Abstract
Free full text
Clinical Pharmacokinetics of the Novel HIV-1 Non-Nucleoside Reverse Transcriptase Inhibitor Doravirine: An Assessment of the Effect of Patient Characteristics and Drug–Drug Interactions
Abstract
Doravirine (MK-1439) is a novel non-nucleoside reverse transcriptase inhibitor indicated for the combination treatment of human immunodeficiency virus type-1 (HIV-1) infection. The recommended dose is 100 mg once daily. This review summarizes the pharmacokinetics of doravirine, the influence of intrinsic factors, and its drug–drug interaction (DDI) profile. Following oral administration, doravirine is rapidly absorbed (median time to maximum plasma concentration, 1–4 h) and undergoes cytochrome P450 (CYP)3A-mediated oxidative metabolism. Steady-state geometric means for AUC0–24, C24, and Cmax in individuals with HIV-1 following administration of doravirine 100 mg once daily are 37.8 μM·h, 930 nM, and 2260 nM, respectively. Age, gender, severe renal impairment, and moderate hepatic impairment have no clinically meaningful effect on doravirine pharmacokinetics, and there is limited potential for DDIs. No dose adjustment is necessary when doravirine is co-administered with strong CYP3A inhibitors. However, doravirine is contraindicated with strong CYP3A inducers (e.g., rifampin), and dose adjustment of doravirine is recommended for co-administration with the moderate CYP3A inducer, rifabutin. Included in this review are clinical trial data from phase I pharmacokinetic trials, including DDI trials and trials in participants with renal and hepatic disease but without HIV-1 infection (N=
326), as well as phase I, II, and III safety and efficacy trials in participants living with HIV-1 (N
=
991). Based on these data, the pharmacokinetic profile of doravirine supports its use in diverse populations living with HIV-1 and allows co-administration with various antiretroviral agents and treatments for commonly occurring co-morbidities.
Electronic supplementary material
The online version of this article (10.1007/s40261-020-00934-2) contains supplementary material, which is available to authorized users.
Key Points
There is limited potential for demographics and organ impairment to affect the pharmacokinetics of doravirine. |
There is limited potential for drug–drug interactions with doravirine; however, co-administration with strong CYP3A inducers is contraindicated and dose adjustment is recommended for co-administration with the moderate CYP3A inducer rifabutin. |
The pharmacokinetic profile of doravirine supports its use across diverse populations living with HIV-1. |
Introduction
Human immunodeficiency virus (HIV) infection continues to be a global concern. In 2017, nearly 37 million people worldwide were living with HIV, and 1.8 million people were newly diagnosed with the virus [1]. Current guidelines recommend that all individuals with HIV infection be treated with antiretroviral therapy [2, 3]. First-line three-drug regimens generally consist of two nucleoside reverse transcriptase inhibitors (NRTIs) in combination with a third drug from another class, usually an integrase strand transfer inhibitor (InSTI) for most individuals with HIV initiating antiretroviral therapy; a non-nucleoside reverse transcriptase inhibitor (NNRTI) or a boosted protease inhibitor (PI) may be given instead of an InSTI in certain clinical situations [2, 3]. Although many treatment options are available, multiple factors can reduce the appropriateness of certain therapies for some individuals, including viral resistance to antiretroviral drugs, tolerability and associated side effects, and drug–drug interactions (DDIs). Among NNRTIs, efavirenz has been associated with central nervous system-related adverse events (AEs) as well as significant DDIs, and rilpivirine has reduced efficacy in individuals with high baseline HIV RNA and a low CD4+ cell count [3].
Doravirine is a novel NNRTI designed to overcome the limitations of other drugs in the class [4, 5]. Doravirine is active in vitro against typical NNRTI-associated mutations that are susceptible to other NNRTIs and exhibits a low rate of resistance and a unique resistance pathway [6, 7]. In two pivotal non-inferiority phase III trials (DRIVE-FORWARD, ClinicalTrials.gov NCT02275780; DRIVE-AHEAD, ClinicalTrials.gov NCT02403674), doravirine, as part of a combination therapy, demonstrated robust antiretroviral efficacy and a favorable safety profile [4, 5]. Additionally, doravirine had an improved neuropsychiatric profile compared with efavirenz and a favorable lipid profile compared with efavirenz or darunavir [4, 5]. Based on these data, doravirine 100 mg administered orally once daily in combination with other antiretroviral agents, and as part of a three-drug single-tablet regimen with lamivudine (3TC) 300 mg and tenofovir disoproxil fumarate (TDF) 300 mg, is indicated for use in adults with HIV-1 infection [8, 9]. In the USA, it is indicated for those with no prior antiretroviral treatment history or to replace the current antiretroviral regimen in those who are virologically suppressed on a stable antiretroviral regimen with no history of treatment failure and no known substitutions associated with resistance to doravirine (or to 3TC and TDF for the three-drug single-tablet regimen) [8, 9].
This article reviews the pharmacokinetics of doravirine and includes assessments of the potential effects of demographic factors and the DDI profile of doravirine.
Methods of Literature Search
All sponsor-conducted/funded and published phase I DDI, special population, and pharmacokinetic-profiling trials evaluating doravirine available at the time of writing are included in this review. The phase I trials include those in participants without HIV-1 and a single phase I trial in people living with HIV-1. In addition, this review includes published data from sponsor-conducted phase II and phase III trials, which evaluated safety and efficacy of doravirine in people living with HIV-1. All sponsor data were published in peer-reviewed journals. Details of each trial in this review, including design, sample size, treatments, and trial population, can be found in Table S1. In addition, the bounds of clinical relevance for doravirine based on the totality of the phase I, II, and III data are also discussed, which provide context for the effects on doravirine pharmacokinetics observed in DDI and special population trials.
Doravirine Pharmacokinetics
Doravirine is a drug with low intrinsic clearance (3.73 L/h following intravenous administration) [10]. Its volume of distribution of 60.5 L—which is slightly larger than the volume of body water—suggests that doravirine distributes into tissues [10]. Doravirine undergoes oxidative metabolism mediated primarily by cytochrome P450 (CYP) 3A4, and to a lesser extent by CYP3A5 [10]. In an absorption, metabolism, and excretion (AME) study of [14C]doravirine, the major circulating component in plasma was unchanged [14C]doravirine (75%); M9, a metabolite that results from oxidation of the triazolone ring of doravirine, was the most abundant metabolite in plasma, where it accounted for 12.9% of the drug-related material [10]. M9 was not active against HIV reverse transcriptase in vitro. In addition, M9 was the major metabolite excreted in urine and feces (~39% and
~
16% of absorbed dose, respectively). Other measurable metabolites in urine accounted for
<
5% of the absorbed dose. Only a small proportion of doravirine was excreted unchanged in urine (6.3–13%) [10, 11], and based on the mass balance and excretion of doravirine in the [14C] AME study, biliary excretion is anticipated to be minor. Thus, excretion of unchanged drug is not a major pathway in the elimination of doravirine [10].
Following administration of single oral doses of doravirine (6–1200 mg) to healthy men, doravirine is rapidly absorbed with a median time to maximum plasma concentration (Tmax) of 1–4 h [11]. Plasma concentrations declined in a single exponential phase, with an apparent terminal half-life of 12–19 h (Fig. 1). Plasma area under the concentration–time curve from time zero to infinity (AUC0–∞), maximum plasma concentration (Cmax), and plasma concentration at 24 h post-dose (C24) increased in a slightly less than dose-proportional manner over the 6–1200 mg dose range. Median time to steady state was~
2 days, and mean area under the concentration–time curve from time zero to 24 h post-dose (AUC0–24), C24, and Cmax accumulation ratios (geometric mean ratio [GMR] Day 10/Day 1) were 1.2–1.4 [11]. These data are consistent with the apparent terminal half-life and indicate time-independent pharmacokinetics. Multiple-dose pharmacokinetics are supportive of once-daily dosing of doravirine [11], and it has been shown that plasma concentrations following 7 days of once-daily dosing with 100 mg of doravirine are maintained above the half maximal effective concentration (IC50) for inhibition of wild-type virus for 72 h after the final dose in adults without HIV infection [12].
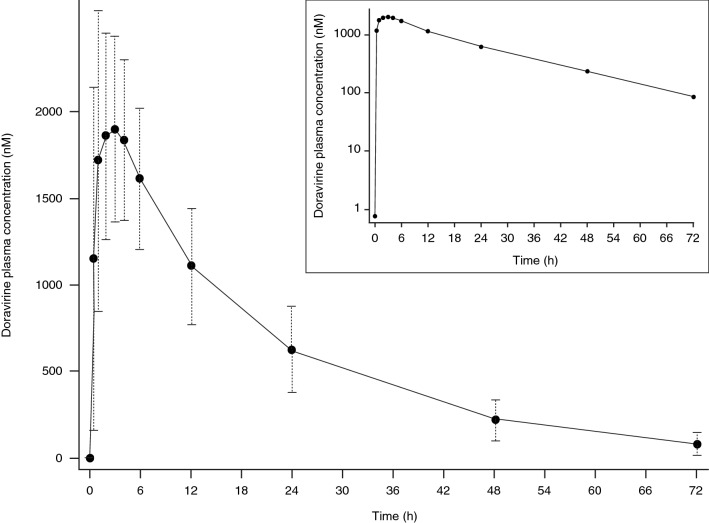
Mean (±SD) doravirine plasma concentration–time profile following single oral dose administration of doravirine 100 mg in healthy individuals (N
=
24). The inset shows the same data plotted on a semi-log scale. SD standard deviation
The pharmacokinetics of doravirine in individuals with HIV-1 infection were similar to those in healthy individuals [13, 14]. Based on a population pharmacokinetic analysis of individuals with (n=
959) and without (n
=
341) HIV-1 infection, individuals with HIV-1 infection receiving doravirine 100 mg once daily have a doravirine geometric mean (% coefficient of variation) AUC0–24 of 37.8 μM·h (27.0%), C24 of 930 nM (41.6%), and Cmax of 2260 nM (18.4%) [14]. Corresponding steady-state AUC0–24, C24, and Cmax in healthy individuals following administration of 100 mg once daily were 41.1 μM·h, 902 nM, and 2880 nM, respectively [15]. Therefore, findings from trials evaluating doravirine pharmacokinetics carried out in participants without HIV-1 infection are expected to be applicable to those with HIV infection.
Definition of the Bounds of Clinical Relevance
The term “bounds of clinical relevance” refers to the range of doravirine pharmacokinetic values relative to those associated with the recommended dose of 100 mg once daily at steady state where safety and efficacy are expected to be comparable to the clinical dose. When altered pharmacokinetic exposure of doravirine falls within these bounds, safety and efficacy are expected to be comparable to those of 100 mg once-daily doravirine. The definition of clinical relevance bounds for doravirine is based on demonstrated efficacy and safety in the phase I, IIb, and III trials, as described below.
Exposure–response analyses [14] of efficacy endpoints from the two phase III trials [4, 5] were used to identify the lower bound of clinical relevance for doravirine, which was defined with respect to steady-state C24; maintenance of minimal effective trough levels is typically associated with efficacy for antiretroviral therapies [16]. Based on analyses for the proportion of individuals achieving HIV-1 RNA<
50 copies/mL (virologic response) and occurrence of virologic failure at week 48, both exposure–response relationships were generally flat over the entire range of doravirine steady-state C24 values from the phase III trials, except for a slight decrease in efficacy in individuals with the lowest 10% of doravirine steady-state C24 values [14]. Above the tenth percentile of doravirine steady-state C24 values, there were no meaningful changes in the exposure–response relationships for virologic response and failure across the entire range of doravirine steady-state C24 values [14]. The observed exposure–response trends in the first decile of doravirine steady-state C24 values were likely driven by individuals with lower adherence, and therefore lower efficacy rates, rather than reflecting a true decrease in response at lower exposures. In fact, flat exposure– and dose–response relationships were observed over the 25–200 mg once-daily dose range in the phase IIb trial [14]. Nevertheless, the tenth percentile of doravirine steady-state C24 values observed in phase III, corresponding to a 40% decrease in doravirine C24, was set as a conservative lower bound for clinical relevance with respect to efficacy. Steady-state C24 values above this bound are expected to correspond to efficacy similar to the 100 mg once-daily dose.
A 40% decrease in doravirine C24 also corresponds approximately to the C24 values associated with the 50 mg once-daily dose and is nearly twice the C24 associated with the 25 mg once-daily dose [14], which were studied in the phase IIb trial and had efficacy similar to 100 mg once daily [17]. Even at this decreased C24, doravirine plasma concentrations exceed the pharmacokinetic target of 78 nM, which is greater than 6-fold the in vitro IC50 for inhibition of wild-type virus, and exceeds IC50 values for common single resistance mutations (K103N, Y181C, G190A), and of the K103N/Y181C double mutant [16, 18, 19].
Steady-state AUC was selected as the exposure measure from which to judge clinical relevance from a safety perspective, as this parameter is an integration of concentrations over the 24-h dosing interval in which individuals are exposed to doravirine at steady state. In phase I trials, there was no evidence that the incidence of overall AEs or specific AEs was temporally associated with doravirine Tmax [11, 15, 20–31], therefore suggesting that Cmax does not play a major role in safety or tolerability. In the phase IIb trial, there was no evidence of exposure- or dose-related toxicities across the range of 25–200 mg doses evaluated, and no meaningful association between doravirine steady-state exposure and the incidence of neuropsychiatric AEs or fasting lipid levels that would further limit the exposure of doravirine from a safety perspective [17].
Concomitant use of moderate and strong CYP3A inhibitors, which are expected to be associated with 2- to 3-fold higher doravirine exposures relative to the 100 mg once-daily dose, were not prohibited in the pivotal phase III DRIVE-FORWARD trial [5]; however, they were prohibited in the DRIVE-AHEAD trial due to potential interactions with the comparator regimen [4]. In the phase III trials, the 100 mg once-daily dose was generally well tolerated and no treatment-related AEs of concern were identified [4, 5].
For doravirine steady-state exposures at least 3-fold those observed with the 100 mg once-daily dose in the phase III trials, there were no safety signals in short-term clinical pharmacology trials. Specifically, multiple doses of up to 750 mg once daily for 10 days and single doses of up to 1200 mg doravirine [11] were generally well tolerated and provided exposure multiples of~
3.7- and
~
6.5-fold, respectively, relative to the steady-state AUC values observed following administration of 100 mg once-daily doravirine. In addition, a single dose of 1200 mg doravirine had no clinically meaningful effect on QTc values [32] at
~
4.3- and
~
3.3-fold exposure multiples to Cmax and AUC0–24, respectively. Thus, based on the totality of clinical experience for doravirine, increases in exposure up to
~
3-fold are not clinically meaningful.
Effect of Demographic Factors
The effects of demographic factors including age, gender, hepatic impairment, and renal impairment on doravirine pharmacokinetics were evaluated in dedicated phase I trials in participants without HIV-1 infection [22, 24, 25]. In addition, a population pharmacokinetic analysis of individuals with (n=
959) and without (n
=
341) HIV-1 infection was conducted across the phase I, II, and III trials to evaluate the effects of these factors—as well as body weight, body mass index (BMI), race, or ethnicity—on doravirine pharmacokinetics [14].
Age
Physiological changes that occur with aging can result in altered drug pharmacokinetics in older compared with younger people [33]. As the population of people living with HIV-1 is aging [34], evaluation of doravirine in the older population was essential.
The effect of age on doravirine pharmacokinetics was investigated in a phase I trial of healthy elderly men and women (aged 65–80 years) compared with healthy young men and women (18–50 years); results showed that age did not have a clinically meaningful effect on the pharmacokinetics of doravirine among these age groups as the observed changes in AUC0–∞, Cmax, and C24 were<
20% [22] (Table 1, Fig. 2). Similarly, in a population pharmacokinetic analysis [14], although age was identified as a significant covariate for doravirine clearance (AUC0–24 was 30% higher for individuals with HIV-1 infection aged
≥
65 years compared with those aged
<
65 years), the effect was consistent with the observations from the phase I trial, and not clinically meaningful as the increase falls within the clinical bounds described above. Similar findings were previously identified for other HIV treatments, where it was reported that age did not have a clinically relevant effect on the pharmacokinetics of boosted darunavir, dolutegravir, and lamivudine in people living with HIV, though boosted darunavir exposure was modestly increased in older people [35].
Table 1
Summary of the effect of demographic factors/disease/food on doravirine pharmacokinetics
Factor | Dosing | Comparison | Doravirine GMR (90% CI) | Reference | |||
---|---|---|---|---|---|---|---|
AUC0–∞ | AUC0–24 | Cmax | C24 | ||||
Age | Doravirine 100 mg SD | Elderly men/young men | 0.85 (0.67–1.10) | – | 0.92 (0.73–1.16) | 0.81 (0.59–1.11) | [22] |
Doravirine 100 mg SD | Elderly women/young women | 0.97 (0.79–1.19) | – | 1.18 (0.98–1.42) | 0.94 (0.72–1.21) | [22] | |
Gender | Doravirine 100 mg SD | Women/men | 1.20 (1.03–1.40) | – | 1.42 (1.23–1.64) | 1.02 (0.84–1.24) | [22] |
Hepatic impairment | Doravirine 100 mg SD | Moderate hepatic impairment/normal hepatic function | 0.99 (0.72–1.35) | 0.93 (0.74–1.18) | 0.90 (0.66–1.24) | 0.99 (0.74–1.33) | [25] |
Renal impairment | Doravirine 100 mg SD | Severe renal impairment/normal renal function | 1.43 (1.00–2.04) | – | 0.83 (0.61–1.15) | 1.38 (0.99–1.92) | [24] |
Food | Doravirine 100 mg SD | Fed/fasted | 1.16 (1.06–1.26) | – | 1.03 (0.89–1.19) | 1.36 (1.19–1.55) | [23] |
Doravirine 100 mg/lamivudine 300 mg/TDF 300 mg SD | Fed/fasted | 1.10 (1.01–1.20) | – | 0.95 (0.80–1.12) | 1.26 (1.13–1.41) | [23] |
AUC0–24 area under the concentration–time curve from time zero to 24 h, AUC0–∞ area under the concentration–time curve from time zero to infinity, C24 plasma concentration 24 h post-dose, CI confidence interval, Cmax maximum plasma concentration, GMR geometric mean ratio, SD single dose, TDF tenofovir disoproxil fumarate
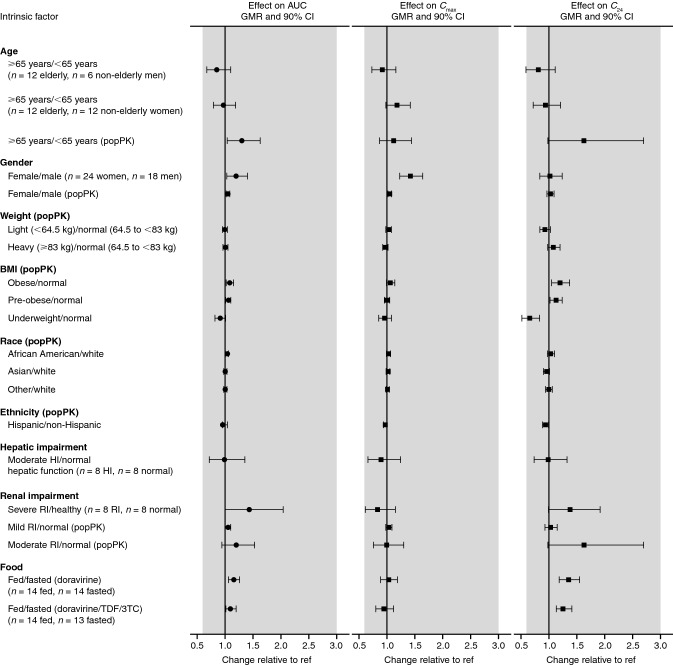
Visual summary of the effect of demographic factors/disease/food on doravirine exposure. Modified from Yee et al. [14]. Copyright © American Society for Microbiology, Antimicrob Agents Chemother, vol 63, 2019, e02502–18, DOI 10.1128/AAC.02502-18. 3TC lamivudine, AUC area under the concentration–time curve, BMI body mass index, C24 plasma concentration 24 h post-dose, Cmax maximum plasma concentration, CI confidence interval, GMR geometric mean ratio, HI hepatic impairment, popPK population pharmacokinetics, ref reference value, RI renal impairment, TDF tenofovir disoproxil fumarate. Shaded region represents the clinical bounds
Gender
Differences in drug absorption, distribution, metabolism, and excretion due to physiological differences between genders can result in pharmacokinetic differences [36]. CYP3A4, the enzyme primarily responsible for doravirine metabolism [10], has been shown in a meta-analysis to have significantly greater hepatic activity in women than in men, though no difference in intestinal CYP3A activity was observed [37]. However, the clinical relevance of this difference is unclear [37].
The effect of gender on doravirine pharmacokinetics was investigated in a trial comparing the pharmacokinetics between women and men [22] (Table 1, Fig. 2). Although Cmax was shown to be slightly higher (42%) in women than in men, any differences in pharmacokinetics due to gender were not considered clinically meaningful [22]. In line with these results, a population pharmacokinetic analysis [14] found no statistically significant effect of gender on doravirine pharmacokinetics. Taken together, the results do not indicate a need for dose adjustment based on gender.
Other Characteristics
A population pharmacokinetic analysis [14] also showed that there were no clinically meaningful effects on doravirine pharmacokinetics due to body weight, BMI, race, or ethnicity (Fig. 2). Race and ethnicity did not have any statistically significant effects on doravirine pharmacokinetics [14]. While weight (<64.5 kg, 64.5 <
83 kg,
≥
83 kg) was identified as a significant covariate, the magnitude of its effect was shown to be small (differences in AUC0–24, C24, and Cmax were all
<
10%, hence exposure was within the clinical bounds), as were differences in AUC0–24 and Cmax in individuals with a BMI defined as underweight (<
18.5 kg/m2), pre-obese (25.0–29.99 kg/m2), or obese (≥
30.0 kg/m2) compared with individuals in the normal range (18.5–24.99 kg/m2) [14]. Therefore, no dose adjustments for doravirine are required for any of these demographic factors.
Pharmacokinetics in Special Populations
Hepatic Impairment
Liver disease affects 6–11% of people living with HIV in the USA [38] and can result from a variety of causes, such as chronic hepatitis B or C infection, heavy alcohol consumption, and medication-induced toxicity [39]. Therefore, hepatic impairment can reasonably be expected to affect a sizeable proportion of people living with HIV; for those receiving antiretroviral therapy, this impairment may alter the pharmacokinetics of CYP3A-metabolized antiretroviral drugs [40]. A trial of doravirine in participants without HIV infection showed similar doravirine pharmacokinetics in the presence or absence of moderate hepatic impairment, and indicated that moderate hepatic impairment had no clinically meaningful effect on doravirine pharmacokinetics (Table 1, Fig. 2) and that dose adjustment is not required for individuals with moderate or mild hepatic impairment [25]. Doravirine was not studied in individuals with severe hepatic impairment [8, 9].
Renal Impairment
Chronic kidney disease affects 5–23% of people living with HIV in the USA [38], and may result from a number of causes, including HIV infection itself, co-morbidities such as diabetes, and toxicity associated with certain antiretroviral drugs [38, 41]. Given that doravirine has minimal renal excretion (<10%) [10, 11], renal impairment was not expected to have a clinically meaningful effect on doravirine pharmacokinetics. In a clinical trial [24], severe renal impairment was shown to have an effect on doravirine pharmacokinetics, moderately increasing exposure by 43% (Table 1, Fig. 2). Increased oral bioavailability, decreased clearance via decreased expression of gut/liver metabolic enzymes in rats, or enzyme inhibition by uremic toxins in individuals with severe renal impairment have been suggested to result in increased AUC, Cmax, and apparent terminal half-life of drugs eliminated via CYP3A-mediated metabolism [42]. However, the observed changes in doravirine pharmacokinetics were not anticipated to be clinically meaningful, as they fall within the clinical bounds of (0.6, 3.0) [24]. It was therefore concluded that doravirine may be administered to individuals with HIV-1 infection and mild, moderate, or severe renal impairment without dose adjustment [24]. This finding was supported by a population pharmacokinetic analysis that assessed the impact of renal function, as determined by the Modification of Diet in Renal Disease study estimated glomerular filtration rate, on doravirine pharmacokinetics across the phase I, II, and III population, and found no statistically significant effect of renal function on doravirine pharmacokinetics [14].
Food Effects
The NNRTIs etravirine and rilpivirine are recommended to be taken following and with a meal, respectively; exposure to each of these drugs is substantially decreased in a fasted versus a fed state [43, 44]. To investigate the effect of food on doravirine pharmacokinetics, doravirine was administered following a fast and following a high-fat, high-calorie meal, both alone and as part of a single-tablet regimen combined with 3TC and TDF [23]. Although doravirine AUC0–∞ and C24 were slightly increased in the fed state compared with fasted state, by 10–36%, these changes were not considered clinically meaningful (Table 1, Fig. 2), suggesting doravirine may be administered with or without food [23]. In both phase III trials, doravirine was administered without regard to meals [4, 5].
In Vitro Drug–Drug Interaction (DDI) Assessment
In vitro assessment confirmed that oxidative metabolism of doravirine is mediated mainly by CYP3A4, with some minor contribution by CYP3A5 [10]. As a result, doravirine pharmacokinetics may be altered in the presence of CYP3A modulators. Doravirine did not inhibit the activity of CYP1A2, 2B6, 2C8, 2C9, 2C19, 2D6, or 3A4, or uridinediphosphate glucuronosyltransferase (UGT)1A1 (IC50>
100 μM for all enzymes) [45], at concentrations well above the doravirine mean peak plasma concentration of 2.26 μM, which corresponds to a maximal unbound concentration of 0.54 μM. Additionally, doravirine does not induce CYP1A2 or CYP2B6 mRNA or enzyme activity in vitro [45], and although a low level of CYP3A4 mRNA induction was observed with 10 μM doravirine, no effect on CYP3A4 enzyme activity was observed and it was projected to have no clinically meaningful impact [45]. Doravirine is a substrate of P-gp in vitro; however, P-gp does not appear to have a significant role in the disposition of doravirine [10]. Doravirine is not a substrate of the breast cancer receptor protein (BCRP), organic anion-transporting polypeptide (OATP)1B1, or OATP1B3 [45], and, since renal excretion is not a major route of elimination of doravirine, it is therefore not likely to be affected by modulation of major hepatic or renal drug transporters [10]. In addition, doravirine is unlikely to cause DDIs via inhibition of major drug transporters, including P-gp, OATP1B1, OATP1B3, organic anion transporter (OAT)1, OAT3, organic cation transporter (OCT)2, multidrug and toxin extrusion protein (MATE)1, MATE2K, and bile salt extrusion pump (BSEP) [45]. Systemic exposure to doravirine is not likely to cause DDIs via inhibition of BRCP; however, with an IC50 value of 51 μM, inhibition of intestinal BCRP by doravirine is possible [45].
In Vivo DDI Assessment
Polypharmacy in individuals with HIV infection is common, and co-administration of antiretroviral drugs with drugs for treating co-morbidities of HIV-1 infection is often required, leading to the potential for DDIs [46]. One study estimated that potential DDIs occurred in approximately two-thirds of people receiving antiretroviral therapy for HIV infection; around one-quarter of these potential interactions were between antiretroviral medications themselves [46]. Common co-morbidities in individuals with HIV infection that require treatment include hepatitis C virus (HCV) infection, tuberculosis, diabetes, and hyperlipidemia [1, 38, 47].
The following sections discuss the clinical investigations of potential DDIs between doravirine and a range of drug classes likely to be co-administered, including concomitant antiretroviral drugs and medications for treating common co-morbidities. These findings are also extrapolated to other medications on the basis of known pharmacokinetic characteristics.
Doravirine and Antiretroviral Drugs for the Treatment of HIV Infection
Doravirine and Nucleoside Reverse Transcriptase Inhibitors (NRTIs)
To support the development of a single-tablet regimen with doravirine, two DDI trials were conducted with doravirine and two commonly used NRTIs, 3TC and TDF [31]. 3TC and tenofovir are primarily eliminated via organic cationic secretion, glomerular filtration, and active tubular secretion, pathways that are not affected by doravirine [48, 49]. Neither NRTI is expected to affect CYP metabolism [48, 49]. Consistent with the disposition of all three agents, the DDI trials demonstrated no clinically meaningful effect on doravirine, tenofovir, or 3TC pharmacokinetics (Tables 2 and and3,3, Figs. 3 and and4),4), supporting co-administration without the need for dose adjustment [31] and the co-formulation of these agents into a single-tablet regimen.
Table 2
Summary of doravirine drug–drug interactions: the effect of concomitant drugs on doravirine pharmacokinetics
Concomitant medication dosing | Doravirine dosing | Doravirine GMR (90% CI) [(doravirine![]() ![]() | Reference | |||
---|---|---|---|---|---|---|
AUC0–∞ | AUC0–24 | Cmax | C24 | |||
TDF 300 mg once daily | Doravirine 100 mg SD | 0.95 (0.80–1.12) | – | 0.80 (0.64–1.01) | 0.94 (0.78–1.12) | [31] |
TDF 300 mg![]() ![]() | Doravirine 100 mg SD | 0.96 (0.87–1.06) | – | 0.97 (0.88–1.07) | 0.94 (0.83–1.06) | [31] |
Efavirenz prior dosing 600 mg once daily | Doravirine 100 mg SD (Day 1 following efavirenz cessation) | – | 0.38 (0.33–0.45) | 0.65 (0.58–0.73) | 0.15 (0.10–0.23) | [15] |
Efavirenz prior dosing 600 mg once daily | Doravirine 100 mg once daily (Day 14 following efavirenz cessation) | – | 0.68 (0.58–0.80) | 0.86 (0.77–0.97) | 0.50 (0.39–0.64) | [15] |
Ritonavir 100 mg once daily | Doravirine 50 mg SD | 3.54 (3.04–4.11) | – | 1.31 (1.17–1.46) | 2.91 (2.33–3.62) | [26] |
Dolutegravir 50 mg once daily | Doravirine 200 mg once daily | – | 1.00 (0.89–1.12) | 1.06 (0.88–1.28) | 0.98 (0.88–1.09) | [20] |
Elbasvir 50 mg![]() ![]() | Doravirine 100 mg once daily | – | 1.56 (1.45–1.68) | 1.41 (1.25–1.58) | 1.61 (1.45–1.79) | [27] |
Ledipasvir 90 mg/sofosbuvir 400 mg SD | Doravirine 100 mg SD | 1.15 (1.07–1.24) | – | 1.11 (0.97–1.27) | 1.24 (1.13–1.36) | [27] |
Ketoconazole 400 mg once daily | Doravirine 100 mg SD | 3.06 (2.85–3.29) | – | 1.25 (1.05–1.49) | 2.75 (2.54–2.98) | [26] |
Rifampin 600 mg once daily | Doravirine 100 mg SD | 0.12 (0.10–0.15) | – | 0.43 (0.35–0.52) | 0.03 (0.02–0.04) | [74] |
Rifabutin 300 mg once daily | Doravirine 100 mg SD | 0.50 (0.45–0.55) | – | 0.99 (0.85–1.15) | 0.32 (0.28–0.35) | [75] |
Maintenance methadone 20–200 mg once daily | Doravirine 100 mg once daily | – | 0.74 (0.61–0.90) | 0.76 (0.63–0.91) | 0.80 (0.63–1.03) | [30] |
Antacid oral suspension (1600 mg aluminum hydroxide/1600 mg magnesium hydroxide/160 mg simethicone) SD | Doravirine 100 mg SD | 1.01 (0.92–1.11) | – | 0.86 (0.74–1.01) | 1.03 (0.94–1.12) | [28] |
Pantoprazole 40 mg once daily | Doravirine 100 mg SD | 0.83 (0.76–0.91) | – | 0.88 (0.76–1.01) | 0.84 (0.77–0.92) | [28] |
AUC0–24 area under the concentration–time curve from time zero to 24 h, AUC0–∞ area under the concentration–time curve from time zero to infinity, C24 plasma concentration 24 h post-dose, CI confidence interval, Cmax maximum plasma concentration, GMR geometric mean ratio, SD single dose, TDF tenofovir disoproxil fumarate
Table 3
Summary of doravirine drug–drug interactions: the effect of doravirine on the pharmacokinetics of concomitant drugs
Concomitant medication dosing | Doravirine dosing | Concomitant medication GMR (90% CI) [(doravirine![]() ![]() | Reference | |||
---|---|---|---|---|---|---|
AUC0–∞ | AUC0–24 | Cmax | C24 | |||
TDF 300 mg SD![]() ![]() | Doravirine 100 mg SD | Tenofovir: | ||||
1.11 (0.97–1.28) | – | 1.17 (0.96–1.42) | – | [31] | ||
Lamivudine: | ||||||
0.94 (0.88–1.00) | – | 0.92 (0.81–1.05) | – | [31] | ||
Midazolam 2 mg SD | Doravirine 120 mg once daily | 0.82 (0.70–0.97) | – | 1.02 (0.81–1.28) | – | [11] |
Dolutegravir 50 mg once daily | Doravirine 200 mg once daily | – | 1.36 (1.15–1.62) | 1.43 (1.20–1.71) | 1.27 (1.06–1.53) | [20] |
Elbasvir 50 mg once daily![]() ![]() | Doravirine 100 mg once daily | Elbasvir: | ||||
– | 0.96 (0.90–1.02) | 0.96 (0.91–1.01) | 0.96 (0.89–1.04) | [27] | ||
Grazoprevir: | ||||||
– | 1.07 (0.94–1.23) | 1.22 (1.01–1.47) | 0.90 (0.83–0.96) | [27] | ||
Ledipasvir 90 mg/sofosbuvir 400 mg SD | Doravirine 100 mg SD | Ledipasvir: | ||||
0.92 (0.80–1.06) | – | 0.91 (0.80–1.02) | – | [27] | ||
Sofosbuvir: | ||||||
1.04 (0.91–1.18) | – | 0.89 (0.79–1.00) | – | [27] | ||
GS-331007: | ||||||
1.03 (0.98–1.09) | – | 1.03 (0.97–1.09) | – | [27] | ||
Metformin 1000 mg SD | Doravirine 100 mg once daily | 0.94 (0.88–1.00) | – | 0.94 (0.86–1.03) | – | [29] |
Atorvastatin 20 mg SD | Doravirine 100 mg once daily | 0.98 (0.90–1.06) | – | 0.67 (0.52–0.85) | – | [21] |
Maintenance methadone 20–200 mg once daily | Doravirine 100 mg once daily | (R)-methadone: | ||||
– | 0.95 (0.90–1.01) | 0.98 (0.93–1.03) | 0.95 (0.88–1.03) | [30] | ||
(S)-methadone: | ||||||
– | 0.98 (0.90–1.06) | 0.97 (0.91–1.04) | 0.97 (0.86–1.10) | [30] | ||
Total methadone: | ||||||
– | 0.96 (0.90–1.03) | 0.98 (0.92–1.03) | 0.96 (0.87–1.05) | [30] | ||
Ethinyl estradiol 0.03 mg/levonorgestrel 0.15 mg SD | Doravirine 100 mg once daily | Ethinyl estradiol: | ||||
0.98 (0.94–1.03) | – | 0.83 (0.80–0.87) | – | [26] | ||
Levonorgestrel: | ||||||
1.21 (1.14–1.28) | – | 0.96 (0.88–1.05) | – | [26] |
AUC0–24 area under the concentration–time curve from time zero to 24 h, AUC0–∞ area under the concentration–time curve from time zero to infinity, C24 plasma concentration 24 h post-dose, CI confidence interval, Cmax maximum plasma concentration, GMR geometric mean ratio, SD single dose, TDF tenofovir disoproxil fumarate
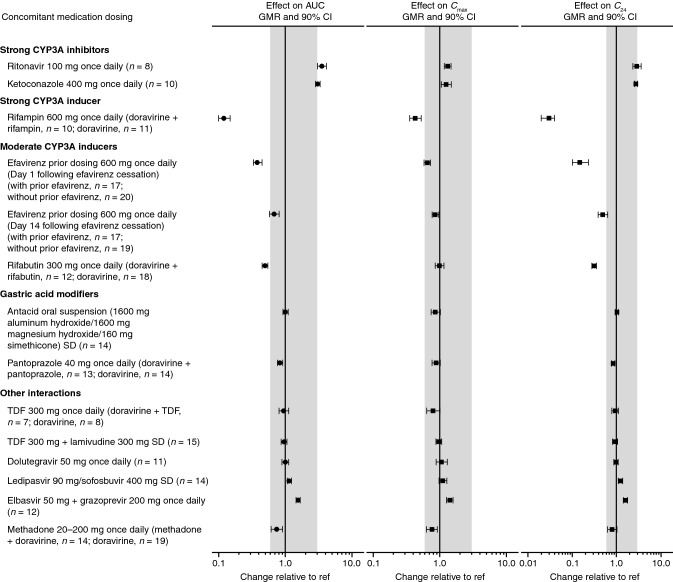
Visual summary of doravirine DDIs: the effect of concomitant drugs on doravirine exposure. AUC area under the concentration–time curve, C24 plasma concentration 24 h post-dose, Cmax maximum plasma concentration, CI confidence interval, DDI drug–drug interaction, GMR geometric mean ratio, ref reference value, SD single dose, TDF tenofovir disoproxil fumarate. Shaded region represents the clinical bounds
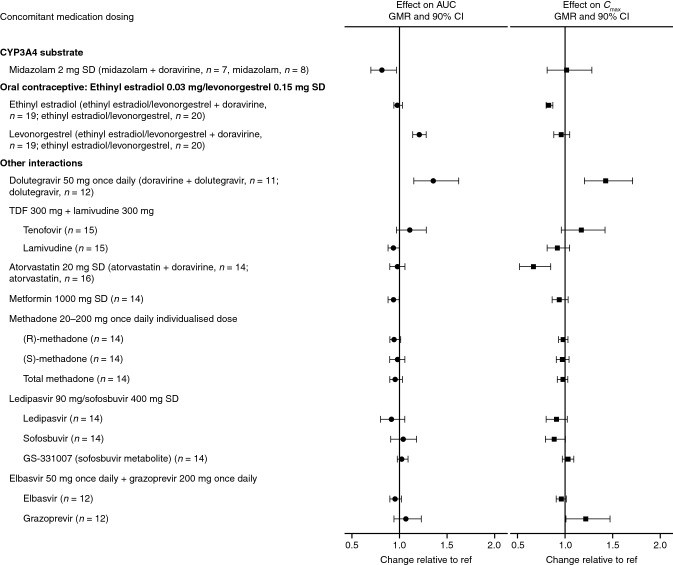
Visual summary of doravirine DDIs: the effect of doravirine on the exposure of concomitant drugs. AUC, area under the concentration–time curve, Cmax maximum plasma concentration, CI confidence interval, DDI drug–drug interaction, GMR geometric mean ratio, ref reference value, SD single dose, TDF tenofovir disoproxil fumarate
Abacavir and emtricitabine have no known interactions with CYP3A [3]. Abacavir is known to be a substrate of UGT1A1 and alcohol dehydrogenase, neither of which are likely to be impacted by doravirine [45]. Hence, interactions of abacavir or emtricitabine with doravirine are unlikely. Doravirine is not expected to cause any significant interactions with P-gp, BCRP, OATP1B1, and OATP1B3 [45], for which tenofovir alafenamide (TAF) is a substrate [50]. As the prescribing information for TAF does not suggest that it inhibits or induces CYP3A (TAF did not affect the pharmacokinetics of the sensitive CYP3A substrate midazolam) [50], a DDI with doravirine is not expected, and therefore doravirine and TAF may be co-administered. Furthermore, phase III trials have demonstrated the safety and efficacy of doravirine when co-administered with TDF and 3TC, or with other NRTIs such as emtricitabine, TAF, or abacavir [4, 5].
Doravirine and Non-Nucleoside Reverse Transcriptase Inhibitors (NNRTIs)
Due to associated psychiatric and central nervous system effects of the NNRTI efavirenz [51], doravirine may be a suitable alternative for individuals who have difficulties tolerating efavirenz. Efavirenz is a known CYP3A inducer, and in order to maintain viral suppression during regimen switch, the interaction of doravirine with residual efavirenz concentrations and the persistent CYP3A4 induction effects following cessation of efavirenz [52] should be considered. Doravirine exposure was transiently decreased in the days following a switch from efavirenz to doravirine in healthy adults (Table 2, Fig. 3) [15]. Following discontinuation of efavirenz, efavirenz concentrations exceeded the therapeutic threshold (1000 ng/mL [53]) until Day 3, and at this time doravirine concentrations exceeded the pharmacokinetic target for efficacy (six times the IC50) [15]; however, doravirine concentrations remained below the bounds for clinical efficacy through Day 14. Nevertheless, during this transient decrease in doravirine concentrations, dose adjustment is not considered necessary as individuals with HIV-1 infection are expected to be virologically suppressed prior to switch and will continue to receive other antiretroviral agents during switch, which supports the low likelihood of viral rebound or development of viral resistance [15]. Switching to a doravirine-based regimen was assessed in a phase III trial (DRIVE-SHIFT; ClinicalTrials.gov NCT02397096) that evaluated switching from a stable antiretroviral regimen to a single-tablet regimen of doravirine 100 mg with 3TC 300 mg and TDF 300 mg (DOR/TDF/3TC) [54]. In this trial, a similar high level of antiretroviral efficacy of DOR/3TC/TDF was observed in participants who switched from an efavirenz-based regimen and those who switched from another baseline regimen, showing that the transient decrease in doravirine concentration is not clinically meaningful [55].
As doravirine was shown not to be a significant inducer or inhibitor of a number of CYP enzymes in vitro [45], and in vivo doravirine did not significantly impact the pharmacokinetics of midazolam (Table 3) [11], doravirine is not expected to cause clinically meaningful DDIs with etravirine, which is a substrate for CYP3A, CYP2C9, and CYP2C19 enzymes [43], or with nevirapine, which is a substrate for CYP3A and CYP2B6 enzymes [56]. Etravirine and nevirapine are also inducers of CYP3A4 [3]. Based on the transient reduced exposure of doravirine following switch from efavirenz (a CYP3A inducer) [15], there is potential that switch from etravirine or nevirapine would result in a minor inductive effect on doravirine pharmacokinetics. However, based on the shorter half-lives of these agents [43, 51, 56], it is unlikely that the duration of effect would exceed that of efavirenz, and therefore switch from etravirine or nevirapine to doravirine is also supported. Rilpivirine does not induce or inhibit CYP3A4, and although it is a substrate for CYP3A4 [3], it is unlikely to result in DDIs with doravirine, similar to other CYP3A substrates [26].
Doravirine and HIV-1 Boosted and Unboosted Protease Inhibitors (PIs)
Ritonavir is an HIV-1 PI commonly used as a pharmacokinetic-enhancing agent for other HIV-1 drugs metabolized by CYP3A4, including other PIs [3, 57]. Because it is a strong CYP3A4 inhibitor, ritonavir increases the exposure of drugs—and “boosts” activity—via inhibition of CYP3A4-mediated drug metabolism [57]. Although ritonavir is not anticipated to be used as a pharmacokinetic enhancer for doravirine, the interaction between ritonavir and doravirine was evaluated in the event that doravirine is administered as part of a boosted-PI regimen or used concomitantly with other strong CYP3A inhibitors. Co-administration of doravirine and ritonavir increased doravirine exposure~
3-fold compared with doravirine alone (Table 2, Fig. 3), consistent with the inhibition of CYP3A4 metabolism, the primary clearance pathway of doravirine [10, 26]. However, Cmax increased only by
~
30%. In accordance with the clinical bounds described above, these changes in doravirine pharmacokinetics were not considered clinically meaningful, and no dose adjustment is required for co-administration of doravirine and ritonavir [26].
Cobicistat is a strong CYP3A inhibitor used as a pharmacokinetic enhancer for the PIs atazanavir and darunavir [58]. As the CYP3A inhibitor effect is expected to be similar to ritonavir [59], a~
3 fold increase in doravirine exposure is expected when the two agents are co-administered.
Several other HIV-1 boosted or unboosted PIs have potential for perpetrating DDIs through modulation of various enzymes and/or transporters, including CYP3A, OATP, UGT1A1, and P-gp [3]. However, the magnitude of CYP3A inhibition for atazanavir, darunavir, indinavir, and saquinavir is not likely to be greater than that observed for ritonavir, and lopinavir is not a CYP3A inhibitor [59]. Therefore, these agents are unlikely to have a clinically meaningful effect on doravirine pharmacokinetics, despite being CYP3A4 substrates and/or inhibitors [3]. While lopinavir is considered a moderate inducer when given alone, it is only available for use in combination with ritonavir, and in this setting boosted lopinavir behaves as a CYP3A4 inhibitor, in addition to being a CYP3A4 substrate [3]. Similarly, while tipranavir is considered a CYP3A4 inducer, it is only recommended for use with ritonavir, and the net effect of these two agents is CYP3A4 inhibition [3]. As doravirine may be concomitantly administered with strong CYP3A inhibitors without dose adjustment [26], a dose adjustment would not be required for boosted tipranavir. Fosamprenavir, the prodrug of amprenavir, is considered a moderate CYP3A inhibitor and weak inducer [3], and therefore the net effect on doravirine exposure following co-administration is unknown. However, if this agent is used in combination with a pharmacokinetic enhancer, it is likely that there would be a net increase in doravirine exposure.
Doravirine and Integrase Strand Transfer Inhibitors (InSTIs)
In some instances, an NNRTI may be co-administered with an InSTI [3], and the combination of NNRTIs and InSTIs may offer an alternative to NRTI-based therapies [60]. Dolutegravir has shown good efficacy and tolerability along with a high barrier to resistance [20]; in vitro, low potential for DDIs was reported [61]. It is metabolized by UGT1A1/1A3/1A9 and CYP3A4, and is a substrate for P-gp and BCRP [61]. Although a weak inhibitor of CYP3A in vitro, dolutegravir does not inhibit or induce CYP3A in vivo [62]. In line with this finding, the pharmacokinetics of doravirine (200 mg once daily) in healthy subjects were shown to be similar in the presence or absence of dolutegravir (50 mg once daily) (Table 2, Fig. 4) [20]. The modest increases in dolutegravir AUC0–24, Cmax, and C24 observed at a supratherapeutic doravirine dose of 200 mg once daily, thought to result from inhibition of BCRP in the gut by doravirine, were considered not clinically meaningful (Table 3, Fig. 4) [20].
Other InSTIs are unlikely to interact with doravirine based on their known metabolism or elimination pathways and their propensity to modulate major CYPs and transporters. For example, elvitegravir is a UGT1A1 and CYP3A4 substrate and CYP2C9 inhibitor, while raltegravir is a UGT1A1 substrate [3]; therefore, neither drug is likely to affect CYP3A-mediated metabolism of doravirine via induction or inhibition of CYP3A4. Elvitegravir may be boosted with either cobicistat or ritonavir [3] and, as discussed above, the increases in doravirine expected with these agents are not considered clinically meaningful.
Doravirine and Anti-Hepatitis C Virus (HCV) Drugs
People living with HIV infection are almost six times more likely to be infected with HCV than people without HIV infection [47]. HIV/HCV co-infection rates are particularly high in people who inject drugs; an estimated 82% of people living with HIV and who inject drugs are co-infected with HCV [47]. Consequently, co-administration of medications for these two viral infections is often required. The drug interaction potential following co-administration of doravirine with two anti-HCV treatments, elbasvir/grazoprevir and ledipasvir/sofosbuvir, has been evaluated [27]. Elbasvir and grazoprevir are CYP3A and P-gp substrates and grazoprevir is also a substrate for OATP1B1/B3. Elbasvir and grazoprevir are partially eliminated via CYP3A-mediated oxidative metabolism, and are primarily (>90%) eliminated in feces [63]. Ledipasvir and sofosbuvir are P-gp and BCRP substrates [64]. Ledipasvir is eliminated primarily in feces as the unchanged drug (70%), indicating that biliary excretion is the major route of elimination, while sofosbuvir is extensively metabolized in the liver to the active entity GS-461203, then eventually to the dephosphorylated metabolite GS331007. Renal clearance of GS-331007 is the main elimination pathway of sofosbuvir, accounting for 78% of the dose [64].
Increases in doravirine exposure following co-administration with either elbasvir/grazoprevir (56%) or ledipasvir/sofosbuvir (15%) were observed (Table 2, Fig. 3), but were within the established clinical bounds for doravirine, and hence not considered to be clinically significant [27]. Grazoprevir, but not elbasvir, is a weak inhibitor of CYP3A [63]; thus, the observed modest increase in doravirine exposure is likely due to inhibition of CYP3A [27]. Small (10–25%) increases in doravirine AUC0–∞, C24, and Cmax seen following co-administration of ledipasvir/sofosbuvir with doravirine were thought to result from inhibition of P-gp by ledipasvir [64]; the high permeability of doravirine limits the effect of P-gp on its absorption [10], so that the effect of P-gp inhibitors on doravirine pharmacokinetics is small. Meanwhile, doravirine had no clinically meaningful effect on the pharmacokinetics of elbasvir, grazoprevir, ledipasvir, sofosbuvir, or the sofosbuvir metabolite GS-331007 (Tables 2 and and3,3, Fig. 4), which supports the limited effects of doravirine on the transporters involved in uptake of these drugs, despite doravirine being a known weak inhibitor of BCRP [27, 45]. Therefore, antiviral treatment of HIV/HCV co-infection with doravirine and elbasvir/grazoprevir or ledipasvir/sofosbuvir is a viable option [27].
Similarly, other anti-HCV direct-acting antivirals are anticipated to have minimal effect on doravirine pharmacokinetics. Several are known to be CYP3A4 substrates (e.g., ombitasvir, daclatasvir, paritaprevir, velpatasvir, voxilaprevir), or substrates and weak inhibitors (e.g., simeprevir, glecaprevir) [65]; based on other clinical trials of doravirine, clinically meaningful DDIs with doravirine are unlikely with substrates or inhibitors of CYP3A [26].
Doravirine and Antifungal Agents
Antifungal agents are vital for treating opportunistic infections that can occur in people living with HIV [66]. Ketoconazole is a broad-spectrum antifungal agent that is a strong CYP3A4 inhibitor [59, 67], a CYP3A4 substrate [68], and a potent inhibitor of UGT1A1 [67] and P-gp [69]. As expected, doravirine exposure was increased following co-administration of ketoconazole with doravirine compared with doravirine alone (Table 2, Fig. 3), likely due to reduced CYP3A-mediated clearance of doravirine following CYP3A4 inhibition by ketoconazole [26]. However, the~
3-fold increase in AUC0–∞ was accompanied by only a 25% increase in Cmax, despite P-gp inhibition by ketoconazole, further supporting that the increased exposure of doravirine was due to decreased metabolism by CYP3A rather than P-gp inhibition [26]. Overall, the observed changes to doravirine pharmacokinetics were not considered clinically meaningful in the context of the clinical trial experience with doravirine [11, 26, 32]; therefore, co-administration of doravirine with ketoconazole or other strong CYP3A4 inhibitors is supported [26].
Like ketoconazole, several other azole antifungals are inhibitors of CYP3A4, including fluconazole, itraconazole, posaconazole, and voriconazole [70]. However, based on the finding above that ketoconazole, as a strong CYP3A4 inhibitor, does not lead to clinically meaningful changes in doravirine pharmacokinetics, co-administration of doravirine with other azoles will not necessitate dose adjustment [8, 9].
Doravirine and Antimycobacterial Agents
Tuberculosis remains the leading cause of death in people living with HIV [1]. The combination of antiretroviral and tuberculosis treatments has averted an estimated 9 million deaths from 2000 to 2016 [71]. Rifampin is a first-line agent for the initial treatment of tuberculosis in people living with HIV, taken in combination with isoniazid, ethambutol, and pyrazinamide [72]. As a strong CYP3A inducer [73], rifampin is known to have clinically significant DDIs with a number of antiretroviral drugs [72]. Consistent with the strong induction of CYP3A4 by rifampin, doravirine exposure was reduced by 88% and C24 was reduced by 97% following co-administration with multiple-dose rifampin in healthy adult men (Table 2, Fig. 3), indicating that the efficacy of doravirine when co-administered with rifampin in individuals with HIV/tuberculosis co-infection would be substantially compromised [74]. As a result, co-administration of rifampin with doravirine is contraindicated [8, 9]. Rifabutin is another member of the rifamycin class of antimycobacterials but is a less potent CYP3A inducer than rifampin [73]. Doravirine exposure in healthy adults was also reduced following co-administration with rifabutin but to a lesser extent than with rifampin (50% reduction; Table 2, Fig. 3) [75]. Based on projections from doravirine pharmacokinetics in the presence of rifabutin, the interaction is mitigated by increasing the doravirine dose from 100 mg once daily to 100 mg twice daily [75]. As such, dose adjustment of doravirine to 100 mg twice daily is recommended for co-administration with rifabutin [8, 9], allowing doravirine to be used in HIV/tuberculosis co-infection treatment.
The other components of first-line therapy for tuberculosis include isoniazid, ethambutol, and pyrazinamide. Isoniazid metabolism results mainly from the actions of N-acetyltransferase 2 and amidase, and there is evidence of interactions with various CYPs, including inhibition of CYP3A4 [76, 77]. Ethambutol was shown to inhibit a number of CYPs but had only a weak inhibitory effect on CYP3A [78]. As no dose adjustment is required for the concomitant use of doravirine and strong CYP3A inhibitors [26], interactions between ethambutol or isoniazid and doravirine are not expected to be clinically meaningful. Pyrazinamide was shown to have no inhibitory effect on CYP enzymes, including CYP3A4 [77].
Doravirine and Antidiabetic Drugs
Type 2 diabetes mellitus is a common co-morbidity in people with HIV-1 infection [38]. Metformin is the recommended first-line therapy [79], and therefore concomitant administration with doravirine may be required. Co-administration of doravirine and metformin had no clinically meaningful effect on metformin pharmacokinetics (Table 3, Fig. 4) [29]. Metformin is excreted unchanged in urine [80] and therefore not dependent on CYP3A-mediated metabolism. The lack of interaction of doravirine with metformin demonstrates that it has no clinically meaningful effect on the transporters involved in uptake and transport of metformin in vivo [29]—namely PMAT, OCT1, OCT2, OCT3, MATE1, and MATE2 K [80, 81]—and confirms in vitro findings that doravirine has low potential for interactions with OCT2 and MATE1/2K [45].
Overall, it is anticipated that doravirine has low potential for interaction with antidiabetic drugs. Most clinically meaningful DDIs of antidiabetic drugs result from inhibition or induction of hepatic CYPs [82]. Sulfonylureas are metabolized by various CYP enzymes, including CYP2C9 and CYP3A4 [82]. Due to a lack of modulation of these enzymes by doravirine, interactions are thought to be unlikely. In addition, these agents are not perpetrators of CYP3A-mediated DDIs, and therefore would not have a clinically meaningful effect on doravirine pharmacokinetics. It should be noted that glyburide is a weak inhibitor of CYP3A [83]; however, a clinically meaningful interaction with doravirine would not be anticipated. Dipeptidyl peptidase-4 inhibitors, with the exception of saxagliptin, have no known DDIs with commonly used concomitant medications [82]. Saxagliptin is a CYP3A4/5 substrate and may be affected by inducers and inhibitors. As with sulfonylureas, saxagliptin is unlikely to be affected by doravirine via this pathway. Sitagliptin and saxagliptin are not CYP3A inhibitors or inducers, and would therefore not be expected to affect the pharmacokinetics of doravirine [84, 85].
The sodium-glucose co-transporter (SGLT)-2 inhibitors ertugliflozin, empagliflozin, dapagliflozin, and canagliflozin undergo hepatic glucuronidation and are not cleared to a major extent by CYP enzymes [82]. Canagliflozin requires dose adjustment when co-administered with UGT inducers; however, no other clinically meaningful DDIs of SGLT-2 inhibitors have been identified [82]. Therefore, doravirine and SGLT-2 inhibitors can be co-administered without interactions. Pioglitazone and rosiglitazone, members of the thiazolidinedione drug class, are metabolized mainly via CYP2C8, with lesser contributions of CYP3A4 and CYP2C9 metabolism, respectively [82], and are therefore unlikely to be affected by doravirine. While doravirine is not expected to affect the metabolism of pioglitazone, pioglitazone is a weak CYP3A inducer [59], and therefore doravirine concentrations may potentially be slightly reduced with co-administration.
Doravirine is not anticipated to affect the pharmacokinetics of liraglutide, a subcutaneously administered glucagon-like peptide-1 (GLP1) receptor agonist, since liraglutide metabolism does not rely on a specific organ for elimination and is instead endogenously metabolized in a similar fashion to large proteins [86]. Liraglutide has been shown to alter absorption of concomitantly administered oral agents through delayed gastric emptying [86]. However, liraglutide did not affect the absorption of tested drugs to a clinically meaningful degree: AUC changes for digoxin, lisinopril, atorvastatin, acetaminophen, and ethinyl estradiol/levonorgestrel ranged from a decrease of 16% to an increase of 18% [86]. The effect of liraglutide on doravirine, a Biopharmaceutical Classification System Class II compound, is therefore not expected to be clinically meaningful.
Doravirine and Statins
The 3-hydroxy-3-methylglutaryl coenzyme A reductase inhibitors (statins) are used for the treatment of hypercholesterolemia, a common co-morbidity in individuals with HIV infection [87]. Doravirine had no clinically meaningful effect on atorvastatin pharmacokinetics following co-administration (Table 3, Fig. 4) [21]. Although the Cmax of atorvastatin was decreased by 33% with co-administration, the difference was not considered clinically meaningful due to efficacy being demonstrated in the presence of other factors that caused similar atorvastatin pharmacokinetic changes [21, 88, 89]. These results indicated that doravirine does not meaningfully impact the activity of CYP3A4, OATP1B1, P-gp, or BCRP, for which atorvastatin is a substrate [21]. As simvastatin [90], pravastatin [91], rosuvastatin [92], and lovastatin [93] are also substrates for one or more of these pathways, doravirine is not anticipated to have a clinically meaningful effect on the pharmacokinetics of these statins. Additionally, fluvastatin [94] is also a substrate for CYP2C9 while pitavastatin is a substrate for OATP1B1/3 and primarily metabolized by UGT1A3 and UGT2B7 [95, 96]. As in vitro data support that doravirine is not a modulator of CYP2C9 and is unlikely to modulate UGT enzymes [45], doravirine is also not expected to affect the disposition of fluvastatin or pitavastatin. Furthermore, to the best of our knowledge, none of these statins are known to be clinically meaningful perpetrators of DDIs via CYP3A-mediated metabolism [59], and are therefore not expected to have a clinically meaningful effect on the pharmacokinetics of doravirine. Therefore, doravirine may be co-administered with atorvastatin, fluvastatin, lovastatin, pravastatin, pitavastatin, or simvastatin, without dose adjustment of doravirine or any of these statins.
Doravirine and Opioid Substitution Therapies
Injection drug use was responsible for~
6% of new diagnoses of HIV infection in the USA in 2016 [97], and
~
2.1% of people living with HIV in the USA reported recent opiate use in a 2013 study [98]. Methadone, an opioid substitution therapy widely used to treat opioid addiction [99], is often co-administered with HIV antiretroviral therapy [100]. Potential interactions between methadone and doravirine were investigated in individuals participating in a methadone maintenance program. Methadone pharmacokinetics (for (R)-, (S)-, and total methadone) were not meaningfully affected with doravirine co-administration (Table 3, Fig. 4) [30]. Comparison with data from a previous trial in participants receiving doravirine without methadone co-administration [15] revealed that doravirine exposure was modestly reduced by 26% following co-administration with methadone (Table 2, Fig. 3). The mechanism for this reduction is not clear, although it was suggested that reduced gut motility limiting doravirine absorption may be responsible [30]. However, the changes were not considered clinically meaningful on the basis of the established clinical bounds for doravirine [30].
Another treatment for opioid dependence is buprenorphine/naloxone [101]. CYP3A is important in the metabolism of buprenorphine but would not be affected by co-administration of doravirine, and naloxone does not undergo CYP-mediated metabolism. While buprenorphine is a CYP3A4 and CYP2D6 inhibitor in vitro, DDIs are not a concern as therapeutic concentrations are expected to be too low to perpetrate clinically meaningful DDIs [101]. Therefore, doravirine and buprenorphine/naloxone may be co-administered without dose adjustment.
Doravirine and Oral Contraceptives
The use of hormonal contraceptives is anticipated in the doravirine target population. Ethinyl estradiol 0.03 mg/levonorgestrel 0.15 mg is a fixed-dose oral contraceptive [102] and both components are CYP3A substrates [103, 104]. No clinically meaningful changes to the pharmacokinetics of ethinyl estradiol or levonorgestrel were observed following co-administration with doravirine (Table 3, Fig. 4). Although levonorgestrel AUC0–∞ was slightly elevated, this increase was not considered clinically meaningful on the basis of levonorgestrel exposures observed in other marketed contraceptives containing the same dose of levonorgestrel [26]. Hence, it was concluded that dose adjustment of ethinyl estradiol and levonorgestrel are not required for co-administration with doravirine [26].
Doravirine and Antacids
Gastric acid-reducing agents are commonly used by people living with HIV-1 [105]. However, some antiretroviral drugs are restricted or contraindicated for use with such agents due to impaired absorption leading to reductions in plasma concentrations of the anti-HIV drugs [3]. Co-administration of either an aluminum-/magnesium-containing antacid or the proton-pump inhibitor pantoprazole with doravirine did not have a clinically meaningful effect on doravirine pharmacokinetics (Table 2, Fig. 3) [28]. Slight decreases (12–17%) in doravirine AUC0–∞, Cmax, and C24 following co-administration with pantoprazole, and in doravirine Cmax following co-administration with an antacid, were not considered clinically meaningful on the basis of the established clinical bounds for doravirine. Gastric acid-reducing agents may be co-administered with doravirine without need for dose adjustments or restrictions [28].
Clinical Perspective
The population living with HIV is clinically and demographically diverse and has changed over time [1, 34, 38]. The presence of co-morbidities has increased in recent years [38], and with it comes the likelihood of polypharmacy and potential for DDIs with antiretroviral drugs [46]. The success of antiretroviral therapy has led to improvements in life expectancy, resulting in an aging population living with HIV [34]. Chronic kidney disease [41], hepatic disease [39], and cardiovascular disease [38] are complications commonly experienced by people living with HIV, so consideration of these populations may be particularly important. Doravirine is a novel NNRTI that has pharmacokinetic properties that support once-daily administration and address the complex HIV patient population needs. The clinical trials and population pharmacokinetic analysis described here support the use of doravirine across a wide range of populations with HIV-1 infection: age, gender, race, ethnicity, weight/BMI, renal function, and moderate hepatic impairment did not have an appreciable effect on doravirine pharmacokinetics, nor did food (fed or fasted) have a clinically meaningful impact [14, 22–25].
Doravirine was shown to have limited potential for clinically meaningful DDIs based on data obtained from in vitro studies of drug-metabolizing enzymes and drug transporters [10, 45] and clinical studies [15, 20, 21, 26–31]. Since doravirine is a substrate of CYP3A4 [10], the most likely route of its involvement in DDIs is via inhibition and induction of CYP3A activity by strong and moderate CYP3A inhibitors/inducers. CYP3A inhibition does not result in a clinically meaningful change in doravirine pharmacokinetics, and doravirine can be co-administered with these agents without dose adjustment. However, moderate and strong CYP3A inducers reduced doravirine exposure. For strong inducers, the magnitude of the decrease may impact efficacy; therefore, doravirine is contraindicated for use with any strong CYP3A inducer (e.g., rifampin, mitotane, avasimibe, phenytoin, carbamazepine, and enzalutamide) [8, 9, 74]. Similar restrictions for rifampin are also in place for other antiretroviral medications, including all PIs and several NNRTIs (including etravirine, nevirapine, and rilpivirine) [3]. TAF and cobicistat-boosted elvitegravir are contraindicated for both rifampin and rifabutin [3]. Dose adjustment of doravirine is recommended for the moderate inducer rifabutin [75]. As the magnitude of induction associated with CYP3A moderate inducers varies across compounds (e.g., etravirine, nevirapine, bosentan, nafcillin, and modafinil), and is also dependent on the substrate, the effect of other moderate inducers on the pharmacokinetics of doravirine cannot easily be predicted. However, if co-administration with moderate inducers cannot be avoided, doravirine dosing frequency should be increased from 100 mg once daily to 100 mg twice daily. A fixed-dose combination of doravirine with 3TC and TDF at the recommended adult doses of 100 mg doravirine and 300 mg each of 3TC and TDF is also available [9]. While the pharmacokinetic characteristics of doravirine support the administration of 100 mg doravirine with minimal restrictions due to intrinsic factors and DDIs, dose recommendations for the fixed-dose combination may be influenced by the effect of intrinsic factors, such as renal impairment, on the pharmacokinetic profiles of 3TC and/or TDF, which are primarily renally cleared [9]. The use of TDF has also been associated with the onset or worsening of renal impairment in some patients [9]. Additionally, drug interactions specific to 3TC or TDF may also result in recommendations of additional monitoring for AEs related to increases in concentration of the 3TC or TDF component, as in the case of co-administration of the fixed-dose combination with ledipasvir/sofosbuvir [9].
The collective data demonstrate that the overall DDI profile of doravirine allows the co-administration of doravirine with a diverse range of concomitant medications and may offer advantages over existing antiretroviral therapies. For example, several boosted PIs, including ritonavir-boosted atazanavir, ritonavir-boosted darunavir, and ritonavir-boosted lopinavir, are contraindicated with elbasvir/grazoprevir due to OATP1B1/3 inhibition leading to increased plasma grazoprevir concentrations [3]; efavirenz and etravirine/nevirapine are also contraindicated with elbasvir/grazoprevir due to the resulting decrease in elbasvir and grazoprevir exposures, and ritonavir-boosted tipranavir is expected to reduce ledipasvir and sofosbuvir concentrations [3]. Rilpivirine is contraindicated with proton-pump inhibitors, and raltegravir should not be co-administered with aluminum-/magnesium-containing hydroxide antacids [3]. Meanwhile, care must be taken when co-administering cobicistat-boosted elvitegravir with atorvastatin, and dolutegravir with metformin [3]. The broad suitability of doravirine in the presence of concomitant medications therefore offers freedom from some of the restrictions associated with other antiretroviral regimens and provides additional flexibility in managing polypharmacy. Similar conclusions have been drawn by other authors [106, 107].
As doravirine will be co-administered with other antiretroviral agents, the favorable drug-interaction profile supports the combination of doravirine with the most commonly used drugs, including 3TC and TDF [31]. These results contrast with the alterations in pharmacokinetics seen with other agents. For example, when TDF is co-administered with ritonavir-boosted atazanavir, decreased atazanavir exposure occurred [108]. Switching from a PI-, NNRTI-, or InSTI-based regimen to a doravirine-based regimen is also an important consideration. Phase III results supported a switch from commonly used regimens to doravirine [54], and thus doravirine has been approved to replace the current antiretroviral therapy in appropriate patients who are virologically suppressed on their current regimen [8, 9]. Based on drug interaction properties of other antiretroviral agents, this ease of switch may not be feasible for other drugs.
While it should be taken into consideration that data to date have been generated either under strictly controlled phase I trial conditions or in well-controlled phase II and phase III trials, which began to emulate real-world conditions but still included several restrictions for inclusion and exclusion, the safety, tolerability, and efficacy profile of doravirine is expected to evolve with its expanded use in clinical settings as a greater number of patients with co-morbidities and polypharmacy are treated with doravirine-based regimens. However, given the overall pharmacokinetic profile of doravirine and the benefits it brings compared with existing treatment options, it is expected that doravirine has the potential to become a valuable addition to the available treatments for HIV-1 infection across a diverse range of populations living with HIV.
Conclusions
In summary, evaluation of published clinical pharmacokinetics trials of doravirine demonstrates a low potential for DDIs with doravirine and limited impact of patient characteristics. Although doravirine is contraindicated for co-administration with strong CYP3A inducers and requires dose adjustment for co-administration with the moderate CYP3A inducer rifabutin, DDIs with other drugs are unlikely. As a result, its pharmacological profile makes doravirine a potential treatment option for a broad range of people living with HIV, including older populations and those with co-morbidities requiring the use of concomitant medications.
Electronic supplementary material
Below is the link to the electronic supplementary material.
Acknowledgements
Medical writing assistance, under the direction of the authors, was provided by Kirsty Muirhead, PhD, of CMC AFFINITY, McCann Health Medical Communications, in accordance with Good Publication Practice (GPP3) guidelines. This assistance was funded by Merck Sharp & Dohme Corp., a subsidiary of Merck & Co., Inc., Kenilworth, NJ, USA.
Author Contributions
MI, SK, SAS, and KLY designed the research. MI, SK, RS, SAS, LW. and KLY analyzed/interpreted the data. MI, SK, RS, SAS, LW, and KLY wrote or revised the manuscript.
Compliance with Ethical Standards
Funding for this research was provided by Merck Sharp & Dohme Corp., a subsidiary of Merck & Co., Inc., Kenilworth, NJ, USA. Medical writing assistance was funded by Merck Sharp & Dohme Corp., a subsidiary of Merck & Co., Inc., Kenilworth, NJ, USA.
SK, KLY, RS, SAS, LW, and MI are current employees of Merck Sharp & Dohme Corp., a subsidiary of Merck & Co., Inc., Kenilworth, NJ, USA, and may own stock and/or hold stock options in the Merck & Co., Inc., Kenilworth, NJ, USA.
References
Full text links
Read article at publisher's site: https://doi.org/10.1007/s40261-020-00934-2
Read article for free, from open access legal sources, via Unpaywall:
https://link.springer.com/content/pdf/10.1007/s40261-020-00934-2.pdf
Citations & impact
Impact metrics
Citations of article over time
Article citations
Efficacy, Safety, and Tolerability of Doravirine/Lamivudine/Tenofovir Disoproxil Fumarate Fixed-Dose Combination Tablets in Adolescents Living With HIV: Results Through Week 96 from IMPAACT 2014.
J Pediatric Infect Dis Soc, 12(12):602-609, 01 Dec 2023
Cited by: 0 articles | PMID: 37815035 | PMCID: PMC11494224
Clinical Relevance of Drug Interactions in People Living with Human Immunodeficiency Virus on Antiretroviral Therapy-Update 2022: Systematic Review.
Pharmaceutics, 15(10):2488, 18 Oct 2023
Cited by: 2 articles | PMID: 37896248 | PMCID: PMC10610003
Review Free full text in Europe PMC
Microphysiological model reveals the promise of memory-like natural killer cell immunotherapy for HIV± cancer.
Nat Commun, 14(1):6681, 21 Oct 2023
Cited by: 2 articles | PMID: 37865647 | PMCID: PMC10590421
Synthesis and Biological Evaluation of Benzothiazolyl-pyridine Hybrids as New Antiviral Agents against H5N1 Bird Flu and SARS-COV-2 Viruses.
ACS Omega, 8(40):36636-36654, 25 Sep 2023
Cited by: 1 article | PMID: 37841136 | PMCID: PMC10568744
Diffuse Large B-Cell Lymphoma in the HIV Setting.
Cancers (Basel), 15(12):3191, 15 Jun 2023
Cited by: 5 articles | PMID: 37370801 | PMCID: PMC10296551
Review Free full text in Europe PMC
Go to all (9) article citations
Data
Data behind the article
This data has been text mined from the article, or deposited into data resources.
BioStudies: supplemental material and supporting data
Clinical Trials (3)
- (1 citation) ClinicalTrials.gov - NCT02397096
- (1 citation) ClinicalTrials.gov - NCT02275780
- (1 citation) ClinicalTrials.gov - NCT02403674
Similar Articles
To arrive at the top five similar articles we use a word-weighted algorithm to compare words from the Title and Abstract of each citation.
Clinical Pharmacodynamics, Pharmacokinetics, and Drug Interaction Profile of Doravirine.
Clin Pharmacokinet, 58(12):1553-1565, 01 Dec 2019
Cited by: 11 articles | PMID: 31388941
Review
Safety, tolerability and pharmacokinetics of doravirine, a novel HIV non-nucleoside reverse transcriptase inhibitor, after single and multiple doses in healthy subjects.
Antivir Ther, 20(4):397-405, 03 Dec 2014
Cited by: 36 articles | PMID: 25470746
The Effect of Single and Multiple Doses of Rifampin on the Pharmacokinetics of Doravirine in Healthy Subjects.
Clin Drug Investig, 37(7):659-667, 01 Jul 2017
Cited by: 27 articles | PMID: 28353169
Doravirine and the Potential for CYP3A-Mediated Drug-Drug Interactions.
Antimicrob Agents Chemother, 63(5):e02016-18, 25 Apr 2019
Cited by: 10 articles | PMID: 30783000 | PMCID: PMC6496093
Funding
Funders who supported this work.