Abstract
Background
Highly efficacious vaccines against severe acute respiratory syndrome coronavirus 2 (SARS-CoV-2) have been developed. However, the emergence of viral variants that are more infectious than the earlier SARS-CoV-2 strains is concerning. Several of these viral variants have the potential to partially escape neutralizing antibody responses, warranting continued immune-monitoring.Methods
We used a panel of 30 post-mRNA vaccination sera to determine neutralization and RBD and spike binding activity against a number of emerging viral variants. The virus neutralization was determined using authentic SARS-CoV-2 clinical isolates in an assay format that mimics physiological conditions.Findings
We tested seven currently circulating viral variants of concern/interest, including the three Iota sublineages, Alpha (E484K), Beta, Delta and Lambda in neutralization assays. We found only small decreases in neutralization against Iota and Delta. The reduction was stronger against a sub-variant of Lambda, followed by Beta and Alpha (E484K). Lambda is currently circulating in parts of Latin America and was detected in Germany, the US and Israel. Of note, reduction in a receptor binding domain and spike binding assay that also included Gamma, Kappa and A.23.1 was negligible.Interpretation
Taken together, these findings suggest that mRNA SARS-CoV-2 vaccines may remain effective against these viral variants of concern/interest and that spike binding antibody tests likely retain specificity in the face of evolving SARS-CoV-2 diversity.Funding
This work is part of the PARIS/SPARTA studies funded by the NIAID Collaborative Influenza Vaccine Innovation Centers (CIVIC) contract 75N93019C00051. In addition, this work was also partially funded by the Centers of Excellence for Influenza Research and Surveillance (CEIRS, contract # HHSN272201400008C), the JPB Foundation, the Open Philanthropy Project (research grant 2020-215611 (5384), by anonymous donors and by the Serological Sciences Network (SeroNet) in part with Federal funds from the National Cancer Institute, National Institutes of Health, under Contract No. 75N91019D00024, Task Order No. 75N91020F00003.Free full text

Evidence for retained spike-binding and neutralizing activity against emerging SARS-CoV-2 variants in serum of COVID-19 mRNA vaccine recipients
Abstract
Background
Highly efficacious vaccines against severe acute respiratory syndrome coronavirus 2 (SARS-CoV-2) have been developed. However, the emergence of viral variants that are more infectious than the earlier SARS-CoV-2 strains is concerning. Several of these viral variants have the potential to partially escape neutralizing antibody responses, warranting continued immune-monitoring.
Methods
We used a panel of 30 post-mRNA vaccination sera to determine neutralization and RBD and spike binding activity against a number of emerging viral variants. The virus neutralization was determined using authentic SARS-CoV-2 clinical isolates in an assay format that mimics physiological conditions.
Findings
We tested seven currently circulating viral variants of concern/interest, including the three Iota sublineages, Alpha (E484K), Beta, Delta and Lambda in neutralization assays. We found only small decreases in neutralization against Iota and Delta. The reduction was stronger against a sub-variant of Lambda, followed by Beta and Alpha (E484K). Lambda is currently circulating in parts of Latin America and was detected in Germany, the US and Israel. Of note, reduction in a receptor binding domain and spike binding assay that also included Gamma, Kappa and A.23.1 was negligible.
Interpretation
Taken together, these findings suggest that mRNA SARS-CoV-2 vaccines may remain effective against these viral variants of concern/interest and that spike binding antibody tests likely retain specificity in the face of evolving SARS-CoV-2 diversity.
Funding
This work is part of the PARIS/SPARTA studies funded by the NIAID Collaborative Influenza Vaccine Innovation Centers (CIVIC) contract 75N93019C00051. In addition, this work was also partially funded by the Centers of Excellence for Influenza Research and Surveillance (CEIRS, contract # HHSN272201400008C), the JPB Foundation, the Open Philanthropy Project (research grant 2020-215611 (5384), by anonymous donors and by the Serological Sciences Network (SeroNet) in part with Federal funds from the National Cancer Institute, National Institutes of Health, under Contract No. 75N91019D00024, Task Order No. 75N91020F00003.
1. Introduction
Severe acute respiratory syndrome coronavirus 2 (SARS-CoV-2) emerged in late 2019 in China and has since then caused the coronavirus disease 2019 (COVID-19) pandemic. Vaccines were developed and distributed in record speed with emergency use authorizations as early as December 2020 (in the US) [1]. Coronaviruses are an exception among RNA viruses because their replication machinery possesses proofreading activity [2]. It was, therefore, expected that they would evolve slower than other RNA viruses. The emergence of variant viruses, first in Europe in the summer of 2020 [3], [4], [5] - including in minks in Denmark - and then in late 2020 in the UK [6], South Africa [7] and Brazil [8] was a surprising event. Since the detection of these first variants, several other Variants of Interest (VoIs) and Variants of Concern (VoCs) have spread locally as well as globally [9]. These include Iota (B.1.526) which emerged in New York City and comprises three different sub-lineages [10,11], Epsilon (B.1.427/B.1.429) which emerged in California [12], Lambda (C.37) which emerged in Peru (preprint [13]), Delta (B.1.617.2) and Kappa (B.1.617.1) which emerged in India [14], B.1.1.7 carrying E484K (Alpha+484K), which was detected in several countries, as well as A.23.1 which was first detected in Uganda [15]. Several of these lineages are in decline, including the Iota lineages and Epsilon while others such as Delta are increasing in prevalence. Many of these variants display extensive changes in the N-terminal domain (NTD) and/or the receptor binding domain (RBD) of spike, both of which harbor neutralizing epitopes. In addition, several of these variants also contain additional mutations in their spike gene that may enhance affinity to human angiotensin converting enzyme 2 (ACE2)(16). These changes may impact negatively on binding and neutralization by vaccine-induced antibodies, which are discussed as mechanistic correlates of protection [17,18]. It is, therefore, important to monitor neutralizing and binding activity of sera from vaccinees against these emerging variants.
Here, we tested the neutralizing activity of a panel of 30 post-mRNA SARS-CoV-2 vaccination sera against the seven emerging variants (three Iota sub-lineages, Alpha-E484K, Beta, Delta and a Lambda sub-variant) using a microneutralization assay designed to allow for multi-cycle replication with sera/antibodies being present in the overlay at all times to better mimic physiological conditions [19]. In addition, we performed binding assays against recombinant RBD and full-length spike proteins of several VoIs and VoCs.
2. Methods
2.1. Ethics
Post-vaccine sera were collected from participants in the longitudinal observational PARIS (Protection Associated with Rapid Immunity to SARS-CoV-2) study. The study was reviewed and approved by the Mount Sinai Hospital Institutional Review Board (IRB-20-03374). All participants signed written consent forms prior to sample and data collection. All participants provided permission for sample banking and sharing.
2.2. The SAVE initiative post-vaccine sera reference sample panel
A panel of 30 post-vaccination sera (Supplementary Table 3) was generated for use and distribution by BEI Resources for the NIAID SARS-CoV-2 Assessment of Viral Evolution (SAVE) “in vitro” group which is part of the US Department of Health and Human Services (HHS) SARS-CoV-2 Interagency Group (SIG), to determine the immune escape of different VoCs and VoIs [20]. This reference panel allows harmonization of the phenotypic characterizations of viral variants of concern/interest across different labs and neutralization assay platforms given that these functional assays are notoriously difficult to compare. It is not powered to distinguish between vaccine type specific immune responses or determine the effects of pre-existing SARS-CoV-2 immunity on vaccine induced antibody responses.
Briefly, 30 PARIS participants were invited to provide additional serum samples after receiving their second mRNA vaccine dose. All specimens were coded prior to processing, banking and sharing with BEI Resources. 15 participants had received two doses of the mRNA-1273 (Moderna) and 15 participants had received two doses of the BNT162b2 (Pfizer/BioNTech). The majority of participants were naïve prior to vaccination with four or five COVID-19 survivors being included in each vaccine group (see Supplemental Table 3). Of note, serum samples from 11/30 participants were collected at least 14 days after the second vaccine dose (“full immunization”).
2.3. Cells
Vero.E6 cells were cultured in complete Dulbecco's Modified Eagles Medium (DMEM; Gibco, Ref. 11995065) containing 10% fetal bovine serum (FBS; Gibco, Ref. 10082-147) supplemented with 100U/ml penicillin, 100
μg/ml streptomycin (Gibco, Ref. 15140122). Vero.E6 cells, originally purchased from ATCC, were not independently validated in our laboratories but we routinely perform mycoplasma testing.
2.4. Replication competent SARS-CoV-2 isolates
Nasopharyngeal swab specimens were collected as part of the routine SARS-CoV-2 surveillance conducted by the Mount Sinai Pathogen Surveillance program (IRB approved, HS#13-00981). Specimens were selected for viral culture on Vero-E6 cells based on the complete viral genome sequence information [21]. The SARS-CoV-2 virus USA-WA1/2020 was obtained from BEI resources (NR‐52281) and used as wild-type reference. Viruses were grown in Vero.E6 cells for 4-6 days; the supernatant was clarified by centrifugation at 4,000 g for 5 min and aliquots were frozen at -80°C for long term use. Expanded viral stocks were sequence-verified and tittered on Vero.E6 cells prior to use in microneutralization assays. Supplemental Table 2 summarizes the amino acid substitutions and deletions in the spike region of each viral isolate.
2.5. Phylogeny of SARS-CoV-2 lineages
The phylogenetic relationships of the SARS-CoV-2 isolates from the Pathogen Surveillance Program of the Mount Sinai Health System (PSP-MSHS) are depicted in a New York-focused background from GISAID (2021-06-02 download). The time-calibrated tree was built with Nextstrain v7 for SARS-CoV-2 with default parameters (https://github.com/nextstrain/ncov) [22]. The final tree contained a total of 10,605 background sequences in addition to the PSP-MSHS isolates. PANGO lineages were assigned with pangolin v3.1.7 with PANGO v1.2.36 [23,24] and are depicted for the VoIs and VoCs used in the neutralization assays.
2.6. ELISA
Antibody titers in sera from vaccines were determined by a research grade enzyme-linked immunosorbent assay (ELISA) using recombinant versions of the receptor binding domain (RBD) and the spike (S) of wild type SARS-CoV-2 and variant viruses (RBD: wild-type WA1, B.1.1.7, B.1.351, P.1; spike: wild-type WA1, B.1.1.7, B.1.351, P.1, B.1.617.1, A.23.1, see Supplemental Tables 1 and 2 for specific substitutions in each variant). All samples were analysed in a blinded manner. Briefly, 96-well microtiter plates (Corning, Ref. 353227) were coated with 50 μl/well of recombinant protein (2 μg/ml) overnight at 4°C. Plates were washed three times with phosphate-buffered saline (PBS; Gibco, Ref. 10010-031) supplemented with 0.1% Tween-20 (PBS-T; Fisher Scientific ref. 202666) using an automatic plate washer (BioTek 405TS microplate washer). For blocking, PBS-T containing 3% milk powder (American Bio, Ref. AB1010901000) was used. After 1 h incubation at room temperature (RT), blocking solution was removed and initial dilutions of heat-inactivated sera (in PBS-T 1%-milk powder) were added to the plates, followed by 2-fold serial dilutions. After 2 h incubation, plates were washed three times with PBS-T and 50 μl/well of the pre-diluted secondary antibody anti-human IgG (Fab-specific) horseradish peroxidase antibody (produced in goat; Sigma-Aldrich, Cat# A0293, RRID:AB_257875) diluted 1:3,000 in PBS-T containing 1% milk powder were added. After 1 h incubation at RT, plates were washed three times with PBS-T and SigmaFast o-phenylenediamine dihydrochloride (Sigmafast OPD; Sigma-Aldrich, Ref. P9187-50SET) was added (100 μl/well) for 10min, followed by addition of 50 μl/well of 3 M hydrochloric acid (Thermo Fisher, Ref. S25856) to stop the reaction. Optical density was measured at a wavelength of 490 nm using a plate reader (BioTek, SYNERGY H1 microplate reader). The area under the curve (AUC) values were calculated and plotted using Prism 9 software (GraphPad).
2.7. Live SARS-CoV-2 microneutralization assay
Sera from vaccinees were used to assess the neutralization of wild type SARS-CoV-2 and selected VoIs and VoCs (Supplementary Table 3). All procedures were performed in the Biosafety Level 3 (BSL-3) facility at the Icahn School of Medicine at Mount Sinai following standard safety guidelines. Remdesivir (Medkoo Bioscience inc., Ref. 329511) was used as a control for wild type and variant viruses to monitor assay variation. The day before infection, Vero E6 cells were seeded in 96-well high binding cell culture plates (Costar, Ref. 07620009) at a density of 20,000 cells/well in complete Dulbecco's Modified Eagle Medium (cDMEM). After heat inactivation of sera (56°C for 1 h), serum samples were serially diluted (3-fold) in minimum essential media starting at 1:10. For dilutions of sera, infection media consisting of minimum essential media (MEM; Gibco, Ref. 11430-030) supplemented with 2mM L-glutamine (Gibco, Ref. 25030081), 0.1% sodium bicarbonate (w/v, HyClone, Ref. SH30033.01), 10
mM 4-(2-hydroxyethyl)-1-piperazineethanesulfonic acid (HEPES; Gibco, Ref. 15630080), 100
U/ml penicillin, 100
μg/ml streptomycin (Gibco, Ref. 15140122) and 0.2% bovine serum albumin (MP Biomedicals, Cat#. 810063) was used. On the next day, sera dilutions were incubated with 1000 tissue culture infectious dose 50 (TCID50) of USA-WA1/2020 SARS-CoV-2 (BEI, NR‐52281) or the corresponding variant virus for one hour at RT, followed by transfer of 120μl of virus-sera mix to Vero-E6 plates. Infection was led to proceed for one hour at 37°C and inoculum was removed. 100 μl/well of the corresponding antibody dilutions plus 100μl/well of infection media supplemented with 2% fetal bovine serum (FBS; Gibco, Ref. 10082-147) were added to the cells. Plates were incubated for 48h at 37°C.
For staining of the nucleoprotein antigen, cells were fixed by addition of 150 μl/well of a 10% formaldehyde solution and incubated overnight at 4°C. Fixed cell monolayers were washed with PBS (Gibco, 10010-031) and permeabilized with 150μl/well of PBS, 0.1% Triton X-100 (Fisher Bioreagents, Ref. BP151-100) for 15
min at RT. Permeabilization solution was removed, plates were washed with 200
μl/well of PBS (Gibco, 10010-031) twice and 200 μl/well of blocking solution consisting of PBS 3% milk (American Bio, Ref. AB1010901000) were added for an
hour at RT. Blocking solution was removed and 100
μl/well of a PBS 1% milk (American Bio, Ref. AB1010901000) solution containing 1μg/ml of the mAb 1C7C7, a mouse anti-SARS nucleoprotein (NP) monoclonal antibody generated at the Center for Therapeutic Antibody Development at The Icahn School of Medicine at Mount Sinai ISMMS (Millipore Sigma, Cat# ZMS1075) were added for 1
h at RT. Primary antibody was removed and plates were washed with 200
μl/well of PBS twice, followed by addition of 100
μl/well of goat anti-mouse IgG-HRP (Rockland Immunochemicals, Ref. 610-1302, RRID:AB_219656) diluted in PBS 1% milk (American Bio, Ref. AB1010901000) 1:3000. After 1 h incubation, antibody solution was removed, plates were washed twice with PBS and 100
μl/well of o-phenylenediamine dihydrochloride (Sigmafast OPD; Sigma-Aldrich, Ref. P9187-50SET) were added for 10 min at RT. The reaction was stopped by addition of 50
μl/well of HCl 3M (Thermo Fisher, Ref. S25856). Optical density (OD) was measured (490
nm) with a microplate reader (BioTek, SYNERGY H1 microplate reader). Initial subtraction of background and calculation of the percentage of neutralization with respect to the “virus only” control was done in excel. A non-linear regression curve fit analysis, using Prism 9 software (GraphPad), was performed to calculate the inhibitory dilution 50% (ID50), using top and bottom constraints of 100% and 0%, respectively. All samples were analysed in a blinded manner.
2.8. Statistics
A mixed effect analysis with Dunnett's multiple comparisons test was used to compare the absolute EC50 neutralization values. A one-way ANOVA with Dunnett's multiple comparisons test was used to compare the binding antibody titers. Statistical analyses were performed using Prism 9 software (GraphPad).
2.9. Role of the funders
The funders had no role in study design, data collection, data analysis, data interpretation, or writing of this manuscript.
3. Results
3.1. Selection of SARS-CoV-2 isolates
Eight replication competent SARS-CoV-2 isolates were selected for testing in this study. In addition to the wild-type reference WA1 isolate, we choose seven clinical isolates derived from nasopharyngeal swabs obtained from patients diagnosed with COVID-19 at the Mount Sinai Health System in New York City. These specimen were deidentified and banked by the Mount Sinai Pathogen Surveillance Program (Fig. 1). These isolates represent the three sublineages found in the NYC variants of interest: Iota (no change in RBD), Iota+E484K, Iota+S477N. In addition, we included a viral isolate representing Alpha+E484K, Beta, Delta as well as a subvariant of Lambda in our experiments (Fig. 1 and Supplementary Table 3 for a summary of the substitutions in spike). The Lambda subvariant (PV29369) contained additional changes compared to the consensus Lambda sequence including a 13 amino acid deletion eliminating the NTD substitutions G75V and T76I and an additional E471Q substitution in the RBD. The large NTD deletion (T63-G75) has only been reported in LambdaC.37 with a frequency of approximately 1% (GISAID). Lambda subvariants, similar to PV29369, have been co-circulating at low frequency in, at least, eight different countries since its first detection in January 2021.
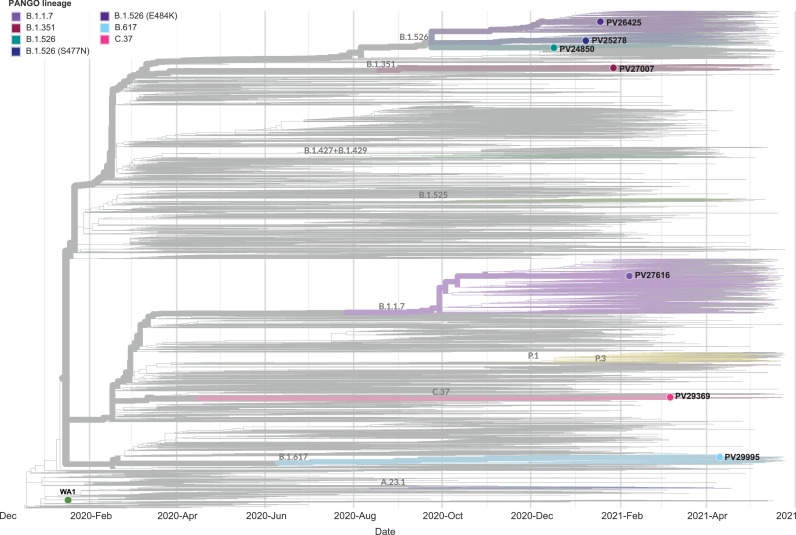
Phylogenetic relationships of the seven SARS-CoV-2 isolates selected. The time-calibrated tree contains a total of 10,605 background sequences in addition to the seven PSP-MSHS isolates. Viral variants of concern (VoC): Alpha, Beta and Gamma. Viral variants of interest (VoI): Iota, Lambda. Of note the Lambda isolate represents a subvariant with additional mutations relative to the main Lambda variant.
3.2. Description of the SAVE initiative post vaccine reference sample panel
A post-vaccination reference panel comprising sera from 30 distinct individuals vaccinated with mRNA-1273 (Moderna) or BNT162b2 (Pfizer/BioNTech) was generated in collaboration with the NIH SAVE initiative to allow for rapid harmonization of phenotypic characterization of viral variants of concern/interest across different labs and neutralization assay platforms. The participants were selected from the on-going longitudinal observational PARIS study based on their vaccination status: 15 had received two doses of the mRNA-1273 vaccine (Moderna, average time after 2nd vaccine dose: 5 days [range: 2–10], 33% COVID19 survivors) and 15 had received two doses of the BNT162b2 vaccine (Pfizer, average time after 2nd vaccine dose: 19 days [range: 6-29], 26% COVID19 survivors). The metadata for the serum samples are summarized in Supplementary Table 3.
3.3. Antibody neutralization activities
We used a well-established neutralization assay to assess the neutralizing activity of sera from vaccinees against the seven different VoCs and VoIs as well as the wild type WA1 isolate [20]. In this assay, 60 tissue culture infectious doses of virus per well are pre-incubated with the respective serum dilutions for one hour. The serum/virus mix is then used to infect Vero.E6 cells. After the infection step, the cell culture medium is replaced with fresh medium containing the respective concentration of serum. The assay allows for production of the virus and multi-cycle replication of the test virus in the continuous presence of different concentrations of test serum, which recapitulates infection conditions in vivo.
The absolute titers in ID50 and the fold changes in mean GMT are summarized in Fig. 2 (N=28 sera, 8 viruses). The ID50 values range over two-order of magnitude for each viral variant. There was no statistically significant difference in neutralization activity between the WA1/USA wild type isolate (GMT: 576), the ancestral Iota variant (no mutation in RBD, GMT: 478, 1.2-fold reduction) and the Iota variant with the E484K substitution (GMT: 375, 1.5-fold reduction). There was, however, a small but statistically significant reduction in neutralization activity against the Iota variant with the S477N substitution (GMT: 298, 1.9-fold reduction; P < 0.5) and the Delta (GMT: 218, 2.6-fold, P < 0.01). The Alpha+E484K (GMT: 169, 3.4-fold, P < 0.01), the Beta variant (GMT: 160, 3.6-fold, P < 0.01) and the Lambda subvariant (GMT: 145, 4.0-fold, P < 0.01; Fig. 2) showed a moderate reduction in susceptibility. Of note, as mentioned in Section 3.1 above, virus isolate PV29369 carries additional substitutions/deletions in spike (see Supplemental Table 3) compared to the majority of circulating C.37 variants. A mixed effect analysis with Dunnett's multiple comparisons test was used for the statistical analysis.
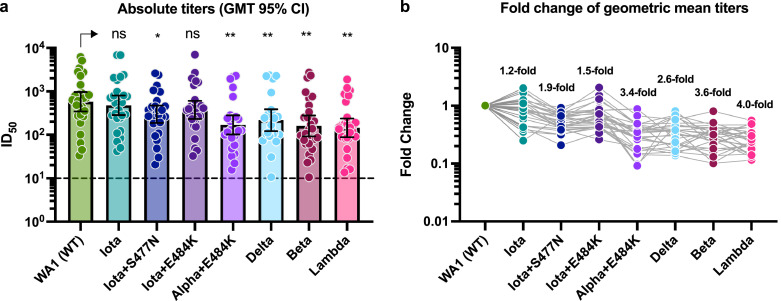
Neutralizing activity of post-mRNA vaccination sera against different VoIs and VoCs. a: Absolute neutralization titers against the respective variant SARS-CoV-2 isolates. The geometric mean titers (GMT) with the 95% confidence intervals are depicted for each viral variant. A mixed effect analysis with Dunnett's multiple comparisons test was used for statistical analysis. NS: not significant, *: P < 0.05; **: P < 0.01
b: Fold reduction of geometric mean neutralization titers as compared to the wild type reference WA1 isolate. The grey connecting line identifies a given serum sample. A list of specific mutations found in the spike region of the clinical isolates tested can be found in Supplementary Table 2.
Since we and other have reported that pre-existing immunity increases vaccine-inducted antibody responses [25], [26], [27], we next analysed the neutralization data shown in Fig. 2 depending on whether or not the sera was collected from participants with or without documented COVID-19 prior to immunization. Sera from participants who were naïve prior to vaccination (N=20, 8 viruses) neutralized WT WA1/USA (GMT: 356) and Iota (parental, GMT: 289, P: not significant) to comparable levels followed by – in decreasing order – Iota+E484K (GMT: 233, P: 0.0019), Gamma (GMT: 120, P: 0.0004), Iota+S477N (GMT: 197, P: 0.0009), Alpha+484K (GMT: 99, P: 0.0001), Beta (GMT: 91, P: < 0.0001) and Lambda subvariant (GMT: 84, P: < 0.0001) (Fig. 3a). A mixed effect analysis with Dunnett's multiple comparisons test was used for statistical analysis. The same pattern was observed for the sera from eight participants who had documented COVID-19 prior to vaccination alby the overall neutralization titers were 6-fold higher and the range of titers varied more (Fig. 3b).
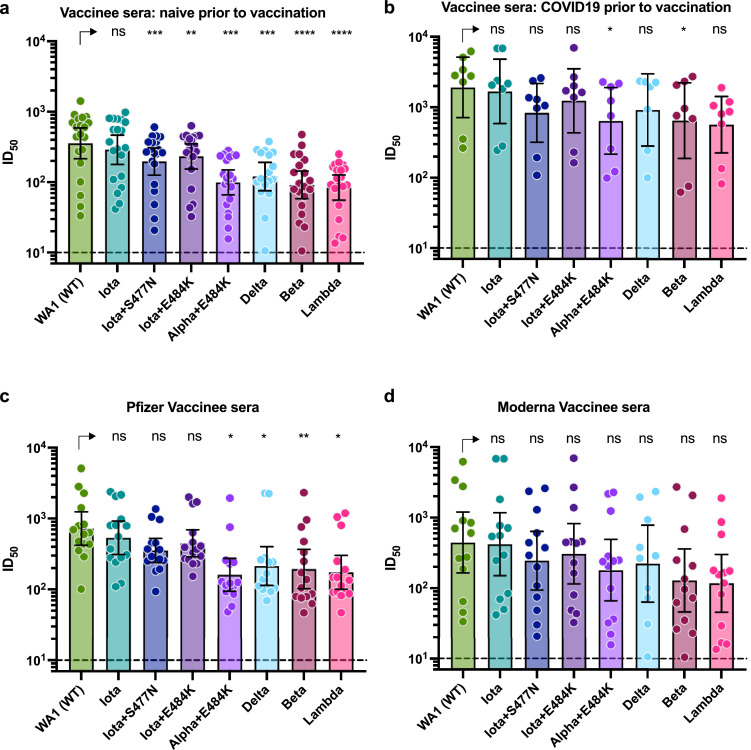
Neutralizing activity of post-mRNA vaccination sera relative to pre-existing immunity or vaccine type a and b: Absolute neutralization titers of sera from participants that were seronegative prior to vaccination (a) and participants that were seropositive prior to vaccination (b). Individual data points depicting the absolute neutralization titers for each sample against the respective variant viruses are shown. Error bars depict the GMT with 95% confidence intervals.
c and d: Absolute neutralization titers of sera from that received two doses of the Pfizer vaccine (c) or the Moderna vaccine (d). Individual data points depicting the absolute neutralization titers for each sample against the respective variant viruses are shown. Error bars depict the GMT with 95% confidence intervals.
A mixed effect analysis with Dunnett's multiple comparisons test was used for statistical analysis in all four panels. NS: not significant, *: P < 0.05; **: P < 0.01; ***: P < 0.001; ***: P < 0.0001
Lastly, we analysed the neutralization data set depending the vaccine type administered (sera from 15 BNT162b2/Pfizer vaccinees, Fig. 3c, and 13 mRNA-1273/Moderna vaccinees, Fig. 3d). For the BNT162b2/Pfizer vaccine recipients, Delta (GMT: 214, P: 0.0143), B.117+484K (GMT: 161, P: 0.0134), Beta (GMT: 194, P: 0.0092) and Lambda subvariant (GMT: 174, P: 0.0240) showed significant reductions in neutralization while the reduction in neutralization titers for mRNA-1273/Moderna recipients did not reach level of significance (Fig. 3c and d). A mixed effect analysis with Dunnett's multiple comparisons test was used for statistical analysis. Of note, the samples from vaccinees who received mRNA-1273/Moderna were collected within the first two weeks after receiving the second vaccine dose while the majority of samples from BNT162b2/Pfizer vaccinees were collected two or three weeks after the second vaccination which likely explains the differences between vaccine types.
3.4. Antibody binding activity
We next determined antibody-binding activity against four variant RBDs and six variant spike proteins. While we would have ideally matched the recombinant proteins exactly with the strains tested in the neutralization assays, access to the recombinant proteins was limited. However, we decided that including these data, even if incomplete, would provide important information. The fold- reduction in binding against variant RBDs from Alpha, Beta and Gamma was negligible with a maximum reduction of 1.6-fold against the Beta RBD (Fig. 4a and b). Only the reduction for B.1.351 reaches the level of statistical significance (P < 0.05). An one way Anova analysis with Dunnett's multiple comparisons test was used for statistical analysis.
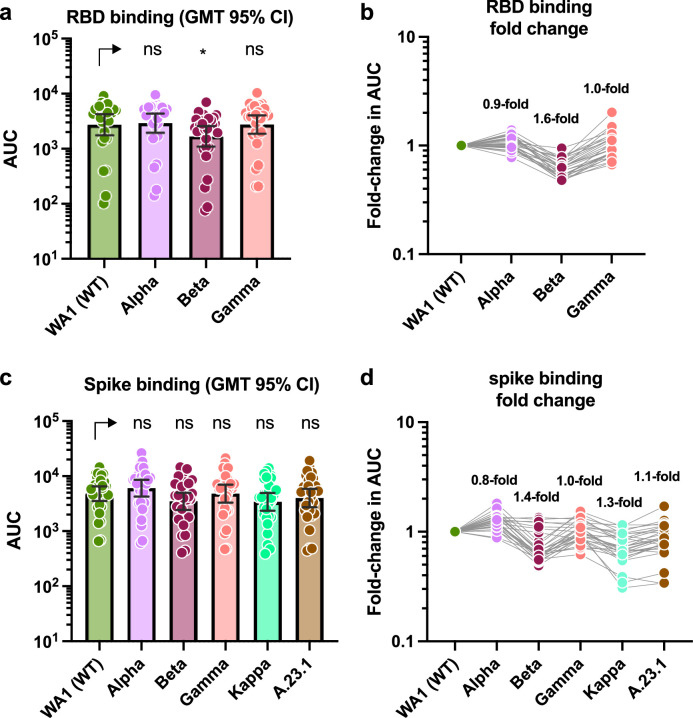
Binding activity of post-mRNA vaccination sera against RBD and spike proteins of different VoIs and VoCs. a and c show absolute area under the curve (AUC) binding values against various RBD and spike proteins. The geometric mean titers (GMT) with the 95% confidence intervals are depicted for each protein. One way Anova analysis with Dunnett's multiple comparisons test was used for statistical analysis. NS: not significant, *: P < 0.05 b and d show fold-reduction in binding as compare to the Washington derived wild type proteins.
Similarly, reduction in binding to Alpha, Beta, Gamma, Kappa and A.23.1 spike proteins was minimal, again with Beta showing the strongest impact with only a 1.4-fold reduction (Fig. 4c and d). However, this change was not statistically significant. An one way Anova analysis with Dunnett's multiple comparisons test was used for statistical analysis.
The reduction in binding to full length spike of e.g. B.1.351 was lower than the reduction of binding to the RBD of the same strain, which was expected since the full length spike does include many additional epitopes which remain unchanged. Interestingly, the impact of VoCs on binding was much lower than on neutralization. This can be explained by the fact that more binding than neutralizing epitopes exist on the spike and that mutations in VoCs often preferentially accumulate in neutralizing epitopes of the RBD and the NTD.
4. Discussion
Monitoring the effectiveness of vaccination in the context of evolving viral variants is of great public health concern for control of the COVID-19 pandemic. Indeed, neutralizing antibodies play a major role in protection from COVID-19, as shown both in animal models as well as in humans [17,18,[28], [29], [30]]. It is, therefore, important to continuously monitor emerging variants and their impact on neutralizing activity of post-vaccination serum.
Our data show a spectrum of neutralizing activities against a panel of VoIs and VoCs. The least negative impact on neutralization was seen for Iota variant, which is reasonable since this isolate has very few changes in the spike protein (T95I, D253G and D614G) compared to the wild type WA1 isolate. The impact was higher for the Iota isolates that carry, in addition, E484K or S477N substitutions in the RBD. Interestingly, S477N exerted a stronger impact than E484K, which was surprising given the reduction caused by introduction of E484K into the WA1 wild type backbone [31]. However, S477N has also been described as escape mutation [32]. The combination of Alpha (which already harbours two deletions in the NTD) with E484K led to a some reduction in neutralizing activity. However, although Alpha (E484K) emerged several times independently, it does not seem to spread well – potentially due to the detrimental effect that the E484K mutation can have on binding affinity to human ACE2 [16]. As expected and already shown by several publications, [33,[34], [35], [36], [37]] neutralizing activity to Beta was significantly reduced likely due to the three changes in RBD and extensive changes in the NTD. Interestingly, neutralizing activity was similarly reduced for the local Lambda isolate used in the current study which has more extensive changes in the NTD (Δ63-75, Δ246-252) and the RBD (L452Q, E471Q, F490S) compared to the predominant Lambda variant (NTD: G75V, T76I, Δ246-252; RBD: L452Q, F490S) [13]. While there was a clear reduction in neutralizing activity of post-vaccination sera against this variant, all sera tested retained, at least partial, neutralization activity against this Lambda variant virus which may indicate that-similar to Beta [38]-mRNA vaccines will remain effective against these VoIs. Of note, the additional changes in the local Lambda isolate likely contribute to the immune evasion phenotype shown here and should be seen as worst-case scenario for the Lambda variant. In support of this notion, others have tested the impact of ‘consensus’ Lambda variants on neutralization, which seems to be much lower than what we observe here with our local subvariant (preprints [39,40]).
The reduction in neutralization against Delta was modest, which in general aligns well with the preserved vaccine effectiveness against this variant ([41], [42], [43], [44]; [42], [43], [44] are pre-prints). However, more recent reports suggest a sharper drop in vaccine effectiveness against Delta than anticipated [44,45]. This could have several reasons including the ability of Delta to replicate to very high titers in the upper respiratory tract (preprint [46]) (contributing to its higher R0) [47] and its stronger fusogenicity (preprint [48]) that may lead to fusion at the cell surface instead of using an endosomal entry pathway as well as to more cell to cell spread (making it harder to neutralize). However, there are also host factors that need to be taken into account. Many individuals in high-income countries were vaccinated in spring and antibody levels are declining to some degree. This, combined with the viral factors may explain the somewhat sharper than expected drop in vaccine effectiveness seen in some but not all studies.
Of note, we used a testing panel consisting of 30 sera from individuals with or without pre-existing immunity to SARS-CoV-2 who then got vaccinated with the Pfizer/BNT162b2 or mRNA-Moderna/1273 vaccines. We provide aggregated data as well as data sets in which we divide the 30 serum samples according to these criteria. However, due to the small number of samples and the differences in terms of when the Pfizer/BNT162b2 samples and the Moderna/mRNA-1273 were taken, no direct comparison between these modalities can be made. However, they all show the same trend in reduction of neutralization pattern against the selected variants, which is reassuring. Another important limitation is, that samples were taken relatively shortly post-dose two, and reduction in neutralizing activity after a longer period of time might be different than during the peak of the antibody response.
Another interesting finding was that the reduction in binding activity to variant RBD and spike proteins was negligible. However, while neutralization activity is mostly dependent on only a few epitopes in the RBD and NTD, many more binding epitopes exist on the spike [16] which may explain our findings. Interestingly, a recent study found even better correlation between binding activity and vaccine effectiveness than for neutralizing activity and vaccine effectiveness [17]. This may indicate that binding antibodies – even if they lack neutralizing activity – are involved in protection. It is well known that non-neutralizing antibodies raised upon infection or vaccination by Ebola or influenza viruses, can be protective via Fc-mediated effector functions [49], [50], [51], [52]. Future studies are needed to establish if this is also the case for SARS-CoV-2. However, the finding that the drop in binding activity is negligible even against spike proteins from variants with extensive changes like Beta is reassuring in that context.
Contributors
K.S., E.M.S., and V.S. provided clinical samples for the study. H.A., and A.A. accessioned clinical sample. H.A. performed RNA extraction and measured viral titers. A.S.GR. and A.G. performed NGS experiments. H.B. provided NGS services. A.S.GR. and H.B. developed assembly pipeline and performed genome assembly. J.M.C., G.S., and D.N.S performed ELISA assays. J.M.C., H.A., and A.R. performed MNT assays. J.M.C., H.A., G.S., A.R., F.A., A.S.GR., E.M.S., H.B., F.K., and V.S. analyzed, interpreted, or discussed data. J.M.C., H.B., F.K., and V.S. wrote the manuscript. H.B., F.K., and V.S. conceived the study. J.M.C., H.B., F.K., and V.S. supervised the study. F.K., and V.S. raised financial support. J.M.C, F.K and V.S. have verified the underlying data. J.M.C., H.A., G.S., A.R., F.A., A.A., A.S.GR., A.G., K.S., E.M.S., D.N.S, H.B., F.K., and V.S. reviewed the final version of the manuscript. The PARIS study group selected, reached out and coordinated additional study visits of well-characterized study participants and processed, banked, analysed and distributed the collected serum samples.
Declaration of Competing Interest
The Icahn School of Medicine at Mount Sinai has filed patent applications relating to SARS-CoV-2 serological assays and NDV-based SARS-CoV-2 vaccines which list Florian Krammer as co-inventor. Viviana Simon and Fatima Amanat are also listed on the serological assay patent application as co-inventors. Mount Sinai has spun out a company, Kantaro, to market serological tests for SARS-CoV-2. Florian Krammer has consulted for Merck and Pfizer (before 2020), and is currently consulting for Pfizer, Seqirus and Avimex. The Krammer laboratory is also collaborating with Pfizer on animal models of SARS-CoV-2.
Acknowledgments
We thank the study participants for their generosity and willingness to participate in longitudinal COVID19 research studies. None of this work would be possible without their contributions.
We gratefully acknowledge the authors, originating and submitting laboratories of the genetic sequence and metadata made available through GISAID that were used as background to place our samples in the context of the global diversity. We would like to thank Dr. Randy A. Albrecht for oversight of the conventional BSL3 biocontainment facility, which makes our work with live SARS-CoV-2 possible. We are also grateful for Mount Sinai's leadership during the COVID-19 pandemic. We want to especially thank Drs. Peter Palese, Carlos Cordon-Cardo, Dennis Charney, David Reich and Kenneth Davis for their support and Daniel Caughey for expert administrative assistance.
Funding
This work is part of the PARIS/SPARTA studies funded by the NIAID Collaborative Influenza Vaccine Innovation Centers (CIVIC) contract 75N93019C00051. In addition, this work was also partially funded by the Centers of Excellence for Influenza Research and Surveillance (CEIRS, contract # HHSN272201400008C), the JPB Foundation, the Open Philanthropy Project (research grant 2020-215611 (5384); by anonymous donors. ASGR was supported by the Marion Alban MSCIC Scholars Award and the 2020 Robin Chemers Neustein Postdoctoral fellowship. Finally, this effort was also supported by the Serological Sciences Network (SeroNet) in part with Federal funds from the National Cancer Institute, National Institutes of Health, under Contract No. 75N91019D00024, Task Order No. 75N91020F00003. The content of this publication does not necessarily reflect the views or policies of the Department of Health and Human Services, nor does mention of trade names, commercial products or organizations imply endorsement by the U.S. Government.
Data sharing statement
All of the data generated during this study are included in this published article. All data supporting the findings in this study are also available upon reasonable requests from the corresponding authors upon request.
Footnotes
PARIS study group (in alphabetical order, Study group/Consortium): Mahmoud Awawda, Radhika Banu, Katherine Beach, Carolina Bermúdez-González, Dominika Bielak, Liyong Cao, Rachel Chernet, Parnavi Desai, Shelcie Fabre, Emily. D. Ferreri, Daniel Floda, Charles Gleason, Hisaaki Kawabata, Zenab Khan, Dr. Giulio Kleiner, Dr. Denise Jurczyszak, Julia Matthews, Wanni Mendez, Dr. Lubbertus CF Mulder, Dr. Alberto E Paniz-Mondolfi, Kayla Russo, Ashley Salimbangon, Dr. Miti Saksena, A. Shin, Levy A. Sominsky, Johnston Tcheou
Supplementary material associated with this article can be found in the online version at 10.1016/j.ebiom.2021.103626.
References
Articles from eBioMedicine are provided here courtesy of Elsevier
Citations & impact
Impact metrics
Citations of article over time
Alternative metrics

Discover the attention surrounding your research
https://www.altmetric.com/details/115733658
Article citations
Neutralisation resistance of SARS-CoV-2 spike-variants is primarily mediated by synergistic receptor binding domain substitutions.
Emerg Microbes Infect, 13(1):2412643, 13 Nov 2024
Cited by: 0 articles | PMID: 39392057 | PMCID: PMC11562025
SARS-CoV-2 serosurvey across multiple waves of the COVID-19 pandemic in New York City between 2020-2023.
Nat Commun, 15(1):5847, 11 Jul 2024
Cited by: 1 article | PMID: 38992013 | PMCID: PMC11239669
Binding and Avidity Signatures of Polyclonal Sera From Individuals With Different Exposure Histories to Severe Acute Respiratory Syndrome Coronavirus 2 Infection, Vaccination, and Omicron Breakthrough Infections.
J Infect Dis, 228(5):564-575, 01 Aug 2023
Cited by: 4 articles | PMID: 37104046 | PMCID: PMC10469125
A SARS-CoV-2: Companion Animal Transmission and Variants Classification.
Pathogens, 12(6):775, 29 May 2023
Cited by: 4 articles | PMID: 37375465 | PMCID: PMC10302237
Review Free full text in Europe PMC
Multi-omic longitudinal study reveals immune correlates of clinical course among hospitalized COVID-19 patients.
Cell Rep Med, 4(6):101079, 23 May 2023
Cited by: 16 articles | PMID: 37327781 | PMCID: PMC10203880
Go to all (27) article citations
Data
Data behind the article
This data has been text mined from the article, or deposited into data resources.
BioStudies: supplemental material and supporting data
Similar Articles
To arrive at the top five similar articles we use a word-weighted algorithm to compare words from the Title and Abstract of each citation.
Antibody affinity maturation and cross-variant activity following SARS-CoV-2 mRNA vaccination: Impact of prior exposure and sex.
EBioMedicine, 74:103748, 10 Dec 2021
Cited by: 12 articles | PMID: 34902788 | PMCID: PMC8662368
Elicitation of Broadly Neutralizing Antibodies against B.1.1.7, B.1.351, and B.1.617.1 SARS-CoV-2 Variants by Three Prototype Strain-Derived Recombinant Protein Vaccines.
Viruses, 13(8):1421, 22 Jul 2021
Cited by: 6 articles | PMID: 34452287 | PMCID: PMC8402859
Establishment of human post-vaccination SARS-CoV-2 standard reference sera.
J Immunol Methods, 530:113698, 31 May 2024
Cited by: 2 articles | PMID: 38823574
Will Mutations in the Spike Protein of SARS-CoV-2 Lead to the Failure of COVID-19 Vaccines?
J Korean Med Sci, 36(18):e124, 10 May 2021
Cited by: 47 articles | PMID: 33975397 | PMCID: PMC8111046
Review Free full text in Europe PMC
Funding
Funders who supported this work.
NCI NIH HHS (1)
Grant ID: 75N91019D00024
NIAID NIH HHS (2)
Grant ID: 75N93019C00051
Grant ID: HHSN272201400008C
NIH HHS (1)
Grant ID: S10 OD026880