Abstract
Free full text

Growth differentiation factor-15 slows the growth of murine prostate cancer by stimulating tumor immunity
Abstract
Growth Differentiation Factor-15 (GDF15) is a divergent TGF-beta superfamily cytokine that is overexpressed by most cancers and is induced by anticancer therapy. Transgenic and induced animal models suggest that it protects from cancer development but the mechanisms are uncertain. We investigated the role of immunity in GDF15 induced reduction in prostate cancer (PCa) growth. The C57BL/6 transgenic TRAMP prostate cancer prone mice were bred with mice that were immunodeficient and/or systemically overexpressed GDF15. We developed a novel orthotopic TRAMP PCa model in which primary TRAMP tumor cells were implanted into prostates of mice to reduce the study time. These mice were administered recombinant mouse GDF15, antibody to CD8, PD1 or their respective controls. We found that GDF15 induced protection from tumor growth was reversed by lack of adaptive immunity. Flow cytometric evaluation of lymphocytes within these orthotopic tumors showed that GDF15 overexpression was associated with increased CD8 T cell numbers and an increased number and proportion of recently activated CD8+CD11c+ T cells and a reduced proportion of "exhausted" CD8+PD1+ T cells. Further, depletion of CD8 T cells in tumor bearing mice abolished the GDF15 induced protection from tumor growth. Infusion of GDF15 into mice bearing orthotopic TRAMP tumor, substantially reduced tumor growth that was further reduced by concurrent PD1 antibody administration. GDF15 overexpression or recombinant protein protects from TRAMP tumor growth by modulating CD8 T cell mediated antitumor immunity and augments the positive effects of anti-PD1 blockers.
Introduction
Growth Differentiation Factor-15 (GDF15) is a divergent TGF-beta superfamily cytokine [1], most closely linked to the glial derived neurotrophic factor family because its recently identified receptor Glial-derived neurotrophic factor receptor α-like (GFRAL) is an orphan member of the Glial-derived neurotrophic factor receptor α (GFRa) family [2–5]. GFRAL and other members of the GFRα family signal through the Ret tyrosine kinase co-receptor, which can propagate signals through many different pathways. GDF15 does not utilize the classical Transforming growth factor-b receptor I (TBRI) and II (TBRII) and does not signal through the canonical smad signaling pathway family [2, 3, 5].
GDF15 is a stress response cytokine whose expression and serum levels may increase with injury, inflammation and malignancy and whose biology has been recently extensively reviewed elsewhere [6]. In normal humans, GDF15 circulates at a concentration of about 200–1200 pg/ml but these levels rise in some diseases. GDF15 is overexpressed by the majority of cancers and its serum levels rise broadly in line with stage and extent of disease [6]. Expression can also be increased by anti-cancer therapies such as radiotherapy and chemotherapy [7–11]. Especially in advanced cancers, serum levels can rise markedly by up to 10–100 fold leading to anorexia and loss of lean and fat mass and the development of an anorexia/cachexia syndrome [12, 13]. This is mediated largely through systemic GDF15 induced modulation of central appetite regulatory circuits [12, 14].
There is considerable epidemiological data linking GDF15 to cancers [6]. A polymorphism (H6D) in the GDF15 gene manifests as a non-conservative amino acid change in the sequence of the mature domain of GDF15 and alters the risk and behavior of colorectal and prostate cancers [15–18]. Serum levels of GDF15 progressively rise with the evolution of colonic polyps to colorectal cancer [16]. These increased levels predict a worse outcome in patients with colorectal carcinoma [16, 19] and many other cancers [6]. However, these may not always be a good reflection of local availability of GDF15 within the tumor. Especially in cancer, GDF15 is sometimes secreted in an unprocessed form with its propeptide still attached [20, 21]. As the propeptide contains a heparan sulphate binding motif, this form of GDF15 can bind to extracellular matrix and thus remain localized to the tumor [20], from where it might be slowly released to provide a local pool of GDF15. This tumor localized GDF15 may be important because prostate tumors from patients with early cancer and with increased staining for tumor associated GDF15 have a significantly better prognosis [20].
The role of GDF15 in the biology of cancer has been studied using a number of different approaches, which have yielded somewhat contradictory results. In vitro studies are difficult to interpret as at least one major commercial supplier of GDF15 has sold a product known to be contaminated by TGF-beta and this is the likely cause of studies erroneously demonstrating GDF15 induced smad signaling [22–24]. In vivo studies using transfected tumor cell lines which are xenografted into immunodeficient mice have suggested, overall, that GDF15 may facilitate tumor growth and spread [6].
Transgenic and induced cancer models more closely reflect the progressive molecular changes of carcinogenesis, more often mimic early cancer and use rodents with intact immune systems. GDF15 overexpressing mice are protected from urethane induced lung cancer [25] and azoxymethane induced colonic cancers [26]. Additionally, utilizing the mutant adenomatous polyposis coli (APC) gene mouse model of colonic polyps and cancer, mice overexpressing GDF15 are protected from the development of polyps and cancer [26]. Apc mutant mice loose NSAID induced protection from the development of colonic polyps if they are germline Gdf15 gene deleted [27]. These findings in mice with colonic polyps might translate to humans is suggested by data that human serum GDF15 levels are directly influenced by the presence of adenomatous polyps and elevated GDF15 serum levels rapidly reduce with removal of the polyp [28]. Further, only patients that display a rise in serum GDF15 levels with NSAID use are protected from adenomatous polyp development [29].
We have been studying the role of GDF15 in the spontaneous development of prostate cancer (PCa) in C57BL/6 background TRAMP mice in which we have genetically manipulated GDF15 expression. We have used C57BL/6 background mice, as unlike FVB background mice, they do not develop a high proportion of neuroendocrine PCa, which is rare in humans [30,31]. Further, unlike many cancers, TRAMP PCa do not express GDF15 [32]. Thus, this model is most reflective of the substantial minority of prostate cancer patients that do not express GDF15. These studies indicate that TRAMP mice with a germline deletion in Gdf15 develop PCa more quickly, have larger tumors and die earlier than TRAMP mice with wild type Gdf15 [33]. On the other hand, TRAMP mice with transgenic overexpression of GDF15 develop PCa much more slowly, have lower histological grade, smaller tumors and live much longer than either TRAMP mice with WT Gdf15 [32]. Such a protective role is supported by studies indicating that tumor tissue localized GDF15 staining is associated with a better outcome in patients with early stage PCa [20]. Interestingly however, with aging TRAMP mice overexpressing GDF15 develop more metastases than the other genotype TRAMP mice, suggesting that GDF15 may play a dual role in cancer [32]. It may protect from the development, growth and spread of early cancer but with advanced disease facilitate cancer spread. This dual role in cancer is seen with other cytokines, most prominently TGF-beta. Whatever the relationship of GDF15 to cancer outcome, because of its widespread expression by cancers and induction by many cancer therapies, understanding the role of GDF15 in cancer is likely to be of substantial clinical relevance as any impact on its expression is likely to have clinical consequences outside of GDF15’s role in cancer anorexia/cachexia syndrome.
One potential mechanism that may explain the differences in the action of GDF15 on early and advanced cancer and the differences in the data from induced or transgenic cancer models and mouse tumor xenograft models is modulation of antitumor immunity. To investigate this, we have again utilized the C57BL/6/TRAMP model of spontaneous PCa in which we have both studied and manipulated the immune environment.
Materials and methods
Ethical approval of the study
All animal experiments were approved by the Garvan/St. Vincent’s Hospital Animal Ethics Committee (Ethic approval number: 13/08 and 16/05). The experiments were performed in accordance with guidelines of Australian Code for the Care and Use of Animals for Scientific Purposes (8th Ed).
Mice
Transgenic PCa prone TRAMP mice [34] and mice overexpressing GDF15 under the control of the murine CSF-1 (fms) promoter (TRAMPfmsmic-1) have been previously described [12, 32, 35]. TRAMPrag-/- mice that lacked adaptive immunity were generated by crossing TRAMP+/- females with homozygous male WTrag-/- (B6.129S7-Rag1tm1Mom/J, The Jackson Laboratory) on a C57BL/6 background. A double transgenic MIC-1fms/rag-/- mouse line was generated by crossing homozygous MIC-1fms females with homozygous WTrag-/- males. Triple transgenic TRAMPfmsmic/rag1-/- mice were produced by crossing TRAMPrag-/- females with MIC-1fms/rag-/- males. All mice were on a C57BL/6 background. For mouse genotyping see S1 Data.
Survival study
TRAMP, TRAMPfmsmic-1, TRAMPrag-/- and TRAMPfmsmic/rag1-/- mice (n = 35/group) at 4–6 weeks of age were assigned to a survival study. Mice were housed in a pathogen-free animal facility at 22–23 °C with a 12:12 h light-dark cycle. Survival study was run for duration of 18 months. Mice were weighed weekly and checked twice a week for the presence of tumor by palpating of their abdomen. A 5% of morbidity and mortality was approved by ethics committee in the study but mice were euthanized on the same day as they reached ethical endpoint for the tumor size (11 mm x 11 mm) or meet any other ethical endpoint criteria such as: if the animal exhibits a ≥ 20% body weight loss, hunched posture, ruffled hair coat, a Body Condition Score of ≤ 2, bleeding from any orifices and has impaired mobility that restricts feeding, drinking and normal behaviors. Out of 35 mice per group, 3 mice in TRAMP group, 4 mice in TRAMPfmsmic-1 group, 3 mice in TRAMPrag-/- group and 5 mice in TRAMPfmsmic/rag1-/- group were euthanized for the reasons other than tumor growth. All the mice that reached ethical endpoint were killed by trained staff (Biological Testing Facility, Garvan Institute of Medical Research) by first anaesthetizing mice using Isoflurane (3% at a flow rate of 3 litres of oxygen per minute) and then by cervical dislocation. Mice killed for a reason other than seminal vesicle (SV) or prostate tumor were excluded from the study. Survival distribution was estimated by Kaplan-Meier method as previously described [32].
Primary tumor size in transgenic mice
Prostate tumor growth was compared in a group of TRAMP (n = 22), TRAMPfmsmic-1 (n = 15), TRAMPrag-/- (n = 18) and TRAMPfmsmic/rag1-/- (n = 14) mice at 25 weeks age. At necropsy, prostate tumor was excised from the GU complex and tumor weight was recorded. Prostate weight was normalized for the mouse body weight (tumor wt/body wt).
Orthotopic TRAMP tumor transplantation into WT, MIC-1fms, Rag-/- and MIC-1fms/rag-/- mice
To reduce the study time of 30–50 weeks in TRAMP mice, a period that is markedly increased by breeding of double or triple transgenic mice, we developed a more efficient approach based on tumor engraftment by intraprostatic injection of primary tumor cells from a donor TRAMP mouse into up to 50–60 recipient mice. We have called this the Orthotopic TRAMP Tumor Engraftment Model (OTTEM). A primary prostate tumor from a TRAMP mouse was excised, weighed, cut into small pieces of about 3 mm3 and then teased through a 100 u strainer. The cells were then washed and passed through 70 u and 40 u strainers to generate a single cell suspension. The tumor and dispersed cells were kept in DMEM with 10% FBS and maintained on ice throughout the tumor processing. Cells were given a final wash in serum free DMEM and the viable tumor cells were counted and the number adjusted to 106 cells/30 ul. Following surgical exposure and bladder retraction, a 30 ul volume of tumor cells was injected using a 0.5 ml insulin syringe by direct vision, into the dorsal prostate lobe where spontaneous TRAMP cancers develop. By ~4–6 weeks the TRAMP tumor cells orthotopically engrafted into WT syngeneic C57BL/6J mice, resulted in a tumor of about 1 cm diameter in the majority of mice. For experiments, donor tumors from TRAMP, TRAMPrag-/- or TRAMPMIC-/- mice were engrafted into a number of different WT or transgenic C57BL/6J background mice. Prostate tumor were excised and weighed at the end of the experiment, but in this instance not normalized to body weight. Since a different primary donor tumor is used for each experiment, there is experiment to experiment variability in the engrafted tumor growth, based on the aggressiveness of the donor tumor.
Systemic delivery of recombinant GDF15 by mini-osmotic pump
The in-house production and purification of recombinant mouse GDF15 (muGDF15), has been previously described [36]. One million primary prostate tumor cells from a donor TRAMP mouse were implanted into WT (n = 20) mouse prostates by intraprostatic injections as described above. On the same day 10 mice were implanted subcutaneously with a 28-day mini-osmotic pump (Model 2004, ALZET Osmotic pump, Cupertino, CA) containing recombinant muGDF15 to deliver 0.5 ug GDF15/g BW/day or vehicle, as previously described [37]. After 28 days, mice were sacrificed and prostate tumors were excised and weighed.
Systemic recombinant GDF15 and anti-PD1 antibody treatment
One million primary prostate tumor cells from a donor TRAMP mouse were implanted into prostates of 48 mice by intraprostatic injections, using the OTTEM model described above. After 72 hours post tumor implantation, 24 mice were implanted subcutaneously with 28-day mini-osmotic pump filled with recombinant muGDF15 to deliver 0.5 ug GDF15/g BW/day. The other 24 mice were implanted with pump containing vehicle. Starting from the day of osmotic pump implantation, half of both groups were also given twice weekly intraperitoneal injections of 250 ug/mouse rat anti-mouse PD1 monoclonal antibody (Clone RMP1-14, Bio X Cell) and the rest received 250 ug/mouse isotype control antibody (Rat IgG2a, Clone 2A3, Bio X Cell). After 28 days mice were sacrificed and prostate tumors were excised and weighed.
Detection of TRAMP tumor infiltrating lymphocytes
One million dispersed cancer cells from a primary TRAMPMIC-/- prostate tumor were injected into WT (n = 15) and MIC-1fms (n = 15) mouse prostate using the OTTEM model described above. At 5–6 weeks, intraprostatic tumors were excised and weighed. Tumors were cut into small pieces of about 3 mm3 and then teased through a 100 u strainer. Tumor cells were then washed with staining wash buffer (SWB, PBS with 2% FBS and 1 mM EDTA), passed through 70 u and 40 u strainers to generate a single cell suspension. Cell numbers were counted and one million cells from each tumor were centrifuged and blocked with purified anti-mouse CD16/CD32 monoclonal antibody clone 2.4G2 (0.5 ug/million cells in 50 ul) on ice for 10 minutes. Cells were then stained with the fluorochrome labelled anti mouse monoclonal antibodies (see S1 Table), for 20–30 minutes on ice in the dark. Stained cells were washed twice with SWB and subjected to multiparameter flow cytometry on LSRFortessa™ X-20 flow cytometer with BD FACSDiva™ software. Flow cytometry data was analyzed using FlowJo following the gating strategy shown in S1 Fig and described in S1 Data.
In order to compare proportion of tumor infiltrating CD8 T cells expressing PD1, dispersed tumor cells were stained with fluorochrome labeled anti mouse monoclonal antibody to identify, leukocytes (CD45+), CD4 T cells (CD3+CD4+) CD8 T cells (CD3+CD8+) and PD1 expressing CD8T cells (CD3+CD8+PD1+) (see panel 2, S1 Table). Gating strategy for the CD3+CD8+PD1+ cells is shown in S2 Fig.
In vivo CD8 T cell depletion of tumor bearing mice
We have depleted CD8 T cells in mice as previously described [38]. We validated the procedure first as described in S1 Data and S2 Table. We next depleted CD8 T cells from WT (n = 6) and MIC-1fms (n = 5) mice by injecting 400 ug of rat anti-CD8-α monoclonal antibody one day prior to intraprostatic tumor implantation. A control group of WT (n = 6) and MIC-1fms (n = 5) mice received 400 ug of isotype matched control antibody. On the next day we injected, TRAMPMIC-/- primary prostate tumor cells as mentioned above into prostate of all the above mice. CD8 depletion of mice was maintained by injecting same dose of anti CD8-α or control antibody, as above, twice weekly. After 5 weeks, mice were sacrificed; prostate tumors excised and weights were recorded.
Statistical analysis
Statistical evaluations of all the experiments were performed with GraphPad Prism software version 7 (GraphPad Software, San Diego, CA, USA). All the data are presented as the mean ± standard error of the mean (s.e.m.). Comparisons between groups were made using unpaired t tests. Survival curves were analyzed by Kaplan–Meier analysis and log-rank statistic is reported. A p value of 0.05 or less was considered statistically significant.
Results
GDF15 associated increase in survival of TRAMP mice is reversed in the absence of adaptive immunity
GDF15 reproducibly increases survival and reduces cancer growth of PCa prone transgenic TRAMP mice [32, 33]. In order to determine if this is mediated by adaptive immunity, we compared overall survival of C57BL/6J background TRAMP mice and TRAMP mice that overexpress GDF15 (TRAMPfmsmic-1) with their counterparts that also lack an adaptive immune system because of a concurrent deletion of the Rag1 gene (TRAMPRag1-/-, TRAMPfmsmic/rag1-/-; Fig 1a). We monitored this cohort of TRAMP, TRAMPfmsmic-1, TRAMPRag1-/- and TRAMPfmsmic/rag1-/- mice till they reached ethical endpoint. Kaplan-Meier survival analysis again demonstrated, as we have previously reported, that TRAMPfmsmic-1 mice have a significantly longer median survival of 51.3 weeks compared to 40.6 (Fig 1a; p = 0002) in TRAMP mice. Mice lacking overexpression of GDF15, with or without adaptive immunity, TRAMP, TRAMPrag-/-, respectively had a similar overall survival (median survival 40.6 weeks and 41.6 weeks respectively, p = 0.5, log-rank test). However, the longer survival of TRAMPfmsmic-1 was abrogated in the triple transgenic TRAMPfmsmic/rag1-/- mice (median survival 51.3 and 43.9 weeks respectively, p = 0.02, log-rank test). The absence of adaptive immunity reduced the median survival of TRAMPfmsmic/rag1-/- mice such that it was similar to that of TRAMP mice (median survival 43.9 and 40.6 weeks respectively, p = 0.08, log-rank test). These data indicate that the improvement in survival of GDF15 overexpressing TRAMPfmsmic-1 mice requires intact adaptive immunity.
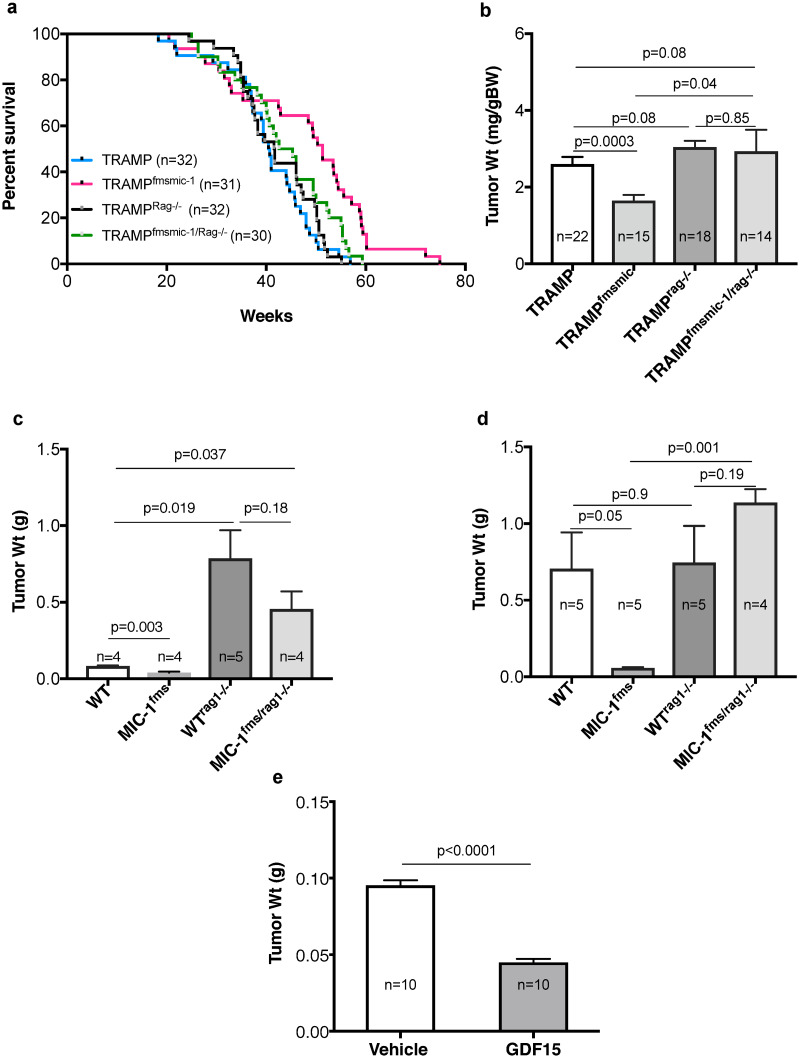
(a) Comparison of survival curves for C57BL/6 background TRAMP mice, TRAMPfmsmic-1 mice that also overexpress GDF15, immunodeficient TRAMPrag-/- mice and TRAMPfmsmic/rag1-/- mice that both overexpress GDF15 and lack adaptive immunity. Overall survival of individual mice from birth to death was plotted using the Kaplan-Meier method. (b) Body weight corrected prostate tumor weights of TRAMP, TRAMPfmsmic-1, TRAMPrag-/- and TRAMPfmsmic/rag1-/- mice at 25 weeks are presented as mean ± s.e.m. (c) Prostate tumor weights of WT, MIC-1fms, WTrag-/- and MIC-1fms/rag-/- mice 5 weeks after orthotopic implantation of primary tumor cells from a TRAMP mouse lacking adaptive immunity because of a germline deletion of the Rag-1 gene (TRAMPrag-/-). Results are presented as mean tumor weight ± s.e.m. (d) Prostate tumor weight of C57BL/6 mice that are WT, overexpress GDF15 (MIC-1fms), lack adaptive immunity (WTrag-/-) and or both overexpress GDF15 and lack adaptive immunity (MIC-1fms/rag-/-) 5 weeks after orthotopic engraftment of prostate tumor cells from a single C57BL/6 TRAMP mouse with a germline deletion of Gdf15 (TRAMPMIC-/-). The results are presented as mean tumor weight ± s.e.m. (e) To evaluate the effect of exogenous recombinant muGDF15 on tumor growth, C57BL/6 WT mice were implanted orthotopically with primary TRAMPMIC-/- prostate tumor cell and infused with vehicle or muGDF15 using 28-day mini-osmotic pump. Mice were sacrificed at 28 days. Results are presented as mean tumor weight ± s.e.m. All tumor weight data was analyzed using an unpaired 2-tailed t test and the p values are shown on the graphs.
GDF15 associated protection from TRAMP tumor growth is reversed in the absence of adaptive immunity
To directly assess the impact of lack of adaptive immunity on our previously observed GDF15 mediated reduction in PCa growth, we compared prostate tumor growth in a cohort of TRAMP (n = 22), TRAMPfmsmic-1 (n = 15), TRAMPrag-/- (n = 18) and TRAMPfmsmic/rag1-/- (n = 14) mice (Fig 1b). Mice were sacrificed at 25 weeks of age and the prostate tumors were isolated and weighed. There was 36.8% reduction in normalized tumor weight in TRAMPfmsmic-1 mice (Fig 1b, p = 0.0003), consistent with our previous findings [32]. There was no significant difference in the normalized prostate tumor weight between TRAMP and TRAMPrag-/- or TRAMPfmsmic/rag1-/- mice (Fig 1b, p = 0.08). This also demonstrated that the tumor size reduction that was present in the TRAMPfmsmic-1 cohort was abolished in the TRAMPfmsmic/rag1-/- group that overexpressed GDF15 but also lacked adaptive immunity (Fig 1b). Consistent with the survival study above, these data indicate that protection from prostate tumor growth in GDF15 overexpressing TRAMP mice requires intact adaptive immunity.
The immune system plays an important role in control of growth of orthotopically transplanted TRAMP prostate tumors
To be able to more efficiently study the impact of lack of adaptive immunity on GDF15 mediated reduction in PCa growth, we developed and used Orthotopic TRAMP Tumor Engraftment Model. We isolated a TRAMP tumor from a donor mouse then orthotopically implanted cells from this tumor into the prostates of multiple syngeneic genetic background recipient experimental mice. We implanted primary tumor cells isolated from a single TRAMPrag-/- prostate tumor into 4–5 per group of each of the following C57BL/6J background mice, WT, MIC-1fms, WTrag-/- and MIC-1fms/rag-/- mice. After 5wks, mice were sacrificed and their prostate tumors isolated and weighed (Fig 1c). TRAMPrag-/- tumor cells engrafted into WT mice show marked reduction in tumor growth compared to when the same tumor cells are engrafted into WTrag-/- (Fig 1c). This growth is further reduced when TRAMPrag-/- cells are engrafted into GDF15 overexpressing transgenic MIC-1fms mice (Fig 1c). In fact the weights of engrafted prostates excised from the MIC-1fms mice are not significantly different from those of age matched normal MIC-1fms mice (0.0396±0.007g and 0.037±0.001g respectively, p = 0.75) suggesting that all tumor cells might well have been eliminated in this group. The size of tumors in MIC-1fms/rag-/- mice, that overexpressed GDF15 but lacked adaptive immunity, engrafted with TRAMPrag-/- tumor cells, did not differ from that of the same tumor cells engrafted into WTrag-/-. The cells from the TRAMPrag-/- prostate tumor have never come in to contact with an adaptive immune system and will thus displayed increased sensitivity to adaptive immune mediated killing. For this reason, whilst adaptive immunity has no visible impact on the growth of tumors growing spontaneously in TRAMP mice (Fig 1b) it has a major impact on TRAMPrag-/- tumor cells (Fig 1c) and that impact is even greater in GDF15 overexpressing transgenic MIC-1fms mice. However, GDF15 cannot exert its protection from tumor growth without intact adaptive immunity.
GDF15 protection from growth of orthotopically transplanted prostate tumor in GDF15 overexpressing mice is reversed in Rag-/- mice
We next implanted isolated TRAMP primary prostate tumor cells into 5 per group of each of the following C57BL/6J background mice, WT, MIC-1fms, WTrag-/- and MIC-1fms/rag-/-. After 5wks, mice were sacrificed and their prostate tumors isolated and weighed. The pattern of tumor growth in these mice (Fig 1d) was similar to that in double and triple transgenic mice reported above (Fig 1b). GDF15 overexpressing MIC-1fms mice again had significantly smaller prostate tumors than WT mice (Fig 1d, p = 0.05). There was no significant difference in the prostate tumor weight between WT and WTrag-/- mice (Fig 1d, p = 0.9) nor was there any difference in tumor weight between WTrag-/- and MIC-1fms/rag-/- groups (Fig 1d, p = 0.19). Again, the protection from tumor growth in the MIC-1fms group was reversed when these mice were also immunodeficient because of concurrent Rag1 gene deletions (Fig 1d). Like the data from transgenic TRAMP mice, the orthotopic tumor size data suggests that the GDF15 mediated protection from growth of engrafted TRAMP tumors requires intact adaptive immunity.
Systemic recombinant GDF15 protects from PCa development
To determine if systemic GDF15 would also protect mice from the growth of TRAMP PCa, we first implanted the prostates of 20 WT mice with tumor cells from TRAMP with a normal immune system and bearing no other genetic modifications, using the OTTEM model. On the same day as tumor implantation, using a 28-day mini-osmotic pump, we started infusion of recombinant muGDF15 (0.5 ug/g BW/day) or vehicle for 28 days. Serum GDF15 levels in the cytokine infused group raises to about 6–8 ng/ml, which is less than the 10–14 ng/ml usually found in the blood of MIC-1fms mice. muGDF15 treated mice had markedly reduced prostate size compared to vehicle treated mice (Fig 1e, p<0001). Thus in the modest doses used, systemic GDF15 provides substantial protection from early PCa growth, indicating its effects on adaptive immunity can be reproduced with systemic delivery of this protein.
GDF15 protection from TRAMP prostate cancer growth is reversed by anti-CD8 antibody
A major cell responsible for adaptive immune mediated tumor killing is the CD8 T cell. To test if the protection from TRAMP PCa growth was mediated by this cell, we orthotopically implanted TRAMPMIC-/- tumor from one mouse into groups of WT and MIC-1fms mice. One day before implantation, we started injecting 400 ug of anti-CD8-α monoclonal antibody or 400 ug of Isotype matched anti KLH monoclonal antibody, intraperitoneally twice weekly for 35 days at which time mice were sacrificed and the tumors dissected and weighed. At necropsy, isotype control antibody treated MIC-1fms mice had significantly smaller tumor than isotype control antibody treated WT mice (Fig 2a, p<0.0001), consistent with the previously reported actions of GDF15. After CD8 T cell depletion WT and MIC-1fms tumor grew larger than their respective isotype control antibody treated mice (Fig 2a). However, CD8 T cell depleted MIC-1fms mice had significantly larger tumors than CD8 T cell depleted WT mice (Fig 2a, p = 0.019). Whilst some other immune cells such as dendritic cells may express CD8-α, in the context of the other available data, this suggests that CD8 T cells mediate the GDF15 induced reduction in TRAMP tumor growth and development.
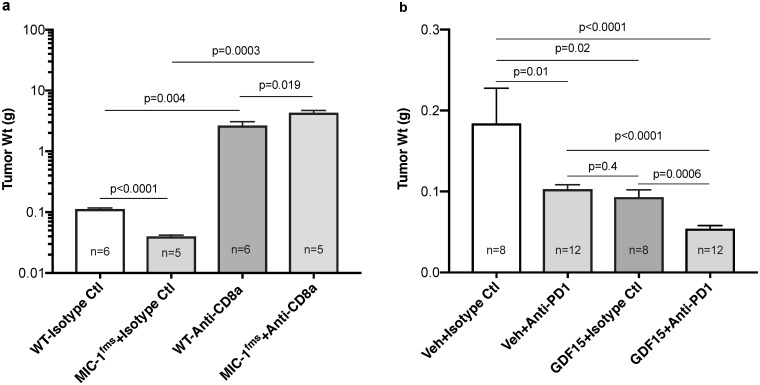
(a) All mice were injected twice weekly with anti-CD8-α monoclonal antibody or isotype matched anti KLH antibody starting one day before tumor cell implantation. TRAMPMIC-/- primary prostate tumor cells were engrafted orthotopically into WT and MIC-1fms mice and 35 days later mice were sacrificed and the tumors dissected and weighed. Results are presented as mean tumor weight ± s.e.m. (b) WT mice were engrafted orthotopically with TRAMPMIC-/- tumor cells. Three days later mice were infused with recombinant muGDF15 (0.5 ug/g BW/day) or vehicle via 28-day mini-osmotic pump and also given twice-weekly intraperitoneal injections of anti-PD1 monoclonal antibody (250 ug/mouse) or isotype control antibody. After 28 days mice were sacrificed and prostate tumors were excised and weighed. The graph represents compiled data from two experiments. Results are presented as mean tumor weight ± s.e.m. All tumor weight data was analyzed using unpaired 2-tailed t test and the p value are shown on the graphs.
Systemic recombinant GDF15 provides additional protection from PCa development to that provided by anti-PD1
Our data suggests that systemically delivered muGDF15 protects from TRAMP tumor growth by stimulating adaptive immunity. We therefore wanted to test whether GDF15 would provide additional protection to that from antibody to PD1, a well-characterized stimulator of antitumor immunity, being used in the clinic. Forty-eight WT mice in two batches were implanted intraprostatically with PCa cells derived from a single TRAMPMIC-/- donor mouse using the OTTEM model. On this occasion, to ensure that GDF15 was not acting by altering primary tumor cell implantation, the commencement of treatment was delayed for 3 days after which muGDF15 (0.5 ug/g BW/day) or vehicle via mini-osmotic pump was commenced. Mice were then given twice-weekly intraperitoneal injections of anti-mouse PD1 monoclonal antibody (250 ug/mouse) or isotype control antibody. After 28 days mice were sacrificed and prostate tumors were excised and weighed. Treatment with vehicle plus anti-PD1 or muGDF15 plus isotype control antibody displayed reduced prostate sizes compared to their respective control mice (Fig 2b, p = 0.01 and p = 0.02 respectively). Treatment of mice with both anti-PD1 antibody and muGDF15 further substantially reduced tumor growth over either of the two treatments alone (Fig 2b, p<0.0001 and p = 0.0006 respectively). In the combined treatment group, the average prostate weight of 0.054+/-0.003 g was indistinguishable from a group of 5 age matched WT mice prostate of 0.050 g+/-0.001 g (p = 0.346), suggesting that the tumor may have been almost, or completely eliminated. Thus, addition of muGDF15, to a well characterized stimulator of tumor immunity results in significant additional benefit.
GDF15 alters the tumor infiltrating lymphocytes of TRAMP prostate tumors
Our earlier data suggested that GDF15 mediated protection from tumor growth required intact adaptive immunity and CD8 T cells. To further investigate adaptive immunity, we studied the lymphocytic infiltrate of TRAMP tumors. In order to determine whether GDF15 overexpression altered the lymphocyte composition of tumors, using the OTTEM model, we orthotopically engrafted both WT and MIC-1fms mice with cells derived from prostate tumors of TRAMPMIC-/- mice. At 5–6 weeks, implanted intra-prostatic tumors from WT and MIC-1fms mice were excised and weighed an average of 3.0 g and 1.5 g respectively (p = 0.0001). Based on weight, a known proportion of the tumor from each mouse was then processed into a single cell suspension. Without any purification or fractionation, these cells were stained with fluorochrome labeled monoclonal antibodies and one million cells were subjected to multiparameter flow cytometry on BD LSRFortessa™ X-20 flow cytometer, followed by data analysis using FlowJo. Panels were designed with monoclonal antibodies to: Panel 1: CD45, CD3, CD4, CD8, NK1.1, CD11c, CD11b, and B220. Using the gating strategy shown in S1 Fig, focusing on the CD45 positive population, these antibodies allowed us to identify major T cell subsets (CD4, CD8), NK cells and B cells.
The overall results, reported in Table 1, indicate that the MIC-1fms tumors have a significantly higher proportion of T cells (48.3±0.28% versus 40.2±2.7%; p = 0.048) and more than twice the number of T cells/g of tumor compared to WT tumors (7.30x105±1.9x105 versus 3.18x105±0.7x105; p = 0.047). Tumors from MIC-1fms mice also had almost 2 fold more CD8+ T cells/g tumor in comparison to tumors from WT mice (Table 1, Fig 3a; p = 0.044). There was a trend for an increase in the number of CD4 T cells/g of tumor, but this fell just short of statistical significance (Table 1, p = 0.07). The number of CD4-CD8- T cells/g of tumor, that may represent gamma delta T cells were more than 2 fold higher in MIC-1fms tumors than WT tumors. There was no difference in the absolute number of B or NK cells but MIC-1fms tumors have significantly higher proportion of NK cells than WT tumors (Table 1, p = 0.043).
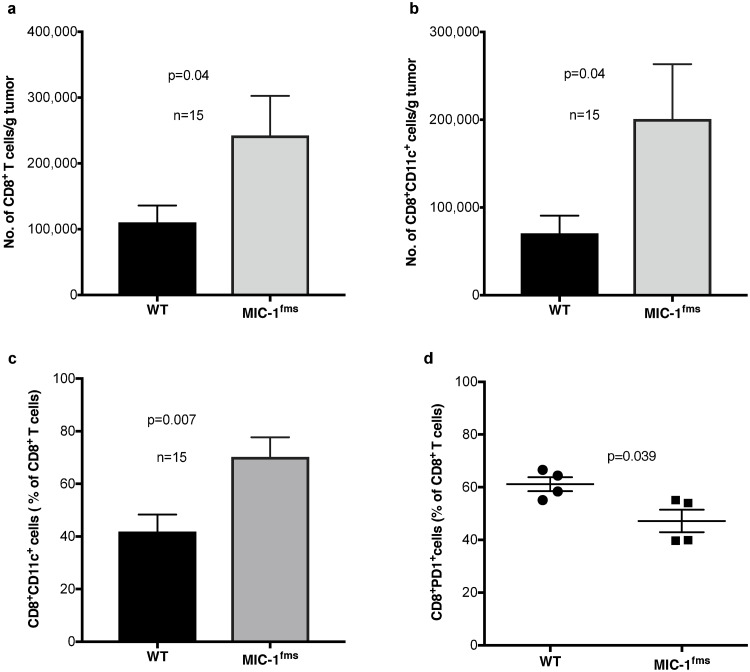
TRAMPMIC-/- primary prostate tumor cells were engrafted orthotopically into WT and MIC-1fms mice and 35 days later mice were sacrificed and cells from a known proportion of the orthotopically engrafted prostate tumor was subjected to multiparameter flow cytometry. (a) Number of tumor infiltrating CD3+CD8+T cells/g of intraprostatic tumor from WT and MIC-1fms mice. (b) Number of tumor infiltrating CD3+CD8+CD11c+ T cells/g of intraprostatic tumor from WT and MIC-1fms mice. (c) Proportion of tumor infiltrating CD3+CD8+ T cells also expressing CD11c in intraprostatic tumors from WT and MIC-1fms mice. (d) Proportion of tumor infiltrating CD3+CD8+ T cells expressing PD1 in the intraprostatic tumor from WT and MIC-1fms mice. All data are presented as mean cell number or percentage ± s.e.m. Data was analyzed using unpaired test and p value are shown on the graphs.
Table 1
TRAMPMIC-/- primary prostate tumor cells were engrafted orthotopically into WT and MIC-1fms mice and 35 days later mice were sacrificed and cells from a known proportion of the orthotopically engrafted prostate tumor was subjected to multiparameter flow cytometry. Numbers and proportion of cells between two groups were compared using unpaired t tests (GraphPad Prism software version 7,GraphPad Software, San Diego, CA, USA). All data are presented as mean cell number or percentage ± s.e.m.
Number of cells/g tumor ± s.e.m. x 103 | Lymphocytes | % Cells ± s.e.m. | |||||
---|---|---|---|---|---|---|---|
WT Tumor | MIC-1fms Tumor | p value | WT Tumor | MIC-1fms Tumor | p value | ||
T cells | |||||||
CD3+ | 318 ± 68 | 730 ± 191 | 0.047 | CD3+ (% of CD45+ cells) | 40.2 ± 2.7 | 48.3 ± 2.8 | 0.048 |
CD4+ | 39 ± 10 | 99 ± 31 | 0.070 | CD4+ (% of CD3T cells) | 11.2 ± 0.9 | 13.1 ± 1.0 | 0.174 |
CD8+ | 110 ± 25 | 242 ±60 | 0.044 | CD8+ (% of CD3T cells) | 39.9 ± 3.5 | 45.02 ± 3.1 | 0.287 |
CD4+CD8+ | 5 ± 2 | 8 ± 3 | 0.415 | CD4+CD8+ (% of CD3T cells) | 1.3 ± 0.4 | 1.2 ± 0.2 | 0.949 |
CD4-CD8- | 148 ± 29 | 329 ± 80 | 0.038 | CD4-CD8- (% of CD3T cells) | 47.3 ± 3.6 | 45.5 ± 4.1 | 0.741 |
B cells | |||||||
B220+ | 61 ± 15 | 83 ± 16 | 0.299 | B220+ (% of CD45+ cells) | 8.2 ± 0.7 | 7.4 ± 0.4 | 0.319 |
NK cells | |||||||
NK1.1+ | 9 ± 2 | 29 ± 12 | 0.105 | NK1.1+ (% of CD45+ cells) | 0.8 ± 0.1 | 1.1 ± 0.3 | 0.043 |
MIC-1fms mice with orthotopically transplanted TRAMP tumors display altered CD8 T cell subsets suggestive of reduced "exhaustion"
CD8 T cell mediated tumor killing is an important mechanism of antitumor immunity. We have therefore examined in more detail the impact of GDF15 on CD8 T cells subpopulations in WT and MIC-1fms mice implanted intraprostatically with TRAMP tumors. Surface expression of CD11c on CD8 T cells identifies a population of antigen stimulated cytotoxic effector cells that secrete large amounts of IFNg [39]. As well as having more CD8 T cells (Fig 3a), MIC-1fms tumors have higher numbers of CD8+CD11c+ T cells/g tumor (Fig 3b, p = 0.04) and the proportion of these cells is also increased from 41.8±6.5% to 70.3±7.4% (Fig 3c, p = 0.007) of CD8 T cells.
Cells surface expression of the immune checkpoint protein PD1 is a hallmark of "exhausted" CD8 T cells that have a depressed capacity to mediate antitumor immunity. The CD8 T cells from MIC-1fms mice showed a substantially reduced proportion of exhausted CD8+PD1+ T cells (Fig 3d, p = 0.039). Overall, these phenotypic changes in CD8 T cells from tumor bearing MIC-1fms mice suggest that they are less exhausted and may have a greater capacity for tumor cell killing.
Discussion
Using a robust transgenic model our data clearly shows that the GDF15 mediated protection from growth of the PCa that develops spontaneously in C57/BL6 TRAMP mice, is dependent on intact adaptive immunity and in mice with adoptively transferred TRAMP tumors this response is at least in part mediated by CD8 bearing cells that are almost certainly T cells. The improved survival and reduced tumor size that is seen in GDF15 overexpressing TRAMPfmsmic mice is completely abrogated in triple transgenic TRAMPfmsmic/rag-/- mice, that also lack adaptive immunity because of deletion of the Rag1 gene (Figs 1a and 1b). This data is consistent with other studies, which demonstrate that immune system manipulation does, as expected, impact the progressions to TRAMP tumors [40–43].
Whilst transgenic mice that spontaneously develop cancer, such as TRAMP mice, display a biology that more closely corresponds to the biology of the cancer under study, they also suffer from some important disadvantages. Foremost amongst these is the time required to develop double and triple transgenic mouse lines and the length of time required for tumor development which in TRAMP mice is approximately 6 months. To overcome some of these limitations and thus to be able to investigate the role of GDF15 more efficiently, we have established the OTTEM model in which prostate tumors derived from mice carrying the TRAMP transgene are engrafted orthotopically into the dorsal lobe of the prostates of mice of various genotypes. This strategy allows the engraftment of multiple mice from a tumor derived from a single donor mouse as well as the much more rapid evolution of tumor development (4–6 weeks versus 6 months), whilst still utilizing a primary spontaneous TRAMP tumor.
Using this OTTEM model, we can demonstrate similar GDF15 mediated, adaptive immunity dependent changes in tumor development, as that seen using spontaneous cancer development in TRAMP and TRAMPfmsmic mice (Fig 1d). This GDF15 mediated protection from tumor growth is even more marked when the orthotopically engrafted cells come from TRAMPrag-/- whose tumor has not undergone editing by the immune system prior to engraftment (Fig 1c). The effect of transgenic GDF15 overexpression can be reproduced by administering recombinant muGDF15 (Fig 1e) Further, consistent with GDF15 actions on adaptive immunity, the effect of recombinant muGDF15 infusion is additive to the protection offered by a monoclonal antibody to the immune checkpoint protein PD1 (Fig 2b). Thus, using multiple approaches our data indicates that the GDF15 protection from early TRAMP tumor growth requires adaptive immunity including CD8 T cells. Further, because the effects of GDF15 can be reproduced using systemically delivered GDF15 protein, it might be suitable as a therapeutic stimulator of antitumor immunity.
The direct target immune cell for the action of GDF15 is still uncertain. However, by direct or indirect mechanisms, its ultimate impact is likely to be at least in part on the CD8 T cells. Evidence for this is data that a monoclonal antibody to CD8, which causes CD8 T cell depletion, reverses the protective actions of GDF15 (Fig 2a). The phenotypic changes in CD8 T cells we have identified are also consistent with GDF15 stimulation of tumor immunity. The absolute number and proportion of tumor infiltrating CD3 expressing T cells is increased and also the absolute number of its CD8 subset, but the proportion of CD8 cells is not increased. Further, a more detailed evaluation of these CD8 T cells from tumors from GDF15 overexpressing mice indicates they have both a higher number and proportion of CD8+CD11c+ cells, a population that is thought to have been recently activated and produce large amounts of IFNg (Fig 3b and 3c). They also have more CD8 cells with reduced cell surface expression of PD1, a marker of T cell exhaustion (Fig 3d).
Overall these studies indicate that GDF15 overexpression or administration of recombinant muGDF15 leads to protection from the progress of spontaneous PCa in TRAMP and development of tumors in OTTEM mice that is dependent on having intact adaptive immunity and the presence of CD8 T cells. Additionally, muGDF15 infusion has at least an additive effect with checkpoint blocker therapy. This suggests that further studies may be warranted to determine if GDF15 has application as a stimulator of tumor immunity, in the therapy of cancer.
Supporting information
S1 Fig
Gating strategy for the cells from prostate tumor.(PDF)
S2 Fig
Gating strategy for the CD3+CD8+ T cells expressing PD1 in the prostate tumor.(PDF)
S1 Table
List of antibodies used for flow cytometry.(DOCX)
S2 Table
Effect of anti-CD8-alpha treatment of mice on CD8+ T cells proportions.(DOCX)
Funding Statement
This work was financially supported by grants from the National Health and Medical Research Council (NHMRC) of Australia to SNB (568762, https://www.nhmrc.gov.au/), Cancer Council NSW to SNB (400932 and 1042661, https://www.cancercouncil.com.au/) and St. Vincent’s Clinic Foundation grant to SNB and YH (https://www.stvincentsclinic.com.au/svc-portal/research-grants). The funders had no role in study design, data collection and analysis, decision to publish, or preparation of the manuscript.
Data Availability
All relevant data are within the manuscript and its Supporting Information files.
References
Decision Letter 0
1 Apr 2020
PONE-D-19-32258
Growth Differentiation Factor-15 slows the growth of murine prostate cancer by stimulating tumor immunity
PLOS ONE
Dear Dr Breit:
Thank you for submitting your manuscript to PLOS ONE. After careful consideration, we feel that it has merit but does not fully meet PLOS ONE’s publication criteria as it currently stands. Therefore, we invite you to submit a revised version of the manuscript that addresses the points raised during the review process. During the review process, we identified this manuscript very informative and identified some minor issues which need to be addressed. These are as follows:
1. There are numerous typographical errors in the manuscript.
2. Authors should write appropriate scientific names of genes such "Tgfb should be written as TGF beta (or symbol).
3. Authors should write correctly the genes of mouse and human origin as per scientific nomenclature. In the manuscript there is no distinction between human and mouse genes and appears confusing in various places.
Please include the following items when submitting your revised manuscript:
A rebuttal letter that responds to each point raised by the academic editor and reviewer(s). This letter should be uploaded as separate file and labeled 'Response to Reviewers'.
A marked-up copy of your manuscript that highlights changes made to the original version. This file should be uploaded as separate file and labeled 'Revised Manuscript with Track Changes'.
An unmarked version of your revised paper without tracked changes. This file should be uploaded as separate file and labeled 'Manuscript'.
Please note while forming your response, if your article is accepted, you may have the opportunity to make the peer review history publicly available. The record will include editor decision letters (with reviews) and your responses to reviewer comments. If eligible, we will contact you to opt in or out. We look forward to receiving your revised manuscript.
Kind regards,
M. Saleem
Academic Editor
PLOS ONE
Journal requirements:
When submitting your revision, we need you to address these additional requirements.
Please ensure that your manuscript meets PLOS ONE's style requirements, including those for file naming. The PLOS ONE style templates can be found at
https://journals.plos.org/plosone/s/file?id=wjVg/PLOSOne_formatting_sample_main_body.pdf and
1. As per our survival studies guidelines please amend the Methods section which describes humane endpoints by adding the number of mice that reached these endpoints or were found dead, if any.
2. We note that you have a patent relating to material pertinent to this article. Please provide an amended statement of Competing Interests to declare this patent (with details including name and number), along with any other relevant declarations relating to employment, consultancy, patents, products in development or modified products etc. Please confirm that this does not alter your adherence to all PLOS ONE policies on sharing data and materials, as detailed online in our guide for authors http://journals.plos.org/plosone/s/competing-interests by including the following statement: "This does not alter our adherence to PLOS ONE policies on sharing data and materials.” If there are restrictions on sharing of data and/or materials, please state these. Please note that we cannot proceed with consideration of your article until this information has been declared.
This information should be included in your cover letter; we will change the online submission form on your behalf.
Please know it is PLOS ONE policy for corresponding authors to declare, on behalf of all authors, all potential competing interests for the purposes of transparency. PLOS defines a competing interest as anything that interferes with, or could reasonably be perceived as interfering with, the full and objective presentation, peer review, editorial decision-making, or publication of research or non-research articles submitted to one of the journals. Competing interests can be financial or non-financial, professional, or personal. Competing interests can arise in relationship to an organization or another person. Please follow this link to our website for more details on competing interests: http://journals.plos.org/plosone/s/competing-interests
3. PLOS requires an ORCID iD for the corresponding author in Editorial Manager on papers submitted after December 6th, 2016. Please ensure that you have an ORCID iD and that it is validated in Editorial Manager. To do this, go to ‘Update my Information’ (in the upper left-hand corner of the main menu), and click on the Fetch/Validate link next to the ORCID field. This will take you to the ORCID site and allow you to create a new iD or authenticate a pre-existing iD in Editorial Manager. Please see the following video for instructions on linking an ORCID iD to your Editorial Manager account: https://www.youtube.com/watch?v=_xcclfuvtxQ
Author response to Decision Letter 0
12 May 2020
Response to Reviewer and Editors Comments
1. There are numerous typographical errors in the manuscript.
Response: We have corrected all typographical errors we have been able to identity
2. Authors should write appropriate scientific names of genes such "Tgfb should be written as TGF beta (or symbol).
Response: We have replaced TGF-b with TGF-beta.
3. Authors should write correctly the genes of mouse and human origin as per scientific nomenclature. In the manuscript there is no distinction between human and mouse genes and appears confusing in various places.
Response: We have corrected the representation of genes in this manuscript using official designations. For example the gene for murine GDF15 becomes Gdf15. Additionally we have clarified the species of GDF15 protein used in the studies e.g. GDF15 becomes muGDF15.
1. As per our survival studies guidelines please amend the Methods section which describes humane endpoints by adding the number of mice that reached these endpoints or were found dead, if any.
Response:We have amended the methods on P8, L164-166, as follows:
Out of 35 mice per group, 3 mice in TRAMP group, 4 mice in TRAMPfmsmic-1 group, 3 mice in TRAMPrag-/- group and 5 mice in TRAMPfmsmic/rag1-/- group were euthanized for the reasons other than tumor growth.
2. We note that you have a patent relating to material pertinent to this article. Please provide an amended statement of Competing Interests to declare this patent
Response:Please note that the numbers of patents are too numerous to list individually as there are multiple patent families and each family has a different number in each different country/jurisdiction. Because of this, in our last PLOS ONE publication (Husaini, Y et al (2015) PLoS One 10:e0115189.) we had agreed on the format below, which I hope you find acceptable.
DAB and SNB are named inventors on patents owned by St Vincent’s Hospital that pertain to the clinical use of a MIC-1/GDF15 diagnostic assay and modulatory therapy. DAB and SNB declare no competing non-financial interests. YH, VWWT, RM, HPZ, KMLN, HL and CPM declare no competing financial and/or nonfinancial interests. St Vincent’s Hospital agrees to make freely available any materials and information described in this publication that may be reasonably requested for the purpose of academic, non-commercial research. Due to the proprietary nature of the materials, the parties will need to enter into a material transfer agreement. This does not alter the authors’ adherence to all the PLOS ONE policies on sharing data and materials.
3.PLOS requires an ORCID iD for the corresponding author in Editorial Manager on papers submitted after December 6th, 2016
Samuel Breit’s ORCID ID is 0000-0002-9021-9879
Attachment
Submitted filename: Response to reviewers.docx
Decision Letter 1
14 May 2020
Growth Differentiation Factor-15 slows the growth of murine prostate cancer by stimulating tumor immunity
PONE-D-19-32258R1
Dear Dr. Samuel N Breit,
We are pleased to inform you that your manuscript has been judged scientifically suitable for publication and will be formally accepted for publication once it complies with all outstanding technical requirements.
Within one week, you will receive an e-mail containing information on the amendments required prior to publication. When all required modifications have been addressed, you will receive a formal acceptance letter and your manuscript will proceed to our production department and be scheduled for publication.
Shortly after the formal acceptance letter is sent, an invoice for payment will follow. To ensure an efficient production and billing process, please log into Editorial Manager at https://www.editorialmanager.com/pone/, click the "Update My Information" link at the top of the page, and update your user information. If you have any billing related questions, please contact our Author Billing department directly at gro.solp@gnillibrohtua.
If your institution or institutions have a press office, please notify them about your upcoming paper to enable them to help maximize its impact. If they will be preparing press materials for this manuscript, you must inform our press team as soon as possible and no later than 48 hours after receiving the formal acceptance. Your manuscript will remain under strict press embargo until 2 pm Eastern Time on the date of publication. For more information, please contact gro.solp@sserpeno.
With kind regards,
Mohammad Saleem
Academic Editor, PLOS ONE
Masonic Cancer Center, University of Minnesota, Minneapolis, USA
Acceptance letter
28 May 2020
PONE-D-19-32258R1
Growth Differentiation Factor-15 slows the growth of murine prostate cancer by stimulating tumor immunity
Dear Dr. Breit:
I am pleased to inform you that your manuscript has been deemed suitable for publication in PLOS ONE. Congratulations! Your manuscript is now with our production department.
If your institution or institutions have a press office, please notify them about your upcoming paper at this point, to enable them to help maximize its impact. If they will be preparing press materials for this manuscript, please inform our press team within the next 48 hours. Your manuscript will remain under strict press embargo until 2 pm Eastern Time on the date of publication. For more information please contact gro.solp@sserpeno.
For any other questions or concerns, please email gro.solp@enosolp.
Thank you for submitting your work to PLOS ONE.
With kind regards,
PLOS ONE Editorial Office Staff
on behalf of
Dr. MOHAMMAD Saleem
Academic Editor
PLOS ONE
Articles from PLOS ONE are provided here courtesy of PLOS
Full text links
Read article at publisher's site: https://doi.org/10.1371/journal.pone.0233846
Read article for free, from open access legal sources, via Unpaywall:
https://journals.plos.org/plosone/article/file?id=10.1371/journal.pone.0233846&type=printable
Citations & impact
Impact metrics
Citations of article over time
Alternative metrics
Article citations
GDF15: Immunomodulatory Role in Hepatocellular Carcinoma Pathogenesis and Therapeutic Implications.
J Hepatocell Carcinoma, 11:1171-1183, 19 Jun 2024
Cited by: 0 articles | PMID: 38911292 | PMCID: PMC11193986
Review Free full text in Europe PMC
Suppression of malignant melanoma by knocking down growth differentiation factor-15 via inhibiting PTEN/PI3K/AKT signaling pathway.
J Cancer, 15(4):1115-1123, 01 Jan 2024
Cited by: 1 article | PMID: 38230211 | PMCID: PMC10788716
Consensus cluster analysis of apoptosis-related genes in patients with osteoarthritis and their correlation with immune cell infiltration.
Front Immunol, 14:1202758, 04 Oct 2023
Cited by: 2 articles | PMID: 37860011 | PMCID: PMC10582959
Expression of microRNA-379 reduces metastatic spread of prostate cancer.
Front Oncol, 13:1252915, 12 Sep 2023
Cited by: 0 articles | PMID: 37781173 | PMCID: PMC10539900
Interactions between B cells and T follicular regulatory cells enhance susceptibility to Brucella infection independent of the anti-Brucella humoral response.
PLoS Pathog, 19(9):e1011672, 18 Sep 2023
Cited by: 4 articles | PMID: 37721965 | PMCID: PMC10538787
Go to all (14) article citations
Data
Data behind the article
This data has been text mined from the article, or deposited into data resources.
BioStudies: supplemental material and supporting data
Similar Articles
To arrive at the top five similar articles we use a word-weighted algorithm to compare words from the Title and Abstract of each citation.
Macrophage inhibitory cytokine-1 (MIC-1/GDF15) slows cancer development but increases metastases in TRAMP prostate cancer prone mice.
PLoS One, 7(8):e43833, 27 Aug 2012
Cited by: 42 articles | PMID: 22952779 | PMCID: PMC3428289
Macrophage inhibitory cytokine-1 (MIC-1/GDF15) gene deletion promotes cancer growth in TRAMP prostate cancer prone mice.
PLoS One, 10(2):e0115189, 19 Feb 2015
Cited by: 12 articles | PMID: 25695521 | PMCID: PMC4335046
Adoptive transfer of tumor-reactive transforming growth factor-beta-insensitive CD8+ T cells: eradication of autologous mouse prostate cancer.
Cancer Res, 65(5):1761-1769, 01 Mar 2005
Cited by: 81 articles | PMID: 15753372
Growth differentiation factor 15 (GDF15) in cancer cell metastasis: from the cells to the patients.
Clin Exp Metastasis, 37(4):451-464, 05 Jun 2020
Cited by: 21 articles | PMID: 32504264
Review