Abstract
Free full text

Distinctive clinical presentation and pathogenic specificities of anti-AK5 encephalitis
Abstract
Limbic encephalitis with antibodies against adenylate kinase 5 (AK5) has been difficult to characterize because of its rarity. In this study, we identified 10 new cases and reviewed 16 previously reported patients, investigating clinical features, IgG subclasses, human leucocyte antigen and CSF proteomic profiles. Patients with anti-AK5 limbic encephalitis were mostly male (20/26, 76.9%) with a median age of 66years (range 48–94). The predominant symptom was severe episodic amnesia in all patients, and this was frequently associated with depression (17/25, 68.0%). Weight loss, asthenia and anorexia were also highly characteristic, being present in 11/25 (44.0%) patients. Although epilepsy was always lacking at disease onset, seizures developed later in a subset of patients (4/25, 16.0%). All patients presented CSF abnormalities, such as pleocytosis (18/25, 72.0%), oligoclonal bands (18/25, 72.0%) and increased Tau (11/14, 78.6%). Temporal lobe hyperintensities were almost always present at disease onset (23/26, 88.5%), evolving nearly invariably towards severe atrophy in subsequent MRIs (17/19, 89.5%). This finding was in line with a poor response to immunotherapy, with only 5/25 (20.0%) patients responding. IgG1 was the predominant subclass, being the most frequently detected and the one with the highest titres in nine CSF-serum paired samples. A temporal biopsy from one of our new cases showed massive lymphocytic infiltrates dominated by both CD4+ and CT8+ T cells, intense granzyme B expression and abundant macrophages/microglia. Human leucocyte antigen (HLA) analysis in 11 patients showed a striking association with HLA-B*08:01 [7/11, 63.6%; odds ratio (OR)
=
13.4, 95% confidence interval (CI): 3.8–47.4], C*07:01 (8/11, 72.7%; OR
=
11.0, 95% CI: 2.9–42.5), DRB1*03:01 (8/11, 72.7%; OR
=
14.4, 95% CI: 3.7–55.7), DQB1*02:01 (8/11, 72.7%; OR
=
13.5, 95% CI: 3.5–52.0) and DQA1*05:01 (8/11, 72.7%; OR
=
14.4, 95% CI: 3.7–55.7) alleles, which formed the extended haplotype B8-C7-DR3-DQ2 in 6/11 (54.5%) patients (OR
=
16.5, 95% CI: 4.8–57.1). Finally, we compared the CSF proteomic profile of five anti-AK5 patients with that of 40 control subjects and 10 cases with other more common non-paraneoplastic limbic encephalitis (five with antibodies against leucine-rich glioma inactivated 1 and five against contactin-associated protein-like 2), as well as 10 cases with paraneoplastic neurological syndromes (five with antibodies against Yo and five against Ma2). These comparisons revealed 31 and seven significantly upregulated proteins in anti-AK5 limbic encephalitis, respectively mapping to apoptosis pathways and innate/adaptive immune responses. These findings suggest that the clinical manifestations of anti-AK5 limbic encephalitis result from a distinct T cell-mediated pathogenesis, with major cytotoxicity-induced apoptosis leading to a prompt and aggressive neuronal loss, likely explaining the poor prognosis and response to immunotherapy.
Introduction
Limbic encephalitis with antibodies against adenylate kinase 5 (AK5) is a rare non-paraneoplastic encephalitis, with only 16 patients reported to date.1-4 Its clinical picture is characterized by severe amnesia, mood disorders and, intriguingly, a lack of seizures in most cases.1–3 This last feature contrasts with the most common non-paraneoplastic limbic encephalitis, anti-leucine-rich glioma-inactivated 1 (LGI1) and anti-contactin-associated protein-like 2 (CASPR2), which are typically associated with temporal lobe epilepsy.5
Besides clinical presentation, anti-AK5 limbic encephalitis differs from anti-LGI1 and anti-CASPR2 limbic encephalitis in the fact it is poorly responsive to immunotherapy.1–3,6–9 Furthermore, whereas antibodies against LGI1 and CASPR2 are thought to be pathogenic, based on the surface location of the antigens and in vitro and in vivo evidence,10–14 AK5 is a cytosolic antigen and AK5 antibodies are not believed to play a direct role in the disease pathogenesis.2 In addition, anti-LGI1 and anti-CASPR2 limbic encephalitis have both recently been found to have strong associations with specific human leucocyte antigen (HLA) class II haplotypes.9,15–18 In contrast, whether or not a particular HLA association exists in anti-AK5 limbic encephalitis remains unknown.
In this study, we report on the clinical characterization of 10 new anti-AK5 limbic encephalitis cases and explore the pathogenic distinctness of anti-AK5 limbic encephalitis based on neuropathology findings, IgG subclasses, HLA profiles, and CSF proteomic analysis.
Materials and methods
Subjects and clinical data
We identified new patients with AK5 antibodies diagnosed in our centre from June 2015 to October 2020. Anti-AK5 CSF positivity was first screened using immunohistochemistry on rat brain slides and subsequently confirmed by a cell-based assay, as previously described.2 Clinical and paraclinical data of interest were obtained from hospital charts, and new information was also collected for Patients 1–7 from our previous report,2 as they were part of our database. The assessment of outcome was based on the results of neuropsychological evaluations, behaviour and daily activities described by relatives and whether patients were able to return to work. In addition to the data collected by us on these 17 patients, clinical information on nine additional patients was added based on the literature.1–4 The Institutional Review Board of Université Claude Bernard Lyon 1 and Hospices Civils de Lyon approved the study (ICARE-II). Written informed consent was obtained for all patients recruited locally.
IgG subclasses
IgG subclasses were determined using mouse anti-human antibodies that specifically recognize immunoglobulin (Ig)G1 (mouse anti-human IgG1 CH2 domain; Bio-Rad), IgG2 [purified mouse anti-human IgG2 clone G18-21 (RUO); BD Biosciences], IgG3 (mouse anti-human IgG3; Bio-Rad) or IgG4 [purified mouse anti-human IgG4 clone G17-4 (RUO); BD Biosciences]. A total of 100μl of the IgG solution of interest (diluted 1/1000 for IgG1 and IgG3, 1/250 for IgG2, and 1/500 for IgG4) in PBS1×-0.2% gelatine-0.1% Triton was used and incubated for 1
h at room temperature in the dark. After aspiration, cells were washed twice with PBS1× (100
μl). A secondary goat anti-mouse antibody (100
μl) coupled to a fluorochrome (Alexa Fluor® 555; ThermoFisher Scientific), diluted 1/1000 in PBS1×-0.2% gelatine-0.1% Triton, was added and incubated for 1
h at room temperature in the dark. After aspiration, cells were washed twice with PBS (100
μl). Additionally, to determine the predominant IgG subclass, end point dilutions were performed and titres were considered as the lowest dilution with positive signal by cell-based assay. All of the cell-based assays were read using a Zeiss Axiovert 200
M microscope (Zeiss).
HLA analysis
HLA genotyping, reported here at a four-digit level (amino acid) resolution, was performed in patients with anti-AK5 limbic encephalitis and available DNA using next generation sequencing with a Holotype sequencer system (Omixon). The most likely haplotypes were selected based on the public database HaploStats of the National Marrow Donor Program (https://www.haplostats.org, accessed 3 June 2020), after taking into account each patient’s ethnic origin. Controls (courtesy of EFS Auvergne-Rhône-Alpes) were 442 healthy potential bone marrow donors, whose genotypes were previously obtained using the same technique.
Differences in the HLA carrier frequencies between patients and controls were analysed using two-tailed Fisher’s exact tests (SPSS software package version 26.0; IBM Corp). Bonferroni’s method was used to correct for multiple comparisons according to the number of alleles of each locus; corrected P<
0.05 was considered significant.
Peptide binding prediction and search for homologous peptides from pathogens
Full-length sequences of the three known AK5 isoforms (UniProt; https://www.uniprot.org, accessed 25 June 2020) were downloaded as FASTA files (accession number Q9Y6K8) and used to predict HLA class I binding for nine amino acid-long consecutive overlapping peptides using NetMHCpan 4.1,19 as well as HLA class II binding for 15 amino acid-long overlapping peptides using NetMHCIIpan 4.0.20 These servers provide prediction values for each peptide as percentile rank affinity versus scores obtained using a large number of random natural peptides that are of the same length as the query peptide (850000 for NetMHCpan 4.1; and 500
000 for NetMHCIIpan 4.0). A peptide of rank value <0.5% by NetMHCpan 4.1, and <1% by NetMHCIIpan 4.0 is considered a strong binder.19,20
Next, peptides predicted as strong binders were analysed using the Basic Local Alignment Search Tool, BLAST (https://blast.ncbi.nlm.nih.gov/Blast.cgi, accessed 30 June 2020) to identify possible homologous peptides that could be present in common human pathogens. Peptides sequences were entered in FASTA, non-redundant protein sequences database and the blastp algorithm selected, with the search limited to bacteria (taxon id: 2), viruses (taxon id: 10239) and fungi (taxon id: 4751). First, the 9- and 15-amino acid-long peptides obtained in the peptide binding prediction analysis were investigated. Second, peptides with adjacent or overlapping sequences were studied together, as well as core peptides. Only peptides showing homology E-values <0.01 were considered relevant.
Proteomic analysis
Relative expression levels of 643 proteins were assayed in the CSF of healthy controls (n=
40) and patients with antibodies against AK5 (n
=
5), LGI1 (n
=
5), CASPR2 (n
=
5), Yo (n
=
5) and Ma2 (n
=
5). The technology used was SomaScan (SomaLogic Inc), a highly multiplexed aptamer approach that uses a large panel of SOMAmers (synthetic single-stranded DNA-based molecular recognition elements) recognizing specific proteins. SOMAmer quantification was then performed by hybridization to microarrays containing single-stranded DNA that was complementary to each unique SOMAmer DNA sequence.21 The SomaScan platform has been validated through genetic analysis of protein quantitative trait loci and by comparing results to a Luminex based platform.22,23 SomaScan was designed to have an extended dynamic range—from femtomolar to micromolar—including both extracellular and intracellular proteins (also comprising soluble domains of membrane proteins), with predominantly proteins in the secretome being targeted.
To preprocess the proteomic data, raw expression values were log2-transformed to ensure heteroskedasticity, as the variance of raw expression measurements was dependent on the relative abundance of each protein. Univariate analysis was subsequently performed using a non-parametric Wilcoxon sum-rank test for unpaired comparisons of proteins distributions between anti-AK5 patients and controls, anti-AK5 and anti-LGI1/CASPR2 patients and anti-AK5 and anti-Yo/Ma2 patients. Multiple comparison correction was performed using the Benjamini-Hochberg procedure; corrected P<
0.05 were considered significant. All these calculations were performed using R (http://www.r-project.org/).
Protein-protein interactions
STRING v.11 (https://string-db.org, accessed 15 December 2020) was used to establish whether upregulated AK5-specific proteins formed a protein-protein association network based on their interactions at seven levels: gene fusion, gene co-occurrence, genome neighbourhood, co-expression, biochemical or genetic experimental data, text mining and known association in curated databases. For every network, a functional enrichment analysis was performed and a P-value provided. Among the different outputs offered, we selected the functional enrichments according to the biological processes defined by the Gene Ontology resource (https://geneontology.org, accessed 15 December 2020), where the number of genes from the network out of the total of those involved in a certain biological process is provided, along with its false discovery rate (FDR).
Additionally, tovisualize differences in CSF expression of relevant proteins between patients with antibodies against AK5, Yo, Ma2, CASPR2 and LGI1, we first extracted the median expression by diagnostic groups after log2 preprocessing for every protein involved in the most significant pathways. Then, we plotted a heat map of the selected proteins’ median expression Z-score transformed and showed the different expression for each diagnostic group using the ‘pheatmap’ R package.
Data availability
The authors confirm that the data supporting the findings of this study are available within the article. Additional data that were not published are available and will be shared by request from any qualified investigator.
Results
Description of 10 new patients with anti-AK5 limbic encephalitis
Ten new patients were identified from June 2015 to October 2020, 9/10 (90.0%) male, with a median age of 56years (range 48–94). All patients developed severe episodic amnesia accompanied by depression in 5/10 (50.0%), which was the most frequent psychiatric symptom. Epilepsy was identified in a single patient (Patient 7), a 94-year-old male presenting two clear focal seizures with impaired awareness 2
weeks after a stroke-like episode (also possibly of epileptic nature, though several EEGs were normal), followed by fluctuating altered mental status. Confusion improved progressively, but this led to the discovery of severe cognitive dysfunction; since his previous baseline situation was unknown, longer term, independent cognitive decline cannot be excluded. Four additional patients were also treated at least temporarily with anti-epileptic drugs despite an absence of evident clinical seizures; moreover, EEG recordings were normal in three of them, the remaining showing temporal epileptiform activity without actual discharges. Brain MRI showed temporal lobe fluid-attenuated inversion recovery (FLAIR) hyperintensities in all patients (Fig. 1A), except in Patients 7 and 8; Patient 7 was also the only one without pleocytosis or oligloclonal bands. Additionally, Patient 10 showed right temporal enlargement with contrast enhancement (Fig. 1B), which led to a brain biopsy showing a predominantly T-cell type parenchymal infiltrate of both CD4+ and CD8+ T cells, intense granzyme B expression, and abundant activated macrophages/microglia (Fig. 2). All patients were treated with immunotherapy excepting Patient 7; mild improvement (notably of behavioural disturbances and anxiety) was achieved in only three patients (Table 1).
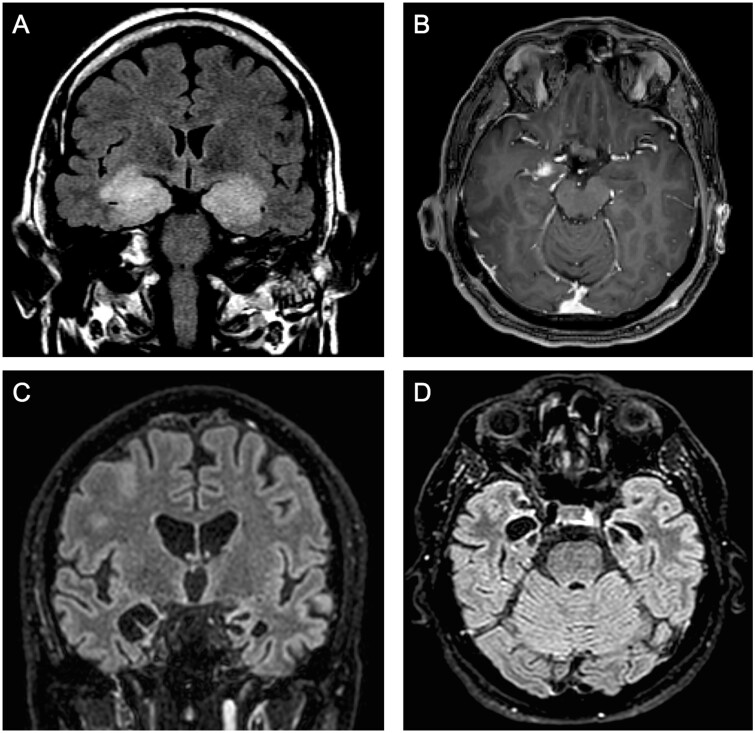
Typical neuroimaging findings in anti-AK5 limbic encephalitis. (A) Coronal FLAIR MRI showing bilateral, enlarged, hyperintense medial temporal lobes from Patient 10. (B) Axial T1 MRI with gadolinium from the same patient, showing right medial temporal contrast enhancement. (C and D) Severe hippocampal atrophy in Patient 8 involving both medial temporal lobes on coronal (C) and axial (D) FLAIR-MRI.
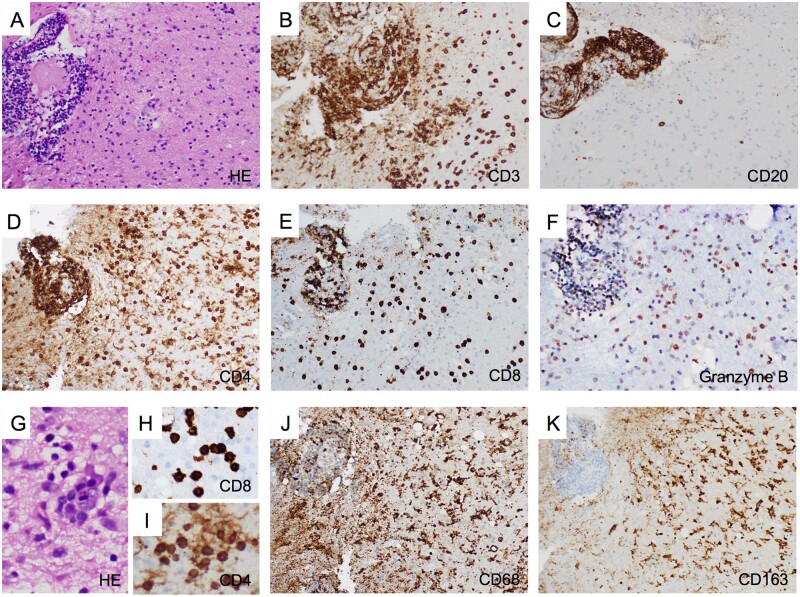
Histopathological characteristics of anti-AK5 limbic encephalitis. Right uncal biopsy from Patient 10 showing intense perivascular and parenchymal inflammatory infiltrates (A, haematoxylin and eosin staining, ×200), which was dominated by T cells that were abundant in both the perivascular space and the parenchyma (B, CD3 immunostaining, ×200). Conversely, B cells, though highly present in the perivascular space, were scattered in the parenchyma (C, CD20 immunostaining, ×200). The T-cell infiltrate included both CD4+ (D, ×200) and CD8+ T cells (E, ×200), which were especially abundant in the perivascular space but also present diffusely in the parenchyma. Cytotoxic CD8+ T cells expressing granzyme B (a protein stored in the granules of cytotoxic cells that induces apoptosis) were detected in perivascular and parenchymal infiltrates (F, ×200). Nodular clusters of inflammatory cells were detected in the parenchyma using haematoxylin and eosin staining (G, ×400) and CD8+ and CD4+ T-cell clusters were also observed (H and I, CD8 and CD4 immunostainings, respectively, ×400). Additionally, macrophages/microglia diffusely infiltrated the tissue, as shown by CD68 (J, ×100) and CD163 (K, ×100) immunostainings, the latter being associated with M2 differentiation related to an anti-inflammatory response including clearance of apoptotic bodies.
Table 1
Clinical characteristics, ancillary tests and treatment of the 10 new patients with anti-AK5 antibodies
Patient Sex, age (years) | Prodromal symptoms | Main clinical featuresa | Brain MRI | Epileptic abnormalities on EEG | CSF | Anti-epileptic drugs | Immuno therapy | Follow-up (mo) | |
---|---|---|---|---|---|---|---|---|---|
1 M, 56 | – | Amnesia, depression, anxiety, apathy, altered circadian rhythm, disinhibited behaviour, psychomotor agitation (6) | Bilateral temporal HS → severe atrophy | No | 29 cells/µl, proteins 0.27![]() ![]() | – | Corticosteroids (i.v.), rituximab, cyclophosphamide | Mild improvement (18) | |
2 M, 80 | Episodes of paleness, instability, and sweating lasting 5–10![]() | Loss of weight (10![]() ![]() | Bilateral temporal HS → severe atrophy | No | 26 cells/µl, proteins 0.49![]() | Lamotrigine | Corticosteroids (i.v. and p.o.), iIVIg, azathioprine | Mild improvement (8) | |
3 M, 54 | Depression, loss of weight (10![]() | Amnesia, prosopagnosia, dysexecutive syndrome (4) | Bilateral temporal HS → severe atrophy | No | 1 cell/µl, proteins 0.6![]() ![]() | – | IVIg, rituximab, cyclophosphamide | Stable (22) | |
4 M, 50 | Asthenia | Amnesia, episodes of anxiety, headache (4) | Bilateral temporal HS → severe atrophy | No | 35 cells/µl, proteins 0.97![]() | Levetiracetam | IVIg, corticosteroids (i.v.), rituximab, cyclophosphamide | Mild improvement (7) | |
5 F, 48 | – | Amnesia, depression, anxiety, headache (3) | Bilateral temporal HS → severe atrophy | Spikes, spike-and -waves T4-T6 | 76 cells/µl, proteins 0.58![]() ![]() | Lacosamide | IVIg, corticosteroids (i.v.), rituximab, cyclophosphamide | Stable (18) | |
6 M, 64 | – | Amnesia, aphasia, prosopagnosia, irritability, headaches, asthenia, loss of weight (2![]() | Bilateral temporal HS → severe atrophy | No | 15 cells/µl, proteins 0.61![]() ![]() | – | IVIg, corticosteroids (i.v. and p.o.), rituximab, cyclophosphamide | Stable (27) | |
7 M, 94 | Asthenia, temporal and spatial disorientation, gait instability | Stroke-like episode of aphasia and right faciobrachial palsy, followed by fluctuating confusion and two focal seizures with impaired awareness. Progressive resolution in 1![]() | Global atrophy, diffuse leukoencephalopathy, small acute ischaemic left paraventricular lesion | No | 5 cells/µl, proteins 1.7![]() ![]() | Levetiracetam | – | Deceased (3) | |
8 M, 64 | Depression | Amnesia, aphasia, impulsivity, irritability, bilateral otalgia, ageusia (4) | Bilateral severe hippocampal atrophy | No | 2 cells/µl, proteins 0.37![]() ![]() | – | Cyclophosphamide | Stable (19) | |
9 M, 54 | Schizophrenia diagnosed 11![]() | Amnesia, aphasia, dysexecutive syndrome (12) | Bilateral temporal HS and hippocampal atrophy | No | 1 cell/µl, proteins 0.73![]() ![]() | – | IVIg | Stable (8) | |
10 M, 57 | – | Amnesia, asthenia, disgeusia, anxiety, psychomotor agitation (8) | Bilateral temporal HS, right Gd+ | No | 9 cells/µl, proteins 0.70![]() ![]() | Levetiracetam, divalproex sodium | Corticosteroids (iv and per os), IVIg, PEX, rituximab, cyclophosphamide | Worsened (5) |
F = female; Gd = gadolinium enhancement; HS = hypersignal; IVIg = intravenous immunoglobulin; M = male; mo = months; NA = not available; OCB = oligloclonal band; PEX = plasma exchange; p.o. = per os. aInstallation in weeks is shown in parentheses.
Clinical characterization of anti-AK5 limbic encephalitis
Including all previously reported cases, patients with anti-AK5 limbic encephalitis were predominantly male (20/26, 76.9%) of median age 66years (range 48–94). No cancer or autoimmune comorbidities were identified. Severe, rapidly progressive (usually <3
months), episodic amnesia was a constant and major feature in all patients, while depression was the most commonly reported psychiatric symptom (17/25, 68.0%), followed by anxiety, behavioural disturbances and psychotic symptoms (11/25, 44.0% each). Epilepsy was absent early in the disease, but seizures were diagnosed in 4/25 (16.0%) patients at late stages (generalized tonic-clonic in three, focal with impaired awareness in one). Particular features were weight loss and/or asthenia and/or anorexia, which were identified in 11/25 (44.0%) patients, as well as new-onset headache (4/25, 16.0%) and anosmia and/or dysgeusia (4/25, 16.0%; Table 2).
Table 2
Main characteristics of all reported patients with anti-AK5 antibodies
n/N (%) | |
---|---|
Males | 20/26 (76.9) |
Clinical features | |
![]() | 25/25 (100) |
![]() | 17/25 (68.0) |
![]() | 11/25 (44.0) |
![]() | 11/25 (44.0) |
![]() | 0/25 (0.0) |
![]() | 4/25 (16.0) |
![]() | 6/25 (24.0) |
![]() | 5/25 (20.0) |
![]() | 11/25 (44.0) |
![]() | 4/25 (16.0) |
![]() | 4/25 (16.0) |
Ancillary tests | |
![]() | 23/26 (88.5) |
![]() | 6/26 (23.1) |
![]() | 17/19 (89.5) |
![]() | 2/17 (11.8) |
![]() | 18/25 (72.0) |
![]() | 18/25 (72.0) |
Treatments | |
![]() | 22/25 (88.0) |
![]() | 13/25 (52.0) |
![]() | 3/25 (12.0) |
Outcome | |
![]() | 8/25 (32.0) |
![]() | 12/25 (48.0) |
![]() | 4/25 (16.0) |
![]() | 1/25 (4.0) |
Regarding the neuroimaging findings, almost all patients (23/26, 88.5%) had temporal lobe FLAIR abnormalities on MRI that evolved into severe hippocampal atrophy in 17/19 (89.5%) patients with available data (Fig. 1C and D). Gadolinium enhancement was observed in 6/26 (23.1%) cases on the first MRI. Only 2/17 (11.8%) patients had epileptiform abnormalities on EEG, though none of them presented epileptic discharges. All patients had CSF abnormalities, including pleocytosis (18/25, 72.0%) and/or oligloclonal bands (18/25, 72.0%). The tau protein CSF level was elevated in 11/14 (78.6%). First-line immunotherapy was used in 22/25 (88.0%) patients, while second-line was utilized in 13/25 (52.0%). Only 5/25 (20.0%) patients responded; moreover, improvement was mild in four of these five patients, and they still had significant cognitive sequelae after therapy (Table 2).
IgG subclasses
IgG subclasses were analysed in nine paired CSF and serum samples belonging to Patients 1–4, 6 and 7 from our previous report2 and Patients 4, 5 and 8 from the present series. All (9/9, 100%) CSF and sera were positive for IgG1, eight (8/9, 88.9%) CSF and seven (7/9, 77.8%) serum paired samples were also positive for IgG2, eight CSF (8/9, 88.9%) and five sera (5/9, 55.6%, all of them CSF-positive) were positive for IgG3, while eight CSF (8/9, 88.9%) and six sera (6/9, 66.7%, all of them CSF-positive) were positive for IgG4. In both CSF and serum, IgG1 was the predominant subclass with considerably higher titres [CSF median=
1/5120, range (1/640–1/40
960); serum median
=
1/51
200, range (1/1600–1/204
800)] than IgG2 [CSF median
=
1/120, range (1/40–1/1280); serum median
=
1/3200, range (1/100–1/25
600)], IgG3 [CSF median
=
1/60, range (1/20–1/320); serum median
=
1/400, range (1/100–1/1600)], and IgG4 [CSF median
=
1/80, range (1/20–1/640); serum median
=
1/1200, range (1/200–1/3200)].
HLA genotyping
DNA was available for 11/15 (73.3%) patients with anti-AK5 limbic encephalitis (Patients 1–4, 6 and 7 from our previous report2 and Patients 1, 3–5 and 8 from the present series), all of Caucasian origin. The following alleles were found to be significantly more common in patients compared to controls: B*08:01 (7/11, 63.6% versus 51/442, 11.5%; corrected P=
0.005808; OR
=
13.4, 95% CI: 3.8–47.4), C*07:01 (8/11, 72.7% versus 86/442, 19.5%; corrected P
=
0.008349; OR
=
11.0, 95% CI: (2.9–42.5), DRB1*03:01 (8/11, 72.7% versus 69/442, 15.6%; corrected P
=
0.002214; OR
=
14.4, 95% CI: 3.7–55.7), DQB1*02:01 (8/11, 72.7%; versus 88/442, 19.9%; corrected P
=
0.00128; OR
=
13.5, 95% CI: 3.5–52.0), and DQA1*05:01 (8/11, 72.7% versus 69/442, 15.6%; corrected P
=
0.000918; OR
=
14.4, 95% CI: 3.7–55.7; Fig. 3A). No other statistically significant difference in allele carrier frequencies was observed (Supplementary Table 1). The class II haplotype DRB1*03:01-DQA1*05:01-DQB1*02:01 was carried by 8/11 (72.7%) patients compared to 69/442 (15.6%) controls (OR
=
14.4, 95% CI: 3.7–55.7); the extended haplotype B*08:01-C*07:01-DRB1*03:01-DQA1*05:01-DQB1*02:01 was carried by 6/11 (54.5%) patients compared to 30/442 (6.8%) controls (OR
=
16.5, 95% CI: 4.8–57.1); and the complete ancestral 8.1 haplotype (A1*01:01-B*08:01-C*07:01-DRB1*03:01-DQA1*05:01-DQB1*02:01) was carried by 5/11 (45.5%) patients, compared to 21/442 (4.8%) controls (OR
=
16.7, 95% CI: 4.7–59.2; Fig. 3B). This result strongly suggests an association with HLA class II DRB1*03:01-DQA1*05:01-DQB1*02:01, with a possible increased risk with the ancestral 8.1 extended haplotype.
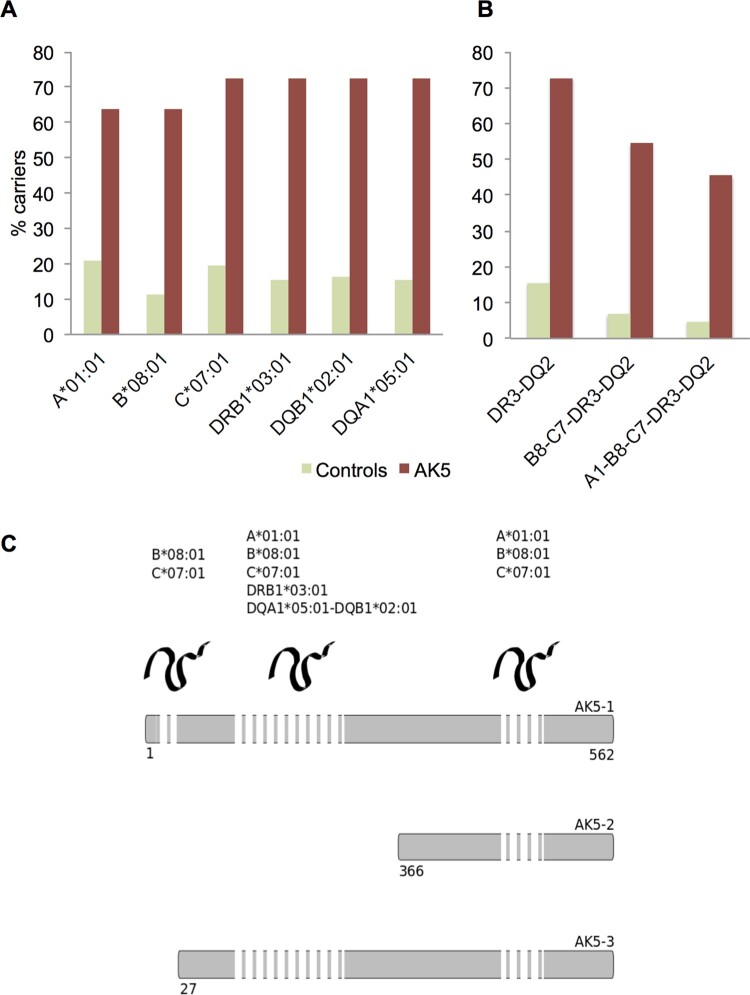
HLA analysis in patients with anti-AK5 limbic encephalitis. (A) Carrier frequencies (%) for the alleles of the ancestral haplotype 8.1 in the control group (n=
442) and anti-AK5 limbic encephalitis patients (n
=
11). (B) Carrier frequencies (%) for different combined components of the ancestral haplotype 8.1 in the control group (n
=
442) and patients with anti-AK5 limbic encephalitis (n
=
11). (C) Location of AK5-derived peptides predicted as strong binders within the protein sequence. AK5-derived peptides predicted as strong HLA binders were located preferentially within the N-terminus of the protein, which is shared by the isoforms 1 and 3. The length of the dashed bar represents the amount of predicted peptides as strong binders for the HLA shown. Numbers correspond to the sequence of the canonical isoform 1.
AK5-derived peptide-binding prediction and search for homologous sequences from pathogens proteins
Thirty-one 9-amino acid-long AK5-derived peptides were predicted as strong binders for HLA class I A*01:01 (n=
6), B*08:01 (n
=
12) and/or C*07:01 (n
=
15). The peptide ASIPVIAYY (positions 524 for AK5 isoform 1, 159 for isoform 2, and 498 for isoform 3) was predicted as a strong binder for A*01:01 and C*07:01; the peptide YFQEKGLIM (positions 288 for AK5 isoform 1, and 262 for isoform 3) was predicted to be a strong binder for B*08:01 and C*07:01. Excepting two peptides located in the first 26 amino acid-long segment of AK5 isoform 1 that is not present in isoform 3, the remaining were all shared by both of these AK5 isoforms; in contrast, only eight were common to AK5 isoform 2. Thus, 23/31 (74.2%) peptides belonged to the N-terminal region (Fig. 3C and Supplementary Table 2).
Five 15-amino acid-long AK5-derived peptides (containing two distinct binding register cores) were predicted as strong binders for DRB1*03:01, while 13 strong binding peptides (six cores) were predicted for the heterodimer DQA1*05:01-DQB1*03:01. No peptide was predicted as strong binder for both DRB1*03:01 and its associated DQ2 heterodimer. All 18 peptides were common to AK5 isoforms 1 and 3, and none for the isoform 2; thus, all peptides predicted to strongly bind DRB1*03:01-DQA1*05:01-DQB1*02:01 were located within the N-terminal region of the AK5 protein (Fig. 3C and Supplementary Table 3).
No relevant homology was found between any of the 46 predicted strong binders and associated eight binding register cores, and peptides sequences from bacteria, viruses and fungi using BLAST. Thirteen additional peptides made up of adjacent or overlapping sequences from previously known peptides were also investigated but no relevant homology was identified (data not shown).
Proteomic analysis and protein networks
Thirty-one proteins were found to have significantly higher expression in the CSF of anti-AK5 patients when compared to both controls and anti-LGI1/CASPR2 limbic encephalitis cases (Supplementary Table 4). These proteins formed a network with significantly much more interactions than expected (enrichment P-value=
3.28
×
10−14). The biological process with the highest significance was formed by 14-3-3 proteins and labelled as positive regulation of protein insertion into mitochondrial membrane involved in apoptotic signalling pathway (GO:1900740, FDR
=
4.81
×
10−11; Fig. 4), followed by positive regulation of establishment of protein localization (GO:1904951, FDR
=
7.61
×
10−7) and positive regulation of transport (GO:0051050, FDR
=
4.90
×
10−6), which mainly involved the same proteins as GO:1900740. The immune system process was the fourth most significant pathway (GO:0002376, FDR
=
7.50
×
10−6; Fig. 4); moreover, both adaptive (GO:0002250, FDR
=
0.00017) and innate (GO:0045087, FDR
=
0.0023) immune response pathways were additionally enriched. Notably, innate immune specific proteins involved were the complement receptor (C1r), complement C4A/B, and CSF3 (granulocyte colony stimulating factor), others related to antigen uptake and processing by dendritic cells and macrophages [macrophage mannose receptor 1, proteasome activator complex subunit 1 (PSME1), lysozyme C, progranulin], as well as those also associated with adaptive responses such as CD209 [also known as dendritic cell-specific intercellular adhesion molecule-3-grabbing non-integrin (DC-SIGN)], hepatitis A virus cellular receptor 2 [HAVCR2, also known as T-cell immunoglobulin and mucin-domain containing-3 (TIM3)] and SLAM family member 7 (SLAMF7) (Fig. 5). Strikingly, we also found increased glial fibrillary acidic protein (GFAP) expression in anti-AK5 patients, suggestive of a glial activation, which is known to occur in response to a CNS injury. All significant pathways are shown in Supplementary Table 5.
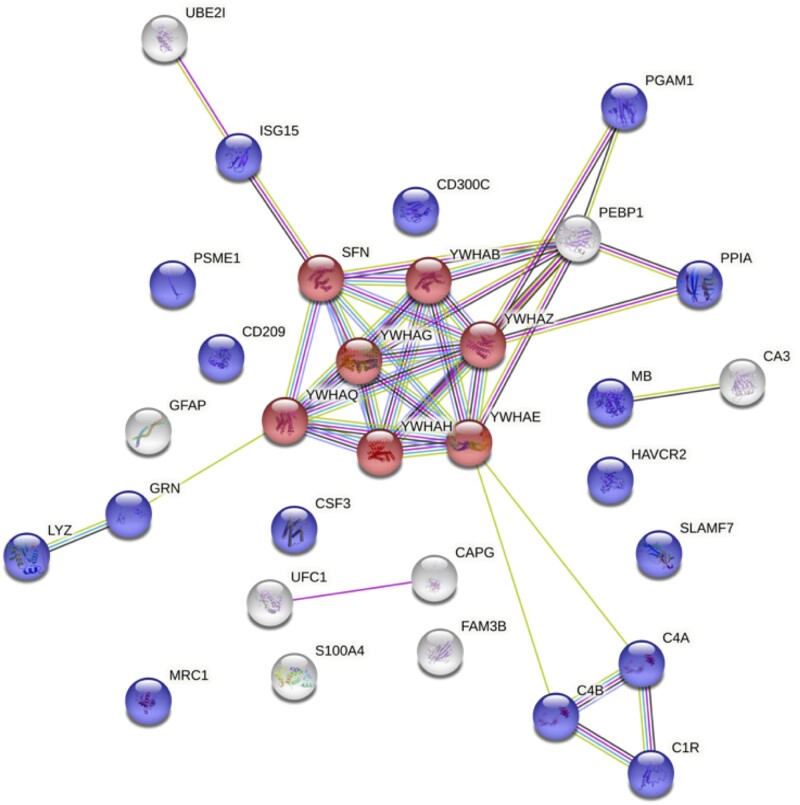
Network of upregulated CSF proteins in anti-AK5 limbic encephalitis compared to anti-LGI1/CASPR2 patients. The two more relevant pathways are highlighted: positive regulation of protein insertion into the mitochondrial membrane involved in apoptotic signalling pathway is coloured in red, while immune system process pathway is coloured in blue. Colour-coded lines indicate an association between two proteins: red, gene fusion; blue, gene co-occurrence; green, genome neighbourhood; black, co-expression; purple, biochemical or genetic experimental data; yellow, text mining; and light blue, known association in curated databases. C1R = complement 1; C4A = complement c4-a; C4B = complement c4-b; CA3 = carbonic anhydrase 3; CAPG = macrophage-capping protein; CD209 = CD 209 antigen; CD300C = CMRF35-like molecule 6; CSF3 = granulocyte colony-stimulating factor; FAM3B = protein FAM3B; GFAP = glial fibrillary acidic protein; GRN = progranulin; HAVCR2 = hepatitis A virus cellular receptor 2; ISG15 = ubiquitin-like protein 15; LYZ = lysozyme C; MB = myoglobin; MRC1 = macrophage mannose receptor 1; PEBP1 = phosphatidylethanolamine-binding protein 1; PGAM1 = phosphoglycerate mutase 1; PPIA = peptidyl-prolyl cis-trans isomerase A; PSME1 = proteasome activator complex subunit 1; S100A4 = protein S100-A4; SFN = protein 14-3-3 sigma; SLAMF7 = SLAM family member 7; UBE2I = SUMO-conjugating enzyme UBC9; UFC1 = ubiquitin-fold modifier-conjugation enzyme 1; YWHAB = protein 14-3-3 beta/alpha; YWHAE = protein 14-3-3 epsilon; YWHAG = protein 14-3-3 gamma; YWHAH = protein 14-3-3 eta; YWHAQ = protein 14-3-3 theta; YWHAZ = protein 14-3-3 zeta/delta.
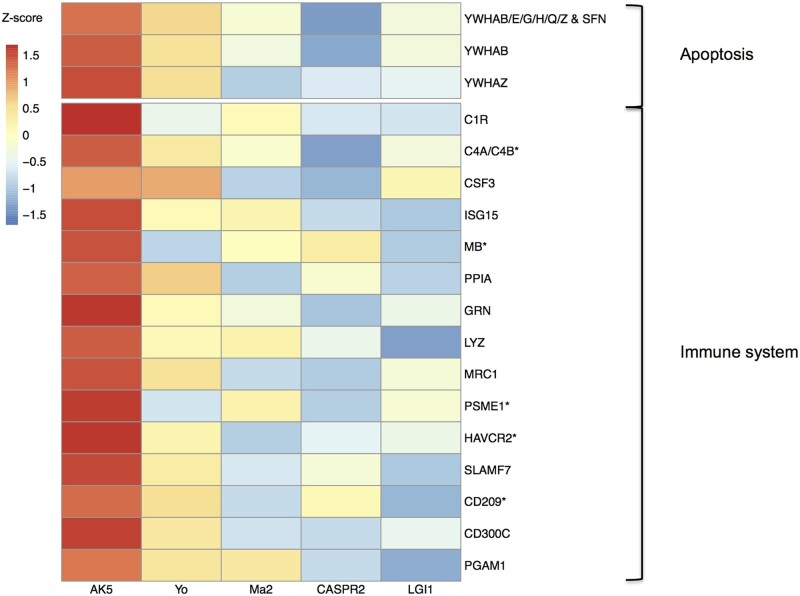
Heat map of CSF expression of proteins involved in the most relevant biological pathways. CSF expression for proteins involved in the two most relevant biological pathways (apoptosis and immune system) is shown as the median Z-score transformed for every protein in each group. All proteins were significantly upexpressed in anti-AK5 patients (n=
5) compared to anti-CASPR2/LGI1 (n
=
5 each) cases, and their expression was also higher when compared to paraneoplastic neurological syndromes with antibodies against Yo/Ma2 (n
=
5 each), although only those marked with an asterisk were statistically significant. C1R = complement 1; C4A = complement c4-a; C4B = complement c4-b; CD209 = CD 209 antigen; CD300C = CMRF35-like molecule 6; CSF3 = granulocyte colony-stimulating factor; GRN = progranulin; HAVCR2 = hepatitis A virus cellular receptor 2; ISG15 = ubiquitin-like protein 15; LYZ = lysozyme C; MB = myoglobin; MRC1 = macrophage mannose receptor 1; PGAM1 = phosphoglycerate mutase 1; PPIA = peptidyl-prolyl cis-trans isomerase A; PSME1 = proteasome activator complex subunit 1; SFN = protein 14-3-3 sigma; SLAMF7 = SLAM family member 7; YWHAB = protein 14-3-3 beta/alpha; YWHAE = protein 14-3-3 epsilon; YWHAG = protein 14-3-3 gamma; YWHAH = protein 14-3-3 eta; YWHAQ = protein 14-3-3 theta; YWHAZ = protein 14-3-3 zeta/delta.
Seven of the aforementioned 31 proteins (carbonic anhydrase 3, C4A/B, CD209, HAVCR2, PSME1 and myoglobin) were also found to have a significantly higher expression in the CSF of anti-AK5 patients when compared to anti-Yo/Ma2 cases (Supplementary Table 4), although the remaining proteins involved in apoptosis and immune system process were also notably upexpressed in anti-AK5 limbic encephalitis (Fig. 5). The network formed by those seven proteins had significantly more interactions than expected (enrichment P-value=
0.021), and the biological processes with the highest significance were again adaptive immune response (GO:0002250, FDR
=
0.0007) and positive regulation of apoptotic cell clearance (GO:2000427, FDR
=
0.00094). All the significant pathways are shown in Supplementary Table 6.
Discussion
Despite anti-AK5 limbic encephalitis being a rare disease, the description herein of 10 new patients, together with the analysis of 16 previously reported cases,1–4 allowed us to better define the clinical characteristics of this disease. Patients with anti-AK5 limbic encephalitis were predominantly males over 60 years of age, a similar demographic profile than that two common non-paraneoplastic limbic encephalitis, anti-LGI1 and anti-CASPR2 limbic encephalitis.6–9,18 Nevertheless, patients with anti-AK5 limbic encephalitis had clinical specificities that clearly differentiated them from patients with anti-LGI1/CASPR2 limbic encephalitis, such as an absence of seizures at disease onset and a constitutional syndrome that preceded the development of cognitive disturbances.
The predominant amnestic-behavioural clinical presentation of anti-AK5 limbic encephalitis is reminiscent of what is observed in neurodegenerative disorders, including prion diseases that often present with a rapidly progressive dementia with constitutional preceding symptoms.24 In addition, the olfactory/taste dysfunction that we found herein, likely a consequence of the damage of the olfactory pathway at the medial temporal lobe level, is also a well-known preclinical symptom of Alzheimer’s and Parkinson’s diseases.25 These clinical similarities contrast with the finding of strikingly inflammatory CSF and MRI abnormalities in patients with anti-AK5 limbic encephalitis. On the contrary, in other autoimmune CNS disorders frequently observed in the elderly, such as anti-LGI1/CASPR2 limbic encephalitis, MRI and routine CSF analysis might be unremarkable in nearly 25% of the patients,26 and, moreover, in those patients with temporal lesions, these are usually smaller, with no mass effect or contrast enhancement.4 Thus, a comprehensive evaluation of potentially autoimmune and treatable conditions should be always performed in patients with rapidly progressive cognitive dysfunction, even in the elderly.
Neuropathological findings observed in anti-AK5 limbic encephalitis resemble those of other neurological diseases with antibodies directed against intracellular proteins, such as onconeural antigens in paraneoplastic neurological syndromes and glutamic acid decarboxylase (GAD).27 Like AK5 and unlike anti-LGI1/CASPR2 limbic encephalitis, these disorders also progress into severe brain atrophy with intense neuronal loss, without immunoglobulin and complement deposition.27,28 Besides, prominent CD8+ T-cell infiltrates and intense granzyme B expression have been described for several paraneoplastic neurological syndromes, while the proportion of cytotoxic lymphocytes in anti-GAD patients was intermediate between that of patients with paraneoplastic neurological syndromes and those with antibodies against surface antigens.27 Herein, we also found abundant cytotoxic granzyme B+ CD8+ T cells in the neuropathology of a patient with anti-AK5 limbic encephalitis, but, strikingly, also a massive, even predominant, CD4+ infiltrate as well as a M2-polarized CD163+ macrophages/microglia.29 These results contrast with those of a necropsy previously reported,30 and are likely a consequence of a different time of sample collection, since an early interaction with CD4+ T cells is necessary to develop a sustained and strong CD8+ T-cell response, which might no longer need the CD4+ cooperation in subsequent activations.31 Similarly, a mixed CD4+ and CD8+ lymphocyte composition has recently been reported in a temporal lobe biopsy from a patient with anti-Kelch-like protein 11 paraneoplastic neurological syndrome.32 Conversely, a clearly predominant CD8+ T-cell infiltrate has been described in classical paraneoplastic neurological syndromes, mostly based on necropsies but also on several biopsies.27,33–36 Thus, it is unknown whether classical paraneoplastic neurological syndromes might also present with abundant CD4+ T-cell infiltrates in early phases of the disease or this aspect is characteristic of anti-AK5 limbic encephalitis. In addition to T cells, macrophage/microglia activation seems to be also important in all these pathologies based on our own results and other previously reported.27,32 Moreover, the pathophysiological mechanisms suggested by neuropathology are also supported by our proteomic analysis, which found enriched pathways involved in innate/adaptive immune responses and apoptosis, likely reflecting the importance of innate effectors and mediators such as macrophages and dendritic cells,37,38 as well as programmed neuronal death induced by cytotoxic T cells.39,40 Despite the proteomic profile of anti-AK5 limbic encephalitis being closer to that of anti-Yo/Ma2 cases than that of anti-LGI1/CASPR2 patients, some differences between the formers were also identified, which along with the aforementioned neuropathological particularities may indicate some distinctiveness of anti-AK5 limbic encephalitis, even when compared to paraneoplastic neurological syndromes with onconeural antibodies. Additionally, frequent inflammatory abnormalities and elevated Tau levels in the CSF as well as presence of temporal MRI hyperintensities, which are accompanied by mass effect and contrast enhancement in some patients, also support the more aggressive nature of anti-AK5 limbic encephalitis compared to other conditions. Moreover, severe hippocampal atrophy develops in most anti-AK5 patients and response to immunotherapy is often unsatisfactory, suggesting that anti-AK5 limbic encephalitis is particularly refractory to current therapies.
The lack of cancer association or other acquired conditions acting as triggers in anti-AK5 limbic encephalitis led us to investigate whether a genetic predisposition may exist in this disease. In the current study, we found that more than 70% of anti-AK5 patients carried DRB1*03:01-DQA1*05:01-DQB1*02:01, with nearly half also having A1*01:01-B*08:01-C*07:01 as part of the extended ancestral HLA haplotype 8.1. Precisely, the strongest association corresponded to the class II haplotype, which further supports an important role of CD4+ T cells in the pathogenesis of anti-AK5 limbic encephalitis as the neuropathology presented herein suggests. Moreover, the haplotype 8.1 is known to be associated with multiple systemic autoimmune diseases, such as type 1 diabetes mellitus or thyroiditis, and the underlying mechanisms of this association are therefore unlikely to be antigen-specific. Furthermore, at least DRB1*03:01 seems to be able to induce specific T-cell responses when presenting several islet and thyroid-derived peptides,41 suggesting that efficient promiscuous epitope recognition might be particularly relevant for this haplotype. In addition, it has been shown that healthy 8.1 carriers have some immune system dysfunctions that might facilitate the development of autoimmunity.42 Indeed, the haplotype 8.1 also includes HLA class III genes such as polymorphisms in the tumour necrosis factor-α promoter region leading to an increased production of this cytokine,43 and a null C4A allele that might impair the removal of immune complexes and increase the synthesis of anti-nuclear antibodies.44 Intriguingly, neurological autoimmune diseases associated so far with the ancestral haplotype 8.1 have antibodies mainly of IgG1 isotype, and most of them are thought to be T-cell mediated,45 such as anti-GAD neurological disorders,46 and anti-Hu paraneoplastic neurological syndromes.47 Likewise, antibodies against AK5 were also predominantly of the IgG1 subclass in the present study, and our and previously reported neuropathological findings showed predominant T-cell infiltrates.30 Together, all these findings suggest that the extended HLA haplotype 8.1 may carry multiple genetic predisposing factors within HLA class I, II, and III loci that coordinate innate, CD4+, CD8+, and B-cell/IgG1-mediated brain autoimmunity.
Following on the HLA association we discovered, we investigated how an anti-AK5 T-cell autoimmune response may develop. Intriguingly, most of the AK5-derived peptides predicted as strong binders for HLA associated alleles were located within the N-terminal region of the protein, a region shared by isoforms 1 and 3 of the enzyme. In contrast, AK5 antibodies reported in the disease have been found to recognize multiple epitopes in both the N- and C-terminal regions of the three AK5 isoforms.2 This suggests that a N-terminal HLA-restricted T-cell response, if primary in the disease, may subsequently lead to epitope-spreading involving the entire AK5 sequence. Furthermore, two peptides predicted as strong binders for HLA class I were located in the initial 26 amino acid-long peptide of AK5 isoform 1, which is absent in AK5 isoform 3 and has been shown to determine the cellular distribution of the enzyme, therefore likely acting as a signal peptide.48 Interestingly, signal peptides seem to be particularly immunogenic due to high CD8+ T-cell epitope density.49 Nevertheless, how this primary response develops is still unknown, as we found no relevant homology of AK5 epitopes with pathogens derived peptides suggestive of molecular mimicry. Similarly, AK5 antibodies have not been found in a large cohort of subjects with other CNS inflammatory disorders, suggesting that neuronal destruction does not easily result in secondary anti-AK5 autoimmunity.1 Interestingly, however, several kinases with predominant brain expression, as is the case for AK5,48 have been described in isolated cases of paraneoplastic neurological syndromes.50–54 Furthermore, the biopsy of one of these patients showed a predominant CD8+ T-cell infiltrate with prominent neuronal apoptosis.54 This suggests that autoimmunity directed against brain kinases may result in severe diseases with CD8+ T-cell-mediated neuronal destruction.
The main limitation of the present study is the small sample size, which prevented precise allele association, hindered in silico predictions, and diminished the statistical power of proteomic analyses, especially between anti-AK5 and paraneoplastic neurological syndrome patients where the differences might be subtler. In addition, it is noteworthy that in silico analyses for peptide-binding prediction do not provide solid evidence but suggest hypothesis that should be further investigated and experimentally demonstrated. The retrospective data collection is another limitation that might underestimate the frequencies of some uncommon symptoms such as anosmia. Nevertheless, considering how rare this disease is, we were able to almost double the number of known anti-AK5 patients, providing novel genetic and proteomic data.
In conclusion, surveying 26 cases of anti-AK5 limbic encephalitis, we found that the disease has very distinctive clinical presentation, outcome, neuropathology, HLA association and CSF protein expression features compared with other conditions, especially the most common non-paraneoplastic anti-LGI1/CASPR2 limbic encephalitis affecting the same age group. This likely reflects a different pathogenesis and warrants a search for novel therapeutic approaches.
Acknowledgements
We thank NeuroBioTec Hospices Civils de Lyon BRC (France, AC-2013-1867, NFS96-900) for banking sera and CSF samples. We thank Dr Clarise Carra (Neurology Department, Centre Hospitalier Universitaire de Montpellier, Montpellier, France), Dr Mary Ann McKee (Neurology Department, Albany Medical Center Hospital, Albany, NY, USA), Pr Jérémie Pariente (Neurology Department, Hôpital Pierre-Paul Riquet, Toulouse, France), and Pr Damien Ricard (Neurology Department, Hôpital d’Instruction des Armées Percy, Clamart, France) for their help in data collection.
Funding
This study is supported by Fondation pour la recherche médicale (FRM-DQ20170336751). This work has been developed within the BETPSY project, which is supported by a public grant overseen by the Agence Nationale de la Recherche (ANR) as part of the second Investissements d’Avenir program (ANR-18-RHUS-0012), and has been performed within the framework of the LABEX CORTEX (ANR-11-LABX-0042) of Université de Lyon operated by the ANR. S.M.-C. is supported by a research grant from Fundación Alfonso Martín Escudero (Spain). E.M. and A.A. were supported by National Institutes of Health (NIH-1U01NS120885).
Competing interests
The authors report no competing interests.
Supplementary material
Supplementary material is available at Brain online.
References
Articles from Brain are provided here courtesy of Oxford University Press
Full text links
Read article at publisher's site: https://doi.org/10.1093/brain/awab153
Read article for free, from open access legal sources, via Unpaywall:
https://academic.oup.com/brain/article-pdf/144/9/2709/40880402/awab153.pdf
Citations & impact
Impact metrics
Citations of article over time
Alternative metrics

Discover the attention surrounding your research
https://www.altmetric.com/details/103967591
Smart citations by scite.ai
Explore citation contexts and check if this article has been
supported or disputed.
https://scite.ai/reports/10.1093/brain/awab153
Article citations
HLA-DR3 ~ DQ2 associates with sensory neuropathy in paraneoplastic neurological syndromes with Hu antibodies.
J Neurol, 271(9):6336-6342, 11 Jul 2024
Cited by: 0 articles | PMID: 38990347 | PMCID: PMC11377461
MR Imaging Findings in Anti-Leucine-Rich Glioma Inactivated Protein 1 Encephalitis: A Systematic Review and Meta-analysis.
AJNR Am J Neuroradiol, 45(7):977-986, 08 Jul 2024
Cited by: 0 articles | PMID: 38871367
Review
Autoimmune encephalitis: what the radiologist needs to know.
Neuroradiology, 66(5):653-675, 20 Mar 2024
Cited by: 2 articles | PMID: 38507081 | PMCID: PMC11031487
Review Free full text in Europe PMC
Anti-adenylate kinase 5 encephalitis: Clinical characteristics, diagnosis, and management of this rare entity.
J Transl Autoimmun, 7:100218, 12 Oct 2023
Cited by: 1 article | PMID: 37859804 | PMCID: PMC10582738
Review Free full text in Europe PMC
Autoimmune Encephalitis Criteria in Clinical Practice.
Neurol Clin Pract, 13(3):e200151, 25 Apr 2023
Cited by: 12 articles | PMID: 37124463 | PMCID: PMC10132262
Go to all (13) article citations
Data
Data behind the article
This data has been text mined from the article, or deposited into data resources.
BioStudies: supplemental material and supporting data
Genes & Proteins
- (1 citation) UniProt - Q9Y6K8
Quick GO (Showing 6 of 6)
- (1 citation) GO - GO2000427
- (1 citation) GO - GO1904951
- (1 citation) GO - GO0045087
- (1 citation) GO - GO1900740
- (1 citation) GO - GO0002250
- (1 citation) GO - GO0051050
Show less
Similar Articles
To arrive at the top five similar articles we use a word-weighted algorithm to compare words from the Title and Abstract of each citation.
Adenylate kinase 5 autoimmunity in treatment refractory limbic encephalitis.
J Neuroimmunol, 186(1-2):177-180, 25 Apr 2007
Cited by: 25 articles | PMID: 17462746 | PMCID: PMC2040128
Characteristics in limbic encephalitis with anti-adenylate kinase 5 autoantibodies.
Neurology, 88(6):514-524, 06 Jan 2017
Cited by: 27 articles | PMID: 28062719
Adenylate kinase 5 (AK5) autoimmune encephalitis: Clinical presentations and outcomes in three new patients.
J Neuroimmunol, 367:577861, 04 Apr 2022
Cited by: 2 articles | PMID: 35405429 | PMCID: PMC9186138
Identification of adenylate kinase 5 antibodies during routine diagnostics in a tissue-based assay: Three new cases and a review of the literature.
J Neuroimmunol, 334:576975, 29 May 2019
Cited by: 7 articles | PMID: 31177032
Review
Funding
Funders who supported this work.
Agence Nationale de la Recherche (1)
Grant ID: ANR-18-RHUS-0012
Fondation pour la recherche médicale (1)
Grant ID: FRM-DQ20170336751
Fundación Alfonso Martín Escudero
LABEX CORTEX (1)
Grant ID: ANR-11-LABX-0042
NINDS NIH HHS (1)
Grant ID: U01 NS120885
National Institutes of Health (1)
Grant ID: NIH-1U01NS120885