Abstract
Free full text

New MOF/COF Hybrid as a Robust Adsorbent for Simultaneous Removal of Auramine O and Rhodamine B Dyes
Abstract
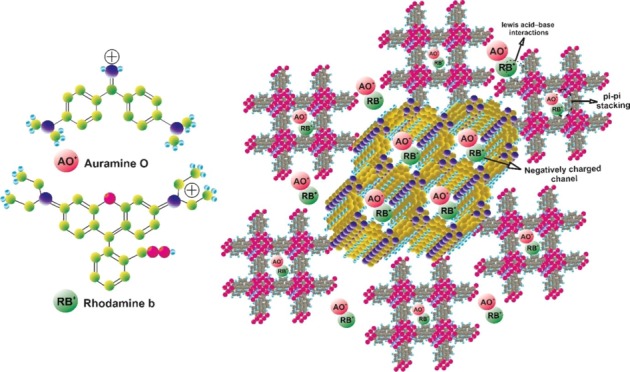
In this study, by hybridization of zinc-based metal–organic framework-5 (MOF-5) and melamine-terephthaldehyde-based intergrade two-dimensional π-conjugated covalent organic framework (COF), a novel MOF-5/COF (M5C) hybrid material was prepared and characterized by Fourier transform infrared, field emission scanning electron microscopy, X-ray diffraction, and thermogravimetric analysis. MOF-5 has a well-defined cubic structure, and the proposed COF has an orderly and spherical nanosize shape. The prepared MOF-5/COF was applied as an effective adsorbent for rapid and high-efficient simultaneous removal of auramine O (AO) and rhodamine B (RB) cationic dyes via electrostatic, H-bonding, Lewis acid–base interactions, and π–π stacking from aqueous solution. The effect of experimental parameters such as pH, M5C mass, contact time, and AO and RB dyes concentration was investigated for removal efficiency and optimized. The M5C adsorbent showed an adsorption capacity of 17.95 and 16.18 mg/g for AO and RB dyes, respectively, at pH 9.5. The adsorption study of AO and RB dyes by M5C comprises both isotherm and kinetic studies. The equilibrium adsorption data followed by Langmuir isotherm and the adsorption kinetic process were found to be a pseudo-second-order model. The robustness adsorption efficiency of MOF/COF hybrids can be attributed to the formation of amide bonds between COF and MOFs, which improve the stability of the adsorbent.
1. Introduction
Recently, because of the rapid development of various industries, large amounts of dyeing wastewater are released into the environment directly without appropriate treatments.1−4 The toxicity of industrial dyes imposes a serious menace to the water perimeter and human health.5 Synthetic dyes from printing and dyeing industries hamper sunlight penetration into water and causes the death of plants and animals, as well as is harmful to humans.6,7 Accordingly, efficient treatment technologies such as photocatalytic oxidation or reduction, membrane filtration, biological treatment, and adsorption have been developed for the removal of organic dyes from wastewater.7−10 Amongst the removal techniques, adsorption is an eco-friendly technology for the removal of dyes from wastewater owing to its simple operation, great efficiency, and economic facility and produces no byproducts.11,12 For the adsorption process, finding a new effective surface adsorbent containing aromatic rings, functional groups, and reactive sites for π–π stacking, H-bonding, and electrostatic interactions with other aromatic-containing compounds with OH, NH, =O, and SH groups as well as surface charges for efficient capture is very important.13,14 A hybrid of two of these compounds is discussed in this study.
New compounds with diverse structures and unique properties are considered for various applications in chemical and material science in recent years. Therefore, metal–organic frameworks (MOFs) and covalent organic frameworks (COFs) have received increasing attention in recent years in various technological and scientific fields owing to the capability to control their pore size, shape, and chemical functionalities.15,16 MOFs consisting of organic linkers for π–π stacking interaction and metal ions for electrostatic interaction are an attractive class of porous crystalline materials because of their outstanding properties such as excellent surface area, adjustable pore sizes, and various functionalities.17,18 MOFs are utilized in various applications while the direct use of MOFs for the adsorption of dyes has limitations such as skimp chemical stability to aqueous and organic and strongly acidic or alkaline media.19−21 We consider that grafting of MOFs with COFs can prevail these limitations impressively which could possess structural features of each component and demonstrate new traits.22,23 COFs are beneficial because of their lower densities and improved chemical and thermal stability and generate hydrogen bonding, electrostatic bonding, and π–π stacking interactions for adsorption of organic dyes.24 They overcame the limitations of chemical instability compared with MOFs because of their excellent porosity, surface area, and adsorption capacities; excellent chemical stability; high thermal stability; low density; ability to transport charge; and highly ordered structures and have drawn remarkable research interest in the adsorption process.25,26 They are produced by the exact assembly of organic molecular building moieties via covalent bonds and commonly comprise nanometer-sized uniform pores.24 Lately, the great advance in COF materials has led to the development of COF materials with potential performance and prolonged their applicability. There are only a few reports available on the hybridization of COFs and MOFs for different applications.
Zhang et al. have successfully produced a type of MOF and COF hybrid, NH2-MIL-68@TPA-COF, with pore structure and crystallinity, and used it as an efficient photocatalyst.23 Lan et al. have constructed a type of MOF/COF hybrid material through covalently anchoring NH2-UiO-66 on the TpPa-1-COF surface. The obtained hierarchical porous hybrid materials display effective photocatalytic H2 evolution.27 Zhao et al. have reported the production of mixed matrix membranes comprising MOF@COF hybrid fillers for effective CO2/CH4 separation.28 Ben et al. have prepared COF–MOF-based membranes and demonstrated that the obtained membranes give desirable separation selectivity of H2/CO2 mixtures than the exclusive COF and MOF membranes.29 Xia et al. have reported electrocatalysts based on MOF/COF for water splitting.30 Therefore, in this work, a type of MOF/COF hybrid with the potential of high π–π stacking, H-bonding, and electrostatic interactions was hydrothermally synthesized and applied for the simultaneous removal of auramine O (AO) and rhodamine B (RB) cationic dyes from aqueous media under sonication conditions. While detection of color and other organic and drug materials in effluents is difficult, many scientists and researchers have focused on designing and fabrication of electroanalytical kits and sensors with good selectivity and high sensitivity for different types of these compounds.2,31−33 The effects of various utilizable parameters, such as pH, M5C mass, initial AO and RB concentration, and process time on removal percentage, were checked and optimized. The adsorption isotherms, kinetics, and mechanism were also studied.
2. Experimental Section
2.1. Materials and Apparatus
All reagents including ethanol, 1,4-benzendicarboxylic acid (BDC, terephthalic acid), zinc acetate dihydrate (Zn(CH3CO2)2·2H2O), ethylenediamine, N,N-dimethylformamide (DMF), melamine, terephthaldehyde, dimethyl sulfoxide (DMSO), AO, and RB were purchased from Merck Company (Darmstadt, Germany). Fourier transform infrared (FT-IR) spectra were recorded with a Jasco-680 spectrometer (Japan) in the range of 4000–400 cm–1 by making their pellets in KBr as a medium. The diffraction patterns of related materials were recorded in the reflection mode using a Bruker, D8 ADVANCE diffractometer. Nickel-filtered Cu Kα radiation (radiation wavelength, λ = 0.154 nm) was produced at an operating voltage of 45 kV and a current of 100 mA. The surface morphology of the resulting materials was investigated using field emission-scanning electron microscopy (FE-SEM; EM10C-ZEISS, 80 kV, Zeiss Co., Germany). Thermogravimetric analysis (TGA) was recorded using a thermogravimetric analyzer TGA-PL-1500 from 30 to 800 °C at a heating rate of 10 °C min–1 under a N2 atmosphere.
2.2. Synthesis of MOF-5
Terephthalic acid (0.5 g) was dissolved in 60 mL of DMF, and subsequently, 2.19 g of Zn(CH3CO2)2·2H2O was added and stirred for 10 min. Then, the mixture was transferred to an autoclave and heated at 100 °C for 12 h. Finally, the resulting solid was collected and washed with ethanol and dried at room temperature.
2.3. Preparation of MOF-5-NH2
A mixture of 0.20 g of MOF-5, 25 mL of ethanol, and 5 mL of ethylenediamine was refluxed for 12 h, and subsequently, the resulting mixture was filtered to yield a white precipitate which was washed with ethanol and dried at 80 °C.
2.4. Preparation of MOF-5/COF (M5C)
MOF-5-NH2 (0.20 g), 0.5 g (3.96 mmol) of melamine, 0.5 g (3.73 mmol) of terephthaldehyde, 25 mL of DMSO, and 5 mL of distilled water were mixed and subsequently, transferred to an autoclave and heated at 180 °C for 12 h. Finally, the resulting solid was collected, washed with ethanol, and dried at room temperature. A schematic illustration of all steps for the synthesis of the MOF/COF hybrid material is shown in Figure Figure11, and the proposed mechanism for the chemical synthesis of M5C is presented in Scheme 1.
2.5. AO and RB Adsorption Method
The adsorption experiment of AO and RB dyes with the help of ultrasound waves by M5C using a batch method was conducted as follows: 0.02 g of M5C was added to 25 mL of AO and RB dyes solution (5 mg/L) at pH 9.5, and then the suspension was dispersed for 1 min at room temperature. Finally, the M5C adsorbent was collected, and the final AO and RB contents were quantified by a UV–vis spectrophotometer. The adsorption capacity (qe, mg/g) and the removal percentage (R %) were calculated according to our previous reports.34,35 The adsorption behaviors in binary solution were investigated by UV–vis spectrophotometry at different M5C masses (Figure Figure22), which shows that M5C merely adsorbs AO and RB. These results further indicate that M5C can serve as a promising superadsorbent material for AO and RB absorption from polluted water. In addition, there was no overlap observed in the UV–vis spectrum of AO and RB, revealing that UV–vis spectrophotometry is a low-cost and an easily available technique for the detection of these compounds.
3. Results and Discussion
3.1. Characterization of the Synthesized Materials
Figure Figure33A shows the FT-IR spectra of MOF-5, MOF-5-NH2, and M5C; the absorption band around 3416 cm–1 in MOF-5 corresponds to O–H stretching vibration of H2O in the MOF. The peaks at 1662 and 1392 cm–1 correspond to the stretching encryptions of the O=C–O bonded to Zn. The absorption band at 532 cm–1 is attributed to the stretching vibration of Zn–O. In the functionalized MOF-5 spectrum, the new absorption peaks at 3324 and 3270 cm–1 are attributed to the stretching vibrations of NH2 groups, and the absorption bands appeared at 2958, 2904, and 2873 cm–1 correspond to the vibration of C–H bonds of ethylenediamine. From the spectral data, it can be deduced that ethylenediamine has been grafted on the MOF-5 surface. The FTIR spectrum of the M5C hybrid displays a series of new characteristic stretching vibrations at 1547, 1465, and 1338 arising from the C=N, C=C, and C–N bonds, respectively, showing condensation reaction and tautomerization.
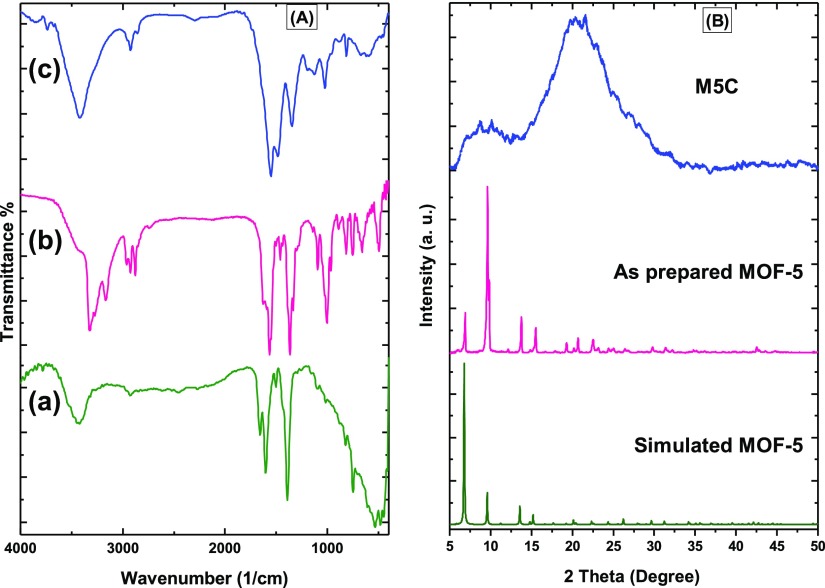
FT-IR spectra (A) of MOF-5 (a), MOF-5-NH2 (b) and M5C (c) and XRD patterns of as simulated, as prepared MOF-5 and MOF-5-COF (B).
Figure Figure33B shows the X-ray diffraction (XRD) patterns of simulated and as-prepared MOF-5 and M5C that exhibit a crystal structure of as-prepared MOF-5, which matches its simulated pattern published previously. The XRD pattern of M5C shows two broad peaks at 2θ of 8 and 20, which is probably related to the overlap of XRD peaks of MOF-5 and M5C, and the corresponding COF and MOF peaks were well-observed with short tentacles and wide spikes.
Figure Figure44a shows FE-SEM images of MOF-5 with well-defined cubic crystals of 2.5 μm in width. The FE-SEM investigation of M5C confirms that the smooth pure MOF-5 structure significantly changes due to the loading of the orderly and spherical shape of the COF on the MOF-5 surface (Figure Figure44b). The FE-SEM images of the resulting M5C hybrid show that the COF is well-dispersed on the MOF-5 surface. The M5C hybrid surface tends to be rougher after the insertion of the COF on MOF-5 and had a fatal effect on the MOF-5 support structure.
Figure Figure55a presents the weight loss of M5C with the increase in temperature, and the observations are explained as follows: weight loss was observed between 50 and 150 °C, which could be ascribed to the loss in moisture and solvent molecules. No obvious mass loss from 150 to 420 °C demonstrates the superior thermodynamic stability of M5C up to 400 °C. Further, the increase in temperature to 520 °C leads to weight loss due to the destruction of MOF and COF structures.
The N2 adsorption–desorption isotherms of the as-obtained M5C (Figure Figure55b) revealed a slight capillary condensation step at high relative pressure, which belongs to type IV isotherm according to IUPAC classification, and the specific surface area BET was found to be 7.025 m2/g.
3.2. Effect of the Operational Parameter on AO and RB Removal
The solution pH as a significant factor can affect the adsorption capacity owing to its effect on the surface properties as well as ionization or dissociation of the adsorbents. To evaluate the pH effect on the simultaneous adsorption of AO and RB, pH values were varied between 2.0 and 9.5 (Figure Figure66a). The pHzpc of M5C hybrid was found to be 6.0, which shows that below the pHzpc value, the M5C surface has a positive charge and above the pHzpc value, the M5C surface possesses a negatively charged surface. Hence, the M5C hybrid possesses high adsorption capacity at pH 9.5 due to the electrostatic interaction between M5C negative surface charge and cationic AO and RB dyes. When pH > 7, the M5C adsorption capacity tends to be constant due to the π–π stacking and electrostatic interaction of aromatic rings of AO and RB and electrostatic charges of M5C, which are stable in weak alkali media. In addition, at low pH, low electrostatic attraction exists between the adsorbent M5C surface and AO and RB because of the presence of positively charged sites on both the AO and RB and M5C surfaces and the lack of free unpaired electrons on the adsorbent surface that hinders increased adsorption; hence acidic pH values do not favor the proposed adsorption system. As the pH of the system increases, free unpaired electrons become available on the adsorbent surface, which in turn will increase the electrostatic attraction forces between AO and RB and the adsorbent sites resulting in high adsorption capacity of AO and RB at high pH values.
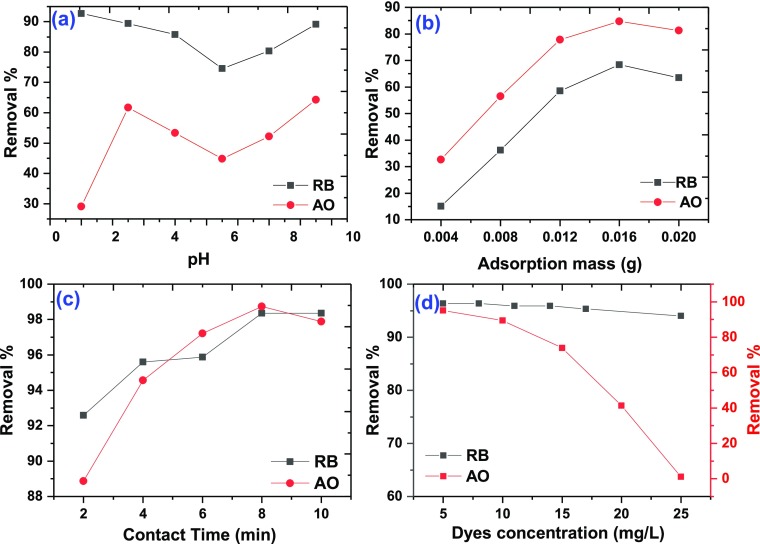
Effect of pH (a), adsorbent mass (b), contact time (c), and initial dye concentration (d) on the adsorption of AO and RB on M5C.
The adsorption of AO and RB on M5C was investigated at various adsorbent doses in the range of 0.004–0.020 g at a dye concentration (5 mg/L) and pH (9.5) at a contact time of 10 min (Figure Figure66b). The removal efficiency was enhanced as the adsorbent dose increased from 0.004 to 0.016 g due to increase in active surface adsorption sites.
The effect of contact time on the removal of AO and RB dyes was studied to evaluate the adsorption rate. The AO and RB removal percentage at pH 9.5 has a synergic and positive relation with contact time (Figure Figure66c). As shown in Figure Figure66c, more than 90% of AO and RB removal occurs in the first 8 min. Subsequently, the adsorption rate has a slight changed trend that is ascribed to diffusion rate diminution and concentration gradient. The rate of dye adsorption by M5C was observed to be very rapid for 8 min, which indicates the efficiency and high ability of M5C in the removal of AO and RB dyes. Afterward, the dye adsorption processes proceeded at a slower rate. The slow rate of dye adsorption for a long time may result from the saturation and coverage of M5C with the dyes.
The effect of the initial AO and RB concentrations and their removal efficiency were assessed by varying the AO and RB concentrations from 5 to 25 mg/L (Figure Figure66d). The obtained results show that the adsorption capacity of AO and RB by the M5C hybrid is a function of the initial AO and RB concentrations and the adsorption percent decreases with the increase in the initial AO and RB dyes concentration. At lower AO and RB concentrations, the ratio of the number of active sites of M5C to the available reactive sites of AO and RB was detracted. In fact, at lower AO and RB concentrations, M5C has more chance to react with the reactive sites of AO and RB and thus, the adsorption capacity is augmented.
For selectivity investigation, some anionic dyes such as fast green FCF, eosin yellow, and quinine yellow were tested, and M5C was able to eliminate 20, 23.5, and 31.2% of the dyes, respectively. Therefore, it can be said that the proposed adsorbent effectively eliminates cationic dyes and repels anionic dyes. In addition, methylene blue and malachite green as cationic dyes were tested in which M5C was able to eliminate a large percentage of the dyes, while due to the spectral overlap of these dyes with colors of AO and RB, they were excluded from simultaneous removal.
3.3. Adsorption Isotherms
The correlation between the adsorbent amount and the retained material in bulk at a constant temperature is significant in MOF- and COF-based adsorption systems. To optimize the conditions of the adsorption of AO and RB, it is important to prescribe the most suitable correlations of the equilibrium data. The most extensively utilized surface adsorption models are the Langmuir, Temkin, Freundlich, and Dubinin–Radushkevich (D–R) isotherms, which are the best-fitting isotherms found by linear regressions as well as the applicability of isotherms are compared by recognizing the correlation coefficients and error analysis. The resulting correlation coefficients provided strong evidence that AO and RB adsorption onto M5C follows the Langmuir isotherm (Table 1). The applicability of the Langmuir model was corroborated by the high correlation coefficients R2 > 0.984. The Qm values obtained from the Langmuir isotherms were 17.95 and 16.18 mg/g for AO and RB, respectively. The R2 values for other models are not fit of equilibrium data as compared with the Langmuir isotherm model.
Table 1
value | |||
---|---|---|---|
isotherm models | parameters | AO | RB |
Langmuir: Ce/qe = 1/KaQm + Ce/Qm | Qm (mg g–1) | 17.95 | 16.18 |
Ka (L mg–1) | 2.34 | 3.33 | |
R2 | 0.9848 | 0.9882 | |
Freundlich: ln qe = ln Kf + (1/n)ln Ce | 1/n | 0.8689 | 0.1396 |
Kf (L mg–1) | 3.092 | 1.909 | |
R2 | 0.8938 | 0.3748 | |
Temkin: qe = Bl ln KT + Bl ln Ce | BT | 4.9542 | 0.5843 |
KT (L mg–1) | 2.49 | 307.07 | |
R2 | 0.9477 | 0.8278 | |
Dubinin–Radushkevich: ln qe = ln Qs – Kε2 | Qs (mg g–1) | 12.69 | 5.92 |
K | 6 × 10–8 | 4 × 10–8 | |
R2 | 0.9477 | 0.8278 |
3.4. Kinetics Models
To examine the adsorption kinetics, pseudo-first-order, pseudo-second-order, Elovich, and intraparticle diffusion models were studied, and the experimental and calculated qe values have good correlation and agreement at different initial AO and RB concentrations and adsorbent mass for the pseudo-second-order model (Table 2).
Table 2
value | |||
---|---|---|---|
kinetic model | parameters | AO | RB |
first-order: log(qe – qt) = log(qe) – (K1/2.303)t | K1 | 0.2975 | 0.3030 |
qe (cal.) | 0.044 | 2.225 | |
R2 | 0.5352 | 0.9196 | |
second-order: t/qt = 1/k2qe2 + (1/qe)t | K2 | 1.160 | 1.242 |
qe (cal.) | 3.13 | 3.15 | |
R2 | 0.9900 | 0.9997 | |
intraparticle diffusion: qt = Kidt1/2 + C | Kid | 0.1046 | 0.1680 |
C | 2.75 | 2.58 | |
R2 | 0.9319 | 0.8572 | |
Elovich: qt = 1/β ln(αβ) + 1/β ln(t) | β | 8.76 | 5.29 |
α | 1.40 | 2.26 | |
R2 | 0.9458 | 0.9258 | |
experimental adsorption capacity | qe(exp) | 0.134 | 2.125 |
3.5. Adsorption Mechanism
The plausible interaction of M5C with AO and RB (Figure Figure77) is controlled by various factors like the AO and RB molecules structure and functional behavior and the M5C surface characteristics. The AO and RB molecules are planar and can be conveniently adsorbed on the M5C via physical interactions in addition to M5C cavities. The cavities of M5C could encapsulate all the AO and RB organic dyes. AO and RB can bind to M5C via electrostatic and van der Waals forces and hydrogen bonding and host–guest interactions with the M5C cavities.
3.6. Regeneration and Reusability of M5C
To examine the reusability of M5C, the adsorption experiments were carried out, and subsequently, M5C was collected by a centrifuge, and the adsorbed AO and RB were washed with methanol for 6 cycles in which the removal percentage only slightly reduced (Figure Figure88). This supports the adsorption process and offers the possibility to reuse chemically stable M5C for multiple cycles, which indicates that M5C is a promising and robust adsorbent for the removal of AO and RB dye pollutants.
3.7. Comparison with the Previously Reported Adsorbents
A comparison study of adsorption capacity, contact time, pH, and adsorbent mass for different methods was performed, and the results are reported in Table 3. Considering the literature, we have reached satisfactory results in particular in the simultaneous adsorption of AO and RB.
Table 3
adsorbent | dye | pH | adsorbent mass (mg) | contact time (min) | qm (mg/g) | refs |
---|---|---|---|---|---|---|
humic acid-modifying Fe3O4 NPs | RB | 2.53 | 100 | 30 | 161.8 | (36) |
Sistan sand | RB | 6.0 | 1000 | 30 | 0.11 | (37) |
3A zeolite | RB | 9.0 | 1000 | 40 | 0.74 | (38) |
carboxylated cellulose derivative | AO | 6.0 | 70 g//L | 24 h | 5.443 | (39) |
MOF-5-Ag2O-NPs | AO | 6.0 | 25 | 50 s | 260.70 | (40) |
ZnS:Cu-NP-AC | AO | 7.0 | 40 | 2.2 | 94.2 | (41) |
Fe3O4/C–COP | AO | 6.5 | 12 | 4 | 107.11 | (42) |
Fe3O4/C–COP | RB | 6.5 | 12 | 4 | 131.23 | (42) |
M5C | AO | 9.5 | 16 | 8 | 17.95 | this work |
M5C | RB | 9.5 | 16 | 8 | 16.18 | this work |
4. Conclusions
As a novel shell structure adsorbent, M5C has electrostatic, hydrogen bonding, Lewis acid–base, and π–π stacking interactions for AO and RB molecule adsorption, which enables rapid and efficient removal of AO and RB; therefore, M5C has a good potential in dyeing wastewater treatment. The optimum conditions for AO and RB dye adsorption were found to be the initial AO and RB concentration of 5 ppm, 0.016 g of M5C, and pH = 9.5. The linear forms of Langmuir and pseudo-second-order models were the best isotherm and kinetic model, respectively. Finally, water treatment agents with MOF and COF hybrids are envisaged to become a new orientation in the development of wastewater treatment engineering.
References
- An Y.; Zheng H.; Yu Z.; Sun Y.; Wang Y.; Zhao C.; Ding W. Functioned hollow glass microsphere as a self-floating adsorbent: Rapid and high-efficient removal of anionic dye. J. Hazard. Mater. 2020, 381, 120971.10.1016/j.jhazmat.2019.120971. [Abstract] [CrossRef] [Google Scholar]
- Karimi-Maleh H.; Shafieizadeh M.; Taher M. A.; Opoku F.; Kiarii E. M.; Govender P. P.; Ranjbari S.; Rezapour M.; Orooji Y. The role of magnetite/graphene oxide nano-composite as a high-efficiency adsorbent for removal of phenazopyridine residues from water samples, an experimental/theoretical investigation. J. Mol. Liq. 2020, 298, 112040.10.1016/j.molliq.2019.112040. [CrossRef] [Google Scholar]
- Lyu F.; Zhang Y.; Zare R. N.; Ge J.; Liu Z. One-pot synthesis of protein-embedded metal-organic frameworks with enhanced biological activities. Nano Lett. 2014, 14, 5761–5765. 10.1021/nl5026419. [Abstract] [CrossRef] [Google Scholar]
- Nourozi S.; Zare-Dorabei R. Highly efficient ultrasonic-assisted removal of methylene blue from aqueous media by magnetic mesoporous silica: experimental design methodology, kinetic and equilibrium studies. Desalin. Water Treat. 2017, 85, 184–196. 10.5004/dwt.2017.21207. [CrossRef] [Google Scholar]
- Pourebrahim F.; Ghaedi M.; Dashtian K.; Kheirandish S.; Goudarzi A. Optimization of solid phase dispersive field-assisted ultrasonication for the extraction of auramine O and crystal violet dyes using central composite design. Appl. Organomet. Chem. 2018, 32, e418110.1002/aoc.4181. [CrossRef] [Google Scholar]
- Kaykhaii M.; Sasani M.; Marghzari S. Removal of dyes from the environment by adsorption process. Chem. Mater. Eng. 2018, 6, 31–35. 10.13189/cme.2018.060201. [CrossRef] [Google Scholar]
- Cui K.; Yan B.; Xie Y.; Qian H.; Wang X.; Huang Q.; He Y.; Jin S.; Zeng H. Regenerable urchin-like Fe3O4@PDA-Ag hollow microspheres as catalyst and adsorbent for enhanced removal of organic dyes. J. Hazard. Mater. 2018, 350, 66–75. 10.1016/j.jhazmat.2018.02.011. [Abstract] [CrossRef] [Google Scholar]
- Wu Y.; Yang F.; Liu X.; Tan G.; Xiao D. Fabrication of N, P-codoped reduced graphene oxide and its application for organic dye removal. Appl. Surf. Sci. 2018, 435, 281–289. 10.1016/j.apsusc.2017.10.118. [CrossRef] [Google Scholar]
- Ramezani F.; Zare-Dorabei R. Simultaneous ultrasonic-assisted removal of malachite green and methylene blue from aqueous solution by Zr-SBA-15. Polyhedron 2019, 166, 153–161. 10.1016/j.poly.2019.03.033. [CrossRef] [Google Scholar]
- Zare E.; Rafiee Z. Cellulose stabilized Fe3O4 and carboxylate-imidazole and Co-based MOF growth as an exceptional catalyst for the Knoevenagel reaction. Appl. Organomet. Chem. 2020, 34, e551610.1002/aoc.5516. [CrossRef] [Google Scholar]
- Jiang D.; Chen M.; Wang H.; Zeng G.; Huang D.; Cheng M.; Liu Y.; Xue W.; Wang Z. The application of different typological and structural MOFs-based materials for the dyes adsorption. Coord. Chem. Rev. 2019, 380, 471–483. 10.1016/j.ccr.2018.11.002. [CrossRef] [Google Scholar]
- Natarajan S.; Bajaj H. C.; Tayade R. J. Recent advances based on the synergetic effect of adsorption for removal of dyes from waste water using photocatalytic process. J. Environ. Sci. 2018, 65, 201–222. 10.1016/j.jes.2017.03.011. [Abstract] [CrossRef] [Google Scholar]
- Zhang W.; Zhang R.-Z.; Huang Y.-Q.; Yang J.-M. Effect of the synergetic interplay between the electrostatic interactions, size of the dye molecules, and adsorption sites of MIL-101 (Cr) on the adsorption of organic dyes from aqueous solutions. Cryst. Growth Des. 2018, 18, 7533–7540. 10.1021/acs.cgd.8b01340. [CrossRef] [Google Scholar]
- Molla A.; Li Y.; Mandal B.; Kang S. G.; Hur S. H.; Chung J. S. Selective adsorption of organic dyes on graphene oxide: Theoretical and experimental analysis. Appl. Surf. Sci. 2019, 464, 170–177. 10.1016/j.apsusc.2018.09.056. [CrossRef] [Google Scholar]
- Zhou H.-C.; Long J. R.; Yaghi O. M.. Introduction to Metal–Organic Frameworks; ACS Publications, 2012. [Abstract] [Google Scholar]
- Kandambeth S.; Dey K.; Banerjee R. Covalent organic frameworks: chemistry beyond the structure. J. Am. Chem. Soc. 2018, 141, 1807–1822. 10.1021/jacs.8b10334. [Abstract] [CrossRef] [Google Scholar]
- Askari H.; Ghaedi M.; Dashtian K.; Azghandi M. H. A. Rapid and high-capacity ultrasonic assisted adsorption of ternary toxic anionic dyes onto MOF-5-activated carbon: artificial neural networks, partial least squares, desirability function and isotherm and kinetic study. Ultrason. Sonochem. 2017, 37, 71–82. 10.1016/j.ultsonch.2016.10.029. [Abstract] [CrossRef] [Google Scholar]
- Yousefian M.; Rafiee Z. Cu-metal-organic framework supported on chitosan for efficient condensation of aromatic aldehydes and malononitrile. Carbohydr. Polym. 2020, 228, 115393.10.1016/j.carbpol.2019.115393. [Abstract] [CrossRef] [Google Scholar]
- Chen L.; Luque R.; Li Y. Encapsulation of metal nanostructures into metal-organic frameworks. Dalton Trans. 2018, 47, 3663–3668. 10.1039/c8dt00092a. [Abstract] [CrossRef] [Google Scholar]
- DeCoste J. B.; Peterson G. W.; Jasuja H.; Glover T. G.; Huang Y.-g.; Walton K. S. Stability and degradation mechanisms of metal-organic frameworks containing the Zr6O4(OH)4 secondary building unit. J. Mater. Chem. A 2013, 1, 5642–5650. 10.1039/c3ta10662d. [CrossRef] [Google Scholar]
- Yuan S.; Feng L.; Wang K.; Pang J.; Bosch M.; Lollar C.; Sun Y.; Qin J.; Yang X.; Zhang P.; Wang Q.; Zou L.; Zhang Y.; Zhang L.; Fang Y.; Li J.; Zhou H.-C. Stable metal-organic frameworks: design, synthesis, and applications. Adv. Mater. 2018, 30, 1704303.10.1002/adma.201704303. [Abstract] [CrossRef] [Google Scholar]
- Pastore V. J.; Cook T. R.; Rzayev J. Polymer-MOF hybrid composites with high porosity and stability through surface-selective ligand exchange. Chem. Mater. 2018, 30, 8639–8649. 10.1021/acs.chemmater.8b03881. [CrossRef] [Google Scholar]
- Peng Y.; Zhao M.; Chen B.; Zhang Z.; Huang Y.; Dai F.; Lai Z.; Cui X.; Tan C.; Zhang H. Hybridization of MOFs and COFs: A New Strategy for Construction of MOF@COF Core-Shell Hybrid Materials. Adv. Mater. 2018, 30, 1705454.10.1002/adma.201705454. [Abstract] [CrossRef] [Google Scholar]
- Waller P. J.; Gándara F.; Yaghi O. M. Chemistry of covalent organic frameworks. Acc. Chem. Res. 2015, 48, 3053–3063. 10.1021/acs.accounts.5b00369. [Abstract] [CrossRef] [Google Scholar]
- Kang Z.; Peng Y.; Qian Y.; Yuan D.; Addicoat M. A.; Heine T.; Hu Z.; Tee L.; Guo Z.; Zhao D. Mixed matrix membranes (MMMs) comprising exfoliated 2D covalent organic frameworks (COFs) for efficient CO2 separation. Chem. Mater. 2016, 28, 1277–1285. 10.1021/acs.chemmater.5b02902. [CrossRef] [Google Scholar]
- Zhi Y.; Shao P.; Feng X.; Xia H.; Zhang Y.; Shi Z.; Mu Y.; Liu X. Covalent organic frameworks: efficient, metal-free, heterogeneous organocatalysts for chemical fixation of CO2 under mild conditions. J. Mater. Chem. A 2018, 6, 374–382. 10.1039/c7ta08629f. [CrossRef] [Google Scholar]
- Zhang F.-M.; Sheng J.-L.; Yang Z.-D.; Sun X.-J.; Tang H.-L.; Lu M.; Dong H.; Shen F.-C.; Liu J.; Lan Y.-Q. Rational design of MOF/COF hybrid materials for photocatalytic H2 evolution in the presence of sacrificial electron donors. Angew. Chem., Int. Ed. 2018, 57, 12106–12110. 10.1002/anie.201806862. [Abstract] [CrossRef] [Google Scholar]
- Cheng Y.; Ying Y.; Zhai L.; Liu G.; Dong J.; Wang Y.; Christopher M. P.; Long S.; Wang Y.; Zhao D. Mixed matrix membranes containing MOF@COF hybrid fillers for efficient CO2/CH4 separation. J. Membr. Sci. 2019, 573, 97–106. 10.1016/j.memsci.2018.11.060. [CrossRef] [Google Scholar]
- Fu J.; Das S.; Xing G.; Ben T.; Valtchev V.; Qiu S. Fabrication of COF-MOF composite membranes and their highly selective separation of H2/CO2. J. Am. Chem. Soc. 2016, 138, 7673–7680. 10.1021/jacs.6b03348. [Abstract] [CrossRef] [Google Scholar]
- Yan Y.; He T.; Zhao B.; Qi K.; Liu H.; Xia B. Y. Metal/covalent-organic frameworks-based electrocatalysts for water splitting. J. Mater. Chem. A 2018, 6, 15905.10.1039/c8ta05985c. [CrossRef] [Google Scholar]
- Bijad M.; Karimi-Maleh H.; Farsi M.; Shahidi S.-A. An electrochemical-amplified-platform based on the nanostructure voltammetric sensor for the determination of carmoisine in the presence of tartrazine in dried fruit and soft drink samples. J. Food Meas. Char. 2018, 12, 634–640. 10.1007/s11694-017-9676-1. [CrossRef] [Google Scholar]
- Tahernejad-Javazmi F.; Shabani-Nooshabadi M.; Karimi-Maleh H. 3D reduced graphene oxide/FeNi3-ionic liquid nanocomposite modified sensor; an electrical synergic effect for development of tert-butylhydroquinone and folic acid sensor. Composites, Part B 2019, 172, 666–670. 10.1016/j.compositesb.2019.05.065. [CrossRef] [Google Scholar]
- Zabihpour T.; Shahidi S.-A.; Karimi-Maleh H.; Ghorbani-HasanSaraei A. Voltammetric food analytical sensor for determining vanillin based on amplified NiFe2O4 nanoparticle/ionic liquid sensor. J Food Meas. Char. 2020, 14, 1039.10.1007/s11694-019-00353-8. [CrossRef] [Google Scholar]
- Dashtian K.; Porhemat S.; Rezvani A. R.; Ghaedi M.; Sabzehmeidani M. M. Adsorption of semisoft pollutants onto Bi2S3/Ag2S-AC under the influence of ultrasonic waves as external filed. J. Ind. Eng. Chem. 2018, 60, 390–400. 10.1016/j.jiec.2017.11.026. [CrossRef] [Google Scholar]
- Ghaedi M.; Dashtian K.; Ghaedi A. M.; Dehghanian N. A hybrid model of support vector regression with genetic algorithm for forecasting adsorption of malachite green onto multi-walled carbon nanotubes: central composite design optimization. Phys. Chem. Chem. Phys. 2016, 18, 13310–13321. 10.1039/c6cp01531j. [Abstract] [CrossRef] [Google Scholar]
- Peng L.; Qin P.; Lei M.; Zeng Q.; Song H.; Yang J.; Shao J.; Liao B.; Gu J. Modifying Fe3O4 nanoparticles with humic acid for removal of Rhodamine B in water. J. Hazard. Mater. 2012, 209–210, 193–198. 10.1016/j.jhazmat.2012.01.011. [Abstract] [CrossRef] [Google Scholar]
- Marghzari S.; Sasani M.; Kaykhaii M.; Sargazi M.; Hashemi M. Simultaneous elimination of Malachite Green, Rhodamine B and Cresol Red from aqueous sample with Sistan sand, optimized by Taguchi L16 and Plackett-Burman experiment design methods. Chem. Cent. J. 2018, 12, 116.10.1186/s13065-018-0486-2. [Europe PMC free article] [Abstract] [CrossRef] [Google Scholar]
- Rahmani M.; Kaykhaii M.; Sasani M. Application of Taguchi L16 design method for comparative study of ability of 3A zeolite in removal of Rhodamine B and Malachite green from environmental water samples. Spectrochim. Acta, Part A 2018, 188, 164–169. 10.1016/j.saa.2017.06.070. [Abstract] [CrossRef] [Google Scholar]
- Teodoro F. S.; Elias M. M. C.; Ferreira G. M. D.; Adarme O. F. H.; Savedra R. M. L.; Siqueira M. F.; da Silva L. H. M.; Gil L. F.; Gurgel L. V. A. Synthesis and application of a new carboxylated cellulose derivative. Part III: Removal of auramine-O and safranin-T from mono-and bi-component spiked aqueous solutions. J. Colloid Interface Sci. 2018, 512, 575–590. 10.1016/j.jcis.2017.10.083. [Abstract] [CrossRef] [Google Scholar]
- Hosseinpour S. A.; Karimipour G.; Ghaedi M.; Dashtian K. Use of metal composite MOF-5-Ag2O-NPs as an adsorbent for the removal of Auramine O dye under ultrasound energy conditions. Appl. Organomet. Chem. 2018, 32, e400710.1002/aoc.4007. [CrossRef] [Google Scholar]
- Asfaram A.; Ghaedi M.; Hajati S.; Rezaeinejad M.; Goudarzi A.; Purkait M. K. Rapid removal of Auramine-O and Methylene blue by ZnS: Cu nanoparticles loaded on activated carbon: a response surface methodology approach. J. Taiwan Inst. Chem. Eng. 2015, 53, 80–91. 10.1016/j.jtice.2015.02.026. [CrossRef] [Google Scholar]
- Shakeri S.; Rafiee Z.; Dashtian K. Fe3O4-based melamine-rich covalent organic polymer for simultaneous removal of auramine O and rhodamine B. J. Chem. Eng. Data 2020, 65, 696.10.1021/acs.jced.9b00945. [CrossRef] [Google Scholar]
Articles from ACS Omega are provided here courtesy of American Chemical Society
Full text links
Read article at publisher's site: https://doi.org/10.1021/acsomega.0c00539
Read article for free, from open access legal sources, via Unpaywall:
https://pubs.acs.org/doi/pdf/10.1021/acsomega.0c00539
Citations & impact
Impact metrics
Citations of article over time
Article citations
Bimetallic metal-organic frameworks (BMOFs) for dye removal: a review.
RSC Adv, 14(43):31777-31796, 08 Oct 2024
Cited by: 0 articles | PMID: 39380644 | PMCID: PMC11459228
Review Free full text in Europe PMC
Adsorbent based on MOF-5/cellulose aerogel composite for adsorption of organic dyes from wastewater.
Sci Rep, 14(1):15623, 07 Jul 2024
Cited by: 1 article | PMID: 38972892 | PMCID: PMC11228018
Functional MOF-Based Materials for Environmental and Biomedical Applications: A Critical Review.
Nanomaterials (Basel), 13(15):2224, 31 Jul 2023
Cited by: 9 articles | PMID: 37570542 | PMCID: PMC10421186
Review Free full text in Europe PMC
Biochar Derived from Chinese Herb Medicine Residues for Rhodamine B Dye Adsorption.
ACS Omega, 8(5):4813-4825, 27 Jan 2023
Cited by: 6 articles | PMID: 36777604 | PMCID: PMC9909799
MOF/COF hybrids as next generation materials for energy and biomedical applications.
CrystEngComm, 24(42):7360-7371, 14 Oct 2022
Cited by: 2 articles | PMID: 36353708 | PMCID: PMC9620950
Review Free full text in Europe PMC
Go to all (9) article citations
Data
Data behind the article
This data has been text mined from the article, or deposited into data resources.
BioStudies: supplemental material and supporting data
Similar Articles
To arrive at the top five similar articles we use a word-weighted algorithm to compare words from the Title and Abstract of each citation.
[Spherical amino-functionalized covalent organic frameworks: Synthesis and adsorption performance toward perfluorinated compounds].
Se Pu, 41(6):472-481, 01 Jun 2023
Cited by: 2 articles | PMID: 37259871 | PMCID: PMC10245219
When covalent organic frameworks meet zeolites: Enhancing rhodamine B removal through the synergy in the emerging organic-inorganic nanoadsorbents.
Environ Pollut, 355:124191, 21 May 2024
Cited by: 0 articles | PMID: 38782164
Green and facile synthesis of cobalt-based metal-organic frameworks for the efficient removal of Congo red from aqueous solution.
J Colloid Interface Sci, 578:500-509, 03 Jun 2020
Cited by: 4 articles | PMID: 32535431
Interfacial chemistries in metal-organic framework (MOF)/covalent-organic framework (COF) hybrids.
Nanoscale, 15(32):13187-13201, 17 Aug 2023
Cited by: 0 articles | PMID: 37539693
Review
Funding
Funders who supported this work.