Abstract
Free full text

GDNF-expressing Macrophages Restore Motor Functions at a Severe Late-stage, and Produce Long-Term Neuroprotective Effects at an Early-stage of Parkinson’s Disease in Transgenic Parkin Q311X(A) Mice
Abstract
There is an unmet medical need in the area of Parkinson’s disease (PD) to develop novel therapeutic approaches that can stop and reverse the underlying mechanisms responsible for the neuronal death. We previously demonstrated that systemically administered autologous macrophages transfected ex vivo to produce glial cell line-derived neurotrophic factor (GDNF) readily migrate to the mouse brain with acute toxin-induced neuroinflammation and ameliorate neurodegeneration in PD mouse models. We hypothesized that the high level of cytokines due to inflammatory process attracted GDNF-expressing macrophages and ensured targeted drug delivery to the PD brain. Herein, we validated a therapeutic potential of GDNF-transfected macrophages in a transgenic Parkin Q311X(A) mice with slow progression and mild brain inflammation. Systemic administration of GDNF-macrophages at a severe late stage of the disease leaded to a near complete restoration of motor functions in Parkin Q311X(A) mice and improved brain tissue integrity with healthy neuronal morphology. Furthermore, intravenous injections of GDNF-macrophages at an early stage of disease resulted in potent sustained therapeutic effects in PD mice for more than a year after the treatment. Importantly, multiple lines of evidence for therapeutic efficacy were observed including: diminished neuroinflammation and α-synuclein aggregation, increased survival of dopaminergic neurons, and improved locomotor functions. In summary, GDNF-transfected macrophages represent a promising therapeutic strategy for PD at both late- and early-stages of the disease.
Graphical Abstract.
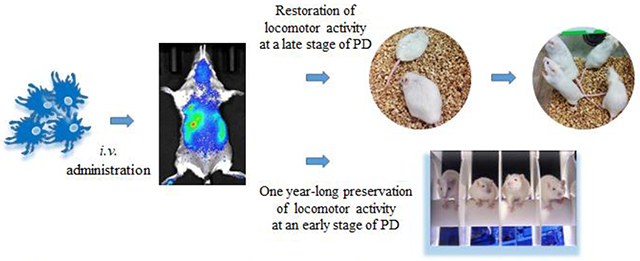
Autologous macrophages were transfected ex vivo to produce glial cell line-derived neurotrophic factor (GDNF) and intravenously injected into Parkin Q311(X)A mice. GDNF-expressing macrophages readily reach brain in PD mice; restore motor functions in transgenic mice at a late stage of PD, and produce one year-long therapeutic effects at an early stage of PD.
1. Introduction
Parkinson’s disease (PD) is one of the fastest growing neurological disorders in the developed world that affects millions of people over 60 years of age [1]. The motor symptoms of PD result from the progressive loss of midbrain dopaminergic neurons that cause a decline in the levels of dopamine (DA) in the central nervous system (CNS), leading to severely impaired motor function. The existing therapeutic regimens provide only symptomatic relief to the patients and the treatments regimens are hindered by the development of drug resistance and progressive adverse side effects such as motor complications and dyskinesia [2]. For instance, in the case of L-DOPA, the current “gold standard” for PD treatment, patients typically respond well for several years, but then become unresponsive to therapy [3]. In addition, due to fast clearance, L-DOPA should be taken several times a day to sustain necessary DA levels in the brain.
A growing body of evidence indicates the usefulness and efficacy of neurotrophic factors for the treatment of PD [4–17]. Glial cell line-derived neurotrophic factor (GDNF) is a founding member of the GDNF family of ligands (GFL) and has been shown to interact with GFRA2 and GDNF family receptor alpha 1. Human GDNF (monomer) contains 135 aminoacidss residues, which is a disulfide-linked homodimer, and it shares 99% and 93% a.a. sequence identity with mouse and rat GDNF, respectively [18]. It is a small protein that was first identified as a potential therapeutic agent for PD based on its ability to promote the survival of embryonic DA neurons in vitro [4]. Later, intracranial infusions of GDNF were shown to provide protection and restoration of DA neurons in rodent [5–7] and primate [12, 14–17] models of PD. Furthermore, it was reported that bone marrow-derived stem cells, which were transduced ex vivo with lentivirus expressing GDNF gene and then transplanted into recipient mice dramatically ameliorated PD-related inflammation and neuronal degeneration resulting in axon regeneration and reversed hypoactivity in mice [19]. In addition, gene delivery systems for GDNF using adeno-associated viral vectors were suggested for the advanced PD patients (Clinical Trials ID: ) [20]. Finally, in a recent clinical trial, GDNF treatment was shown to produce promising therapeutic effects on damaged brain cells in PD patients. Forty-one participants underwent robot-assisted surgery to have four implanted tubes, which allowed GDNF to be infused directly to the affected brain areas with pinpoint accuracy [21]. Unfortunately, these brain infusions carry a high risk of adverse effects, and have poor patient adherence.
Herein, we propose to use genetically modified inflammatory response cells, for the delivery of therapeutics to the brain to treat PD. The chronic inflammation that is present in the PD brain [22] provides a rationale for the targeted drug delivery using such cells as monocytes and macrophages. This approach could offer several advantages over standard drug administration regimens, including targeted transport to local areas of disease where inflammatory processes are active, and sustained release of the therapeutic payload at the disease site. In this work, we evaluated whether the genetically modified autologous macrophages could reach the disease site and elicit therapeutic effects in transgenic mice with mild inflammation. We used Parkin Q311X(A) mice that represents Turkish early-onset PD directed to DA neurons of the Substantia Nigra pars compacta (SNpc) and the ventral tegmentum area (VTA) [23]. These mice have a truncated mutant parkin gene, whose expression is selectively driven in DA neurons [24]. This widely used animal model exhibits several key features of the disease in humans, such as a slow progressive course of phenotypic manifestations and neurodegeneration, adult-onset degeneration of nigrostriatal dopamine circuitry, and motor deficits.
We report here the development of new drug delivery systems for systemic administration neurotrophic factors using autologous macrophages. The specific advances to the field contributed by this body of work can be outlined in three main points. First, we demonstrated that intravenous injections of GDNF-macrophages were able to not only prevent and slow down neurodegeneration but also reverse the course of the disease in Parkin Q311X(A) mice with advanced stages and restore the already impaired locomotor functions to the levels observed in wild type animals. Second, the obtained data regarding biodistribution of autologous macrophages indicated that even mild brain inflammation manifested in transgenic mouse PD model attracted the cell-carriers to the brain and ensured targeted GDNF delivery in Parkin Q311X(A) mice. Finally, prolonged sustained therapeutic effects were recorded in PD mice with early stages of the disease. Thus, ameliorated neuronal degeneration in SNpc, and improved the locomotor functions were recorded for nearly a year after the treatment.
2. Materials and Methods
2.1. Plasmids and Reagents
The gWIZ™ high expression vectors encoding the reporter gene luciferase (Luc) (gWIZ™Luc) under control of an optimized human cytomegalovirus (CMV) promoter followed by intron A from the CMV immediate-early (IE) gene were used throughout the study (Gene Therapy Systems, San Diego, CA). Human GDNF cDNA (NM_199234, Supplementary Figure S1 A) was provided by OriGene (Rockville, MD). The sequence for GDNF-encoding plasmid DNA (pDNA) can be found on web page: http://www.origene.com/human_cdna/NM_199234/SC307906/GDNF.aspx. The GDNF-encoding plasmid under CMV promoter was expanded in DH5α E.coli and isolated using Qiagen endotoxin-free plasmid Giga-prep kits (Qiagen, Valencia, CA) according to the supplier’s protocol. The manufactured GDNF pDNA was then characterized by digestion with a single cut (AlwNI), and a double cut (AlwNI & PflMI) and run in 1% DNA electrophoresis gel (100V, 60 min) as shown on Supplementary Figure S1 B. The encoded human GDNF is a secreted protein with the leading sequence of the first five N-terminal amino acids: Met-Ser-Pro-Asp-Lys. jPEI-macrophage reagent (jPEI) for transfection of primary macrophages was purchased from Polyplus Transfection Co. (New York, NY, USA). A lipophilic fluorescent dye, 1,1′-dioctadecyl-3,3,3′,3′-tetramethylindo-carbocyanine iodide (DIR), was purchased from Thermo Fisher Scientific (Waltham, MA, USA). Murine macrophage colony-stimulating factor (MCSF) was purchased from Peprotech Inc (Rocky Hill, NJ).
2.2. Cells
Bone marrow-derived cells extracted from murine femurs (wild type littermates of Parkin Q311X(A) female mice, 2 mo. old) were seeded in T75 flask, and cultured for 10 days in the media supplemented with 1000 U/ml macrophage colony-stimulating factor (MCSF) to obtain primary bone-marrow macrophages (BMM) [25]. A few non-differentiated cells that do not attach to the flask was removed by washing. The purity of macrophages culture was determined by flow cytometry using FACSCalibur (BD Biosciences, San Jose, CA), and 95% of cells were found to be CD11b+.
2.3. Animals
Two breeding pairs of Parkin Q311X(A) mice were purchased from the Jackson Laboratory (Bar Harbor, ME, USA) twelve weeks of age, and were treated in accordance to the Principles of Animal Care outlined by National Institutes of Health and approved by the Institutional Animal Care and Use Committee of the University of North Carolina at Chapel Hill. Several cohorts of transgenic mice as well as their wild type controls were bred in house. The genotyping of pups was carried by PCR analysis of Parkin Q311X(A) gene was performed for parents, a negative control wild mouse, and several mice generations according to manufacturers protocol (Supplementary Figure S2). Mice were housed in a temperature and humidity controlled facility on a 12 h light/dark cycle and food and water were provided ad libitum.
2.4. Transfection of Macrophages
BMM were incubated with a mixture of Luc- or GFP-, or GDNF-encoding pDNA, and jPEI-Mac or GenePORTER 3000 (GP3K) reagents in serum free media for four hours. Specifically, 14 μg pDNA was added to 2,040 μL diluent, and combined with 762 μL serum-free media and 190 μL jPEI-Mac or GP3K. The obtained mixture was added to the cells grown on T75 flask (approximately 20 x106 cells/flask). Following incubation, an equal volume of full media containing 20% FBS was added bringing final serum concentration to 10%. Additionally, macrophages were transfected by electroporation at various conditions. Briefly, 30x106 BMM were spanned down at 1000 RPM for 5 min, and then re-suspended in 300 μL electroporation buffer (Neon Transfection system, Thermo Fisher Scientific) and supplemented with 30 μg Luc-pDNA. The aliquots of cell suspension with Luc-pDNA or GDNF-pDNA (10 μL) were placed into electroporation cell, and electroporated at various conditions according to manufacturer’s protocol. The electroporation conditions were as follows: #1 (voltage 0; width 1; pulses 1); #2 (voltage 1300; width 20; pulses 2); #3 (voltage 1700; width 20; pulses 2); #4 (voltage 1400; width 20; Pulses 2). Then, the cells were supplemented with 500 μL antibiotic-free media and cultured for various time points. The luciferase activity or percentage of transfected macrophages, or GDNF levels in the cells and cell media were assessed by luminescence or FACS, or ELISA, respectively. For measures of the overexpressed GDNF release, culture media from transfected macrophages was collected at various time points, and assessed for the levels of GDNF by ELISA. The number of GFP-transfected macrophages and the expression levels in relative fluorescent units (RFU) were assessed by FACS as described earlier [26].
2.5. Confocal Microscopy
Studies were carried out to determine expression of the reporter gene (GFP) in transfected macrophages as described earlier [26]. GFP expression in primary macrophages was visualized by a confocal fluorescence microscopic system ACAS-570 (Meridian Instruments, Okimos, MI, USA) with argon ion laser (excitation wavelength, 488 nm) and corresponding filter set. Digital images were obtained using the CCD camera (Photometries) and Adobe Photoshop software. To visualize effects of GDNF-transfected macrophages on neuronal differentiation, PC12 neuronal cells were transduced with lentiviral vector encoding mCherry as well as a puromycin resistance gene. Then, macrophages were transfected with GDNF-encoding pDNA as described above, the media from GDNF-macrophages was collected and added to non-differentiated mChery-PC 12 cells for 72 h. mCherry-PC12 cells incubated with serum-free media or cell media from sham-transfected macrophages (i.e. cells transfected with GFP-encoding pDNA) were used as controls.
2.6. Immunohistochemical and Stereological Analyses
For the assessment of neuroprotective effects, tyrosine hydroxylase staining was used to quantitate numbers of dopaminergic neurons [27]. For the detection of microglial activation, tissue sections were incubated with primary monoclonal rat anti mouse Mac1 antibodies (AbD Serotec, Raleigh, NC, USA), and secondary biotinylated goat anti-rat antibodies (Vector Laboratories, Burlingame, CA, USA). For the assessment of the formation of proteinase K-resistant α-synuclein, the brain tissue slices were incubated with 0.3% Triton X-100 in PBS for 20 min, washed and incubated with 10ug/mL proteinase K in PBS for 10 min to clear tissues from non-aggregated α-synuclein. Then, slides were incubated with primary antibody anti-α-synyclein (Abcam ab131508, 1:100 dilution) for 48 h at 4°C, washed with 3x PBS, and secondary antibody goat anti-rabbit IgG H+L (Alexa Flour 488, Invitrogen A-11008) for 1 h at RT in the dark. Levels of astrocyte activation were determined in the ventral midbrain region by fluorescent analysis of GFAP expression with primary antibody anti-GFAP (Abcam ab7260, 1:500 dilution), and secondary antibody goat anti-rabbit IgG H+L (Alexa Flour 488, Invitrogen A-11008) for 1 h at RT in the dark. The total number of TH-positive SN neurons, MAC1-positive microglial cells were counted using the optical fractionator module in StereoInvestigator software (MicroBrightField, Inc., Williston, VT, USA) [28]. Quantification of the fluorescence levels of α-synuclein and astrocytes was performed as the function of the positive area by ImageJ software for mean Fluorescent Intensity (MFI) and % Area of fluorescence (free access provided by National Institute of Health). Briefly, Region of Interest (ROI) was selected on each slide and fluorescence intensity was measured by the Image J measuring tool. Background was calculated by measuring mean fluorescence level of an area with no cells, and subtracted from Raw Image data (N = 15).
Hematoxylin and eosin (H&E) staining:
Brain tissues were cut into sections of 10 micron thickness and stained with H&E. Briefly, sections were rewarmed at room temperature for 30 minutes, then exposed to xylene and re-hydrated with 100% ethanol, 95 and 70% ethanol, followed by staining with hematoxylin dye for 15 min, prior to washing with distilled water. Subsequently, tissue sections were stained with eosin for 20 sec, dehydrated with gradient ethanol after washing with tap water, and tissues were then cleared by xylene and mounted using mounting media for visualization. Images were analyzed using a Zeiss (Germany) inverted fluorescence microscope with a 560 Axiovision camera at 5x, 20x and 40x.
Nissl staining:
Brain sections of 10-micron thickness were stained with cresyl violet acetate solution (Nissl). Briefly, sections were rewarmed at room temperature for 30 minutes, then exposed to xylene and immersed in 100% ethanol, 95%, 75% ethanol and distilled water, and then stained with a cresyl violet solution for 20 minutes. Sections were washed in distilled water, immersed in 75% ethanol, 95% and 100% ethanol. Tissues were then cleared by xylene and mounted using mounting media for visualization. Images were analyzed using a Zeiss (Germany) inverted fluorescence microscope with a 560 Axiovision camera at 5x, 20x and 40x.
2.7. Bioimaging and Infrared Spectroscopy (IVIS)
To track systemically injected cell-carriers in the PD mice, macrophages were labeled using three different methods. First, primary macrophages were labeled with DIR lipophilic dye (Invitrogen, Carlsbad, CA, USA) according to manufacturer’s protocol. Briefly, BMM (1 x 106 cell/mL) were incubated with 7 μM DIR in PBS for 20 min at 37C. Followed incubation, the cells were spin down and washed 3x with ice-cold PBS, and re-suspended in saline. Finally, primary macrophages were labeled with Celsense (Celsense, Inc., Pittsburg, PA, USA) that is a fluorocarbon-based emulsion engineered to safely label cells ex vivo without use of transfection regents. Briefly, macrophages (4 x 106 cell/mL) were incubated with Celsence in complete media (1.5 mg/ml) for 18 h, and rinsed 3x times with ice-cold PBS. In addition, autologous macrophages were transfected with Luc-encoding pDNA using GP3K transfection reagent as described earlier [29]. In a parallel experiment, Parkin Q311X(A) mice (4 mo. of age) and their wild type littermates were injected with DIR-labeled BMM, or Celsense-labeled BMM, or Luc-expressing macrophages (2 x 106 cell/100 μL/mouse) via the intratail vein (i.v.), and animals were imaged by Xenogen IVIS Optical Imaging System. At the end point, animals were sacrificed and perfused as described earlier [30], main organs were removed, washed, post-fixed in 10% phosphate-buffered paraformaldehyde, and evaluated by IVIS Aura software (Spectral Instrument Imaging, Tucson, AZ, USA).
2.8. Behavioral Tests
For the traditional constant speed Rotarod test, mice were trained and tested as previously described with slight modifications [31]. Parkin Q311X(A) mice (4 mo. old) were i.v. injected with autologous GDNF-macrophages transfected with GDNF-encoding pDNA, or sham macrophages, or saline (once a week, 3x weeks, 2x106 cells/100μL/mouse). WT mice injected with saline were used as a control. GDNF-macrophages were obtained using transfection agent as described above and cultured 24 h prior to the administration. Animals were treated once a week for three weeks at 4 mo. (early stage) or 16 mo. (late stage of the disease). Then, the animals were subjected to standard behavioral tests; Wire hanging test, and accelerating Rotarod procedure, and Escaping activity test were performed as described in [29] before the treatment and later on.
2.9. Statistical Analysis
For the all experiments, data are presented as the mean ± SEM. For analysis of statistical significance of therapeutic effects assessed by behavioral tests and immuniohistochemistry, the difference between scores at baseline and endpoint were compared by group (PD mice injected with GDNF-macrophages, sham macrophages, or saline, as well as WT mice injected with saline). For all three tests the baseline was mo. 4. The immunohistochemical and stereological outcomes were also compared by the same four groups using the values at 16 mo, when the mice were sacrificed. A one-way ANOVA was used to see if there were any significant differences between groups. If the differences between the groups were significant at the 0.05 level after a using the Holm method for to control the family wise error rate for the nine different outcomes that were tested using a one-way ANOVA. Then pairwise tests were conducted using a Bonferroni correction. Because pairwise comparisons for each outcome were only done if the one-way ANOVA was found to be significant, multiple comparisons for the pairwise testing only controlled for the number of pairwise comparisons for the given outcome, not the number of possible pairwise comparisons for all outcomes.
3. Results
3.1. Transfection of Primary Macrophages with pDNA Encoding Reporter and Therapeutic Genes
As part of our strategy, efficient transfection of primary macrophages with pDNA encoding reporter or therapeutic genes is crucial. We acquired two different methods for transfection of primary autologous macrophages including macrophage transfection agent (jPEI) (Figure 1), and the electroporation method (Supplementary Figure S3). Successful transfection of primary bone marrow-derived macrophages (BMM) was accomplished with pDNA encoding green fluorescence protein (GFP) (Figure 1 A, ,B),B), luciferase (Luc) (Figure 1 C), and GDNF (Figure 1 D). The optimal conditions for experiments utilized earlier [26] provided high levels and duration of the encoded proteins expression. For example, up to 30% cells expressed the reporter protein GFP at day 3 (Figure 1 A). Of note, as GDNF is a secreted molecule, a significant amount of GDNF was detected in the transfected cells, as well as in cell culture media (Figure 1D), but not in macrophages transfected with sham vector (dashed line). Successful transfection with GFP-encoding pDNA was also confirmed by confocal microscopy at day 3 (Figure 1 B).

Transfections of macrophages with GFP- (A, B), or luciferase- (C), or GDNF-encoding pDNA (D) were carried out using jPEI-Mac reagent as described in Experimental section. Cells were washed and cultured in complete media for up to 7 days. The percentage of GFP-transfected macrophages (A), or luciferase activity at day 3 (C), or GDNF levels in cells (black bars) or conditioned media (white bars) (D) were accessed by FACS (A), or luminescence (C), or ELISA (D), and visualized by confocal microscopy at day 3 (B). The bar: 50 μm. *p < 0.05, compared to sham-transfected macrophages (dashed line).
Next, efficient transfection of BMM was also accomplished using electroporation (Supplementary Figure S3). Different electroporation conditions #1 - #4 that express different voltage and pulse (described in Experimental Section) were utilized to optimize the transfection efficiency with the reporter pDNA (Supplementary Figure S3 A). The peak level of GDNF in primary macrophages transfected by electroporation was at day 1 (Supplementary Figure S3 B), although the GDNF levels in the cell media were still high at days 4 and 7 with the optimal condition # 4. Confocal images at day 3 confirmed expression of GFP in transfected cells (Supplementary Figure S3 C).
Finally, a profound effect of GDNF released from pre-transfected macrophages in vitro was demonstrated in PC12 neuronal cells that are known to express GDNF receptors. To visualize cells by confocal microscopy, PC12 neuronal cells transduced with a lentiviral vector encoding mCherry (red) were utilized. Macrophages were transfected with GDNF-encoding pDNA with the transfection agent as described above, and conditioned media was collected after 72 h. Non-differentiated mCherry-PC12 cells were supplemented with media from GDNF-transfected or control sham-transfected macrophages, the cells were cultured for 72 h, and visualized by confocal microscopy (Supplementary Figure S4). Confocal images revealed a pronounced outgrowth of axons and dendrites in neuronal PC12 cells cultured with conditioned media from GDNF-macrophages (Supplementary Figure S4 B) compared to control non-differentiated cells cultured in serum-free media (Supplementary Figure S4 A). No effect on axonal growth was observed in neuronal cells supplemented with media from sham-transfected macrophages (Supplementary Figure S4 C).
3.2. Systemically Injected Macrophages Migrate to Inflamed Brain Tissues in Parkin Q311X(A) Mice
To prove the ability of macrophages to target inflamed brain tissues, the cell-carriers were labeled using three different methods: (i) labeling of lipids of macrophages with hydrophobic DIR dye, or (ii) loading of macrophages with fluorocarbon formulation Celsense, or (iii) transfection of macrophages with Luc-encoding pDNA to express Luc. First, kinetics of the appearance of systemically injected macrophages in PD mouse brain at early stage of disease (4 mo. of age) and accumulation in peripheral organs was examined with DIR labeled BMM (6x106 cells/100 μL/mouse, N = 4) (Figure 2 A, ,D).D). Of note, no aggregation of the cell-carriers in the treatment solution was recorded. The same age-matched wild type (WT) mice were used as controls (Figure 2 B, ,E).E). Considerable DIR fluorescence was detected in the PD brain as early as 4 h after i.v. administration of DIR-macrophages, and leveled off after 24 h (Figure 2 A, ,C).C). In contrast, significantly lower levels of macrophages were registered in the brains of healthy WT animals (Figure 2 B, ,C).C). The quantification of levels of fluorescence in the brain of living animals (Figure 2 C), as well as the images of postmortem main organs collected from perfused mice at the end point (Figure 2 D, ,E)E) confirmed this observation. We suggest that this effect is due to the ability of macrophages home to regions of inflammation and neurodegeneration, traveling via the gradient of inflammatory signals, chemokines and cytokines. Of note, the perfusion procedure allowed assessment of macrophages in the organ tissues avoiding possible contamination by these cell-carriers in the blood stream. Furthermore, low fluorescent signals at the first hour on dorsal images might indicate that most DIR-macrophages were circulated in the blood stream and accumulated in main excretion organs, liver, lungs, and kidney, as seen on Supplementary Figure S5.

Parkin Q311(X)A mice (4 mo. of age) were i.v. injected with 6x106 DIR-macrophages (A), or Celsense-macrophages (F), or Luc-transfected macrophages (H) and imaged by IVIS. WT mice were used as healthy controls (B, E, H). At the end point (72 h) mice were sacrificed, perfused, main organs were removed, and images by IVIS for PD mice (D, G), and WT mice (E). Fluorescence intensity in the brain area on IVIS images (A, B) was quantified and plotted vs. time (C) for PD mice (black circles), and WT mice (white circles). Systemically-administered macrophages reached the brain in PD mice at significantly greater levels than in WT mice (C). Imaging living animals injected with Luc-macrophages (H) and isolated organs (i.e. liver (1), lungs (2), spleen (3), kidney (4), and brain (5) of mice injected with Celsense-macrophages (F), as well as quantification of Celsense-macrophages levels in these organs (G) confirmed this result. Significant amount of macrophages reach PD mouse brain (G, red bar). Values are means ± SEM (N = 4 for all experiments), *p < 0.05 compared to WT mice.
Next, the accumulation of macrophages in PD mouse brain at 6 h after the i.v. injection was confirmed with macrophages labeled with Celsense, a dye that enable real-time tracing of cell-based therapeutics in animals (Figure 2 F, ,G).G). The results showed relatively high accumulation of cell-carriers in the lungs, liver, spleen, with lower but detectable levels in the kidney and brain. Notably, macrophages accumulated significant amount of Celsense with almost no toxicity observed at these conditions (Supplementary Figure S6).
Finally, macrophages were transfected with pDNA encoding luciferase (Luc) using transfection reagent as described above. This approach allowed tracing macrophages expressing the encoded protein Luc. PD mice were systemically injected with Luc-macrophages and examined by the IVIS imaging system 4 h after the injection (Figure 2 H). The images revealed a considerable amount of luminescence in the inflamed brain of transgenic PD mice, but not in brains of the healthy WT animals. Of note, along with the greater brain uptake, there was markedly greater systemic uptake of Luc-macrophages in PD mice compared to WT animals (Figure 2 H). This consistent with our earlier reports regarding PD mice with acute brain inflammation induced by i.c. injections of 6-OHDA [26], or LPS [30], suggesting that systemically administered macrophages migrate to sites of inflammation and accumulate in the brain of PD mice. Overall, the results from all three experiments corroborated with each other and collectively suggested that a significant amount of systemically administered autologous macrophages were able to reach the brain in Parkin Q311X(A) mice.
3.3. GDNF-macrophages Restore Motor Functions in Parkin Q311X(A) Mice with Severe Late-stage of PD
Massive striatal DA deficiency causes detectable locomotor deficits in Parkin Q311X(A) transgenic mice. These deficits can be used to assess therapeutic effect of GDNF-macrophages. First, Parkin Q311X(A) transgenic mice at severe late stage of the disease (16 mo. of age) were systemically injected with GDNF-macrophages, or sham macrophages, or saline. WT mice injected with saline were used as a control. Animals were treated once a week for three weeks. To assess changes in the animal motor functions over time, all groups were subjected to a battery of behavioral tests, including: Wire hanging test, and Rotarod test. The null point was established for all groups at 16 mo. immediately before treatment onset.
Systemic administration of GDNF-macrophages at this late severe stage of the disease improved locomotor activity of PD mice (Supplementary Video S1) compared to those injected with saline (Supplementary Video S2). The results of Wire hanging test (Figure 3 A) and Rotarod test (Figure 3 B) revealed significant (Supplementary Tables S1 and S2) increases in remaining time for PD mice treated with GDNF-macrophages up to the levels of healthy WT littermates.
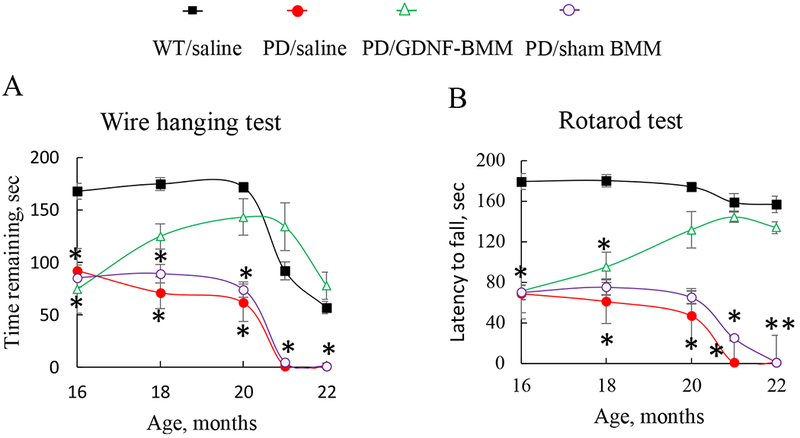
Transgenic mice (16 mo. old) were i.v. injected with GDNF-transfected macrophages (2x106 cells/100μL/mouse, once a week, 3x weeks, green), or saline (red), or sham macrophages (purple) once a week, 3x weeks. Wild type mice were systemically injected with saline (black). Wire hanging test (A), and Rotarod test (B) demonstrated significant improvements in motor functions upon treatment with GDNF-macrophages. Values are means ± SEM (N = 6), *p < 0.05 compared to WT healthy mice. For detailed statistical comparisons, see Supplementary Tables S1 – S2.
3.4. Long-term Sustained Effects of GDNF-macrophages on Motor Functions in Parkin Q311X(A) Mice Treated at Early-stage of PD
All behavioral tests, including Wire Hanging test, Rotarod test, and Escaping Activity test demonstrated statistically significant preservation of locomotor functions in PD mice treated with GDNF-macrophages (Figure 4). Specifically, systemic administration of GDNF-macrophages significantly increased remaining time in Wire hanging and Rotarod tests (Figure 4 A and andB,B, respectively), and reduced escaping time (Figure 4 C) to the levels observed in healthy WT animals. In particular, using one-way ANOVA test gave a p-value of 8.87 × 10−3, indicating that there is a difference between the groups in average time for the Wire hanging test (Supplementary Table S3). The Bonferroni corrected p-value for the pairwise t-test for each pair of groups indicated that the Parkin Q311X(A) mice treated with GDNF-macrophages have significantly higher retention times than those treated with sham-transfected macrophages, or treated with saline (Supplementary Table S4). This signifies that systemic administration of GDNF-macrophages resulted in the preservation of locomotor activity in PD mice. Similar to Wire hanging test scores, the statistical analysis for the Rotarod test by one-way ANOVA gave a p-value of 3.62 x 10−5, suggesting that there was a significant improvement in the remaining time on rotarod for PD mice treated with GDNF-macrophages (Supplementary Tables S5 and S6). Lastly, the escaping activity test also showed qualitative improvement in the escaping time for PD mice treated with GDNF-macrophages, although the differences were not statistically significant (Supplementary Table S7).
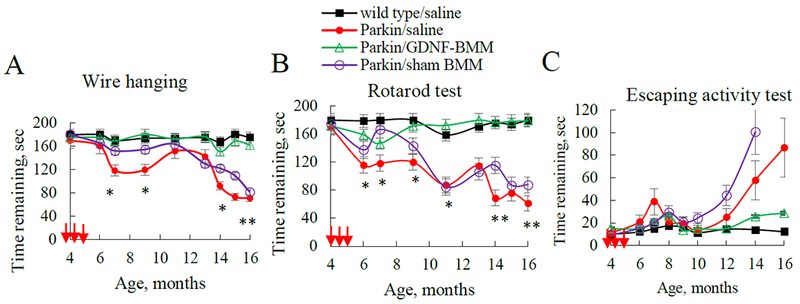
Transgenic mice (4 mo. old) were i.v. injected with GDNF-transfected (green), sham-transfected macrophages (purple), or saline (red) (once a week x 3 weeks, 2x106 cells/100μL/mouse, red arrows). WT mice were i.v. injected with saline (black). Wire hanging test (A), Rotarod test (B), and Escaping activity test (C) demonstrated significant improvements in motor functions upon treatment with GDNF-macrophages. Values are means ± SEM (N = 6), *p < 0.05, **p < 0.005 compared to WT healthy mice. For detailed statistical comparisons, see Supplementary Tables S3–S7.
3.5. Neuroprotective Effect of GDNF-macrophages in Parkin Q311X(A) Mice
At the endpoint of the experiment described above, 16 mo. old animals were sacrificed; postmortem brains were harvested and sectioned using a microtome. The mid-brain slides were stained for the expression of tyrosine hydroxylase (TH), a marker for DA neurons (Figure 5 A–D). The quantitative assessment of the number of DA neurons is shown on Figure 5 E. The brain slides of PD mice showed complete degeneration of DA neurons in the SNpc (Figure 5 A, ,E)E) compared to healthy WT mice (Figure 5 D, ,E).E). In contrast, systemic administration of GDNF-macrophages dramatically ameliorated PD-related neurodegeneration (Figure 5 B, ,E)E) compared to the PD animals treated with saline (Figure 5 A, ,E).E). The p-value for the one-way ANOVA was 0.02, which is significant at the 0.05 level indicating at least one difference between the groups in the number of neurons (Supplementary Table S8). Of note, no statistically significant difference was found between PD mice treated with GDNF-macrophages and healthy WT littermates (Supplementary Table S9). This suggests a near complete abrogation of neurodegeneration process in PD mice treated with GDNF-macrophages. Sham-transfected macrophages showed a subtle neuroprotective effect (Figure 5 C, ,E)E) that was much less than that in GDNF-macrophages treatment groups. The observed intrinsic biological activity of sham macrophages may be due to a particular subset of M2 cell-carriers differentiated with MCSF [26] that show mild neuroprotective effects by themselves. Additional images shown in Supplementary Figure S7.
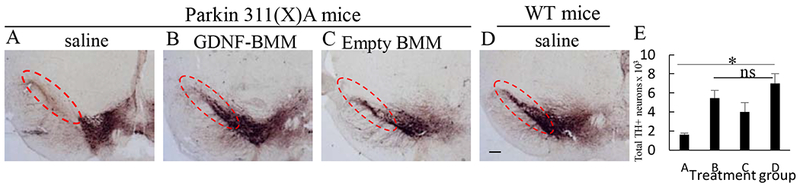
Transgenic mice (4 mo. old) were i.v. injected with PBS (A), or GDNF-transfected (B), or sham-transfected (C) macrophages (2x106 cells/100 μL/mouse) weekly 3x times. Wild type mice were systemically injected with PBS (D). Animals were sacrificed at mo 16, and brain slides were stained with TH, a marker for dopaminergic neurons. The obtained confocal images and quantification of TH+ neurons (E) indicate significant increases in neuronal survival in Parkin Q311(X)A mice upon GDNF-BMM treatment (B) compared to PD mice treated with saline (A). The administration of PD/sham BMM (C) also increased number of dopaminergic neurons in PD mice although at lesser extent than GDNF-transfected macrophages. The bar: 1 mm. Values are means ± SEM (N = 6), and *p < 0.05.
The neuropathology of PD includes brain inflammation, microglial activation, and secretion of neurotoxins, all of which contribute to neurodegeneration and degradation of motor functions [32]. Therefore, we assessed the effect of GDNF-macrophages on microglial activation in PD mice. The neurodegeneration in 16 mo. of age PD mice treated with saline was accompanied with substantial brain inflammation as displayed by up-regulated expression of MAC1 by microglia within the SNpc that exhibited a more amoeboid morphology (Figure 6 A, ,E)E) than ramified microglia in saline-treated WT mice (Figure 6 D, ,E).E). Thus, postmortem brains of PD mice treated with saline showed significant higher number of activated microglia when compared to brains from WT mice (Supplementary Tables S10 and S11). In contrast, a potent anti-inflammatory effect of GDNF-macrophages was demonstrated in PD mice (Figure 6 B, ,E)E) compared to PD mice treated with saline (Figure 6 A, ,E)E) that was confirmed by reduction in microgliosis as measured by MAC1 expression using one-way ANOVA and Bonferroni test (Supplementary Tables S10 and S11). Of note, treatment with sham-transfected macrophages produced a mild anti-inflammatory effect in PD mice (Figure 6 C, ,E).E). Additional images shown in Supplementary Figure S8.
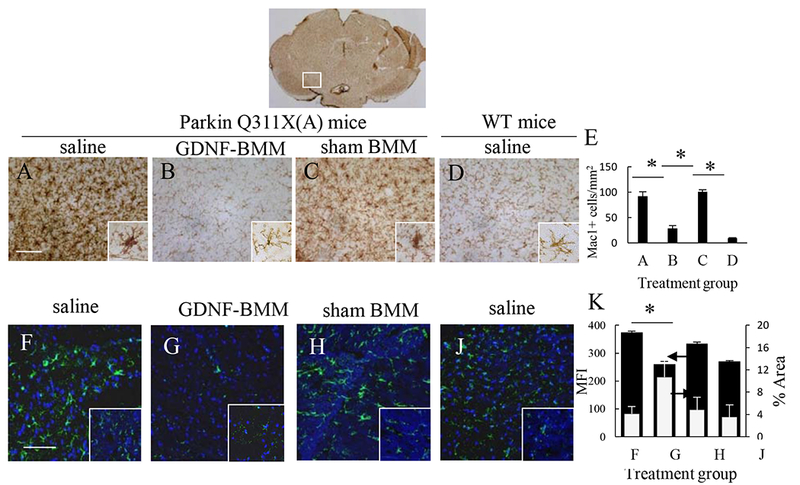
Transgenic mice (4 mo. old) were i.v. injected with saline (A, F), or GDNF-macrophages (B, G), or sham-transfected macrophages (C, H) (2x106 cells/100 μL/mouse, once a week, 3x weeks). WT mice were systemically injected with saline (D, J). Animals were sacrificed at mo. 16, and brain slides were stained with Mac1 (A – D), a marker for activated microglia; or Ab to GFAP (F – J), a marker for activated astrocytes. Insets on the right lower corner are higher-power view of the SNpc area. The obtained bright field (A – D) and confocal (F – J) images, and quantification of activated microglial cells (E) and activated astrocytes (K) determined as the function of the positive area by ImageJ software for mean Fluorescent Intensity (MFI) and % Area of fluorescence, indicate significant decreases neuroinflammation in the brain of PD mice upon GDNF-macrophages treatment (B, G) compared to PD mice treated with saline (A, F). The administration of sham-transfected macrophages (C, H) did not affect the number of activated microglial cells and activated astrocytes in PD mice. Values are means ± SEM (N = 6), and *p < 0.05. The bar: 100 μm. For detailed statistical comparisons, see Supplementary Tables S10 – S13.
Next, systemic administration of GDNF-macrophages significantly (Supplementary Tables S12 and S13) decreased astrocytosis in PD mice (Figure 6 G, ,K)K) compared to healthy wild type mice (Figure 6 J, ,K).K). No effect of sham-transfected macrophages (Figure 6 H, ,K)K) was observed in PD mice. Additional images are shown on Supplementary Figure S9. Overall, the effect on astrocytosis is consistent with decreases of microglial activation in PD mouse brain upon GDNF-macrophages treatment suggesting significant inhibition of the neuro-inflammation process.
An etiological factor, α-synuclein, is known to accumulate in SNpc, as well as other brain regions in PD patients [33, 34]. The effect of GDNF-macrophages on accumulation of proteinase K-resistant α-synuclein was examined using brain slides from PD mice at 16 mo. of age (Figure 7). Proteinase K treatment of brain slides from WT mice almost completely destroyed α-synuclein immunohistochemistry staining (Figure 7 D, ,E),E), while α-synuclein positive neurons were abundantly detected in cells located in the SNpc of PD mice (Figure 7 A, ,E).E). Importantly, PD mice treated with GDNF-macrophages (Figures 7 B, ,E)E) showed a significantly lower number of proteinase K-resistant α-synuclein (Supplementary Tables S14 and S15) compared to other PD animal groups. Interestingly, there was also substantial reduction of proteinase K-resistant α-synuclein in the SNpc of PD mice treated with sham transfected macrophages (Figure 7 C, ,E).E). We also attribute this effect to mild neuroprotective effects of M2 macrophages. Additional images shown on Supplementary Figure S10. Of note, no total weight loss was recorded in PD mice injected with either GDNF-transfected or sham-transfected macrophages (Supplementary Figure S11). This signifies absence of general toxicity of the cell-based formulations upon multiple administrations of macrophage treatments.
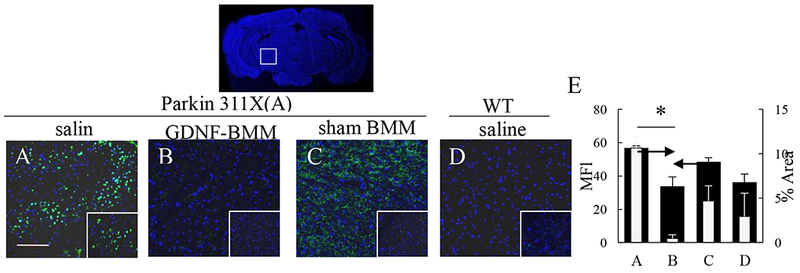
Transgenic mice (4 mo. old) were i.v. injected with saline (A), or GDNF-transfected (B), or sham-transfected (C) macrophages (2x106 cells/100 μL/mouse, once a week, 3x weeks). WT mice were systemically injected with saline (D). Animals were sacrificed at mo. 16, brain slides were treated with protease K, and stained with Ab to α-synuclein. Insets on the right lower comer are higher-power view of the SNpc area. The obtained confocal images, and quantification of α-synuclein staining (E) determined as the function of the positive area by ImageJ software for mean Fluorescent Intensity (MFI) and % Area of fluorescence, indicate significant reduction of aggregates formation upon treatment with GDNF-macrophages. Values are means ± SEM (N = 6), and *p < 0.05. The bar: 100 μm. For detailed statistical comparisons, see Supplementary Tables S12 – S13.
Finally, the expression analysis and histological stain by Nissl (Figure 8) revealed a normal distribution of neurons (N) with minimal loss of cell bodies in the SNpc of WT mice (4 mo). Tissue showed neurons with both pyramidal and non-pyramidal shape and integrative and well maintained morphology. Some characteristics analysis showed extension of the Nissl substance (chromatic substance) into the base of each dendrite (D) but not into the axon hillock that gives rise to the axon (A). The nucleus was visible inside ells with no apparent presence of Nissl-positive chromatin clumps. Presence of glial nuclei (G) and astrocytes (As) were also evident. In contrast, tissues from 4 mo. old PD mice showed lower number of Nissl bodies with fewer numbers of neurons, glial nuclei and astrocytes present, when compared with the 4 mo. old WT mice tissues. In general, all brain tissues removed post-mortem from old mice showed reduced number of cells in comparison to brain tissues from young mice. In the case of the control old WT mice, lower number of Nissl bodies were detected. Despite the lower presence of Nissl bodies, neurons morphology and the number of glial cells present were similar to brain tissues from old mice. Of note, the brain tissues from PD mice treated with saline at 16 mo. of age showed presence of large sized neuronal cells with detectable loss of dendrites and axons, and neuron vacuolation (Figure 8). In contrast, tissues from PD mice treated with the GDNF-macrophages showed better tissue integrity; the neurons appeared healthier with normal morphology and minimal sign of neuronal vacuolation. Finally, tissues from PD mice treated with sham macrophages at 16 mo. showed both flat elongated and dopaminergic neurons.
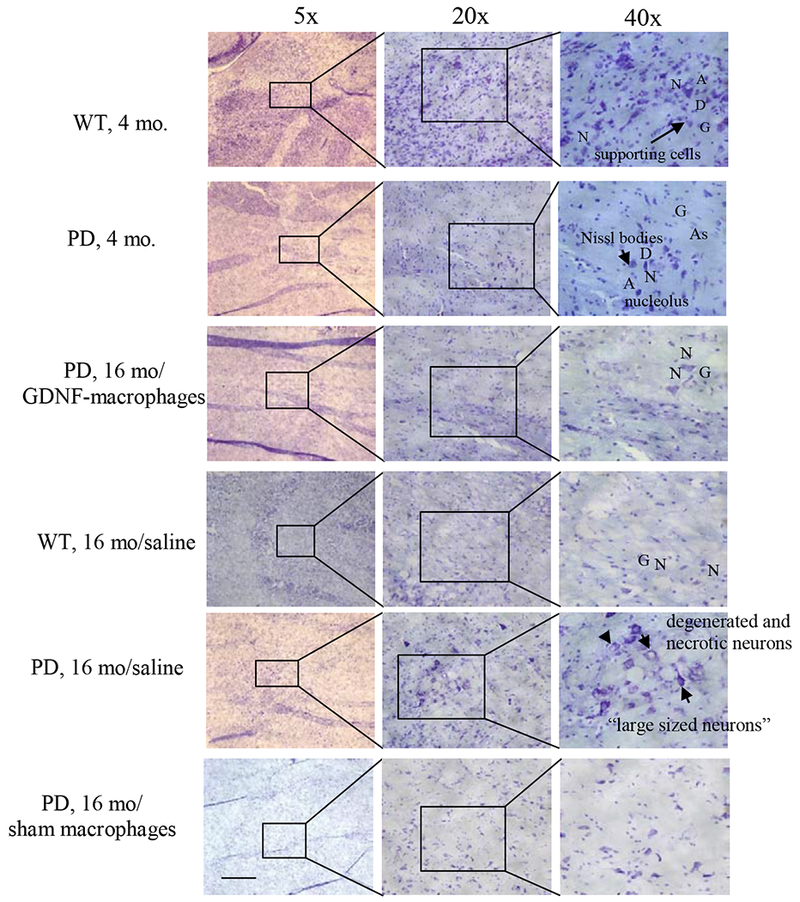
Transgenic mice (16 mo. old) were i.v. injected with GDNF-transfected macrophages (2x106 cells/100μL/mouse, once a week, 3 x weeks) or saline, or sham macrophages. WT mice, or PD mice (16 mo. old) were systemically injected with saline. Animals were sacrificed at mo. 22, and brain slides were stained with Nissl staining. The obtained bright light images indicate neuroprotective effects in the brain of PD mice upon GDNF-macrophages treatment compared to PD mice treated with saline. The administration of sham macrophages did not have significant therapeutic effect in PD mice. The bar: 200 μm.
H&E staining of the brain slides of PD mice at late stages of the disease confirmed the protection of neuronal cells in SNpc by systemic administration of GDNF-macrophages (Supplementary Figure S12). Microscopic appearance of the substantia nigra stained with H&E showed normal cell morphology with minimal vacuolation in brain tissues removed post-mortem from 4 mo. old WT mice and in brain tissues removed post-mortem from 4 mo. old PD mice. In contrast, brain tissues from WT mice or PD mice that were treated with saline at 16 mo. of age showed signs of disruption and vacuolation. Of note, only groups from PD mice treated with the GDNF-macrophages at 16 mo. of age showed higher integrity, with no visible sign of aberrant morphology of neurons.
4. Discussion
Cell therapy for PD patients is an innovative promising approach that may be a key for true neuroregeneration and reverse of the disease progression [35]. Early studies utilized transplantation of fetal nigral DA neurons and autologous DA-secreting cells was first utilized to preserve host neurons and supply brain tissues with DA [36, 37]. Later, allogeneic bone marrow-derived mesenchymal stem cells (MSCs) [19, 38], embryonic stem cells (ESCs) [39], and neural stem cells NSCs [40, 41] were used to support/induce damaged DA neurons in animal PD models. Finally, human induced pluripotent stem cells (iPSCs) were shown to protect neurons after cerebral transplantation [42]. In 2016, the first approved clinical trial using iPSCs to treat PD patients was started in Melbourne, Australia, by the International Stem Cell Corporation [43]. Cell-based interventions showed strong therapeutic effects upon intracranial transplantations that was utilized in most of these studies. Unfortunately, surgery requires relatively invasive maneuvers and involves expensive medical care. Furthermore, a risk of immune rejection, and possibility for tumor formation are among disadvantages of these cell-based therapeutic options [44].
A long-term objective of these investigations is to develop targeted cell-mediated drug delivery system for therapeutic proteins to the brain using autologous macrophages. We demonstrated here that genetically modified macrophages can reach the brain in Parkin Q311X(A) mice upon systemic administration and produce potent long-term therapeutic effects. To take advantage of the ability of macrophages to deliver therapeutic proteins to the inflamed brain, primary macrophages should be efficiently transfected with therapeutic pDNA, which is not a simple task. We verified several approaches for transfection of primary macrophages including using specific jPEI-macrophage reagent (jPEI) and electroporation method. The obtained results indicated that efficient transfection of primary macrophages were achieved with reporter (Luc, or GFP) and therapeutic (GDNF) pDNA ex vivo using both jPEI transfection reagent and electroporation methods. Moreover, media collected from GDNF-macrophages promoted axonal outgrowth in PC 12 neuronal cells in vitro. This signifies that GDNF was released from pre-transfected macrophages in active form. Of note, we demonstrated earlier [26, 45–48] that GDNF-macrophages are secreting extracellular vesicles (EVs), packed with therapeutic protein-encoding genetic material (pDNA and mRNA), active GDNF, and NF-κb, a transcription factor involved in the encoded gene expression. This mechanism may play a significant role in efficient preservation of neurons against degradation [26]. In addition, the membranotropic properties of GDNF-carrying EVs may facilitate GDNF binding to GFRα-1 receptors expressed on DA neurons [18].
These investigations were carried out in a transgenic PD mouse model that manifests in slow developing neurodegeneration upon mild inflammation and accumulation of α-synuclein deposits in the mid brain. One of the most important findings of this work is that GDNF-macrophages injections were able to not only prevent the neurodegeneration in PD mice that were treated at mild early-stages of the disease, but also restore the functions of neurons in mice with already developed neurodegeneration at late severe-stages of the disease. Thus, systemically administered GDNF-expressing macrophages migrated to sites of brain inflammation, accumulated in the brain of transgenic mice and provide potent neuroprotection that is consistent with our previously reported findings for toxin-induced disease models with acute brain inflammation [26, 28, 46, 47, 49, 50]. Of note, accumulation of Luc-macrophages in the brain of PD mice was greater than in their wild type counterparts. These findings are of great importance considering mild neurodegeneration and neuroinflammation process in PD patients.
The treatments resulted in significant improvement in locomotor activity in PD mice that was signified in increased remaining time in Wire hanging and Rotarod tests, and reduced escaping time for transgenic PD mice. The second important finding is that GDNF-macrophages provided long-term effect on motor functions for more than a year. This suggests that future Parkinson’s patients potentially might need receiving treatment only once a year, or even longer, that is very important for this chronic disease. GDNF delivered to the PD brain has direct effects on neuronal integrity that in turn resulted in reduction of astrocytosis and microgliosis. No significant effects were observed in PD mice treated with sham-transfected macrophages, although some tendency for increased neuron survival, and decreased in proteinase K-resistant α-synclein, as well as improved motor functions in these mice by sham-macrophages were recorded. This later effect may be attributed to anti-inflammatory subtype of M2 macrophages utilized in these studies. We reported similar effects in PD mice with acute inflammation earlier [26, 48]. Importantly, systemically-administered M2 macrophages home brain tissues, and sustained their phenotype in PD mouse models. Finally, contrary to toxin-induced conditions, PD patients do not have a high inflammation levels in the brain. Therefore, the note that even mild inflammation process in transgenic mice at early stages of the disease promoted brain accumulation of systemically injected cell-carriers is significant regarding possible targeting of therapeutic proteins to the brain in PD patients.
The long-term sustained therapeutic effect of adoptively transferred GDNF-macrophages was observed for a duration of 12 months after the treatment in ParkinQ311(X)A mice. We suggested that the mechanism of this therapeutic intervention is more complex than a simple GDNF delivery by genetically-modified cell-carriers. We hypothesized that GDNF-macrophages accomplished horizontal gene transfer to surrounding brain tissues as well. In this respect, we reported earlier that systemic administration of macrophages transfected ex vivo with a plasmid DNA (pDNA) encoding a potent antioxidant enzyme, catalase, produced months-long expression levels of catalase in the brain resulting in three-fold reductions in inflammation and complete neuroprotection in different mouse models of PD [26]. As a result, the single systemic administration of catalase-transfected macrophages significantly improved motor functions in PD mice.
Mechanistic studies revealed that transfected macrophages secreted extracellular vesicles, exosomes, packed with catalase genetic material, pDNA and mRNA, active catalase, and NF-κb, a transcription factor involved in the encoded gene expression. Exosomes efficiently transferred their contents to contiguous neurons resulting in de novo protein synthesis in target cells. Therefore, genetically modified macrophages serve as a highly efficient system for reproduction, packaging, and targeted gene and drug delivery to treat inflammatory and neurodegenerative disorders. Furthermore, horizontal gene transfer from macrophages to neuronal cells was confirmed based on different kinetics of gene expression in macrophages and target PC12 neuronal cells in vitro. The co-culture of these cells displays a delayed GFP expression profile with the highest levels shifted to day 8–12, while a pick of GFP expression in macrophages alone is on day 4 [26]. Next, we also demonstrated that macrophages that were pre-transfected with pDNA under tissue-specific Desmin promoter (muscles) pass the transgene to Desmin-positive myotubes, but not to Desmin-negative myoblasts [51]. Thus, the transferred gene is subsequently expressed in the acceptor cells, even if it is not transcribed in the initial cell-carriers, macrophages. Finally, we showed that macrophages can transfer exogenously introduced siRNA to surrounding cancer cells into solid tumors [52]. Thus, the knockdown of GFP expression in the human triple negative breast cancer (TNBC) MDA-231-GFP cells was demonstrated by IC21 macrophages loaded with GFP-siRNA via horizontal transfer of GFP-siRNA. Additionally, the delivery of therapeutic CIB1 -siRNA to MDA-MB-468 cells was confirmed in orthotopic solids in nude mice [52]. Overall, we concluded that the observed in this study sustained therapeutic effects of GDNF-macrophages are likely due to the horizontal gene transfer via GDNF-macrophages, transfection of brain tissue and prolonged expression of GDNF in the brain. Ongoing studies in our lab are investigating more closely the mechanism of therapeutic effects by GDNF-transfected macrophages and the potential reversal of degeneration processes in the PD brain.
Supplementary Material
1
2
3
Acknowledgments
This study was supported in part by the National Institutes of Health grant 1RO1 NS102412 (EVB), the North Carolina Biotechnology Center UNC TEG15-4849 (EVB), the Eshelman Institute for Innovation grant UNC EII29-201 (AVK), and NC TraCS Institute CTSA-UL1TR002489 (JF). We are very grateful to Mr. T. Greenwood for financial support as well as various invaluable comments and suggestions. We are also thankful to the Senior Director of Development at UNC K. Collins for her assistance with communication the strategy and facilitating the support of this project.
Footnotes
Publisher's Disclaimer: This is a PDF file of an article that has undergone enhancements after acceptance, such as the addition of a cover page and metadata, and formatting for readability, but it is not yet the definitive version of record. This version will undergo additional copyediting, typesetting and review before it is published in its final form, but we are providing this version to give early visibility of the article. Please note that, during the production process, errors may be discovered which could affect the content, and all legal disclaimers that apply to the journal pertain.
Reference List
Full text links
Read article at publisher's site: https://doi.org/10.1016/j.jconrel.2019.10.027
Read article for free, from open access legal sources, via Unpaywall:
https://www.ncbi.nlm.nih.gov/pmc/articles/PMC6927551
Citations & impact
Impact metrics
Article citations
Growth Factors and Their Application in the Therapy of Hereditary Neurodegenerative Diseases.
Biomedicines, 12(8):1906, 20 Aug 2024
Cited by: 0 articles | PMID: 39200370 | PMCID: PMC11351319
Review Free full text in Europe PMC
Theoretical basis, state and challenges of living cell-based drug delivery systems.
Theranostics, 14(13):5152-5183, 19 Aug 2024
Cited by: 0 articles | PMID: 39267776 | PMCID: PMC11388066
Review Free full text in Europe PMC
Evaluation of the Safety and Efficacy of Repeated Mesenchymal Stem Cell Transplantations in ALS Patients by Investigating Patients' Specific Immunological and Biochemical Biomarkers.
Diseases, 12(5):99, 12 May 2024
Cited by: 0 articles | PMID: 38785754 | PMCID: PMC11120501
Long-term benefits of hematopoietic stem cell-based macrophage/microglia delivery of GDNF to the CNS in a mouse model of Parkinson's disease.
Gene Ther, 31(5-6):324-334, 16 Apr 2024
Cited by: 1 article | PMID: 38627469 | PMCID: PMC11245959
An updated systematic review of neuroprotective agents in the treatment of spinal cord injury.
Neurosurg Rev, 47(1):132, 28 Mar 2024
Cited by: 4 articles | PMID: 38546884
Review
Go to all (22) article citations
Data
Data behind the article
This data has been text mined from the article, or deposited into data resources.
BioStudies: supplemental material and supporting data
RefSeq - NCBI Reference Sequence Database
- (1 citation) RefSeq - NM_199234
Similar Articles
To arrive at the top five similar articles we use a word-weighted algorithm to compare words from the Title and Abstract of each citation.
GDNF-expressing macrophages mitigate loss of dopamine neurons and improve Parkinsonian symptoms in MitoPark mice.
Sci Rep, 8(1):5460, 03 Apr 2018
Cited by: 23 articles | PMID: 29615705 | PMCID: PMC5882968
Genetically modified macrophages accomplish targeted gene delivery to the inflamed brain in transgenic Parkin Q311X(A) mice: importance of administration routes.
Sci Rep, 10(1):11818, 16 Jul 2020
Cited by: 11 articles | PMID: 32678262 | PMCID: PMC7366622
GDNF-transfected macrophages produce potent neuroprotective effects in Parkinson's disease mouse model.
PLoS One, 9(9):e106867, 17 Sep 2014
Cited by: 80 articles | PMID: 25229627 | PMCID: PMC4167552
Adenoviral vector-mediated delivery of glial cell line-derived neurotrophic factor provides neuroprotection in the aged parkinsonian rat.
Clin Exp Pharmacol Physiol, 28(11):896-900, 01 Nov 2001
Cited by: 9 articles | PMID: 11703392
Review
Funding
Funders who supported this work.
Eshelman Institute for Innovation (1)
Grant ID: UNC EII29-201
Mr. T. Greenwood
NC TraCS Institute (1)
Grant ID: UL1TR002489
NCATS NIH HHS (1)
Grant ID: UL1 TR002489
NINDS NIH HHS (3)
Grant ID: R01 NS112019
Grant ID: R01 NS057748
Grant ID: R01 NS102412
National Institutes of Health (1)
Grant ID: 1RO1 NS102412
North Carolina Biotechnology Center (1)
Grant ID: TEG15-4849