Abstract
Background
Induction of autoantigen-specific Treg cells that suppress tissue-specific autoimmunity without compromising beneficial immune responses is the holy-grail for immunotherapy to autoimmune diseases.Methods
In a model of experimental autoimmune uveitis (EAU) that mimics human uveitis, ocular inflammation was induced by immunization with retinal antigen interphotoreceptor retinoid-binding protein (IRBP). Mice were given intraperitoneal injection of αCD4 antibody (Ab) after the onset of disease, followed by administration of IRBP. EAU was evaluated clinically and functionally. Splenocytes, CD4+CD25- and CD4+CD25+ T cells were sorted and cultured with IRBP or αCD3 Ab. T cell proliferation and cytokine production were assessed.Findings
The experimental approach resulted in remission of ocular inflammation and rescue of visual function in mice with established EAU. Mechanistically, the therapeutic effect was mediated by induction of antigen-specific Treg cells that inhibited IRBP-driven Th17 response in TGF-β and IL-10 dependent fashion. Importantly, the Ab-mediated immune tolerance could be achieved in EAU mice by administration of retinal autoantigens, arrestin but not limited to IRBP only, in an antigen-nonspecific bystander manner. Further, these EAU-suppressed tolerized mice did not compromise their anti-tumor T immunity in melanoma model.Interpretation
We successfully addressed a specific immunotherapy of EAU by in vivo induction of autoantigen-specific Treg cells without compromising host overall T cell immunity, which should have potential implication for patients with autoimmune uveitis.Funding
This study was supported by the Natural Science Foundation of Guangdong Province and the Fundamental Research Fund of the State Key Laboratory of Ophthalmology, Zhongshan Ophthalmic Center.Free full text

Induction of antigen-specific Treg cells in treating autoimmune uveitis via bystander suppressive pathways without compromising anti-tumor immunity
Associated Data
Abstract
Background
Induction of autoantigen-specific Treg cells that suppress tissue-specific autoimmunity without compromising beneficial immune responses is the holy-grail for immunotherapy to autoimmune diseases.
Methods
In a model of experimental autoimmune uveitis (EAU) that mimics human uveitis, ocular inflammation was induced by immunization with retinal antigen interphotoreceptor retinoid-binding protein (IRBP). Mice were given intraperitoneal injection of αCD4 antibody (Ab) after the onset of disease, followed by administration of IRBP. EAU was evaluated clinically and functionally. Splenocytes, CD4+CD25− and CD4+CD25+ T cells were sorted and cultured with IRBP or αCD3 Ab. T cell proliferation and cytokine production were assessed.
Findings
The experimental approach resulted in remission of ocular inflammation and rescue of visual function in mice with established EAU. Mechanistically, the therapeutic effect was mediated by induction of antigen-specific Treg cells that inhibited IRBP-driven Th17 response in TGF-β and IL-10 dependent fashion. Importantly, the Ab-mediated immune tolerance could be achieved in EAU mice by administration of retinal autoantigens, arrestin but not limited to IRBP only, in an antigen-nonspecific bystander manner. Further, these EAU-suppressed tolerized mice did not compromise their anti-tumor T immunity in melanoma model.
Interpretation
We successfully addressed a specific immunotherapy of EAU by in vivo induction of autoantigen-specific Treg cells without compromising host overall T cell immunity, which should have potential implication for patients with autoimmune uveitis.
Funding
This study was supported by the Natural Science Foundation of Guangdong Province and the Fundamental Research Fund of the State Key Laboratory of Ophthalmology, Zhongshan Ophthalmic Center.
1. Introduction
CD4+Foxp3+ regulatory T (Treg) cells have a critical role in the maintenance of immune tolerance and prevention of autoimmunity. Deficiencies in Treg cell development or function result in uncontrolled immune responses accordingly and can lead to inflammatory disorders and autoimmune diseases. Manipulating Treg cells is a promising strategy to treat autoimmunity [1], [2], [3], [4], [5], [6]. However, their clinical applications have been limited to a large extent due to the complexity of human antigens and the difficulty of expanding thymus-derived natural Treg cells. The discovery of TGF-β-mediated inducible Treg cells from peripheral naïve CD4+ T cells has created new opportunities for inducing antigen-specific Treg cells to treat autoimmunity [7,8]. So far, most reports have been limited to the prevention of experimental diseases by pre-treatment of in vitro induced Treg cells in naïve mice or mice before the disease is well established [8], [9], [10]. Due to a huge difference in the immune status between a naïve mouse and a mouse with established inflammation, it was difficult to induce antigen-specific Treg cells in a dysregulated immune system to specifically suppress disease. Upon the plasticity and instability of Treg cells, their suppressive capacity in the immune quiescent state in naïve mice may loss or these cells even convert to effector T cells under the dysregulated immunity in those disease has already developed [11]. This problem is particularly salient in clinical settings, in which patients with autoimmune disease present with a dysregulated immune response. One approach that has been explored is the development of Treg cell–based therapy based on the adoptive transfer of polyclonal Treg cells into patients with autoimmunity, in which may lead to the consequences of antigen-nonspecifically suppressing host defense and T cell immunity [12]. Therefore, the potential therapeutic approach to the restoration of peripheral tolerance in patients is to generate antigen-specific Treg cells in their dysregulated immune system to specifically suppress disease in the target organs without compromising beneficial immune responses.
Intraocular inflammation is a common ophthalmic complication that can lead to visual impairment. Autoimmune uveitis refers to a group of chronic intraocular inflammation, including Behçet's disease, birdshot retinochoroidopathy, Vogt-Koyanagi-Harada disease, Sarcoidosis, and sympathetic ophthalmia [13]. As a sight-threatening autoimmune disease of central nervous system (CNS), uveitis can cause irreversible ocular tissue damage and eventually impaired vision if left untreated, and is estimated to account for approximately 10-15% of blindness in the developed countries [14]. Currently, corticosteroids are the main therapeutic agents for the treatment of uveitis although they may cause side effects such as cataracts, glaucoma, stomach ulcers and osteoporosis and an increased risk of infections and malignancies [13]. Novel therapeutic approaches are needed. The suppressive action of Treg cells in the pathogenesis of human uveitis has been studied and supported by the findings of a correlation between increase in the proportions of Treg cells in patients’ peripheral blood and remission of the disease [15] and decrease in the frequency of Treg cells in parallel with increase in the disease [16]. However, no data have been reported to achieve successful treatment of uveitis patients with Treg cell-based therapy so far.
Experimental autoimmune uveitis (EAU) is a classical model of human uveitis and can be induced in mice by immunization with retinal antigen, interphotoreceptor retinoid-binding protein (IRBP) [17], [18], [19]. EAU shares pathological features with human uveitis, and much of our understanding of pathophysiology of the disease derives from studies of EAU [18,20,21]. It is generally believed that both Th1 and Th17 effector responses are involved in the pathogenesis of human uveitis and EAU model although Th17 effector response has a critical role [20,22]. Using a T-cell transfer model of uveitis, recent study by Salomon group proposed an approach to achieve suppression of ocular inflammation by local intravitreal injection of pre-activated polyclonal Treg cells [23]. However, local delivery of retinal antigen-specific Treg cells to the inflamed eyes does not suppress EAU but escalates the disease limited to the plasticity and instability of Treg cells under conditions of ocular inflammation [24], [25], [26].
We have previously established a system (designated as T cell apoptosis-antigen therapy) to generate antigen-specific Treg cells in vivo and demonstrated therapeutic effects of this approach in experimental autoimmune encephalomyelitis (EAE), a model of multiple sclerosis (MS) [27,28]. In the present study, using the mouse model of EAU, we sought to induce antigen-specific Treg cells in vivo by deleting pathogenic T effectors using antibody (Ab) against CD4 followed by administration of retinal autoantigens (e.g., IRBP or arrestin) in treating EAU. We obtained significant therapeutic effects of this approach on remission of ocular inflammation and rescue of visual function by suppressing IRBP-specific Th17 and Th1 effector responses. Further, we wished to determine whether the immune tolerance could be achieved in mice with active EAU by administration of one or few retinal antigens that is not limited to IRBP, and whether this therapeutic approach would compromise the host overall T cell immunity. As a result, we have discovered a specific immunotherapy of EAU by in vivo induction of antigen-specific Treg cells to the eyes in mice with established disease via bystander suppressive pathways, which should be vital for transferring this approach to clinical studies in patients with CNS autoimmune diseases.
2. Materials and methods
2.1. Mice
C57BL/6, IL-10−/− and Foxp3-GFP reporter mice were purchased from the Jackson Laboratory. Mice were housed in a pathogen-free facility in compliance with institutional guidelines. The animal studies were approved and performed under the Animal Care and Use Committee of the Zhongshan Ophthalmic Center, Sun Yat-sen University (ref no. 2015-108).
2.2. Induction and evaluation of EAU
EAU was induced using 150 μg IRBP1-20 emulsified in an equal volume of CFA containing 2.5 mg/ml Mycobacterium tuberculosis strain 37RA (Sigma-Aldrich) and 0.5 μg of Bordetella pertussis toxin as described [18]. Clinical EAU severity was evaluated by retinal fluorescent imaging system (field of view 1.8 mm) (Phoenix Micron IV)[29,30] and scored on a scale of 0-4 as follows [18,31]: 0.5, mild vasculitis and focal lesions; 1, moderate vasculitis, focal and lineal lesions; 2, severe vasculitis and infiltrations, multiple chorioretinal lesions; 3, confluent lesions, retinal hemorrhages; and 4, retinal detachment, retinal atrophy.
2.3. T cell apoptosis-antigen treatment
Wild-type (WT), Foxp3-GFP reporter and IL-10−/− mice on C57BL/6 background were given an i.p injection of PBS or αCD4 Ab (GK1.5, 100 μg/mouse) at the onset of disease (day 14 post-immunization), followed by i.p. injection of 3 μg/mouse of IRBP1-20, arrestin (HLA-B27/B27PD), MOG35-55 or OVA (Sigma) every other day from day 15 to day 25 post-immunization. To investigate the effect of TGF-β, mice were given i.p. injection of αTGF-β Ab (1D1.16.8, 200 μg/mouse) or isotype control IgG1 on alternative day for 4 times, starting from day 15 to day 21 post-immunization. Ab used for in vivo treatment were purchased from BioXCell. Peptides were purchased from the Shanghai Hanhong Chemical Co Ltd and prepared in PBS for i.p. injection.
2.4. Electroretinography (ERG)
Retinal function was evaluated using an Espion E2 System (Diagnosys LLC) as described [18,32]. Mice were dark adapted for overnight before recording, and all procedures were performed under dim red light.
2.5. Isolation of eye-infiltrating cells
Eyes were dissected, digested in RPMI containing 10% FBS and 1 mg/ml collagenase D (Sigma-Aldrich) and then incubated for 1 hr at 37°C. Single cell suspension was prepared for flow cytometry analysis as described [19]. Eyes from naïve mice did not yield leukocytes for analysis.
2.6. Flow cytometry (FCM)
Single-cell suspensions were prepared by conventional methods. Cells were stained using anti-mouse CD3 (17A2), CD4 (GK1.5), CD8a (53-6.7), CD25 (PC61), Foxp3 (FJK-16s), CD11b (M1/70), CD11c (N418), Gr1 (RB6-8C5) Ab or isotype-control Ab. For intracellular cytokine staining with anti-IFN-γ (XMG1.2) and anti-IL-17A (TC11-18H10) Abs, cells were incubated for 4 hr with PMA (50 ng/ml, Sigma) and ionomycin (500 ng/ml, Sigma) in the presence of GolgiStop (BD) as described [19]. Data were acquired and analyzed using a BD LSRFortessa analyzer and FlowJo software (Tree Star Inc).
2.7. T cell activation and cytokine assays
Cells were isolated from the spleens and draining lymphoid nodes (dLNs) 28 days after EAU induction and stimulated with IRBP or soluble αCD3 (17A2)/αCD28 (37.51) Abs in serum-free medium for 48 hr[19,22]. Cytokines were measured from the cultured supernatants by ELISA: IFN-γ, IL-17A, TNF-α, and IL-6 (eBioscience).
2.8. Real-Time PCR
mRNA was extracted (STAT-60, Tel-Test Inc) and converted into cDNA (High-Capacity cDNA RT kit, Applied Biosystems). Real-time PCR was performed with an ABI 7500 detection system for quantitation of targeted genes (Table S1). The relative fold changes of indicated genes were calculated by using 2−ΔΔCT methods and normalized to GADPH.
2.9. T-cell suppression assay
CD4+CD25− T responding cells (Tresp) and CD3− APCs were sorted from the spleens of PBS-treated EAU mice using a FACSAria Fusion cell sorter (BD Biosciences). Tresp cells were labeled with Far-Red dye (Invitrogen) and cultured with CD4+CD25hi Treg cells (over 95% Foxp3+) sorted from the spleens of EAU mice treated with PBS or αCD4+IRBP, under stimulation of αCD3 Ab or IRBP for 3-4 days. Proliferation of Far Red-labeled Tresp cells was assessed by FCM.
2.10. Adoptive transfer of Treg cells
Foxp3-GFP reporter donor mice with established EAU were given an i.p injection of PBS or αCD4 Ab on day 14 post-immunization, followed by i.p. injection of IRBP or MOG every other day for 6 times. A single cell suspension from pooled lymphoid tissues was prepared for isolation of CD4+ T cells (EasySep™ Mouse CD4 T Cell Isolation Kit, StemCell) and subsequent sorting of CD4+Foxp3+GFP+ Treg cells using a FACSAria Fusion cell sorter (BD Biosciences) on days 28-29 post-immunization. CD4+Foxp3+ Treg cells (1.5 × 106 cells/mouse) were adoptively transferred i.v. into naive recipient mice. One day after cell transfer, recipient mice were immunized for EAU induction, and their clinical severity were evaluated by retinal fluorescent imaging system (Phoenix Micron IV).
2.11. Induction of melanoma
B16 melanoma cells (ATCC, RRID: CVCL_0604) were cultured in DMEM supplemented with 10% FBS. Melanoma [19,33] was induced in naïve mice or EAU mice (day 40 post-immunization) by subcutaneously injection of tumor cells (0.5 × 106 cells/mouse), and mice were monitored daily for survival, tumor growth and tumor size. At the end of the experiment, tissues were harvested and cells isolated from the spleens and tumors were assessed for FCM. Recent mycoplasma testing was not performed.
2.12. Statistics
Mann-Whitney U test for unpaired data and ANOVA for multiple comparisons, and Prism 6 (GraphPad) was used for statistical analysis.
2.13. Reagent Validation
All reagents and their resources are provided in Table S2.
2.14. Role of funders
The Funders had no role in study design, data collection, data analysis, interpretation, or writing of report.
3. Results
3.1. Treatment with αCD4 Ab and IRBP peptides ameliorates EAU by inhibiting uveitogenic Th17 and Th1 immune responses
We and other groups have shown previously that the T cell apoptosis-antigen therapy induces tolerance in animal models of CNS autoimmunity including EAE in a phagocyte-Treg dependent manner through sensing and digesting apoptotic cells [27,[34], [35], [36]]. Based on the previous findings, we hypothesized that tissue-specific immune tolerance could be improved by deleting pathogenic T effectors using Ab against CD4 followed by administration of autoantigen peptides. To address this, we first determined that a single injection of αCD4 Ab (100 μg) eliminated the cells transiently as demonstrated in the spleens of naive mice (Fig. S1a), which was accompanied with a relative increase in the frequency of CD4+Foxp3+ Treg cells within the remaining CD4+ T cells (Fig. S1b). Despite an increase in number of CD11c−CD11b+ cells in the spleens, αCD4 Ab treatment did not affect the population of CD8+ and CD4+CD11b+ subsets (Fig. S2). We then confirmed the findings in EAU model, showing that injection of αCD4 Ab increased the frequency of CD4+Foxp3+ Treg cells in the spleens (Fig. S3a). The administration of αCD4 Ab only could deplete pathogenic CD4+ T effectors to reduce EAU disease transiently, in which the therapeutic effect disappeared within 14 days after Ab injection (Fig. S3b).
After observing the effects of αCD4 Ab-mediated Treg induction, we next investigated whether transient CD4+ T cell depletion followed by retinal antigen IRBP administration could ameliorate established disease in EAU mice. Indeed, we found that administration of αCD4 Ab with IRBP peptides significantly inhibited EAU, leading to over 2-month remission of ocular inflammation (Fig. 1a). Effective treatment with αCD4 and IRBP peptides (αCD4+IRBP) was manifested by a remarkable reduction in vasculitis/retinitis and perivascular cellular exudates assessed by fundus examination and fluorescein angiography (Fig. 1b). In contrast, administration of αCD4 Ab with nonself-antigen ovalbumin (OVA) (αCD4+OVA) failed to inhibit the severity of EAU disease within 14 days after treatment. Notably, electroretinography (ERG)[37] determined significant improvement of visual function in the eyes of tolerized mice (αCD4+IRBP) compared to control groups (PBS; αCD4+OVA), and the ERG amplitudes detected in the eyes of tolerized mice were almost same to those in naive mice 60 days after EAU induction (Fig. 1c). Consistent with reduction of ocular inflammation, we found that the number of eye-infiltrating CD4+ T cells were significantly decreased in the tolerized mice compared to control groups (Fig. 1d), which was accompanied with a decrease in frequency of other inflammatory immune cells (e.g., CD11c+, CD11b+, Gr1+ cells) (Fig. S4). The frequency of IL-17A producing inflammatory cells including IL-17+IFN-γ− and IL-17+IFN-γ+ CD4+ T cells were substantially decreased in the eyes of tolerized mice compared to control groups on day 28, and even more profound on day 60 after EAU induction (Fig. 1e). In the spleen, the tolerized mice showed significantly reduced IL-17A and IFN-γ production by T effectors in response to in vitro IRBP stimulation compared to control groups, without altering over all αCD3/αCD28-induced IL-17A and IFN-γ production (Fig. 1f). Reduced level of IRBP-specific TNF-α was also detected in the spleens of tolerized mice (Fig. S5). Collectively, these data demonstrate that administration of αCD4 Ab with retinal antigen IRBP achieves remission of ocular inflammation and improvement of visual function in mice with established EAU disease, by inhibiting IRBP-driven Th17 and Th1 immune responses.
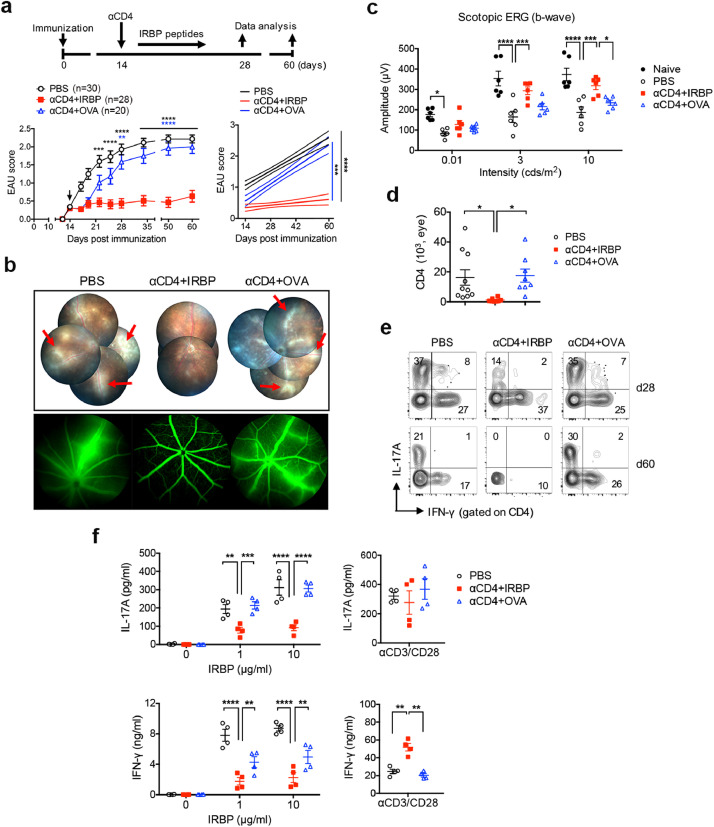
Treatment with αCD4 Ab and IRBP peptides ameliorates EAU by inhibiting uveitogenic Th17 and Th1 immune responses. Mice were immunized for EAU induction and received i.p injection of PBS or αCD4 Ab on day 14 post immunization at the onset of active disease, and followed by i.p. injection of IRBP or OVA on alternative day for 6 times. Mice were monitored at the indicative time-point by fundus examination and ERG. (a) Experimental scheme and clinical scores of EAU in mice treated with PBS, αCD4+IRBP or αCD4+OVA. Arrowhead indicates the initiation of treatment. **p<0.01, ***p<0.001, ****p<0.0001, two-way ANOVA (left); linear regression curve including the 95% confidence band of the regression line. ***p<0.001, ****p<0.0001 (right). (b) Representative retinal images assessed by fundoscopy (upper) and fluorescein angiography (bottom) on day 28 post immunization. Red arrows indicate vasculitis and perivascular cellular exudates. (c) Changes in retinal function accessed by ERG. Amplitudes of scotopic ERG were recorded and analyzed in mice on day 60 post immunization. Data represent the means ± SEM of a representative of 3 individual experiments. *p<0.05, ***p<0.001, ****p<0.0001, two-way ANOVA. (d) Absolute number of the eye-infiltrating CD4+ T cells assessed by FCM on day 28 post immunization. Data represent the means ± SEM of 3 individual experiments. *p<0.05, one-way ANOVA. (e) Representative FCM plot of IFN-γ and IL-17A expression in the eye-infiltrating CD4+ T cells on days 28 and 60 post immunization. (f) IRBP-specific IL-17A and IFN-γ production in the spleens of EAU mice with the indicated treatment. Cells were isolated on day 28 post immunization and stimulated with IRBP or αCD3/αCD28 for 48 hr. Cytokines were measured from the cultured supernatants by ELISA. Data represent the means ± SEM of a representative of 3 individual experiments. **p<0.01, ***p<0.001, ****p<0.0001, two-way ANOVA.
3.2. Ab-mediated T cell apoptosis promotes Treg cell induction through TGF-β and IL-10 produced by phagocytic APCs
To explore the underlying mechanisms for T cell apoptosis-antigen therapy, we first verified αCD4-mediated cell apoptosis, showing that injection of αCD4 Ab rapidly increased the frequency of Annexin-V expressing CD4+ T cells in the spleens of naïve mice in a time-dependent fashion (Fig. 2a). As phagocytic antigen-presenting cells (APCs), including macrophages and dendritic cells, produce TGF-β and IL-10 after ingestion of apoptotic cells[34,38,39], we next established an in vitro culture system to examine the capacity of phacocytic APCs to induce Treg cells. As shown in Fig. 2b, mice were immunized for EAU and received αCD4 Ab on day 14 post immunization. CD3-depleted APCs were sorted from the spleens of Ab-treated EAU mice 14 days later when tolerance was established and co-cultured with naïve CD4+ T cells sorted from naïve mice in response to αCD3 stimulation for 5 days. We found that expression of TGF-β1 and IL-10 were highly induced from the splenic APCs of Αb-treated EAU mice than PBS-treated mice (Fig. 2c). Subsequently, co-culture of naïve CD4+ cells from naïve mice with the splenic APCs of Ab-treated EAU mice significantly increased the frequency of Foxp3+ Treg cells compared to those APCs from the untreated EAU mice (Fig. 2d). These results indicate that Ab-mediated T cell apoptosis enhances TGF-β and IL-10 production by phagocytic APCs to promote Treg cell induction.
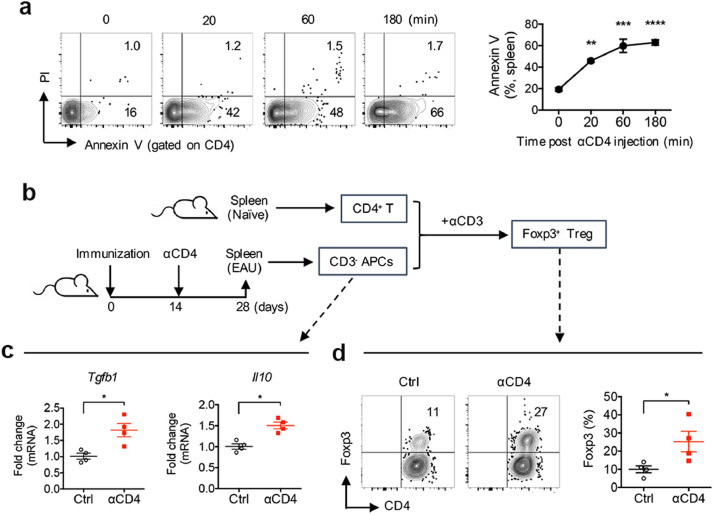
Ab-mediated CD4+ T cell apoptosis enhances TGF-β and IL-10 production by phagocytic APCs to promote Treg cell induction. (a) Representative FCM plot and frequency of Annexin-V expressing CD4+ T cells in the spleens of naïve mice at the indicated time-point after i.p. injection of αCD4 Ab. **p<0.01, ***p<0.001, ****p<0.0001, one-way ANOVA. (b) Experimental scheme of an in vitro culture system. Mice were immunized for EAU induction and received αCD4 Ab injection on day 14 post immunization. CD3− APCs were sorted from their spleens 14 days later and co-cultured with naïve CD4+ T cells sorted from naïve mice with αCD3 Ab stimulation for 5 days. (c) Expression of TGF-β1 and IL-10 in phacocytic APCs from the spleens of Ab-treated EAU mice was determined by real-time PCR, and (d) frequency of Foxp3+ Treg cells induced from naïve T cells co-cultured with the splenic APCs was determined by FCM. Data represent the means ± SEM. *p<0.05, Mann-Whitney U test.
3.3. In vivo generation of IRBP-specific Treg cells by αCD4 and IRBP treatment is vital to ameliorate EAU
Upon the ineffective treatment with αCD4 Ab alone or αCD4+OVA seen in EAU mice, we hypothesized that administration of αCD4 Ab combined with retinal autoantigen IRBP could induce immune tolerance specific to the eyes in suppressing EAU. Consistent to reduction of EAU disease (Fig. 1a), we found evidence showing that the frequency of Foxp3+ Treg cells in the eyes was significantly increased in the tolerized mice treated with αCD4+IRBP compared to untreated (PBS) mice or to mice treated with αCD4+OVA, despite an increase in Foxp3+ Treg cells seen in the spleens of mice treated with αCD4+OVA (Fig. 3a). We then asked whether there is an increase in retinal antigen IRBP-specific Treg cells in the tolerized mice treated with αCD4 and IRBP. To address this, we performed an in vitro T-cell suppression assay by using CD4+CD25high Treg cells from EAU mice treated with PBS or αCD4+IRBP (Fig. S6) and compared their suppressive capacities to T cell proliferation. Notably, we found that CD4+CD25high Treg cells from the tolerized mice treated with αCD4+IRBP were highly suppressive to IRBP-driven T cell proliferation compared to Treg cells from untreated (PBS) mice (Figs. 3b, 33c). In contrast, the CD4+CD25high Treg cells from the tolerized mice (αCD4+IRBP) exhibited same degree of suppression to the αCD3-driven T cell proliferation as those from untreated (PBS) mice (Figs. 3b, 33c), indicating an increase in IRBP-specific Treg cells in the tolerized mice treated with αCD4 and IRBP.
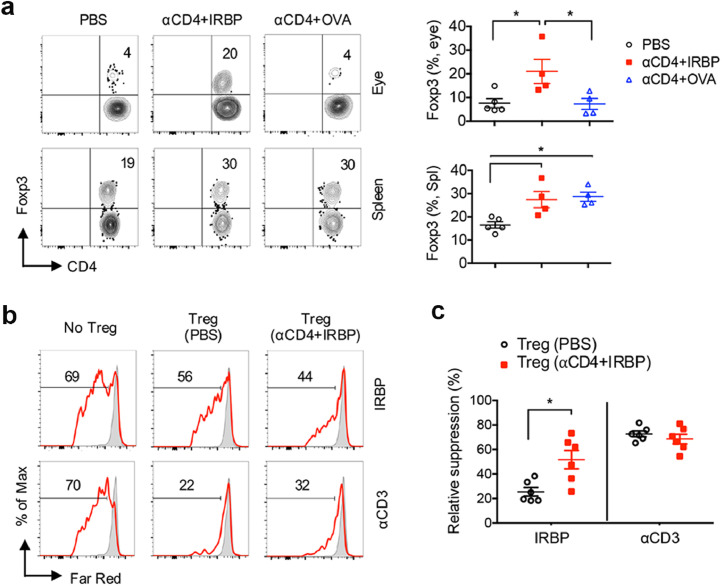
In vivo induction of IRBP-specific Treg cells by αCD4 and IRBP treatment is essential to ameliorate EAU. (a) Representative FCM plot and frequency of Foxp3+ Treg cells in the eyes and spleens of EAU mice treated with PBS, αCD4+IRBP or αCD4+OVA on day 28 post immunization. Data represent the means ± SEM. *p<0.05, one-way ANOVA. (b,c) CD4+CD25− T responding cells (Tresp) and CD3− APCs were sorted from the spleens of untreated EAU mice on day 28 post immunization. Tresp cells were labeled with Far-Red dye and cultured with CD4+CD25hi Treg cells from PBS-treated or αCD4+IRBP treated mice at 1:5 Treg/Tresp ratio with IRBP or αCD3 stimulation for 4 days. (b) In vitro proliferation of Far Red-labeled Tresp cells and (c) relative suppression of Tresp cell proliferation by IRBP-specific Treg cells were assessed by FCM. Data represent the means ± SEM. *p<0.05, Mann-Whitney U test.
Furthermore, we examined the antigen-specific protective effect of Treg cells from the tolerized mice (αCD4+IRBP) in an adoptive transfer system (Fig. 4a). By using administration of αCD4 Ab with retina-irrelevant self-antigen myelin oligodendrocyte glycoprotein (MOG)(αCD4+MOG) as an alternative control, we further verified the suppression of EAU by αCD4+IRBP therapy but not αCD4+MOG, even if they showed increased frequency of Foxp3+ Treg cells in the spleens (Fig. S7). Indeed, passive transfer of Treg cells obtained from EAU donor mice significantly delayed onset and reduced severity of EAU disease in recipient mice (Figs. 4b, 44c). Importantly, Treg cells from the tolerized mice (αCD4+IRBP) had similar suppressive effects on significant reduction of EAU disease in recipient mice compared to Treg cells from control groups (PBS, αCD4+MOG) (Figs. 4b, 44c). Consistent with reduction of ocular inflammation, we found that the number of eye-infiltrating inflammatory cells were significantly decreased in αCD4+IRBP induced Treg recipient mice compared to control groups (Fig. 4d). The frequency of IL-17A producing CD4+ T cells were substantially decreased in the eyes of αCD4+IRBP induced Treg recipient mice compared to control groups (Fig. 4e). Subsequently, Treg cells from the tolerized mice (αCD4+IRBP) significantly reduced IL-17A production by T effectors in response to IRBP stimulation compared to control groups (Fig. 4f). Taken together, these data provide clear evidence to support the hypothesis that in vivo generation of retinal antigen IRBP-specific Treg cells contributes to effective treatment with αCD4 and IRBP in EAU.
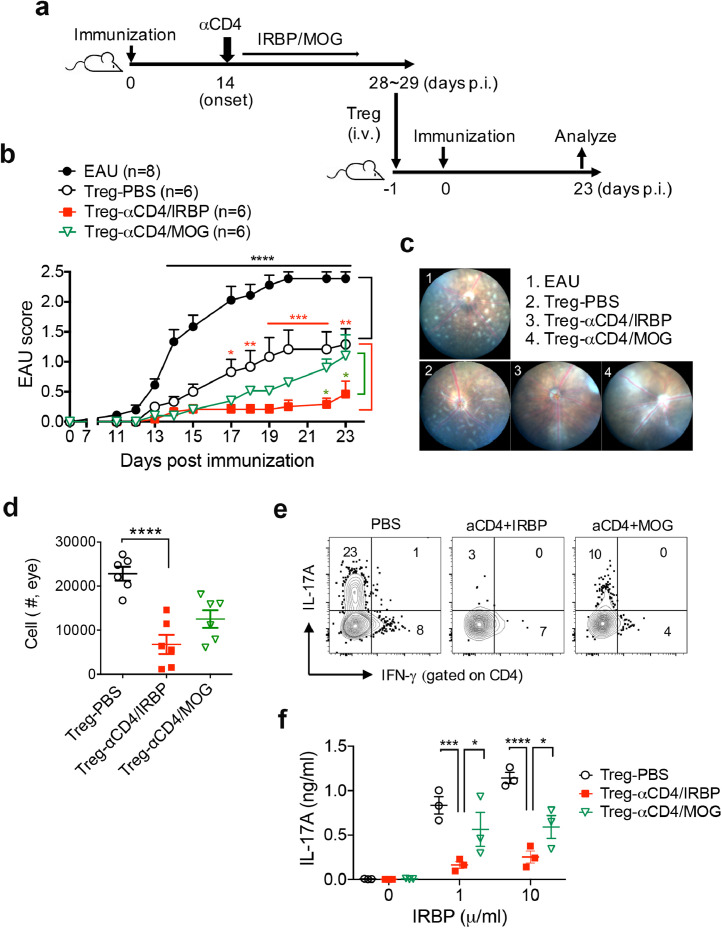
In vivo induction of IRBP-specific Treg cells by αCD4 and IRBP treatment is vital to ameliorate EAU. (a) Experimental scheme of an adoptive transfer system of Treg cells. Foxp3-GFP reporter donor mice were given an i.p injection of PBS or αCD4 Ab on day 14 post-immunization, followed by i.p. injection of IRBP or MOG every other day for 6 times. CD4+Foxp3+ Treg cells were isolated and sorted from lymphoid organs of donor mice on days 28 and 29 post-immunization, these cells (1.5 × 106 cells/mouse) were i.v. injected into naive recipient mice. One day after cell transfer, recipient mice were immunized for EAU induction, and their clinical severity were evaluated by retinal fluorescent imaging system. (b) Clinical scores of EAU in recipient mice untreated or pre-treated with Foxp3+ Treg cells from donor mice with the indicated treatment. *p<0.05, **p<0.01, ***p<0.001, ****p<0.0001, two-way ANOVA. (c) Representative retinal images assessed by fundus imaging system on day 23 post-immunization. (d) Absolute number of the eye-infiltrating inflammatory cells assessed by FCM on day 23 post-immunization. Data represent the means ± SEM. *p<0.05, one-way ANOVA. (e) Representative FCM plot of IFN-γ and IL-17A expression in the eye-infiltrating CD4+ T cells. (f) IRBP-specific IL-17A production by T cells in the dLNs of EAU recipient mice with the indicated treatment. Data represent the means ± SEM. *p<0.05, ***p<0.001, ****p<0.0001, two-way ANOVA.
3.4. Effective treatment with αCD4 Ab and IRBP is IL-10 and TGF-β dependent in EAU
To confirm the findings demonstrated in Fig. 2, we further examined whether TGF-β and IL-10 were required for effective treatment with αCD4 Ab and IRBP in EAU. We found that blockade of TGF-β using its neutralizing Ab abolished the therapeutic effects with αCD4+IRBP (Fig. 5a). Similarly, deficiency of IL-10 reversed the therapeutic effects of αCD4+IRBP treatment in EAU mice (Fig. 5d). Although blockade of TGF-β or deficiency of IL-10 resulted in loss of their suppressive activities on IRBP-driven IL-17 responses in αCD4+IRBP treated EAU mice (Figs. 5b, 55e), elimination of TGF-β but not IL-10 led to reduction of Foxp3+ Treg cells in the draining lymph nodes (dLNs) of αCD4+IRBP treated mice, indicating TGF-β but not IL-10 as the key factor for induction of antigen-specific Treg cells in vivo (Figs. 5c, 55f). On the other hand, IL-10 appeared to be involved in the suppressive function of IRBP-specific Treg cells induced by αCD4+IRBP treatment (Fig. 5e). Thus, we conclude that TGF-β is required for in vivo induction of antigen-specific Treg cells whereas IL-10 participates in suppressing uveitogenic Th17 immune responses for αCD4 and IRBP therapy in EAU.
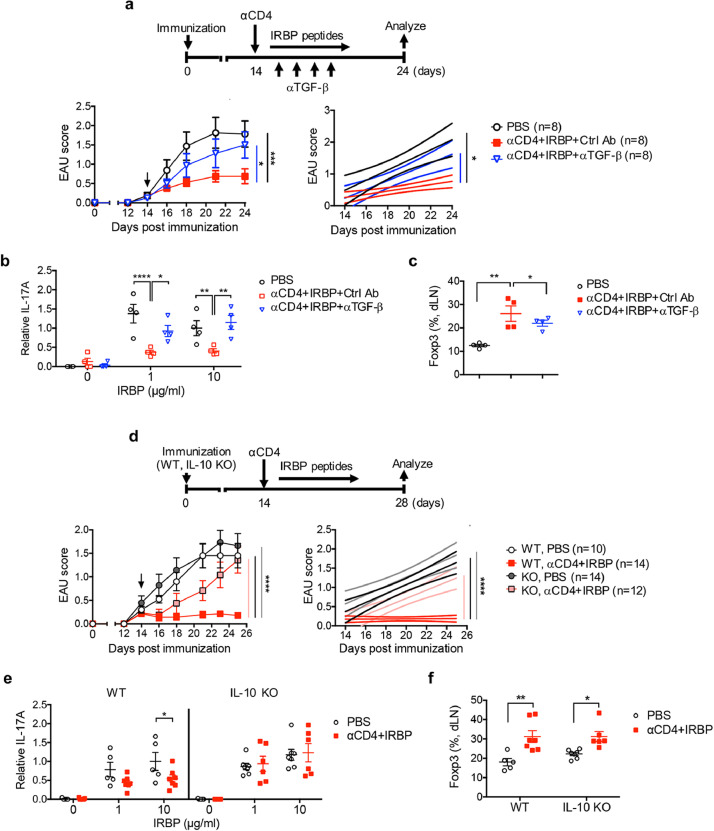
Effective treatment with αCD4 and IRBP is IL-10 and TGF-β dependent. (a-c) Effects of TGF-β in αCD4+IRBP treatment. EAU mice with PBS or αCD4+IRBP treatment received αTGF-β neutralizing Ab or isotype control on alternative day for 4 times, starting from day 14 post immunization. (a) Experimental scheme and clinical scores of EAU in mice with the indicated treatment. *p<0.05, ***p<0.001, two-way ANOVA (left); linear regression curve including the 95% confidence band of the regression line. *p<0.05 (right). (b) Relative antigen-specific IL-17A production by T cells on day 28 post immunization. Cells from dLNs of EAU mice were stimulated with IRBP for 48 hr. Cytokines were measured from cultured supernatants by ELISA. Data represent the means ± SEM. *p<0.05, **p<0.01, ****p<0.0001, two-way ANOVA. (c) Frequency of Foxp3+ Treg cells in the dLNs of EAU mice on day 28 post immunization. Data represent the means ± SEM. **p<0.01, one-way ANOVA. (d-f) Effects of IL-10 in αCD4+IRBP treatment. WT and IL-10 KO mice were immunized for EAU and received αCD4+IRBP treatment. (d) Experimental scheme and EAU scores in mice with the indicated treatment. ****p<0.0001, two-way ANOVA (left); linear regression curve including the 95% confidence band of the regression line. ****p<0.0001 (right). (e) Relative antigen-specific IL-17A production by T cells on day 28 post immunization. Cells from dLNs of EAU mice were stimulated with IRBP for 48 hr. Cytokines were measured from cultured supernatants by ELISA. Data represent the means ± SEM. *p<0.05, one-way ANOVA. (f) Frequency of Foxp3+ Treg cells in the dLNs on day 28 post-immunization. Data represent the means ± SEM. *p<0.05, **p<0.01, two-way ANOVA.
3.5. Administration of αCD4 Ab with retinal autoantigen, arrestin/S-antigen suppress IRBP-mediated EAU
As identification of autoantigens remains an unsolved problem in treating autoimmune diseases by Treg cell therapy, we next examined whether administration of αCD4 Ab with another autoantigen, retinal arrestin (hereafter S-antigen), could induce eye-specific tolerance in treating EAU induced by immunization with IRBP. Strikingly, we found that T cell apoptosis-antigen therapy by either “αCD4 with S-antigen” or “αCD4 with IRBP” showed similar therapeutic effects on significant reduction of ocular inflammation in EAU mice with established disease, as manifested by a reduction in vasculitis/retinitis and perivascular cellular exudates (Figs. 6a, 66b). ERG determined significant increased amplitudes in the eyes of EAU mice treated with either αCD4+IRBP or αCD4+S-antigen compared to untreated mice 60 days after EAU induction and indicated the improvement of visual function (Fig. 6c). Further, the frequency of Foxp3+ Treg cells was significantly increased in the spleens of EAU mice treated with either αCD4+IRBP or αCD4+S-antigen compared to untreated mice. Whereas administration of αCD4 Ab with retina-irrelevant self-antigen MOG failed to suppress EAU, even if they had increased frequency of Foxp3+ Treg cells (Fig. 6d, Fig. S7). Subsequently, the tolerized mice treated with αCD4+IRBP or αCD4+S-antigen significantly reduced IL-17A production by splenic T cells in response to IRBP stimulation compared to untreated mice, without altering over all αCD3/αCD28-induced IL-17A production (Fig. 6e). These data thus indicate that, not limited to EAU-relevant antigen IRBP only, retinal arrestin/S-antigen can induce eye-specific tolerance to inhibit uveitogenic Th17 immune response and suppress IRBP-mediated EAU in an antigen-nonspecific bystander manner.
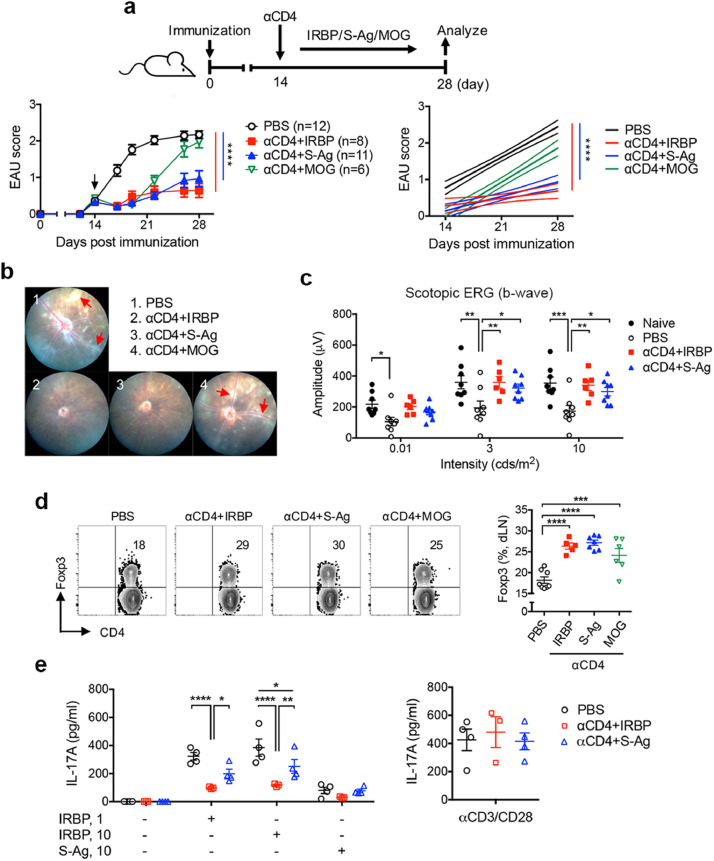
Treatment with αCD4 and retinal antigen “arrestin/S-antigen” emolliates EAU induced by IRBP. Mice were immunized with IRBP for EAU induction and treated with i.p injection of PBS or αCD4 Ab on day 14 post immunization followed by administration of IRBP, S-antigen or MOG on alternative day for 6 times. (a) Experimental scheme and clinical score of EAU in mice with the indicated treatments. Arrowhead indicates the initiation of treatment. ****p<0.0001, two-way ANOVA (left); linear regression curve including the 95% confidence band of the regression line. ****p<0.0001 (right). (b) Representative retinal images of EAU mice with the indicated treatments as assessed by fundoscopy. Red arrows indicate vasculitis and perivascular cellular exudates. (c) Changes in retinal function accessed by ERG. Amplitudes of scotopic ERG were recorded and analyzed in mice on day 60 post immunization. Data represent the means ± SEM. *p<0.05, **p<0.01, ***p<0.001, two-way ANOVA. (d) Representative FCM plot and frequency of Foxp3+ Treg cells in the dLNs of EAU mice with the indicated treatments. Data represent the means ± SEM of a representative of 3 individual experiments. ***p<0.001, ****p<0.0001, two-way ANOVA. (e) IRBP-specific IL-17A production in the spleens of EAU mice. Cells were collected on day 28 post immunization and stimulated with IRBP or αCD3/αCD28 for 48 hr. Cytokines were measured from the cultured supernatants by ELISA. Data represent the means ± SEM. *p<0.05, **p<0.01, ****p<0.0001, two-way ANOVA.
3.6. In vivo induction of autoantigen-specific Treg cells does not compromise the host anti-tumor T cell immunity
As Treg cells are regarded as potent immunoregulatory cells in suppressing anti-tumor immunity[40,41]. Firstly, we asked whether induction of Ab-mediated tolerance could suppress host anti-tumor immunity and affect tumor growth. In a mouse model of melanoma, we demonstrated that, by having tumor induced and grown in naïve mice prior to αCD4 Ab treatment, injection of low dose αCD4 Ab had no difference in the mouse survival rate, tumor growth and size between Ab-treated and untreated groups (Fig. S8). After confirming the effect of αCD4 Ab treatment in host anti-tumor immune response, we then evaluated whether the αCD4+IRBP therapy mediated eye-specific tolerance through generation of antigen-specific Treg cells could compromise host anti-tumor immune response. We designed an experiment approach by injecting melanoma cells into the EAU-suppressed tolerized mice (αCD4+IRBP) as well as other control groups (PBS, αCD4+OVA) (Fig. 7a). Indeed, we found that αCD4+IRBP therapy did not affect the survival rate, tumor growth and size in the EAU-suppressed tolerized mice when compared to control groups, and showed no significant difference in the number of tumor-infiltrating CD4+ and CD8+ T cells and their frequency of IFN-γ production (Figs. 7b-b-77e). These data indicate that in vivo induction of antigen-specific Treg cells to the eyes suppresses EAU but does not compromise the host overall T cell immunity against tumor development.
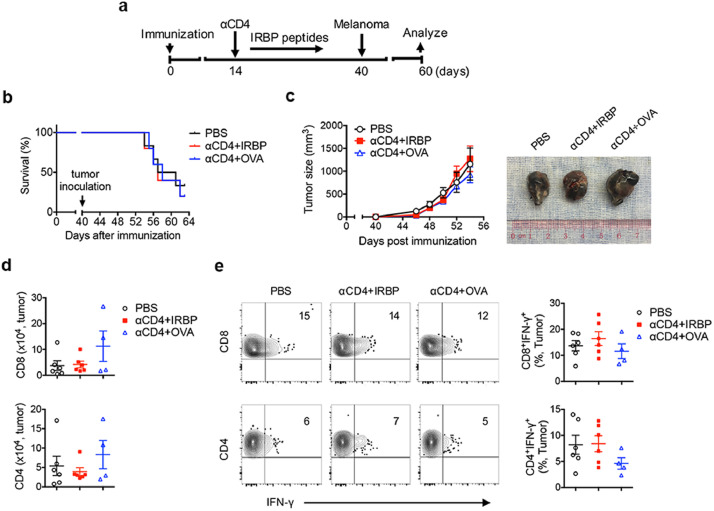
In vivo induction of antigen-specific Treg cells in suppressing EAU does not compromise the host immune responses against tumor development. (a) Mice were immunization for EAU induction and received treatment with PBS, αCD4+IRBP, αCD4+OVA on day 14 post immunization. Melanoma cells (Β16) were s.c. inoculated on day 40 post immunization. Data represent (b) the survival rate of EAU mice with tumor, and (c) the size and images of their developed tumor. (d) Absolute number of the tumor-infiltrating CD4+ and CD8+ T cells in EAU mice with indicated treatment, as assessed by FCM. Data present the means ± SEM of a representative from 3 individual experiments. (e) Representative FCM plot and frequency of IFN-γ production in the tumor-infiltrating CD4+ and CD8+ T cells. Data present the means ± SEM of a representative from 3 individual experiments.
4. Discussion
The ideal goal of Treg cell-based therapy for autoimmune diseases is the restoration of peripheral tolerance by generating antigen-specific Treg cells in the dysregulated immune system to suppress inflammation specific to the targeted organs, without compromising the host overall T cell immunity. However, unknown antigen specificity for human autoimmune diseases has largely limited their clinical application. In the current study, using a mouse model of EAU immunized with retinal antigen IRBP, we developed a new approach to revise the T cell apoptosis-antigen immunotherapy [27,28] in several ways: (i) induction of antigen-specific Treg cells in vivo by deleting uveitogenic T effectors using αCD4 Ab followed by administration of retinal autoantigens was highly effective in treating EAU, leading to suppression of ocular inflammation and rescue of visual function in mice that have already developed active disease; (ii) this therapeutic effect was mediated by induction of IRBP-specific Treg cells that inhibited uveitogenic Th17 effector response in TGF-β and IL-10 dependent fashion; (iii) importantly, once the antigen-specific Treg cells were generated by administration of one or few autoantigens, they can exert their anti-inflammatory functions in an antigen-nonspecific bystander manner, without needs to identify all autoantigens in the eyes; (iv) and finally, the host dysregulated immune system was reprogrammed to control the disease progression of EAU, without compromising the host T cell immunity against tumor in a melanoma model. A summary diagram depicting the immunotherapy is shown in Fig. 8.
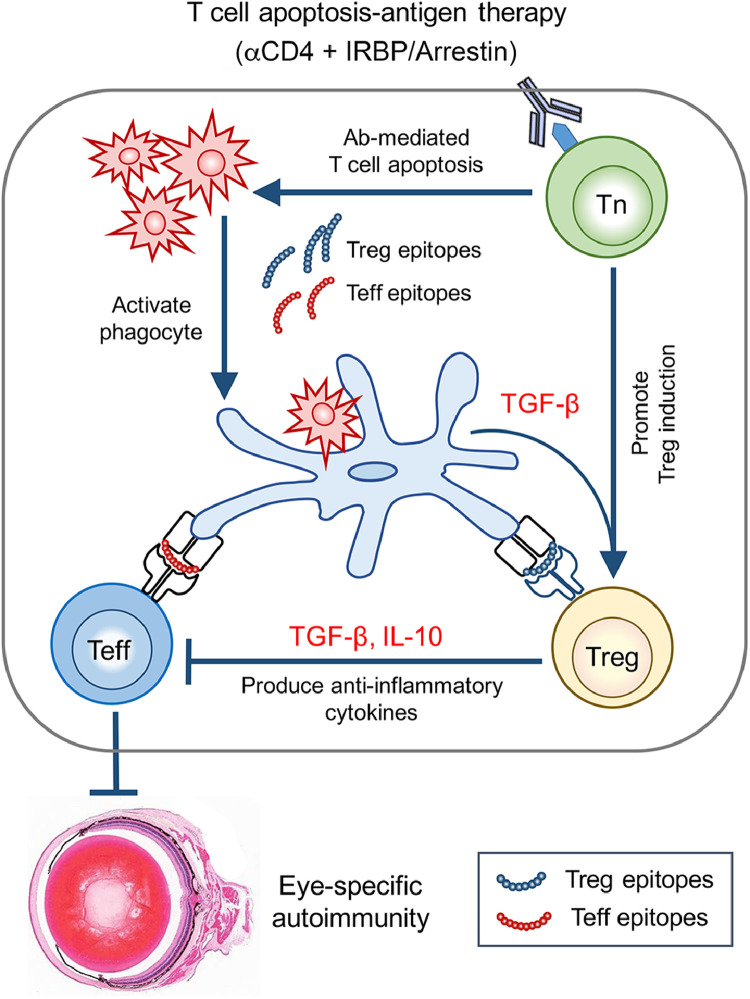
Schematic diagram of the mechanisms of in vivo induction of antigen-specific Treg cells by administration of αCD4 Ab with retinal autoantigens in treating autoimmune eye diseases.
Previous studies by our group and others have shown that induction of retinal antigen IRBP-specific Treg cells brings about resolution and maintains remission of EAU, which involves an interaction with APCs in peripheral lymphoid organ spleen [25,[42], [43], [44]]. However, limited to the instability of Treg cells, local delivery of retinal antigen-specific Treg cells to the eyes could not achieve suppression of EAU in inflammatory ocular environment [24]. In the present study, we showed that the T cell apoptosis-antigen therapy using administration of αCD4 Ab combined with retinal autoantigen IRBP peptides effectively attenuated EAU, leading to prolonged remission of ocular inflammation and rescue of visual function in mice with active disease. This therapeutic effect is likely caused by T cell apoptosis mediated in vivo generation of IRBP-specific Treg cells to inhibit uveitogenic Th17 and Th1 immune responses, in addition to transiently depletion of pathogenic T cells.
Since depletion of pathogenic CD4+ T cells might lead to suppression of CNS autoimmune diseases [45], it is important to distinguish whether the suppressive effect of αCD4+IRBP therapy seen in EAU was caused by decreased pathogenic CD4+ effector T cells or by increased CD4+Foxp3+ Treg cells. In the current study, we demonstrated that as control, administration of “αCD4 with nonself-antigen OVA” or “αCD4 with eye-irrelevant self-antigen MOG” eliminated pathogenic CD4+ T effectors and thus resulted in transient EAU suppression, while their disease restored shortly after CD4+ T cell recovery. Most importantly, these control groups showed similar severity of EAU disease compared to untreated (PBS) group and their T cells produced similar level of IRBP-specific IL-17A, indicating that temporary depletion of CD4+ T cells by Ab treatment itself does not affect their pathogenic function in the progress of EAU disease. The findings thus support the notion that the EAU suppression by αCD4+IRBP therapy is caused by induction of CD4+Foxp3+ Treg cells, but not by decrease of CD4+ T effectors. We further confirmed the increase of antigen-specific Treg cells induced by αCD4+IRBP therapy with assessment of T cell suppression assay. Moreover, using an adoptive transfer system of CD4+Foxp3+ Treg cells, we provided more evidence to explain the specificity of αCD4+IRBP therapy in treating EAU.
Mechanically, we identified that effective treatment with αCD4 Ab and IRBP was TGF-β and IL-10 dependent, demonstrating that Αb-mediated CD4+ cell apoptosis increased production of TGF-β and IL-10 by phagocytic APCs and promoted Treg cell induction, while either blockade of TGF-β or deficiency of IL-10 abolished their therapeutic effects in EAU mice. Our findings support the notion that TGF-β is vital to mediating immune tolerance and suppression of autoimmune disease by T cell apoptosis-antigen therapy through in vivo induction of antigen-specific Treg cells [7,8]. On the other hand, we revealed that IL-10 plays a key role in the suppressive function of Treg cells. Therefore, we conclude that TGF-β is required for in vivo induction of antigen-specific Treg cells and IL-10 participates in suppressing uveitogenic Th17 effector response for αCD4 with autoantigen therapy in EAU model.
Another our major finding is that we have defined the Ab-mediated immune tolerance could be successfully achieved in IRBP-mediated EAU mice by administration of retinal autoantigens, arrestin/S-antigen but not IRBP only, in a bystander manner. The specific antigens that serve as molecular targets for autoimmune uveitis remain unclear [24]. However, given that selective tolerance can be achieved in animals in which treatment with one retinal protein yields suppression of EAU induced by immunization with the same molecule [46,47], it was surprising to find that T cell apoptosis-antigen therapy using one or few retinal autoantigens can induce eye-specific tolerance to exert anti-inflammatory functions in a more general manner. The results are further supported by the finding of ineffective treatment with αCD4 and MOG. Thus, it appears that EAU-relevant Treg cells do not have to be IRBP-specific, Treg cells induced to one autoantigen may suppress inflammatory responses to the other autoantigens in inflammatory tissue environment. Our findings support previous studies, suggesting the dominant tissue-specific autoantigens can alter over time through the progression of disease by epitope spreading that activate Treg cells specific to the other autoantigens and suppress effector T cell response [48,49]. We therefore propose to extend T cell apoptosis-antigen therapy as a potential approach in treating CNS autoimmunity, regardless needs to knowing the dominant autoantigens. Additional studies are required to study mechanisms of the autoantigen-mediated bystander suppression.
It is of note that treatment with αCD4 Ab is not only limited to the depletion of T cells but also other types of immune cells that act as APCs to modulate T effector responses or promote Treg cell induction. Induction of immune tolerance by Ab-mediated depletion of CD4+ T cells to suppress CNS autoimmunity has been previously reported for decades in EAE model and MS patients, which had no effect on CD8+ and B lymphocytes or other leukocytes [50,51]. In parallel with the previous studies, we evaluated the safety profiles of this approach in EAU model, illustrating that a single injection of low dose αCD4 Ab is sufficient enough to promote phagocytic APCs to increase Treg induction, without altering the overall T effectors responses and the proportion of other types of immune cells including CD8+, CD4+CD11b+ and Gr1+ subsets. Retinal microglia cells have been previously reported for their potential contribution to the EAU progress and suppression [52,53]. Additional experiments need to be further investigated the regulatory effect of αCD4 Ab therapy on microglia cells in suppression of EAU.
As Treg cells are regarded as potent immunoregulatory cells in suppressing anti-tumor immunity [40,41]. It is concerned that Ab-mediated immune tolerance would suppress aberrant immune response against self-antigens as well as host anti-tumor immune response. We revealed that αCD4+IRBP therapy could reprogram the host dysregulated immune system by in vivo induction of retinal autoantigen-specific Treg cells to control the disease progression of EAU specifically to the targeted organs, without altering the host over all T cell response. Moreover, using a melanoma model, we revealed that EAU-suppressed mice treated with αCD4+IRBP therapy had no effects on tumor growth and the host anti-tumor T cell response compared to other control groups. The findings are further supported by evidence that αCD4 Ab treatment does not affect melanoma growth by having tumor presented and grown in naïve mice, prior to Ab injection. Thus, these data conclude that in vivo induction of IRBP-specific Treg cells by αCD4+IRBP administration specifically suppresses eye-specific autoimmune disease would not exhibit any compromised anti-tumor T immunity.
In this study we focus on the restoration of peripheral tolerance using Treg cell-specific therapy for autoimmune eye disease. However, the questions remain unsolved are, by applying a retinal antigen, how the therapy exerts their site-directed effect to allow the antigen-specific Treg cells migrating into the retinal tissues as opposed to the remaining in the peripheral immune system. Our previous studies have documented that the IRBP-specific Treg cells have a higher preference to migrate into the target eyes compared to draining lymphoid nodes during the course of EAU [25]. Here we showed a correlation between reduced severity of EAU disease and increased frequency of Foxp3+ Treg cells in the eyes of mice with αCD4+IRBP therapy. Whereas toward the resolution of EAU disease, Treg cells remained at a high level in the eyes of EAU tolerized mice, despite Tregs progressively declined in their spleens. Our findings are supported by the previous reports [25,42,43], indicating that retinal antigen IRBP-specific Treg cells can be generated in periphery lymphoid organs by αCD4+IRBP therapy, but they prefer migrating and remaining within the eyes possibly due to the retinal antigen IRBP expression. Additional studies need to be further investigated.
There are several limitations remained in this experimental approach: (i) the safety profile of the Ab-mediated depletion of CD4+ T cells remains further identified, although CD4+ T cell depletion has been reported to be well tolerated in patients with autoimmunity in several clinical trials; (ii) the antigen specificity of Treg cells remains further characterized to exclude possible protection caused by the depletion of pathogenic CD4+ T cells; (iii) the quantity and timing of peptides administration are required to be optimized prior to their application in clinical setting; and (iv) the long-term consequences of the therapeutic approach remain unknown and require future investigation.
In conclusion, we have addressed a specific approach to induce eye-specific tolerance for treating EAU and provide potential improvements of the system, including the usage of αCD4 Ab to target pathogenic T effectors and administration of multiple self-antigens to enhance specificity and efficacy, which may be vital for transferring this approach to clinical studies in uveitis patients. The T cell apoptosis-antigen therapy established by inducing CD4+ T cell apoptosis with Ab followed by administration of retinal autoantigens (e.g., IRBP or arrestin) redirected the dysregulated host immune system present in settings of inflammatory eye disease toward an immune-tolerant state to disease-causing autoantigens. The therapeutic effect was mediated by in vivo induction of antigen-specific Treg cells and required TGF-β. IL-10 is involved in the Treg cell mediated suppression of inflammatory responses. Importantly, we demonstrated that T cell apoptosis-antigen therapy with one or few autoantigens would be sufficient to suppress autoimmune eye disease in a more global manner. Further, this approach specifically targets the uveitogenic Th17 immune response, without compromising the host general immunity, e.g. T cell immunity against tumor. Our findings could illustrate the clinical potential for patients with autoimmune uveitis.
Contributors
Z. Chen, T. Zhang, H.T. Kam, D. Qiao and Y. Zhong performed experiments and data analysis; W. Jin, M. Zhou and H. Zhou provided technical assistance; W.P. Chong provided scientific input and designed experiments; J. Chen and W. Chen designed and supervised the study, wrote and final proofed the manuscript. Z. Chen and J. Chen verified the underlying data. All authors read and approved the final version of the manuscript.
DAta sharing statement
All data are available in this manuscript and supplementary files.
Declaration of Competing Interest
Patents (ZL201711202986.0 and ZL201711202988.X) for the reported data are granted in China. The authors have declared that no conflict of interest exists.
Acknowledgements
This study was granted by the Natural Science Foundation of Guangdong Province (2017A030313836, 2019A1515010956) and the Fundamental Research Fund of the State Key Laboratory of Ophthalmology, Zhongshan Ophthalmic Center. W. Jin and W. Chen are supported by the Intramural Research Program of NIDCR, NIH.
Footnotes
Supplementary material associated with this article can be found in the online version at 10.1016/j.ebiom.2021.103496.
REFERENCES
Articles from eBioMedicine are provided here courtesy of Elsevier
Full text links
Read article at publisher's site: https://doi.org/10.1016/j.ebiom.2021.103496
Read article for free, from open access legal sources, via Unpaywall:
http://www.thelancet.com/article/S2352396421002899/pdf
Citations & impact
Impact metrics
Citations of article over time
Alternative metrics

Discover the attention surrounding your research
https://www.altmetric.com/details/110001348
Article citations
Immunostimulatory effects of Toll-like receptor ligands as adjuvants in establishing a novel mouse model for pemphigus vulgaris.
Clin Transl Med, 14(7):e1765, 01 Jul 2024
Cited by: 1 article | PMID: 39031979 | PMCID: PMC11259602
Beneficial mechanisms of dimethyl fumarate in autoimmune uveitis: insights from single-cell RNA sequencing.
J Neuroinflammation, 21(1):112, 29 Apr 2024
Cited by: 0 articles | PMID: 38684986 | PMCID: PMC11059727
RBPJ Knockdown Promotes M2 Macrophage Polarization Through Mitochondrial ROS-mediated Notch1-Jagged1-Hes1 Signaling Pathway in Uveitis.
Inflammation, 18 May 2024
Cited by: 0 articles | PMID: 38761249
Role of FoxP3<sup>+</sup> Regulatory T Cells in Modulating Immune Responses to Adeno-Associated Virus Gene Therapy.
Hum Gene Ther, 35(13-14):439-450, 11 Apr 2024
Cited by: 2 articles | PMID: 38450566
Review
Inflammatory cytokines as mediators of retinal endothelial barrier dysfunction in non-infectious uveitis.
Clin Transl Immunology, 12(12):e1479, 12 Dec 2023
Cited by: 5 articles | PMID: 38090668 | PMCID: PMC10714664
Review Free full text in Europe PMC
Go to all (6) article citations
Data
Data behind the article
This data has been text mined from the article, or deposited into data resources.
BioStudies: supplemental material and supporting data
Similar Articles
To arrive at the top five similar articles we use a word-weighted algorithm to compare words from the Title and Abstract of each citation.
Blockade of interleukin-6 signaling suppresses not only th17 but also interphotoreceptor retinoid binding protein-specific Th1 by promoting regulatory T cells in experimental autoimmune uveoretinitis.
Invest Ophthalmol Vis Sci, 52(6):3264-3271, 17 May 2011
Cited by: 46 articles | PMID: 21330657
Ex-vivo tolerogenic F4/80⁺ antigen-presenting cells (APC) induce efferent CD8⁺ regulatory T cell-dependent suppression of experimental autoimmune uveitis.
Clin Exp Immunol, 176(1):37-48, 01 Apr 2014
Cited by: 16 articles | PMID: 24266626 | PMCID: PMC3958152
Interphotoreceptor retinoid binding protein is a potent tolerogen in Lewis rat: suppression of experimental autoimmune uveoretinitis is retinal antigen specific.
Br J Ophthalmol, 81(1):61-67, 01 Jan 1997
Cited by: 6 articles | PMID: 9135411 | PMCID: PMC1722010
Autoimmunity in the immune privileged eye: pathogenic and regulatory T cells.
Immunol Res, 42(1-3):41-50, 01 Jan 2008
Cited by: 58 articles | PMID: 18629448 | PMCID: PMC2756228
Review Free full text in Europe PMC