Abstract
Background
Limited accessibility of the tumour precludes longitudinal characterisation for therapy guidance in pancreatic ductal adenocarcinoma (PDAC).Methods
We utilised dielectrophoresis-field flow fractionation (DEP-FFF) to isolate circulating tumour cells (CTCs) in 272 blood draws from 74 PDAC patients (41 localised, 33 metastatic) to non-invasively monitor disease progression.Results
Analysis using multiplex imaging flow cytometry revealed four distinct sub-populations of CTCs: epithelial (E-CTC), mesenchymal (M-CTC), partial epithelial-mesenchymal transition (pEMT-CTC) and stem cell-like (SC-CTC). Overall, CTC detection rate was 76.8% (209/272 draws) and total CTC counts did not correlate with any clinicopathological variables. However, the proportion of pEMT-CTCs (prop-pEMT) was correlated with advanced disease, worse progression-free and overall survival in all patients, and earlier recurrence after resection.Conclusion
Our results underscore the importance of immunophenotyping and quantifying specific CTC sub-populations in PDAC.Free full text

Characterisation of circulating tumour cell phenotypes identifies a partial-EMT sub-population for clinical stratification of pancreatic cancer
Abstract
Background
Limited accessibility of the tumour precludes longitudinal characterisation for therapy guidance in pancreatic ductal adenocarcinoma (PDAC).
Methods
We utilised dielectrophoresis-field flow fractionation (DEP-FFF) to isolate circulating tumour cells (CTCs) in 272 blood draws from 74 PDAC patients (41 localised, 33 metastatic) to non-invasively monitor disease progression.
Results
Analysis using multiplex imaging flow cytometry revealed four distinct sub-populations of CTCs: epithelial (E-CTC), mesenchymal (M-CTC), partial epithelial-mesenchymal transition (pEMT-CTC) and stem cell-like (SC-CTC). Overall, CTC detection rate was 76.8% (209/272 draws) and total CTC counts did not correlate with any clinicopathological variables. However, the proportion of pEMT-CTCs (prop-pEMT) was correlated with advanced disease, worse progression-free and overall survival in all patients, and earlier recurrence after resection.
Conclusion
Our results underscore the importance of immunophenotyping and quantifying specific CTC sub-populations in PDAC.
Background
Pancreatic ductal adenocarcinoma (PDAC) is projected to be the second leading cause of cancer-related deaths within the next decade and continues to have a dismal 5-year survival rate of 9%.1 Almost 80% of patients are diagnosed at locally advanced or metastatic stages and current treatment options are mostly limited to cytotoxic agents. Even with seemingly curative resection for patients presenting with localised disease many patients suffer early recurrence, suggesting an underlying propensity for early dissemination and resistance to available treatments.2
Elucidation of tumour cell phenotypes as they evolve during therapy can provide predictive insights into emergence of resistance to standard of care therapies in PDAC. A recently applied strategy to profile such dynamic changes in real time has involved the study of disseminated cancer cells, better known as circulating tumour cells (CTCs).3 As obtaining serial biopsies from primary or metastatic lesions in PDAC is clinically challenging, liquid biopsies have gained traction in recent years for understanding tumour kinetics under therapy. As opposed to circulating tumour DNA (ctDNA) and exosomes, CTCs provide the added benefit of representing the phenotypic characteristics of their tumour of origin.4
Classically, CTC isolation has involved the use of epithelial biomarkers such as cytokeratin and epithelial cell adhesion molecule (EpCAM) through immunogenic capture techniques such as CellSearch®, and their enumeration has shown to be a useful clinical prognosticator in breast, prostate, lung and colorectal cancers.5–8 However, a predictive model utilising CTCs as a biomarker has yet to demonstrate strong evidence for clinical validity in PDAC.9 Specifically, the role of CTC sub-populations, including those with non-epithelial characteristics, in clinical stratification remains to be elucidated through a comprehensive unbiased approach. Additionally, other CTC phenotypes such as circulating tumour microemboli (CTMs) may represent unique tumour capabilities such as increased metastatic propensity.10,11 As such, uncovering the dynamic nature of evolving CTC phenotypes correlating to tumour progression and therapeutic response is of clinical importance but poorly characterised, mostly due to a limitation of current enumeration strategies that only utilise epithelial and hematopoietic markers, restricting the definition of a CTC and potentially biasing its quantification. Ultimately, whether certain sub-populations of CTCs may be more clinically relevant than others remains undetermined.12,13
In order to perform a comprehensive phenotypic characterisation of PDAC CTCs, we used an epitope-independent isolation approach utilising a microfluidics-based dielectrophoresis enrichment strategy, followed by a multiplexed immunostaining assay.14–16 This approach provides the opportunity to profile the morphologic and phenotypic characteristics of these cells while bypassing the enrichment of epithelial cells. We evaluated its capability for the isolation of CTCs with different phenotypes and their clinical significance in a longitudinal cohort of PDAC patients.
Methods
Study population
Between April 2015 and January 2018, 74 patients were consented at MD Anderson Cancer Center (MDACC) under protocol number PA14-0867 and PA11-0670. Cohort details are shown in Table 1. The study was performed in accordance with standard ethical guidelines approved by the institutional review board (IRB) and in accordance with the Declaration of Helsinki. All patients had clinically and histologically confirmed localised or metastatic PDAC.
Table 1
Patient demographics and clinicopathological characteristics.
Cohorts characteristics | Patients |
---|---|
Total patient number | 74 |
Peripheral blood samples | 272 |
Mean age | 62.1 years (range 31–82 years) |
Gender | |
![]() | 42 (56.8%) |
![]() | 32 (43.2%) |
Median follow-up time per patient | 15.4 months |
Localised disease | 41 (66.4%) |
Metastatic | 33 (45.6%) |
Localised group: neoadjuvant treatment | 36/41 (87.8%) |
AJCC staging | |
![]() | 9 (12.2%) |
![]() | 22 (29.7%) |
![]() | 10 (13.5%) |
![]() | 33 (44.6%) |
Metastasis side | |
![]() | 23 (69.7%) |
![]() | 7 (21.2%) |
![]() | 3 (9.1) |
Tumour location | |
![]() | 56 (75.7%) |
![]() | 18 (24.3%) |
Treatment status | |
![]() | 65 |
![]() | 9 |
Cell Lines
Human pancreatic cell lines PANC-1, MIAPaCa-2 and HPAFII, Cancer associated fibroblast-19 (CAF-19) and Human brain microvascular endothelial cells (HBMEC) were used as controls. All cell lines were authenticated using a short tandem repeat (STR) DNA test.
Blood sample collection and processing
Whole-blood samples were collected in acid citrate dextrose tubes (BD, Franklin, Lakes NY) and processed within 4h of phlebotomy. Upon arrival, whole blood was centrifuged at 2500
×
g for 10
mins, plasma was removed and peripheral blood mononuclear cells (PBMCs) were isolated using Lymphocyte Separation Medium (Corning, Corning, NY) and centrifugation at 620
×
g for 30
min. PBMCs were stored at −80
°C in CryoStor® CS10 (Stemcell, Vancouver, Canada).
Microfluidic device and sample preparation
Circulating tumour cells were isolated utilising dielectrophoresis-field flow fractionation (DEP-FFF).14,17 The underlying concept of isolation takes advantage of the difference in “crossover frequency” (a parameter that depends on cell size and membrane surface area) of different cell types. Cells can be manipulated by a heterogeneous alternating electric field generated by electrodes in a low conductivity suspending medium. Below a field crossover frequency, cells are repelled from electrodes and above that frequency they are attracted to them. The crossover frequency for different cell types depends sensitively on their sizes and membrane surface areas. To isolate CTCs, we choose a suspension conductivity and electric field frequency to attract CTCs towards electrodes while repelling PBMCs.14,16 In our long, flat DEP-FFF chambers, a low conductivity, isotonic elution medium flows at a constant rate of 1.2mL/min. The specimen is injected from a slit to form a 25-μm layer adjacent to it. Ions and non-ionic molecules exchange by diffusion between the adjacent specimen and elution flows, reducing the conductivity near the cells while maintaining their tonicity. Further along the chamber, the cells pass over a microelectrode array energised at the frequency chosen to attract CTCs and repel PBMCs. A 20-μm layer of the flow closest to the electrode surface, and containing the CTCs, is then skimmed off into culture medium and kept on ice. The chambers are installed vertically to eliminate the influence of gravity in positioning the cells.
In order to deplete ions enough for DEP separation, a running buffer containing 181mM D-Mannitol (Sigma–Aldrich, St. Louis, MO), 67
mM Dextrose (Sigma–Aldrich), 1
mg/mL Kolliphor P188 (Sigma–Aldrich), 12.5% OptiPrep (Sigma–Aldrich) and 9
mM HEPES (Corning) was used. Buffy coats were thawed and resuspended in 0.46
mL of DMEM (Thermo Fisher, Carlsbad) supplemented with 10% FBS (Corning) and penicillin/streptomycin (Corning). 0.1
mL of 1
mg/ml DNase (StemCell) was added and samples were incubated at room temperature for 5
min. 0.44
mL of Cell density correction buffer (87
mM D-Mannitol, 170.4
mM Dextrose, 2.3
mg/mL Kolliphor P188, 20% OptiPrep and 22.7
mM HEPES) was added and samples were loaded into a 1
mL syringe. Samples were injected into the microfluidic device at a constant rate of 25
ul/min and a DEP current of ~0.2
A. The eluate parameters were: flow rate of ~1.2
mL/min, frequency of 45–90
kHz, conductivity of 20–40
ms/m.
Immunofluorescence and ImageStream
Samples collected through the microfluidic device were stained with antibodies against EpCAM conjugated to PE Cy7 (eBiosciences, San Diego, CA), CD133 conjugated to PE (Miltenyi Biotec, Bergisch Gladbach, Germany) and CD45 conjugated to Alexa 700 (Thermo Fisher). Then samples were fixed with 4% paraformaldehyde and permeabilised with 0.1% Triton X-100 (Sigma–Aldrich). This was then followed by staining with anti-vimentin conjugated to eFluor 615 (eBiosciences) and anti-CK conjugated to FITC (Thermo Fisher). Finally, DAPI was added and samples were analysed through Amnis ImageStream Mark II imaging Flow Cytometer (Millipore Sigma, Burlington, MA). In order to establish a reliable pipeline to classify all clinical samples, we first analysed well-characterised PDAC cell lines (PANC-1, MIAPaCa-2 and HPAFII) through this process. Once an expression threshold was determined (after compensation and gating), the same scheme was applied to clinical samples.
Predictive value of CTCs and statistical analysis
Regarding the CTC counts, receiver operating characteristic (ROC) curves and corresponding area of the curve (AUC) were utilised to identify the threshold values for predicting progressive disease. The Youden index was used to determine the cut point with the optimised sensitivity and specificity. The distribution of each continuous variable was summarised by its mean, standard deviation median, interquartile range. The distribution of each categorical variable was summarised in terms of its frequencies and percentages. Continuous variables were compared between two groups by Wilcoxon rank sum test, or by Kuskal-Wallis test if there are more than two groups. Survival curves were estimated using the Kaplan–Meier method, where overall survival is defined as time period from initial diagnosis to death of any reason, and the survival time was censored if the patient was alive at the last follow-up time. Progression-free survival was defined as time from day of first therapy to progression based on most recent RECIST 1.1 criteria or censored at the last follow-up. Log rank tests were used to compare the time-to-event variables between groups. The Cox proportional hazards regression model was used to evaluate the association between patient prognostic variables and overall survival or progression-free survival. If a continuous variable is highly skewed, the appropriate transformation (e.g. log transformation) was applied before modelling. The variables with p≤
0.2 under univariate analyses were included into the initial multivariable Cox model. Backward model selection was used to remove variables from the model until all remaining variables were statistically significant with p
<
0.05. Statistical analyses were performed with SPSS statistical software, version 24 (IBM, Armonk, NY), Prism 8 (GraphPad Software, Inc, San Diego, USA) and SAS (SAS Institute Inc, Cary, NC). Statistical significance was defined as a p-value of <0.05.
Results
Dielectrophoretic based isolation of heterogeneous CTC sub-populations
DEP-FFF enables epitope-independent isolation of viable CTCs based on physical cell properties including the diameter and surface rigidity.14–16 This allows for the capture of distinct sub-populations of CTCs within an hour of blood processing time. In order to demonstrate our capture efficiency, we performed spike-in experiments utilising a PDAC cell line, PANC-1, into healthy human peripheral blood mononuclear cells (PBMCs). Spike-ins of 10, 100, 200, 500, 1000 and 1,200 (n=
3 replicates for each) cells revealed recovery rates of up to 91% (average recovery rate 89.1%) with high linearity (R2
=
0.9873, Fig. 1a). Our ability to capture and characterise multiple unbiased CTC sub-populations was also tested by adding different PDAC cell lines with known phenotypes of interest, including epithelial (HPAFII), mesenchymal (MIAPaCa-2) and stem cell-like (PANC-1 spheroids) subtypes. These cell lines were successfully “re-identified” following DEP isolation, where cells were stained with anti-CD45, EpCAM, pan-cytokeratin (PanCK), vimentin and CD133 antibodies, as well as DAPI (Fig. 1b). Based on characterisation of isolated PDAC cells using DEP and imaging flow cytometry with distinct epithelial, mesenchymal and stem-cell markers, we defined four distinct sub-populations in the peripheral blood of patients with PDAC using expression of markers listed above—epithelial (E-CTC), mesenchymal (M-CTC), bi-phenotypic or partial epithelial-mesenchymal transition (pEMT-CTC) and stem cell-like (SC-CTC)—based on markers of interest (Table 2). CTCs were further curated based on characteristic size (>14
μm) and distinct nuclear structure covering >80% of the cell surface (Fig. 1c). In addition to single CTCs, this technique was able to isolate CTCs associated with lymphocytes as well as clusters of CTCs (Fig. 1d, e).
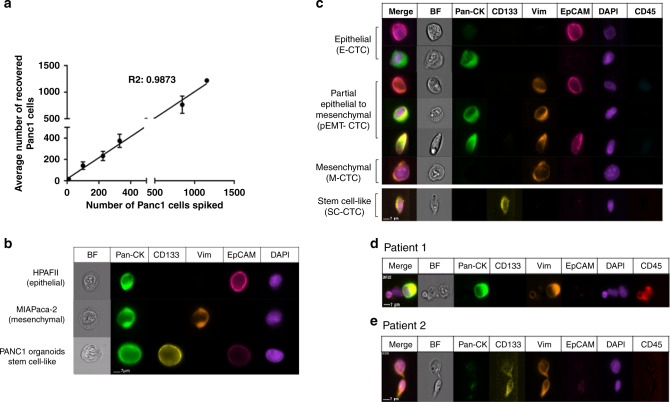
a Scatter plot showing known cell numbers added to PBMC and number of cells recovered from DEP-FFF, b Brightfield and immunofluorescence images of marker proteins of different cell lines recovered using DEP-FFF and analysed by imagestream flow cytometry. BF Brightfield, Pan-CK pan-cytokeratin, Vim vimentin, EpCAM epithelial cell adhesion molecule, DAPI 4′,6-Diamidin-2-phenylindol and CD45 Protein tyrosine phosphatase, receptor type C. Brightfield and immunofluorescence images of CTC populations (c) CTCs lymphocytes (d) and CTC–CTC clusters (e) identified in PDAC patients by ImageStream.
Table 2
Defined subtypes to classify patient-derived CTCs.
Phenotypes identified | Markers |
---|---|
E-CTC | CD45−, EpCAM+ and/or Pan-CK+, DAPI+ |
pEMT-CTC | CD45−, EpCAM+ and/or Pan-CK+, Vim+, DAPI+ |
M-CTC | CD45−, EpCAM−, Vim+, DAPI+ |
SC-CTC | CD45−, CD133+, DAPI+ |
Patient characteristics
A total of 74 patients (mean age 62.1 years, range 31–82 years) with histologically proven PDAC between April 2015 and January 2018 were enrolled in the study (Table 1). From these patients, 272 peripheral blood samples were collected and processed through DEP (median number of blood draws/patient=
3.0, range 1–9). The median follow-up time for all patients was 15.4 months (range 0–43.1 months). Longitudinal follow-up samples were obtained during routine clinical follow-up appointments for patients enrolled in the protocol before administration of chemotherapy and together with clinically necessary blood draws. The cohort was stratified into two groups: 41 out of 74 (55.4%) with localised disease and 33 out of 74 (44.6%) patients with metastatic disease. In the localised disease group, 36 of 41 received neoadjuvant treatment (87.8%), of which 27% (10/36) progressed under treatment and therefore did not receive surgery. Twenty-two patients underwent resection of the primary tumour (22/41, 53.6%) with mostly R0 resections (18/22, 78.6%). In addition, 4/41 (9.8%) patients underwent surgical exploration without resection due to intraoperative diagnosis of metastasis and 5/41 (12.2%) patients did not receive an operation due to comorbidities. In the metastatic disease group, 22/33 (67.7%) progressed on therapy during a median follow-up of 6.2 months (range 0–23.5 months). In patients with metastatic disease, baseline draws were untreated in 8/33 (24%) of cases while 25/33 were treated (75%). In addition, 21/33 (64%) have progression samples collected. The average number of draws was 3.8 (1–7 range) and the average time between draws was 80.5 days (26–294 range).
CTC counts in overall PDAC cohort
Overall CTC detection rate across all samples, and including single CTCs and clusters, was 76.8% (209/272 samples, range 0–692 cells/7.5ml of blood). Mean number of CTCs per 7.5
ml of blood was 29
±
70.6 (range 0–692). Across all samples, mean numbers of CTCs were as follows: E-CTC: 3.5
±
1.1 cells/7.5
ml (12.2%), M-CTC: 9.3
±
1.5 cells/7.5
ml (31.9%), pEMT-CTC: 13.8
±
2.1 cells/7.5
ml (47.7%) and SC-CTC: 2.4
±
0.5 cells/7.5
ml (8.2%) (Fig. 2a).
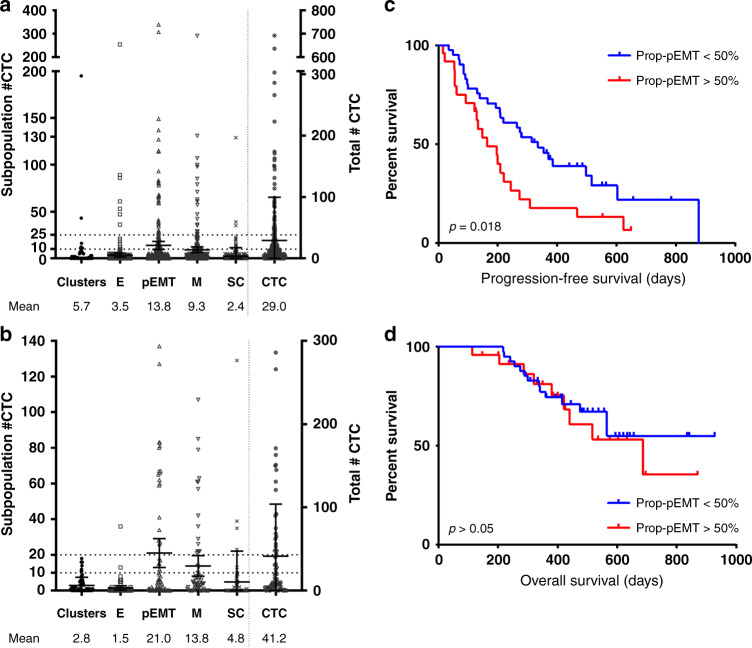
a Scatter plot showing total counts in five phenotypically distinct populations across all collected samples (n=
272) or at baseline treatment naïve status (n
=
65). b Last column shows all counts. Prognostic value of CTCs at baseline treatment-naïve status (n
=
65). c Progression-free survival comparing patients with more vs. less than 50% Prop-pEMT (p
=
0.018). d Overall survival comparing patients with more vs. less than 50% Prop-pEMT (p
=
0.63).
In treatment naïve patients at baseline (n=
65), mean total CTC number was 41.2
±
62.7 and per sub-type: E-CTC-1.5
±
0.6 cells/7.5
ml (3.7%), M-CTC 13.8
±
2.9 cells/7.5
ml (33.5%), pEMT-CTC 21.0
±
4.05 cells/7.5
ml (51.1%) and SC-CTC 4.8
±
2.1 cells/7.5
ml (11.7%) (Fig. 2b). CTC clusters were identified in 32/65 (49.2%, range 0–18 clusters) treatment-naive patients with a mean 2.6
±
12.6 cells/7.5
ml in all draws and 2.8
±
4.6 cells/7.5
ml in BTN patients only (Fig. 2a,2a,bb).
Notably, there was no difference in total CTC counts at baseline (n=
65) based on tumour location, stage and size (all p
>
0.05, Supplementary Table 1, Supplementary Fig. 1A-D). However, the proportion of pEMT-CTC relative to total CTC count (“Prop-pEMT”) was significantly higher in M1 vs. M0 patients (mean 52.4% vs 28.4%, p
=
0.004, Supplementary Fig. 1E, Supplementary Table 1). Additionally, “Prop-pEMT” increased with stage (stage I: 24.4
±
26.9%, stage II: 28.5
±
23.5%, stage III: 32.4
±
35.1%, stage IV: 52.0
±
33.5%, p
=
0.0024, Supplementary Fig. 1F, Supplementary Table 1) and with distal tumour location (head: 33.5
±
29.1%, tail/body: 54.2
±
35.2%, p
=
0.04) Supplementary Fig. 1G Supplementary Table 1).
Liquid biopsies of all patients (n=
139) while on therapy demonstrated that those with progressive disease based on RECIST criteria, compared to those with blood draws on therapy but without progression had significantly more total CTCs (p
=
0.0162), E-CTCs (p
=
0.0489), M-CTCs (p
=
0.0087) and higher Prop-pEMT (p
=
0.0410) (Supplementary Fig. 2 and Supplementary Table 2).
Baseline treatment-naïve (BTN, n=
65) patients with a higher proportion of pEMT-CTC
50% showed a significantly worse progression-free survival (PFS) compared to patients with a lower pEMT-CTC proportion on univariate analysis (p
=
0.020, Fig. 2c, Supplementary Table 3B, 4). On multivariate analysis, proportion of pEMT-CTC remains significantly associated to poorer PFS (HR 1.98, 95% CI 1.05–3.73, p
=
0.036) after adjusting for other factors (Supplementary Table 3A-C). Proportion of pEMT-CTC showed no impact on overall survival in BTN patients (Fig. 2d). In addition, disease stage had a significant impact on PFS (p-value
=
0.009), but was not a significant factor (p-value
=
0.190) in the multivariable model after adjusting for pEMT-CTC, log transformed SC, and age (Supplementary Table 3C). No other subgroup showed a significant prognostic value at BTN status (all, p
>
0.05; Supplementary Table 3A-B and Supplementary Table 5) including total pEMT counts at different cut-offs (Supplementary Table 6).
CTC counts in localised disease
Among patients with localised disease, mean CTC counts (including single CTCs and clusters) dropped significantly following resection across total, pEMT, EMT subgroups and clusters (Fig. 3a–f), but did not drop to zero in any patient. Among resected patients, seven (7/22, 31.8%) had an early recurrence within 12 months after surgical resection. Patients with “Prop-pEMT”>
50% in preoperative blood draws (n
=
22) were at higher risk for an early recurrence (4/7 (57.1%) versus 1/15 (6.7%), p
=
0.02, Fig. 3g). Similarly, patients with preoperative “Prop-pEMT” >50% showed a worse overall survival (OS) after resection (677 days versus not reached p
=
0.01, Fig. 3h). None of the other CTC sub-populations showed a significant impact on early recurrence rate or OS (all p
>
0.05, Supplementary Table 7).
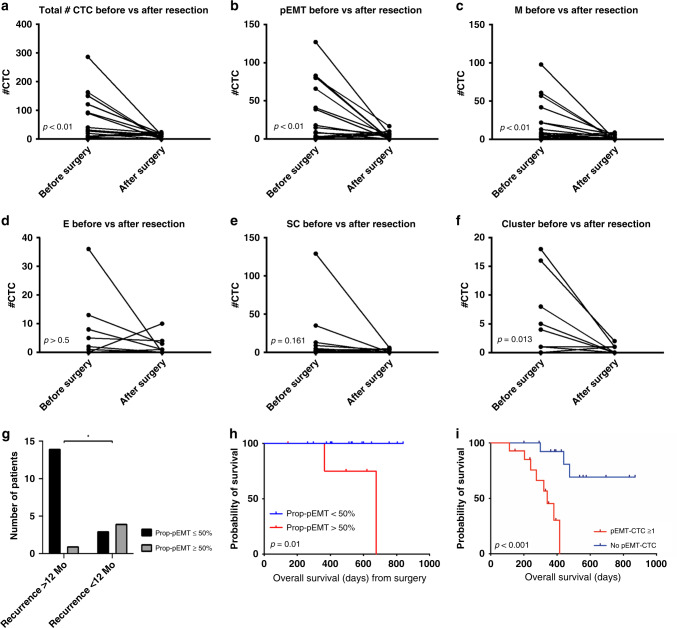
a total CTCs, b pEMT-CTC, c M-CTC, d E-CTC, e SC-CTC, f CTC clusters before and after surgery (n=
22). Prognostic value of CTCs at draw closest to surgery. g Bar graph plotting Prop-pEMT
50% vs. recurrence status (Fisher exact test, p
=
0.021). h Kapla–Meier plot (overall survival from surgery) comparing patients with vs. without 50% Prop-pEMT (p
=
0.01). i Overall survival comparing patients with at least one pEMT-CTC vs none (p
<
0.001).
CTC counts in metastatic disease
In treatment naïve patients with M1 disease (n=
29), the presence of at least one pEMT-CTC at baseline was significantly associated with decreased overall survival compared to patients with undetectable pEMT-CTCs (325.2
±
28.0 days vs. 731.0
±
67.8 days, p
<
0.001, Fig. 3i). Liquid biopsies of M1 patients while on therapy (n
=
132) demonstrated that those with progressive disease had significantly more M-CTCs (13.5
±
23.0 cells/draw vs. 4.2
±
10.3 cells/draw, p
=
0.005).
Molecular characterisation of different CTC sub-populations
To further characterise isolated CTCs, we performed droplet digital PCR (ddPCR) for mutant KRAS as previously described,18 in captured cells from a patient with metastatic PDAC following progressive disease in the liver. Cells were isolated by DEP-FFF from a single draw at the time of progression. They were then stained as described and FACS sorted in either pools of 10 cells prior to amplification (CTC-2, Vim+ and CTC-3, Vim+), or amplified as single cells (CTC-1, E+/CK+/Vim+/−) depending on the number of cells captured. Whole-genome amplification of sorted cells was performed with the REPLI-g Single Cell Kit (Qiagen) per the manufacturer’s protocol. In addition, three pools of 10 CD45+ cells were also isolated and amplified and used as wild-type control and paired tumour tissue from the metastatic side was used as positive control (Table 3). Mutant KRAS was detected in all three CTCs (mutant allele fraction (MAF) 1.27, 84.3 and 78.4, respectively) and in the tumour sample (MAF 50.22) while all three CD45+ cells were KRAS negative. Additionally, to better characterise the M phenotype and exclude other cell populations that also expressed Vimentin, CD31+/Vim+ and FAP+/Vim+ cells were analysed in three clinical samples (Supplementary Fig. 3). In all three cases, we did not detect a single FAP+/Vim+ or CD31+Vim+ cells instead, we detected ~1% of CD45− cells expressing CD31 or FAP in combination with Vim and CK.
Table 3
Molecular characterisation of CTC by ddPCR.
Sample | Number of cells | Phenotype | MAF mutant KRAS by ddPCR |
---|---|---|---|
![]() | 10 | CD45−/Vim+ | 1.27 |
![]() | 10 | CD45−/Vim+ | 84.3 |
![]() | 1 | CD45−/E+/CK+/Vim+/− | 78.4 |
Positive control | Pellet | Tissue | 50.22 |
Negative control 1 | 10 | CD45+ | WT |
Negative control 2 | 10 | CD45+ | WT |
Negative control 3 | 10 | CD45+ | WT |
Discussion
In visceral tumours, such as PDAC, repeated tumour sampling for therapy monitoring and molecular profiling is rarely performed in the clinical setting due to their invasive nature and cost. Thus, strategies involving liquid biopsies have gained traction for their minimally invasive approach in cancer diagnosis, prognostication, therapy stratification and measuring response to therapy. In this study, we interrogated if longitudinal characterisation of CTC sub-populations may better resolve the prognostic and predictive utility of this liquid biopsy compartment. We also describe CTC phenotypes that may be most relevant to metastasis and chemoresistance, which have been previously correlated with the presence of cancer stem cells or cells with epithelial-mesenchymal transition (EMT) profiles.19,20
Biased immunogenic capture of CTCs based on epithelial markers potentially excludes cells that have biological implications in the process of metastasis and chemoresistance.21,22 Even among epithelial markers typically used for CTC capture, such as EpCAM or cytokeratins, there is no consensus regarding specific marker(s) that may more effectively identify clinically relevant CTCs. Utilising DEP-FFF, which allows for isolation of particles based on their physical properties, we isolated CTCs through an antigen independent approach. In doing so, we effectively isolated phenotypically distinct CTC populations that may be grouped into four major sub-populations based on our multiplexed immunostaining assay: E-CTC, M-CTC, pEMT-CTC and SC-CTC
Similar to previous work,23–26 the most common sub-population of PDAC CTCs expressed a combination of epithelial and mesenchymal markers (pEMT-CTC), representing neoplastic cells arrested in a “bi-phenotypic” state as they are shed from their tumour of origin.21,22,27 Increasing evidence suggests that EMT is a highly dynamic process, inclusive of intermediate cell states (“pEMT”), such that only a minority of cancer cells transition to a pure mesenchymal state.22,27,28 Notably, neoplastic cells with a partial-EMT phenotype show greater invasiveness and therapeutic resistance,22 which coincides with our findings of this CTC sub-population correlating with both stage at presentation, as well as with progression on therapy. Specifically, we demonstrate that “prop-pEMT” (the proportion of CTCs with pEMT phenotype overall CTCs) are higher in patients with metastases at diagnosis. Additionally, whereas there are no effective methods for stratification of early recurrence risk in resectable PDAC, we demonstrate that a high “prop-pEMT” at the time of surgery is correlated with early recurrence. Importantly, CTC numbers rarely dropped to zero even after complete surgical resection, which is in-line with recent studies.5,25 This raises the concern that micro-metastatic lesions may have already been established even in patients with apparently curative resection and begun releasing CTCs themselves. Additionally, once overt metastasis is detectable, we show that a single EpCAM/vimentin co-expressing cell, representing a specific pEMT status, in treatment-naive patients is associated with a significantly worse overall survival. As previously described, we also did not detect differences in CTC total counts across PDAC stages, which may depict the aggressive nature of PDAC and its ability to metastasise from its onset.2,26
While most clinical correlates of adverse outcome were associated with pEMT-CTCs, we identified at least one parameter associated with the population of Vim+/EpCAM− CTCs (M-CTC). This M-CTC sub-population was significantly higher at radiographic progression compared to non-progression in metastatic patients under treatment. CTCs predominantly expressing vimentin have been associated with a worse prognosis or aggressive disease in other cancer types6,7 while vimentin only expressing CTCs are seen in rarer numbers in the context of PDAC.5,25,26 Taken together with pEMT-CTCs as described above, the presence of these two populations may portend a dynamic picture of CTCs that exist in the spectrum of EMT and the ability for tumours to adapt to chemotherapeutic pressures.
Among weaknesses of our study, we understand the limits of our characterisation approach in that only a certain number of markers can be included within the Imagestream analysis. We attempted to maximise our approach by including as many conjugated markers as we could reliably and consistently use. As such, we are insightful of the fact that the markers selected may not necessarily be sensitive or specific enough to characterise the entire population of these CTC subtypes. For example, we could only include CD133 and Vimentin as markers to characterise ‘stem cell-like” and mesenchymal populations, respectively. We believe that further studies should be able to take a more comprehensive approach to populations, like those of stem cells by including markers such as CD44, CD24, ALDH and RORγ.29 Additionally, we have not fully characterised the size limit of our device in terms of its ability to isolate CTC clusters and thus feel it is important to interpret these results with caution.
In conclusion, our study identifies at least four distinct sub-populations of CTCs within a single blood draw of PDAC patients. Although such CTC subtypes have been previously described individually,13,19 our study provides a comprehensive characterisation of such populations at baseline treatment naïve status and during longitudinal follow-up. Of all CTC sub-populations, we have isolated in PDAC through an antigen independent retrieval method, the pEMT phenotype showed the greatest predictive and prognostic value in both resectable and metastatic disease. The biological underpinnings of this pEMT sub-population warrants further study to understand the cellular mechanisms driving their aggressive potential and therapeutic resistance, particularly in contrast to M-CTCs. Our detailed phenotypic characterisation of CTCs is important as specific sub-populations, rather than total CTC number, in circulation may have greater clinical relevance and provide insights into treatment response.20
Author contributions
A.M., P.A.G., H.A. Study Design: A.M., P.A.G., H.A., A.S., V.B., D.U.K. Provision of clinical samples and information: B.S., G.R.V., M.H.K. Samples processing: A.S., V.B., P.G., D.U.K. Microfluidics device design: P.G., F.A.S.L. Sample isolation: A.S., V.B., P.A.G., D.U.K., N.K. Samples staining and cytometry: A.S., V.B., P.A.G., D.U.K. Data analysis: J.H., F.A.S.L., J.J.L., W.Q., Y.S. P.A.G. Manuscript writing: A.S., V.B. All authors read and approved the final manuscript.
Ethics approval and consent to participate
The study was performed in accordance with standard ethical guidelines approved by the institutional review board (IRB), protocol numbers PA11-0670 and PA15-0014 at MD Anderson Cancer Center, and in accordance with the Declaration of Helsinki. Patients provided their informed consent to participate in this study.
Data availability
All data generated and analysed during the current study are available from the corresponding author on reasonable request.
Competing interests
A.M. receives royalties for a pancreatic cancer biomarker test from Cosmos Wisdom Biotechnology, and this financial relationship is managed and monitored by the UTMDACC Conflict of Interest Committee. A.M. is also listed as an inventor on a patent that has been licensed by Johns Hopkins University to Thrive Earlier Detection. The remaining authors declare no competing interests.
Funding information
This research was supported in part by the Cancer Prevention and Research Institute of Texas (CPRIT) (No. RP160517), NCI P50 CA221707, U01 CA196403 and U01 CA200468 to A.M. N.K. was supported by the CPRIT Research Training Program (No. RP170067). V.B. was supported by the CPRIT Research Training Program (Nos. RP140106 and RP170067) and NCI (Nos. T32CA217789-03 and U54CA096297). J.J.L. was supported by the National Institutes of Health (NIH) (No. T32CA009599). A.S. was supported by the German Research Foundation (SE-2616/2-1). D.U.K. was supported by the National Research Foundation of Korea (NRF) grant funded by the Korea government (MSIT) (No. 2018R1C1B5086234).
Footnotes
Publisher’s note Springer Nature remains neutral with regard to jurisdictional claims in published maps and institutional affiliations.
These authors contributed equally: Alexander Semaan, Vincent Bernard, Dong U. Kim
Supplementary information
The online version contains supplementary material available at 10.1038/s41416-021-01350-9.
References
Articles from British Journal of Cancer are provided here courtesy of Cancer Research UK
Full text links
Read article at publisher's site: https://doi.org/10.1038/s41416-021-01350-9
Read article for free, from open access legal sources, via Unpaywall:
https://www.nature.com/articles/s41416-021-01350-9.pdf
Citations & impact
Impact metrics
Citations of article over time
Alternative metrics

Discover the attention surrounding your research
https://www.altmetric.com/details/103075111
Article citations
Circulating tumor cells in pancreatic cancer: more than liquid biopsy.
Ther Adv Med Oncol, 16:17588359241284935, 09 Oct 2024
Cited by: 0 articles | PMID: 39421679 | PMCID: PMC11483845
Review Free full text in Europe PMC
Evolution of Liquid Biopsies for Detecting Pancreatic Cancer.
Cancers (Basel), 16(19):3335, 29 Sep 2024
Cited by: 0 articles | PMID: 39409954 | PMCID: PMC11475855
Review Free full text in Europe PMC
Circulating tumor cells in pancreatic cancer: The prognostic impact in surgical patients.
World J Clin Oncol, 15(8):987-991, 01 Aug 2024
Cited by: 0 articles | PMID: 39193164 | PMCID: PMC11346077
Circulating tumor cell phenotype detection based on epithelial-mesenchymal transition markers combined with clinicopathological risk has potential to better predict recurrence in stage III breast cancer treated with neoadjuvant chemotherapy: a pilot study.
Breast Cancer Res Treat, 207(3):517-527, 11 Jul 2024
Cited by: 0 articles | PMID: 38990453
Novel Histone Deacetylase (HDAC) Inhibitor Induces Apoptosis and Suppresses Invasion via E-Cadherin Upregulation in Pancreatic Ductal Adenocarcinoma (PDAC).
Pharmaceuticals (Basel), 17(6):752, 07 Jun 2024
Cited by: 0 articles | PMID: 38931419
Go to all (26) article citations
Data
Data behind the article
This data has been text mined from the article, or deposited into data resources.
BioStudies: supplemental material and supporting data
Similar Articles
To arrive at the top five similar articles we use a word-weighted algorithm to compare words from the Title and Abstract of each citation.
Molecular detection of epithelial-mesenchymal transition markers in circulating tumor cells from pancreatic cancer patients: Potential role in clinical practice.
World J Gastroenterol, 25(1):138-150, 01 Jan 2019
Cited by: 43 articles | PMID: 30643364 | PMCID: PMC6328963
CTC phenotyping for a preoperative assessment of tumor metastasis and overall survival of pancreatic ductal adenocarcinoma patients.
EBioMedicine, 46:133-149, 30 Jul 2019
Cited by: 22 articles | PMID: 31375425 | PMCID: PMC6712350
KRAS mutations in pancreatic circulating tumor cells: a pilot study.
Tumour Biol, 37(6):7547-7554, 18 Dec 2015
Cited by: 32 articles | PMID: 26684803
Clinical applications of circulating tumor DNA and circulating tumor cells in pancreatic cancer.
Mol Oncol, 10(3):481-493, 22 Jan 2016
Cited by: 40 articles | PMID: 26856794 | PMCID: PMC5528974
Review Free full text in Europe PMC
Funding
Funders who supported this work.
Cancer Prevention and Research Institute of Texas (3)
Grant ID: RP160517
Grant ID: RP140106
Grant ID: RP170067
Deutsche Forschungsgemeinschaft (1)
Grant ID: SE-2616/2-1
NCI NIH HHS (1)
Grant ID: U01 CA200468
National Research Foundation of Korea (1)
Grant ID: 2018R1C1B5086234
U.S. Department of Health & Human Services | NIH | National Cancer Institute (6)
Grant ID: U54CA096297
Grant ID: CA196403
Grant ID: CA221707
Grant ID: CA200468
Grant ID: T32CA009599
Grant ID: T32CA217789-03
U.S. Department of Health & Human Services | NIH | National Cancer Institute (NCI) (6)
Grant ID: U54CA096297
Grant ID: CA196403
Grant ID: CA200468
Grant ID: T32CA217789-03
Grant ID: CA221707
Grant ID: T32CA009599