal of Nanomedicine are provided here courtesy of Dove Press
Full text links
Read article at publisher's site: https://doi.org/10.2147/ijn.s199193
Read article for free, from open access legal sources, via Unpaywall:
https://www.dovepress.com/getfile.php?fileID=50575
Citations & impact
Impact metrics
Citations of article over time
Smart citations by scite.ai
Explore citation contexts and check if this article has been
supported or disputed.
https://scite.ai/reports/10.2147/ijn.s199193
Article citations
Serine Rejuvenated Degenerated Volvariella volvacea by Enhancing ROS Scavenging Ability and Mitochondrial Function.
J Fungi (Basel), 10(8):540, 01 Aug 2024
Cited by: 0 articles | PMID: 39194866 | PMCID: PMC11355192
Selenium-Enriched E. coli Bacteria Mitigate the Age-Associated Degeneration of Cholinergic Neurons in C. elegans.
Antioxidants (Basel), 13(4):492, 20 Apr 2024
Cited by: 0 articles | PMID: 38671939 | PMCID: PMC11047679
Recent advances in the therapeutic applications of selenium nanoparticles.
Mol Biol Rep, 51(1):688, 25 May 2024
Cited by: 2 articles | PMID: 38796570 | PMCID: PMC11127871
Review Free full text in Europe PMC
Turmeric Extract-loaded Selenium Nanoparticles Counter Doxorubicin-induced Hepatotoxicity in Mice via Repressing Oxidative Stress, Inflammatory Cytokines, and Cell Apoptosis.
Anticancer Agents Med Chem, 24(6):443-453, 01 Jan 2024
Cited by: 0 articles | PMID: 38204261
The influence of microbiota on ferroptosis in intestinal diseases.
Gut Microbes, 15(2):2263210, 05 Oct 2023
Cited by: 9 articles | PMID: 37795964 | PMCID: PMC10557621
Review Free full text in Europe PMC
Go to all (23) article citations
Similar Articles
To arrive at the top five similar articles we use a word-weighted algorithm to compare words from the Title and Abstract of each citation.
Biogenic selenium nanoparticles by Lactobacillus casei ATCC 393 alleviate the intestinal permeability, mitochondrial dysfunction and mitophagy induced by oxidative stress.
Food Funct, 12(15):7068-7080, 01 Aug 2021
Cited by: 12 articles | PMID: 34156041
Biogenic selenium nanoparticles synthesized by Lactobacillus casei ATCC 393 alleviate diquat-induced intestinal barrier dysfunction in C57BL/6 mice through their antioxidant activity.
Food Funct, 11(4):3020-3031, 01 Apr 2020
Cited by: 26 articles | PMID: 32243488
Preparation, characteristics and antioxidant activity of polysaccharides and proteins-capped selenium nanoparticles synthesized by Lactobacillus casei ATCC 393.
Carbohydr Polym, 195:576-585, 30 Apr 2018
Cited by: 62 articles | PMID: 29805014
Effects of selenium nanoparticles produced by Lactobacillus acidophilus HN23 on lipid deposition in WRL68 cells.
Bioorg Chem, 145:107165, 07 Feb 2024
Cited by: 0 articles | PMID: 38367427
Review