Abstract
Background
Risk of transfusion-transmitted (TT) malaria is mainly associated with whole blood (WB) or red blood cell (RBC) transfusion. Risk mitigation relies mostly on donor deferral while a limited number of countries perform blood testing, both negatively impacting blood availability. This study investigated the efficacy of the pathogen reduction system using amustaline and glutathione (GSH) to inactivate Plasmodium falciparum in WB.Study design and methods
WB units were spiked with ring stage P. falciparum infected RBCs. Parasite loads were measured in samples at time of infection, after 24 hours at room temperature (RT), and after a 24-hour incubation at RT post-treatment with 0.2 mM amustaline and 2 mM GSH. Serial 10-fold dilutions of the samples were inoculated to RBC cultures and maintained up to 4 weeks. Parasitemia was quantified by cytometry.Results
The P. falciparum viability assay has a limit of detection of a single live parasite per sample. Input parasite titer was >5.7 log10 TCID50 per mL. A 24-hour incubation at RT paused parasite development in controls, but they retained viability and infectivity when tested in culture. In contrast, no infectious parasites were detected in the amustaline/GSH-treated sample after 4 weeks of culture.Conclusion
A robust level of P. falciparum inactivation was achieved in WB using amustaline/GSH treatment. Parasite log reduction was >5.7 log10 TCID50 per mL. Development of such a pathogen reduction system may provide an opportunity to reduce the risk of TT malaria and improve blood availability.Free full text
Inactivation of Plasmodium falciparum in whole blood using the amustaline and glutathione pathogen reduction technology
Abstract
BACKGROUND
Risk of transfusion‐transmitted (TT) malaria is mainly associated with whole blood (WB) or red blood cell (RBC) transfusion. Risk mitigation relies mostly on donor deferral while a limited number of countries perform blood testing, both negatively impacting blood availability. This study investigated the efficacy of the pathogen reduction system using amustaline and glutathione (GSH) to inactivate Plasmodium falciparum in WB.
STUDY DESIGN AND METHODS
WB units were spiked with ring stage P. falciparum infected RBCs. Parasite loads were measured in samples at time of infection, after 24hours at room temperature (RT), and after a 24‐hour incubation at RT post‐treatment with 0.2
mM amustaline and 2
mM GSH. Serial 10‐fold dilutions of the samples were inoculated to RBC cultures and maintained up to 4
weeks. Parasitemia was quantified by cytometry.
RESULTS
The P. falciparum viability assay has a limit of detection of a single live parasite per sample. Input parasite titer was >5.7 log10 TCID50 per mL. A 24‐hour incubation at RT paused parasite development in controls, but they retained viability and infectivity when tested in culture. In contrast, no infectious parasites were detected in the amustaline/GSH‐treated sample after 4weeks of culture.
CONCLUSION
A robust level of P. falciparum inactivation was achieved in WB using amustaline/GSH treatment. Parasite log reduction was >5.7 log10 TCID50 per mL. Development of such a pathogen reduction system may provide an opportunity to reduce the risk of TT malaria and improve blood availability.
The protozoan Plasmodium is the responsible agent for malaria, an arthropod‐borne disease transmitted through the bites of infected Anopheles mosquitoes. Five species can infect humans: P. malariae, P. ovale, P. knowlesi, P. vivax, and P. falciparum. Of these, P. falciparum is responsible for the majority of severe malaria cases and is the predominant species in sub‐Saharan Africa, while P. vivax is most commonly found in South America and responsible of half of the cases in Southeast Asia.1 In 2018, an estimated 228 million malaria cases and 405,000 deaths were reported to the World Health Organization.1 A majority of these occurred in sub‐Saharan Africa, affecting mainly children under the age of 5 and pregnant women.1 Malaria is endemic in 87 countries and the number of cases in non‐endemic areas is significant due to global trends in heightened population mobility. According to the 2017 European Centers for Diseases Control (ECDC) annual report, 8023 malaria imported cases were reported in Europe; most of the cases were found in France, United Kingdom, and Germany.2 The notification rate increased from 2013‐2015, decreased in 2016, and has been stable since.2
Parasites in the sporozoite stage, injected into the skin during an Anopheles blood meal, migrate to the liver and infect hepatocytes where parasites undertake a hepatic phase of replication, which is asymptomatic in humans. Parasite division produces thousands of merozoites that, once released into the bloodstream, infect red blood cells (RBCs), where they undergo an asexual life cycle responsible for the clinical symptoms of the disease. Along with the natural infection by mosquito bites, accidental transmissions can also occur through blood transfusions from infected donors to recipients. The first case was reported by Woosley in 1911.3 Transfusion‐transmitted (TT) malaria is mostly associated with RBC concentrates although transmission by plasma and platelet components has also been reported.4, 5
In non‐endemic countries, TT malaria is due to infected travelers and immigrants coming from endemic areas. This kind of malaria transmission may cause severe clinical symptoms especially because recipients are often unaware or immunosuppressed.5 Delayed diagnosis is also a cause of significant morbidity and mortality.6 To avoid TT infections, WHO released guidelines in 2016 for blood transfusion safety and hemovigilance criteria that are defined by each country. Generally, screening starts by a pre‐donation questionnaire leading to the deferral of donors returning from travel to endemic areas. Blood screening for malaria parasites differs between countries,7 several diagnostic screening methods are available (microscopic evaluation, antibody‐based, or PCR methods). However, concerns around the sensitivity and specificity of these tests have been raised3, 8 as it is known that 10 parasites per blood donation are enough to transmit the disease,9 while serology does not give definitive information on the infection status of an individual since positive results can be obtained for many years after infection, and in the absence of reinfection, due to residual antibodies.10
In contrast, in many endemic countries, especially in Sub‐Saharan Africa, deferral of blood donors based on exposure to malaria parasite is not practical because of the high incidence. Malaria deferrals would lead to high rates of donor exclusion (>30%)11 and blood shortages. Furthermore, screening for malaria parasites is not routinely performed.12 Instead, WHO recommends the administration of appropriate and effective malarial prophylaxis to all blood recipients to prevent symptomatic malaria infection.13 However, there is little information in the literature on the level of implementation of the recommendation in the field.14 In addition, the replacement of chloroquine prophylaxis with more expensive artemisinin‐based therapies makes the practice difficult.15
In non‐endemic countries, deferral policies lead to 1.4%‐25% deferred donors in several European countries, USA, and Canada.16 An alternative approach to avoid the loss of blood donations and to manage the potential Plasmodium transfusion‐transmission risk in both endemic and non‐endemic areas is offered by the use of pathogen reduction technology (PRT). Currently, two technologies based on ex vivo photo‐chemical treatments of blood products demonstrated efficient inactivation of P. falciparum: the INTERCEPT Blood System for platelets and plasma using amotosalen (S‐59) and UVA illumination to cross‐link nucleic acids, that is currently FDA licensed in the US,17 and the MIRASOL PRT for the treatment of plasma, platelets, and whole blood (WB) using riboflavin and UV light to cause nucleic acid damage by reactive oxygen species.18, 19 A MIRASOL PRT randomized controlled trial in a malaria endemic region of Ghana showed a significant reduction of incidence of TT malaria in non‐parasitemic patients.19
However, photochemical treatments of RBC or WB is limited by the presence of hemoglobin, which absorbs the light thus reducing the efficiency of the illumination step. Cerus Corporation developed an alternative nucleic acid targeted PRT for RBCs or WB, which is not dependent on UV illumination. The process is based on the use of amustaline (S‐303), designed to target nucleic acids through its acridine moiety, and cross‐link nucleic acids through its bis‐alkylator group, preventing DNA replication. An ester link between the acridine and the bis‐alkylator is hydrolyzed releasing acridine with slower kinetics.20, 21 Incubation for 20‐24hours results in the decomposition of amustaline below detection by liquid chromatography/mass spectrometry and the production of the predominant negatively charged breakdown product, S‐300, which is non‐reactive with nucleic acid.20 Glutathione (GSH) is used as a quencher to prevent non‐specific protein reactions and extracellular protein damage. Amustaline/GSH has been shown to be highly efficient at reducing high levels of a wide range of parasites, viruses, and bacteria in RBC.22, 23, 24 The current study investigated the efficacy of the amustaline/GSH PRT to inactivate P. falciparum in WB.
MATERIALS AND METHODS
P. falciparum culture
P. falciparum strain FCB1 from Colombia (MNHN‐CEU‐224‐PfFCB1) was provided from the Unicellular Eukaryote Collection of the National Museum of Natural History, France. P. falciparum was maintained in culture of human RBCs, at a 2%‐5% hematocrit, in RPMI 1640 medium (Gibco) supplemented with 0.5% AlbuMax II (Gibco), 25mM HEPES, pH 7.3, 2 g/L sodium bicarbonate, 2 g/L D‐glucose, 100
μM hypoxanthine, and 100
U/mL‐100
μg/mL Penicillin–Streptomycin, at 37°C, under a 5% CO2 atmosphere. RBCs were collected in citrate phosphate dextrose (CPD) by the Etablissement Français du Sang (EFS) from Rungis (Ile de France), washed twice (600 × g, 5 min) in RPMI 1640 medium and used within 15
days from the day of collection. Parasite ring stage cultures were obtained via synchronization by a double 5% sorbitol treatment.25 Parasite stages and parasitemia were routinely determined on Diff‐Quik‐stained smears (Medion Diagnostics). If not indicated otherwise, the culture medium was changed every day and cultures diluted with healthy RBCs when parasitemia reached 5%. To achieve a ~20% parasitemia level, cultures starting at ~
1% parasitemia were kept at 1% hematocrit and the medium was changed daily during two invasion cycles. The hematocrit was then increased to 1.5% before the third invasion.26
Experimental design
WB was collected with CPD at EFS Rungis and used within 2days post‐donation. Experimental design was summarized in Fig. Fig.1.1. For each replicate, the volume of the WB units was adjusted to approximately 465
mL. A total of approximately 1.46
×
109 ring‐infected RBC (iRBC) were pelleted by centrifugation (600 × g, 5 min). Approximately 30
mL of WB was withdrawn and used to resuspend the iRBC pellet. The iRBC suspension was delivered back into the WB unit and mixed gently by hand. A 5 mL control was removed at time 0 (UT0h) from the infected WB unit to determine the pre‐treatment P. falciparum titer and was assayed immediately. An additional 5 mL sample was removed and held at room temperature (RT, 22°C ±
2°C) in a 15
mL‐ventilated tube for 24
hours to determine the titer of an untreated control P. falciparum after a 24‐hour incubation (UT24h).
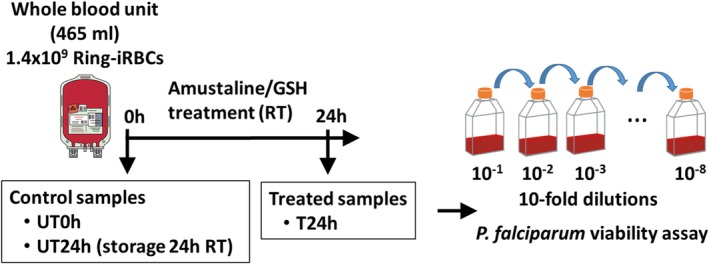
Process and time points of the evaluation of the P. falciparum inactivation by amustaline/GSH in WB. WB unit (465mL) was infected with 1.46
×
109 ring‐infected RBC. Controls UT0h and UT24h were removed before the 24‐hour treatment with amustaline and GSH from the INTERCEPT Blood System for RBC. Controls and treated (T24h) samples were processed for P. falciparum viability assay to determine parasite load and reduction. [Color figure can be viewed at http://wileyonlinelibrary.com]
The remaining infected WB unit was treated with amustaline and GSH components from the INTERCEPT Blood System for RBCs. The system comprises a GSH vial, an amustaline vial and a trifurcated set with two 0.2 μm filters and a blind lead. A 60mM solution of GSH in saline solution was added to the WB unit via the first 0.2 μm filter and the WB unit was mixed in an end‐over‐end motion. The resulting GSH concentration in the WB unit was 2
mM. Next, a 200
mM solution of amustaline was prepared in saline solution just before use, added into the WB unit via the second filter to yield a final concentration of 0.2
mM and mixed in an end‐over‐end motion. The filter set was sterilely detached and the WB unit was stored at RT for 24
hours. After the 24‐hour incubation, a 5 mL sample (T24h) was withdrawn from the treated WB unit and P. falciparum viability assay was performed.
P. falciparum viability assay
The viability assay was performed on the 5 mL control (UT0h and UT24h) and treated (T24h) samples. RBCs were separated from other WB components by a ficoll hypaque (GE Healthcare) centrifugation (400 × g, 30min). RBC pellets were washed twice (600 × g, 5 min) with culture medium and resuspended in culture medium to achieve a 5% hematocrit. Serial 10‐fold dilutions were performed (from 10−1 to 10−8) in culture medium containing 5% of fresh RBCs. For controls UT0h and UT24h, 120
mL of the 10−3 to 10−8 dilutions were split into 30
mL aliquots in four ventilated 75
cm2 flasks and cultured. The amustaline‐treated sample T24h was assayed in a similar fashion but only the 10−1 and 10−2 dilutions were split into four ventilated 75
cm2 flasks, each containing a 30
mL aliquot and cultured. Over a period of 4
weeks the culture medium was exchanged every 2 or 3
days. The cultures were monitored for P. falciparum growth by preparing smears on a microscope slide and staining with Diff‐Quik. If parasites were detected on a smear, parasitemia was quantified by flow cytometry after parasite staining with the nucleic acid cyanine dye YOYO‐1 using a glutaraldehyde‐fixed sample of the culture.27 Flasks were considered positive if parasitemia reached 1%.
Morphologic analysis of P. falciparum after amustaline/GSH treatment
Parasite morphology and development were followed in the cultures of 10−1 dilutions of the control sample UT24h and the amustaline/GSH treated sample T24h on Diff‐Quik‐stained smears. Smears were prepared at the time of culture of the 10−1 dilution (t0) and 36hours after (t0
+
36
hr).
Parasite titer and log reduction determination
Parasite titers were calculated using the Reed and Muench method28 and were expressed as the log10 of the median Tissue Culture Infectious Dose (log10 TCID50) using the formula:
where, PD = proportionate distribution = (% Infected Group 1‐50%) / (% Infected Group 1 ‐ % infected Group 2); Group 1 = Experimental group of highest dilution in which ≥50% of the cultures are positive for viable parasites; Group 2 = Experimental group of next higher dilution from Group 1.
The titer of parasites was calculated by subtracting the greatest dilution that still yielded detectable parasites from the calculated input control titer.
Log reduction was calculated for each unit according to the following formula:
Log10 (pre‐treatment titer ÷ post‐treatment titer). For samples in which no viable parasites were detected, log reduction was calculated using the input titer as the limit of inactivation. Log reduction was reported as the log10 TCID50 per mL.
RESULTS
Inactivation of P. falciparum assessed by viability assay
WB units were inoculated with P. falciparum‐infected RBCs at the ring stage (1.46×
109) to obtain a low level of parasitemia (0.05%‐0.1%) and inactivation was assessed by a 24‐hour incubation at RT of the WB unit with 0.2
mM amustaline with 2
mM GSH (Fig. (Fig.1).1). Two samples were collected just after infection and before addition of GSH and amustaline. One was immediately processed to determine the initial parasite load (untreated control UT0h). The other one was maintained at RT for 24
hours for determining the impact of the incubation on the parasite load (untreated control UT24h). A third sample was taken after GSH and amustaline addition and the 24‐hour incubation to determine the parasite log reduction. Parasite load and reduction were determined by maintaining a culture of 10‐fold serially diluted samples up to 4
weeks (Fig. (Fig.11).
Under these conditions, the sensitivity of the assay theoretically allows for the detection of a single live parasite per sample for the 10−7 to 10−8 dilution range (Table (Table1).1). This level of sensitivity was confirmed experimentally with parasites systematically detected in the assays performed for the 10−7 dilution in all three replicates and for one third of the replicates at the 10−8 dilution. For UT0h, the mean experimental parasite load of P. falciparum as determined by the viability assay was 5.7 ±0.6 log10 TCID50 per mL (Table (Table2).2). As shown in Fig. Fig.2,2, there was a positive and reproducible correlation between the parasitemia development in the different dilution assays and the time of culture (R2 value >
0.95). This indicates that the P. falciparum viability assay was reliable.
Table 1
Sample dilutions and theoretical loads of infected RBC
Whole blood (WB) Sample | Resuspended RBC pellet | 10‐fold dilutions | ||||||||
---|---|---|---|---|---|---|---|---|---|---|
10−1 | 10−2 | 10−3 | 10−4 | 10−5 | 10−6 | 10−7 | 10−8 | |||
Assay volume (mL) | 5 | 50 | 120 | 120 | 120 | 120 | 120 | 120 | 120 | 120 |
iRBC number/assay | 1.55 ×![]() | 1.55 ×![]() | 3.7 ×![]() | 3.7 ×![]() | 3.7 ×![]() | 3.7 ×![]() | 370 | 37 | 3.7 | 0.37 |
iRBC number/mL | 3.1 ×![]() | 3.1 ×![]() | 3.1 ×![]() | 3.1 ×![]() | 310 | 31 | 3.1 | 3.1 ×![]() | 3.1 ×![]() | 3.1 ×![]() |
Calculations are based on a 465mL WB unit, an input of 1.46
×
109 iRBC, and a 5 mL sample collected just after infection of the unit.
Table 2
Inactivation of P. falciparum in WB by the amustaline/GSH treatment
Replicate | Control samples (Log10 TCID50/mL) | Treated samples (TCID50/mL) | Log reduction/mL* | |
---|---|---|---|---|
UT0h | UT24h | T24h | ||
1 | 6.0 | 5.2 | 0.0 | >6.0 |
2 | 6.0 | 5.2 | 0.0 | >6.0 |
3 | 5.0 | 4.0 | 0.0 | >5.0 |
Mean![]() ![]() | 5.7![]() ![]() | 4.8![]() ![]() | 0.0 | >5.7![]() ![]() |
T = test; UT = untreated controls.
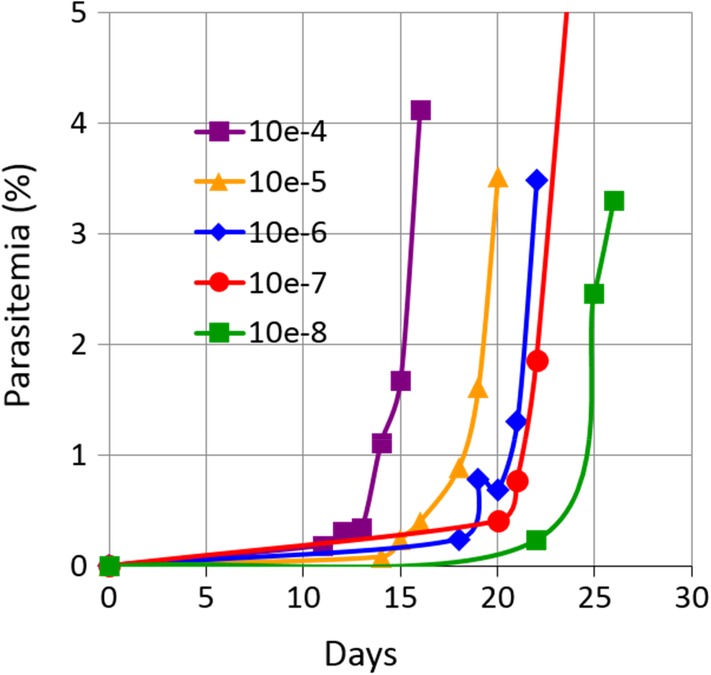
Example of a P. falciparum viability assay from untreated control UT0h. A WB unit (467mL, 40% hematocrit) was infected with 1.46
×
109 ring‐iRBCs. After mixing, a 5 mL sample was collected and processed for P. falciparum viability assay as described in Materials and Methods. Parasitemia was followed in assays of dilution 10−4 to 10−8. Good correlation was measured between parasitemia development for the different dilutions and time of culture (R2 =
0.975 for the time to reach 1% parasitemia). [Color figure can be viewed at http://wileyonlinelibrary.com]
Notably, the 24‐hour RT incubation had an impact by itself on the sensitivity of the viability assay by reducing the TCID50 value almost one log from 5.7 ±0.6 log10 TCID50 per mL (UT0h) to 4.8 ±
0.7 (UT24h) (Table (Table2).2). Such a reduction reflects that a proportion of ring parasites were not able to restart growth and development under appropriate conditions, or that their growth was delayed. It has been shown that under stress conditions, such as a decrease of temperature, ring parasites stay latent and their growth is attenuated.29
Efficiency of the amustaline/GSH treatment to inactivate P. falciparum in WB
After a 24‐hour treatment with amustaline/GSH (T24h), no evidence of parasite growth was found in the 10−1 and 10−2 dilutions up to 4weeks of culture, meaning an average log reduction >5.7 log10 TCID50 per mL compared to the untreated controls UT0h (Table (Table2).2). In the controls, when maintained in culture, ring parasites developed and differentiated into infective merozoites producing new cycles of RBC infection (Fig. (Fig.3A‐C).3A‐C). In contrast, after the amustaline/GSH treatment, ring parasites (Fig. (Fig.3D)3D) were unable to proliferate; they degenerated (Fig. (Fig.3E,3E, F) and were expelled from the RBCs. No surviving parasites were observed after a week of culture.
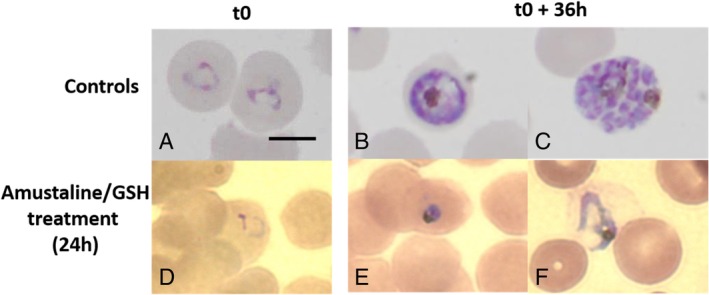
P. falciparum death after amustaline/GSH treatment. Parasite morphology and development were followed on Diff‐Quik stained smears in cultures of 10−1 dilutions of the control sample maintained 24hours at RT (UT24h) and the amustaline/GSH treated sample (T24). Smears were prepared at the time of the culture of 10−1 dilutions (t0) and after 36
hours of culture (t0
+
36
hr). In control UT24h, ring parasites (A) develop into trophozoite (B) and schizont stages with differentiation of infective merozoites (C), which will reproduce a new intraerythrocytic cycle. In contrast, in treated parasites T24h, rings (D) rapidly degenerate after maintaining in culture (E, F) and parasites were expelled from the RBCs. Images were produced on smears of one replicate and are representative of the two other study replicates. Bar: 5 μm. [Color figure can be viewed at http://wileyonlinelibrary.com]
DISCUSSION
The amustaline/GSH PRT was assessed for its ability to inactivate P. falciparum in WB. Among the five species able to infect humans, we evaluated P. falciparum, the most virulent malaria agent and also the most frequent cause of TT malaria. To reproduce malaria infection generally observed in donors, WB units were infected with parasites at the ring stage and at a low level of parasitemia (0.05%‐0.1%). P. falciparum intraerythrocytic development in humans is synchronous and the ring stage is the main parasite stage found in peripheral blood collected for blood transfusion. The results of our study demonstrate robust inactivation of P. falciparum in WB. Treatment with amustaline/GSH resulted in parasite titer reduction of at least 5.7 log10 TCID50 per mL in WB. The P. falciparum viability assay developed here proved highly sensitive, allowing detection of a single viable parasite per collected WB sample, suggesting inactivation of parasites to below the level of detection by the amustaline/GSH treatment using conditions of infection of the WB unit described above (1.46×
109 parasites/465
mL WB unit). No relapse of treated parasites was observed after 4
weeks of culture, supporting a complete parasite inactivation.
This high efficiency of parasite inactivation was corroborated by comparative microscopy, contrasting the morphology and development of treated and untreated parasites. Growth and expansion of treated parasites is completely and irreversibly inhibited after a 24hour treatment in contrast to untreated parasites, which develop into trophozoites and schizonts, divide into infective merozoites (Fig. (Fig.3)3) and undertake new intraerythrocytic cycles when maintained in appropriate culture conditions, even after 24
hours of incubation at RT. After amustaline/GSH treatment, ring parasites rapidly degenerated when cultured. Dead parasites were progressively expelled from RBCs and no intracellular parasites could be observed after 1
week of culture.
Parasite concentration used in the study (3.1×
106 parasites/WB mL) was within the range of the highest parasite concentrations found in blood donations (from 50 to 2.35
×
107 parasites per mL) used to evaluate the MIRASOL PRT efficiency on TT malaria in an endemic region of Ghana.19 Although not total, a mark reduction of incidence of TT malaria was notified in the non‐parasitemic patient cohort transfused with MIRASOL treated blood versus non‐treated blood (4% TT malaria vs. 16%, respectively).19 Based on the high efficiency of the amustaline/GSH treatment, at least similar efficiency would be expected in reducing TT malaria incidence in the field.
Interestingly, amustaline shares some resemblance to the 9‐aminoacridine derivatives found in antimalarial drugs such as quinacrine. These drugs are efficacious against P. falciparum due to high accumulation inside the intracellular parasite, particularly inside the food vacuole, a lysosome‐like compartment. In addition to a parasite DNA cross‐linkage, such accumulation inside the intracellular parasite and the food vacuole corresponded to an inhibition of essential cytoplasmic and vacuolar functions. The reactive alkylating properties of amustaline might explain the high efficiency of amustaline to inactivate P. falciparum in WB units that would overcome the limitation of the efficiency of photochemical PRTs (amotosalen INTERCEPT and MIRASOL) due to the light absorption by hemoglobin.
The amustaline/GSH treatment for WB has high potential for use in low‐ and middle‐income countries (LMIC) that are endemic for malaria. According to WHO, less than 70% of the WB collected is processed into components in low‐ and lower‐middle‐income countries (50 and 59%, respectively) whereas more than 90% of blood collections are processed into components in upper‐middle‐income countries and high‐income countries.30 The main reasons are that many LMIC have to deal with the lack of appropriate infrastructure for blood collection and storage conditions for blood components.31 Development of pathogen inactivation systems for WB for these countries is thus relevant as the most transfused products are WB followed by RBC concentrates to treat life‐threatening emergencies, like massive bleeding (mostly post‐partum hemorrhage) and severe anemia (malaria related anemia in children). In addition, a PRT using a process that does not require an illumination device and can be performed with low electricity requirements may be attractive in limited resource settings where infrastructures are under‐developed and where a limited number of trained staff may be available.32
In conclusion, our study demonstrated that the inactivation of P. falciparum to below the limit of detection can be achieved in WB units using the amustaline/GSH PRT. Development of such a pathogen reduction system may provide an opportunity to reduce the risk of TT malaria in endemic areas where current WHO recommendations assessing for donor suitability (identification of donors at the lowest risk of infection, screening of donations for parasitemia, malaria antigen or DNA) are impractical.
CONFLICT OF INTEREST
MCL and YAG are employees of Cerus Corporation; AL and AS were employees of Cerus Corporation at the time this study was executed. CS, PG, and SAED declare no conflict of interest relevant to this manuscript.
ACKNOWLEDGMENTS
Lisy Raveendran, Soraya Chaouch, Elisabeth Mouray from MCAM, MNHN, and MNHN platform of cytometry are thanked for their help to culture and provide cytometry analyses. Peter Bringmann from Cerus Department of Microbiology is thanked for his critical review of this work.
This work was partially funded by Cerus and the Humanitarian Foundation Swiss Red Cross.
Notes
Source of support in the form of grants, equipment, or drugs.
This work was partially funded by Cerus Corporation (USA) and by the Humanitarian Foundation Swiss Red Cross.
REFERENCES
Full text links
Read article at publisher's site: https://doi.org/10.1111/trf.15734
Read article for free, from open access legal sources, via Unpaywall:
https://europepmc.org/articles/pmc7187285?pdf=render
Citations & impact
Impact metrics
Citations of article over time
Alternative metrics
Smart citations by scite.ai
Explore citation contexts and check if this article has been
supported or disputed.
https://scite.ai/reports/10.1111/trf.15734
Article citations
Transfusion-Transmitted Malaria and Mitigation Strategies in Nonendemic Regions.
Transfus Med Hemother, 49(4):205-217, 15 Jul 2022
Cited by: 4 articles | PMID: 36159954 | PMCID: PMC9421689
Review Free full text in Europe PMC
Robust inactivation of Plasmodium falciparum in red blood cell concentrates using amustaline and glutathione pathogen reduction.
Transfusion, 62(5):1073-1083, 06 Apr 2022
Cited by: 3 articles | PMID: 35385146 | PMCID: PMC9325390
Inactivation of SARS-CoV-2 in All Blood Components Using Amotosalen/Ultraviolet A Light and Amustaline/Glutathione Pathogen Reduction Technologies.
Pathogens, 11(5):521, 28 Apr 2022
Cited by: 2 articles | PMID: 35631042 | PMCID: PMC9147860
Malaria : Stellungnahmen des Arbeitskreises Blut des Bundesministeriums für Gesundheit.
Bundesgesundheitsblatt Gesundheitsforschung Gesundheitsschutz, 65(4):512-524, 01 Apr 2022
Cited by: 1 article | PMID: 35119479 | PMCID: PMC8814801
Impact of different pathogen reduction technologies on the biochemistry, function, and clinical effectiveness of platelet concentrates: An updated view during a pandemic.
Transfusion, 62(1):227-246, 06 Dec 2021
Cited by: 13 articles | PMID: 34870335 | PMCID: PMC9300014
Review Free full text in Europe PMC
Similar Articles
To arrive at the top five similar articles we use a word-weighted algorithm to compare words from the Title and Abstract of each citation.
Robust inactivation of Plasmodium falciparum in red blood cell concentrates using amustaline and glutathione pathogen reduction.
Transfusion, 62(5):1073-1083, 06 Apr 2022
Cited by: 3 articles | PMID: 35385146 | PMCID: PMC9325390
Red blood cells treated with the amustaline (S-303) pathogen reduction system: a transfusion study in cardiac surgery.
Transfusion, 58(4):905-916, 01 Mar 2018
Cited by: 12 articles | PMID: 29498049
Pathogen inactivation of Dengue virus in red blood cells using amustaline and glutathione.
Transfusion, 57(12):2888-2896, 16 Sep 2017
Cited by: 9 articles | PMID: 28921542
Towards pathogen inactivation of red blood cells and whole blood targeting viral DNA/RNA: design, technologies, and future prospects for developing countries.
Blood Transfus, 15(6):512-521, 13 Apr 2017
Cited by: 22 articles | PMID: 28488960 | PMCID: PMC5649960
Review Free full text in Europe PMC
Funding
Funders who supported this work.