Abstract
Free full text

Current Trends in IBD—Development of Mucosal-Based Biomarkers and a Novel Minimally Invasive Recoverable Sampling System
Abstract
Despite recent developments in therapy for inflammatory bowel diseases (IBDs), there have been limited advances in diagnostic tools available to aid in disease management. A growing body of evidence suggests that there are important host-microbe interactions at the mucosal interface that modulate the inflammatory response in patients with IBD. Additionally, the importance of mucosal integrity and its disruption appears to be important in the pathophysiology and perpetuation of the disease. The ability to characterize this interface may provide valuable information for both disease monitoring and identification of new treatment targets. Endoscopy remains the primary tool for disease monitoring, and mucosal healing is the primary therapeutic target in IBD treatment. However, establishing mucosal healing requires repetitive endoscopic procedures, and endoscopy is limited by factors such as invasiveness, cost, and risk of adverse events. Moreover, the use of a bowel preparation for colonoscopies alters the mucus layer and thus perturbs evaluation of the host-microbe interaction. Stool sampling may also be inaccurate because it reflects the end state of metabolites and proteins, failing to take into account the degradation or alteration of substrates of interest by bacterial proteases and other enzymes during passage through the colon.
A novel sampling capsule, called the Recoverable Sampling System (RSS), is being developed as a complementary tool to colonoscopy. The RSS is intended to be a platform for noninvasive autonomous sampling, preservation, handling, and storage of analytes of interest found in the gastrointestinal fluids. A proprietary preservative contained within the chambers of the capsule has been developed to stabilize DNA and proteins for ex vivo microbiome and metabolomics analyses.
Surrogate markers such as SPP24 and GUCA2a have been identified to correlate with gut health, intestinal permeability, and inflammation and could be locally sampled by the RSS. The potential clinical utility of an RSS device is broad and would likely be able to guide therapy by allowing for more frequent disease monitoring, aiding in disease characterization, and facilitating in the identification of novel therapeutic targets.
Background and Unmet Medical Need
Inflammatory bowel diseases (IBDs) are heterogeneous chronic inflammatory conditions of the gastrointestinal (GI) tract with variable rates of progression and outcomes.1,2
In ways that are not fully understood, alterations in the gut microbiome in susceptible individuals contribute to the dysregulation of the immune response.3,4 in ways that are not fully understood. As a consequence, disease severity and treatment response can be heterogeneous, with approximately 80% of patients experiencing relapse and flares.5,6 Diagnosis is based on clinical, endoscopic, and histologic characteristics combined with other supportive laboratory findings. After diagnosis, however, the life-long management of IBD is impeded due to the inability of currently available biomarkers and noninvasive technologies to adequately guide therapy, monitor disease, predict response, and prognosticate disease progression.7 Current means to assess disease activity include invasive and expensive measures such as colonoscopy, magnetic resonance (MR) or computerized tomography enterography, and nonspecific markers such as C-reactive protein (CRP) and fecal calprotectin (FC).8
The goal of therapy is to achieve mucosal healing, which is characterized by endoscopic resolution of inflammation. Growing evidence suggests that histologic resolution of inflammation may result in improved long-term outcomes,9,10 although there is still debate regarding the definition of histological remission. In recent years, the importance of epithelial barrier integrity has garnered interest due to its reported role in the pathogenesis of IBD and disease prognosis.11,12 The interaction between microbes and mucosal immune components in regulating immunity has also been increasingly recognized as a key factor in IBD pathogenesis.13 In IBD, mucosal lesions are generated by an excessive or dysregulated immune response against foreign antigens in the gut. However, a better understanding of the complex human intestinal ecosystem is still needed to understand the exact role of the microbiota in health and disease.14 These recent findings highlight the importance of the mucosal interface in modulating the course of IBD and thus the need for the development of new biomarkers and monitoring technologies. Such technologies should allow clinicians to accurately and frequently collect samples at this interface to measure biomarkers for detailed analysis in a noninvasive manner. Currently, there is an overemphasis on the assessment of inflammation, with limited qualitative evaluation of the intestinal mucosa through colonoscopy and histology. In this setting, there are a number of forthcoming candidate biomarkers aimed at addressing the underrepresented facets of IBD pathology in clinical diagnostic modalities, namely the microbiome and the intestinal mucosal barrier function. In this article, we will review the latest advances in mucosal biomarkers and a new technology currently in development that would allow for more frequent analysis of these evolving biomarkers to help guide therapy.
Gut Dysbiosis as a Biomarker of Inflammatory Bowel Disease
The gut microbiota contributes significantly to IBD pathogenesis and can be manipulated for therapeutic effect.15 Accordingly, there is concurrent evidence that the gut microbiota is a source of highly specific and sensitive indicators that are prognostic and even predictive of therapeutic potential, which have been made accessible through techniques such as 16s RNA and metagenomic sequencing (Table 1).
Table 1.
Recent novel intestinal mucosa-based biomarker, therapeutic target, and agent candidates in inflammatory bowel diseases.
Type | Potential Clinical Utility | Candidate | Description | Reference(s) |
---|---|---|---|---|
Microbial | Biomarker | Roseburia inulivorans/branch-chained amino acids (BCAAs) | Greater baseline abundance of Roseburia inulinivorans has been associated with achieving remission with vedolizumab therapy in CD and UC, and more generally, greater abundance of multiple Clostriales is associated with anti-TNF response. Baseline BCAA pathways predict vedolizumab response in CD and may be the functional biomarkers of R inulinivorans and like species that can transcend individual differences. | Estevinho et al, Ananthakrishnan et al20,55 |
Biomarker/therapeutic agent | Faecalibacterium prausnitzii/anti- microbial peptides (AMPs) | Faecalibacterium prausnitzii is a particularly CD pathogenesis, associated with both medical and surgical treatment response in CD. F. prausnitzii produces short chain fatty acids which in turn elicit AMP production. AMPs such as defensin-5, bactercidal permeability-increasing protein, eosinophil cationic protein, and others, discriminate response and nonresponse in CD. These functional biomarkers may be the mechanism of the homeostatic properties of F. prausnitzii, and stand to have predictive and therapeutic utility. | Solberg et al, Pascal et al4,6,55 | |
Therapeutic target | Type 1 fimbrin D-mannose specific adhesin (fimH) | FimH is an adhesion protein at the tip of type 1 pili expressed by gram-negative bacteria that attaches the organism to intestinal epithelial cells, such as in adherent-invasive E. coli. FimH blockade in phase 1 clinical trials for CD have proved safe thus far and is novel in its action of specific gut microbiota. | Reinisch et al, Sivignon et al56–58 | |
Barrier Function | Biomarker | Secreted Phosphoprotein 24 kDa (SPP24) | SPP24 is a serine protease inhibitor highly correlated with intestinal permeability in human IBD. SPP24 has been implicated in LPS recognition and antimicrobial peptide signaling and may be a clinically useful marker of barrier function when sampled directly from the intestinal mucosa. | Wasinger et al, Tian et al, Zhao et al39,40,42 |
Biomarker | Guanylin (GUC2a) | GUC2a is a neuroendocrine peptide hormone that differentiates human CD and UC. It is secreted by goblet cells that activates the intestinal epithelial receptor guanylate cyclase, regulating the movement of water and ions along the crypt-surface axis. | Wasinger et al42 | |
Therapeutic target | Mas-Related G Protein-Coupled Receptor-X2 (MRGPRX2) | MRGPRX2 is a G-protein-coupled receptor expressed on mast cells of which its variants discriminate between inflamed and noninflamed UC tissue. Mast cell degranulation results in inflammation and psuedo-allergic type reactions. Blocking of MRGPRX2 may be a novel therapeutic target in UC. | Chen et al45 | |
Biomarker | Selenoprotein P | Selenoprotein P is an antioxidant secreting protein that is decreased in the UC intestinal epithelium and is mechanistically linked to colitis associated cancer in mice. Selenoprotein P may have potential as a UC disease activity and colon cancer marker. | Short et al59 | |
Biomarker | Fucosylated haptoglobin (Fuc-Hpt) | Fuc-Hpt is produced by infiltrating lymphocytes in the mucosa and in UC. Fuc-Hpt correlates with CRP in IBD and acute enteritis but not in controls. Fuc-Hpt may have utlity as a large-bowel specific inflammation marker. | Motooka et al60 | |
Therapeutic agent | Tauroursodeoxycholate (TUDCA) | TUDCA is a chemical endoplasmic reticulum (ER) chaperone that reduces ER stress by stabilizing protein-folding intermediates and stopping protein aggregation. ER stress is a feature of the ileal and colonic epithelium in CD and oral administration of TUDCA has shown to attenuate inflammatory tissue damage in mouse colitis. | Cao et al61,62 | |
Therapeutic agent | Ursodeoxycholic acid (UDCA), Lithocholic acid (LCA) | UCDA is a hydrophilic secondary bile acid that protects intestinal epithelial barrier function from LPS-induced injury in mouse colitis. UCDA promotes epithelial cell migration to injury sites through a cyclooxygenase 2 (COX2) and epidermal growth factor receptor (EGFR) mechanism. LCA is a bacterial breakdown metabolite of UCDA that may be the direct effector of intestinal barrier protection. | Golden et al, Ko et al, Lajczak-McGinley et al61,63–65 | |
Therapeutic target/agent/biomarker | Interleukin-22 (IL-22) | In the GI tract, IL-22 is a ubiquitous component of barrier homeostasis; directly inducing mucin production in the epithelial barrier through STAT3-dependent signaling, eliciting antimicrobial peptides, and upregulating claudin-2 to elicit water efflux and pathogen clearance, amongst other roles. Its ubiquity points to a complex paradoxical role in IBD—its administration and neutralization have both attenuated disease in animal models, and its expression may be site and phenotype specific. Detailed sampling of this important entity in the GI tract in vivo stands to have significant clinical implications. | Keir et al66 |
Overall, there is reduced gut microbial diversity in IBD.16 In Crohn’s disease (CD), the gut microbiota is characterized by a decreased abundance of species from the Firmicutes phylum and an increase in Proteobacteria species.15,16 Notably, increased abundance of adherent invasive Escherichia coli appears to be associated with the ileal CD phenotype.15,17 The ulcerative colitis (UC) gut microbial profile is less generalizable but also broadly exhibits a decrease in Firmicutes species such as Roseburia hominis and Faecalibacterium prausnitzii.17,18 Importantly, there is considerable evidence from therapeutic clinical trials that baseline microbial profiles cluster according to therapeutic response. Multiple trials of various IBD therapeutic modalities have shown an association between a greater baseline diversity in gut microbiota and primary response,19,20 with several multivariate models able to predict treatment outcomes with high levels of accuracy. These models are yet to be independently replicated, and specific predictive genera of bacterium are impractical to meta-analyze, given the heterogeneity of treatment, protocol, and patient groups among different trials. Nonetheless, there have been some noteworthy species of interest. Faecalibacterium prausnitzii is decreased in UC and CD and has been independently shown to predict relapse following both medical and surgical treatment in CD.4,6 Increased baseline levels of Roseburia inulivorans have been strongly associated with primary response to vedolizumab and fecal microbiota transplantation (FMT) in CD and UC, respectively.19,20
The mechanism behind the association of Faecalibacterium prausnitzii and Roseburia inulivorans with IBD outcomes may lie in their anti-inflammatory characteristics. Faecalibacterium prausnitzii modulates interleukin (IL)-12 and interferon-γ and stimulates IL-10, possibly through the species’ innate production of anti-inflammatory peptides.19Roseburia inulivorans synthesizes short chain fatty acids (SCFAs), a primary energy source for colonic epithelial cells and a promoter of colonic regulatory T cells. In particular, Roseburia inulivorans is a major producer of butyrates, which downregulate pro-inflammatory responses in intestinal epithelial cells. This is significant because the production of butyrates has consistently shown a strong association with IBD remission across a range of clinical settings.15 Ultimately, there is substantial interindividual variance in the gut microbiome, with factors such as age, body mass index, geography, and lifestyle conferring a greater effect on gut microbial composition than IBD.15,21 This, combined with the complex interactions between different species within the gut ecology, indicates that outcome is more likely to be determined by the resultant functional changes of an altered microbiota than by isolated alteration of a specific microbial species.22 Therefore, measuring alterations in the gut metabolomic and proteomic profiles may be valuable means of gauging the consequences of microbial modulations.
Limitation of Stool as a Diagnostic Medium
Presuming that the metabolic profile of an altered gut ecology is deterministic of disease, proteins and metabolites are then likely to be direct mechanistic biomarkers of IBD.22 Luminal proteins and metabolites may be more robust as biomarkers compared with specific microbes, as the inherent metabolic redundancy of gut ecology transcends individual differences.2 However, this makes stool a very challenging diagnostic medium because it harbors immense numbers of endogenous and exogenous proteases that may digest pathogenically relevant proteins during passage through the colon. Moreover, stool will not capture mucosal adherent microbes or those that reside in the small intestine where the host-microbe interaction is most active.22,23 Finally, stool poses a significant technical challenge for high-sensitivity chromatography-coupled mass spectrometry instruments—the mainstay of proteomic and metabolomic assays.24 Stool samples require made-to-spec protocols to remove major contaminants while preserving the sample for the analytes of interest; failure to do so can cause significant fouling of chromatography columns and unstable mass spectrometer performance.24,25 Method development for new stool-based analytes that have acceptable sample preparation times for automated clinical mass spectrometry is a significant investment.25,26
Importance of Epithelial Integrity
The intestinal lining maintains a balance of immune education, tolerance to commensal organisms and response through cell turnover, and a highly specialized environment of self and nonself interactions within a dynamic ecosystem.27 The intestinal mucosa consists of a single layer of epithelial cells with an overlying, mucin-containing chemical barrier, together forming a physical barrier to the contents of the intestinal lumen. The composition of the chemical barrier varies along the GI tract; the small intestine contains antimicrobial peptides, whereas the large intestine contains immune cells and an additional mucus layer. This barrier is made permeable by junctional proteins connecting the epithelial cells to the intracellular actin framework, thus controlling the paracellular passage of solutes and antigens.28 In UC and CD, the mucosa and the submucosa are breached. In severe cases of CD, the damage can extend through to the serosa, often requiring surgical intervention. This close proximity between sterile and hostile environments places the epithelial barrier at the epicenter of the immune response. Undeniably, it is the prevailing dichotomy of this immune response to commensals and to the extraneous microbiota barrage of the epithelial architecture that contributes to both the unique microbial signature of an individual29–31 and to the relapse and IBD disease progression with dysbiosis, which persists even in long-term remission.32 A loss of barrier function and a breach in permeability precedes active disease in IBD, even in macroscopically normal areas of the intestine.33–37 There is significant primary research into therapeutic candidates that target restoration and protection of the mucosal barrier in the context of IBD, with promising ex vivo results and results in animal IBD models (Table 1). A significant hurdle for progression of many of these candidates is the difficulty in delivering test agents directly to the sites of intestinal injury.
Other Mucosal Markers
Several studies have demonstrated that increased mucosal permeability aligns with IBD and irritable bowel syndrome (IBS)–like symptom severity.36,38 We have recently identified that a relative decrease in intestinal permeability in IBD patients is associated with an increased likelihood of sustained clinical remission, with a particularly strong relation in UC.36 Importantly, we have found that changes in secretion of phosphoprotein 24 (SPP24) are highly correlated with intestinal permeability as measured by confocal laser endomicroscopy.36,39,40 Secretion of phosphoprotein 24 is a serine protease inhibitor that is released into the blood and bound to other proteins to ameliorate the release of tumor necrosis factor (TNF-α).39–42 Peptides from the transforming growth factor (TGF-b) type 2 domain of this protein may act as a pseudoreceptor to bone morphogenic protein 2 (BMP-2)41 and are involved in endocytic signaling and lipid metabolism.39 In addition, a second serum and tissue detectable protein marker, guanylin (GUC2a), has been identified to differentiate between CD and UC patients.42 The neuroendocrine peptide hormone GUC2a is secreted into the lumen by goblet cells and is the endogenous activator of the intestinal epithelial receptor guanylate cyclase, regulating the movement of water and ions along the crypt-surface axis. Through clever molecular mimicry of heat-stable enterotoxins, stimulation of the guanylate cyclase receptor through the same receptor binding region as GUC2a causes fluid loss and diarrhea. Furthermore, guanylin hormone loss has been previously reported in CD42,43 and has been shown to drive the development of colon cancer.44 As such, SPP24 and GUC2a have enormous diagnostic and therapeutic potential as gut-specific biomarkers of intestinal permeability, including as therapeutic targets for the treatment of IBS and IBD. However, to obtain accurate sampling, they would first require protection from gut proteases such as through novel technology sampling systems.
Recent evaluation of mucosal markers of disease activity has revealed promising treatment targets. Bulk RNA sequencing and lamina propria cell isolation for Mas-related G protein-coupled receptor-X2 (MRGPRX2) activation studies and single-cell RNA sequencing have demonstrated that the Ser62 allele in MRGPRX2, a mediator of immunoglobulin E (IgE) activation of mast cells, is protective against the development of UC. Possible blocking of MRGPRX2 may, therefore, represent a novel therapeutic target in UC, though further studies are needed.45 Additionally, in CD, single-cell technologies have identified a unique profile in inflamed ileal tissues characterized by immunoglobulin G (IgG) plasma cells, mononuclear phagocytes, activated T cells, and stromal cells. When present at diagnosis, this profile was associated with failure to achieve steroid-free remission with anti-TNF therapy. These findings highlight the potential for mucosal analysis to allow for the development of individualized treatment approaches.46
RSS Capsule Technology
In response to an unmet need in clinical IBD research for minimally invasive mucosa-targeting devices, revolutionary innovations in capsule technology are bound to occur over time. Such devices are needed for the assessment of intestinal permeability and treatment response via biomarkers and biosensors.47 To date, the only manner to reach mucosal surfaces is with endoscopic procedures. Colonoscopies are limited by their invasiveness; moreover, preprocedural bowel preparation disrupts the mucous layer, thus limiting the usefulness of the samples collected. Upper endoscopic procedures are generally limited in reach to the proximal jejunum without the use of the more invasive small bowel enteroscope. Collection and analysis of stool samples are limited by degradation and modification of target analytes by proteases, nucleases, and other enzymes as they track through the colon. Stool samples are therefore poorly representative of the environment at the site of disease.48 A novel capsule-based technology is currently being developed to address these challenges. The Recoverable Sampling System Capsule (RSS) is an electromechanical capsule that is able to autonomously identify its location in the GI tract and complete sample collection from a target region during the course of natural transit. As a swallowed and naturally transiting device, it represents a minimally invasive approach to investigating the environment of the GI tract (Figure 1). The capsule contains a battery-powered electronics system controlled by a microprocessor. In the center of the capsule is a detection system that illuminates and measures optical signals from the capsule environment. These optical measurements are used by a localization detection system to determine capsule position during transit. When the localization system determines the capsule has reached the targeted region, an internal motor is used to open the sampling port. This opening allows fluid to enter and become trapped in the 2 sampling chambers. The sampling port is then closed, thereby protecting the sampled contents from contamination by the downstream environment. The capsule then continues its natural transit and is excreted. Upon excretion, the capsule is recovered and sent to a lab for extraction of the sampling chamber and analysis of the contents. The RSS localization technology makes use of reflected and scattered light from GI tissue to determine key anatomical transition points during transit. The optical system consists of an array of 3-color LEDs, along with an interleaved array of photodetectors. The LEDs periodically illuminate the environment, and the returned signal is measured by the photodetectors. During transit, there is a characteristic change in relative color signals as the capsule moves from the stomach into the small bowel and again as the capsule moves from the small bowel into the colon. The onboard microprocessor analyzes the measured signals and generates a trigger signal when transitions are determined. With trigger signals in hand, the capsule function is then tailored to the intended operation. For example, when sampling in the proximal small bowel, the sampling function is initiated when the trigger signal for entry into the small bowel is determined. One advantage of this technology is that localization technology is based on anatomy and is not sensitive to individual differences in physiological conditions such as transit time and pH, which can vary significantly in the presence of IBD.49 This localization technology is also being developed for other applications such as local drug delivery at a prespecified location in the GI tract. This application is described in a separate review.50 Once the sampling function is initiated, the electronics system drives an actuator to open the sampling port of the capsule. The sampling port is covered by a movable element that, upon opening, exposes an absorbent wick. The absorbent wick draws in fluid from the capsule environment into a pair of sponges housed in the sampling chamber at the top of the capsule. The sampling port is held open for several minutes, and then the actuator is reversed to draw the movable element down, thus closing the port and protecting the sample fluid from further interaction with luminal contents. Although closing the sampling port protects the sampled content from downstream contamination, the sample itself must be protected from changing in the time between sample acquisition and biochemical analysis. This is accomplished by preloading the sample sponges with a preservative. The intestinal fluid contains cells, exosomes, protein, metabolites, nucleic acids, bacteria, and other live organisms. Conditions inside this intestinal milieu result in rapid degradation of protein and nucleotide analytes by host enzymes, in addition to enzymes from the intestinal microbiome.51,52 To stabilize proteins and the microbiome sampling that are collected by the ingestible device, preservative formulations have been developed that include a cocktail of protease inhibitors effective at preserving proteins of interest such that they can be identified when the dried preservative is solubilized into the fluid sample from the GI environment (Figure 2).
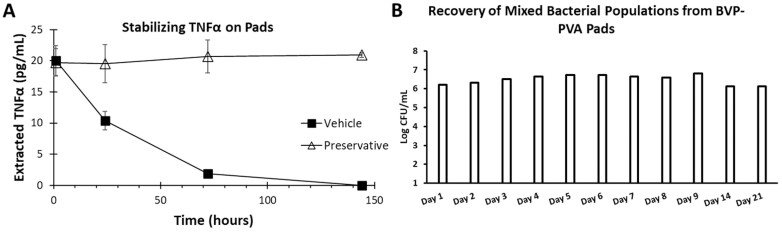
Importance of preservation of samples prior to analysis. The preservative stabilizes antitumor necrosis factor (TNF)-α spiked into fecal slurry for up to 144 hours at room temperature (A). Additionally, the ingestible device will sample the intestinal microbiome. In order to prevent bacterial overgrowth or loss of viability prior to extracting the sample from the ingestible device, a bacterial viability preservative (BVP) was also developed. B, Fecal slurry spiked with 1 × 106 colony forming units (CFUs) of mixed bacterial populations was used to solubilize the dried BVP. The BVP eliminated bacterial overgrowth and loss of viability for up to 21 days at room temperature. The RSS capsule can sample fluid inside the gastrointestinal tract, which offers the ability for frequent and real-time evaluation of drug levels and biomarkers of disease, inflammation, and mucosal healing similar to evaluating biopsies.
The novel construction of the RSS capsule lends itself to a wide and potentially expanding scope of applications. In its current design, the capsule can easily be programmed to sample different regions of the GI tract. For example, sampling in the ascending colon can be accomplished by programming the capsule to initiate the sample function immediately after determining entry into the colon. Although the RSS capsule is intended for recovery and analysis in a lab, the system may be reconfigured for onboard analysis. This “lab on pill” construction is enabled by a miniaturized assay system. An example of such a construction is a related system in development for employing a total bacterial count assay to aid in the determination of small intestinal bacterial overgrowth.53 In this system, the electronics further make use of wireless reporting to a receiver unit such that the result is fully determined in situ, thus eliminating the need to recover the capsule for later laboratory analysis. The onboard assay system may be further reconfigured towards other targets, thus creating a wide range of noninvasive, real-time diagnostic capsules.
There are currently a few limitations with the RSS technology. Based on the existing localization algorithm, an intact ileo-cecal valve is required for identification of the region to trigger sampling. Future enhancement of the algorithm may allow the capsule to sample at the site of inflammation or lesion. Intestinal stricture that can result in capsule retention in CD is also a limitation. However, the use of prior small bowel imaging and patency capsules to screen for potential capsule retention can be utilized to overcome this barrier; this is already part of common clinical practice in the use of video capsule endoscopy. Finally, cost is a limitation, but the anticipated scaling up of production and evolution of device design is expected to drive costs down, especially if their use results in widespread improved clinical care.
To confirm the potential clinical utility of the RSS capsule, several studies are planned to confirm the localization technology, associated algorithm, and functionality. The objective of one such study is to further demonstrate the accuracy of localization technology by testing it against the gold standard of scintigraphy. Next, a study is planned in healthy volunteers to assess the functionality and reproducibility of the samples collected by serial capsule ingestion within individuals. Following this, a large study is planned in IBD patients. In the first phase of this study, patients with IBD will be evaluated cross-sectionally. Next, patients requiring a change in medical therapy due to a flare of disease will be monitored longitudinally and correlated with colonoscopic findings. The study will provide extensive data to support the clinical utility of the RSS in the IBD patient population.
Conclusions
The inadequacy of our understanding of the initiation and perpetuation of IBD drives the need for research. Despite the extension of treatment paradigms towards endoscopic and histological healing, only 40% of reported IBD patients achieved clinical remission after 1 year on therapy.54 Restoration of intestinal epithelial integrity may be a more specific end point for representing full mucosal healing and, in turn, be associated with improved long-term outcomes. Increased intestinal permeability may be an important driver of the inflammatory response not only in IBD but potentially in other chronic diseases, as well. The difficulty of assessing data on barrier function in vivo underlies our current limitation in the study of this field. There is a need for tools that would allow for quantitation of intestinal permeability, monitoring local disease activity, and guiding therapy. Revolutionary innovations in capsule technology may address this unmet need, with the RSS capsule representing the first of many technologies that will be developed in the future to improve the care of IBD patients.
Glossary
Abbreviations
BMP | bone morphogenic protein |
BVP | bacterial viability preservative |
CD | Crohn’s disease |
CFU | colony forming units |
CRP | C-reactive protein |
DNA | deoxyribonucleic acid |
FCP | fecal calprotectin |
FMT | fecal microbiota transplantation |
GI | gastrointestinal |
GUCA2a | guanylin |
IBDs | inflammatory bowel diseases |
IBS | irritable bowel syndrome |
Ig | immunoglobulin |
IL | interleukin |
MRGPRX2 | Mas-related G-protein coupled receptor member X2 |
MR | magnetic resonance |
RNA | ribonucleic acid |
RSS | Recoverable Sampling System |
SCFA | short chain fatty acids |
SIBO | small intestinal bacterial overgrowth |
SPP24 | secretion of phosphoprotein-24 |
TGF | transforming growth factor |
UC | ulcerative colitis |
Contributor Information
Yunki Y Yau, School of Medical Sciences, Faculty of Medicine, The University of New South Wales, Sydney, NSW, Australia. Department of Gastroenterology, Concord Repatriation General Hospital, Sydney, NSW, Australia.
Valerie C Wasinger, Bioanalytical Mass Spectrometry Facility, Mark Wainwright Analytical Centre, The University of New South Wales, Sydney, NSW, Australia.
Robert P Hirten, Dr. Henry D. Janowitz Division of Gastroenterology, Icahn School of Medicine at Mount Sinai, New York, NY, USA.
Merodean Huntsman, University of Utah, School of Medicine, Salt Lake City, UT, USA.
Jack Stylli, Georgetown University, School of Medicine, Washington D.C., USA.
Jeff Shimizu, Progenity, Inc, San Diego, CA, USA.
Vijay Yajnik, Takeda Pharmaceuticals, Cambridge MA, USA.
Jeffrey Smith, Progenity, Inc, San Diego, CA, USA.
Shaoying N Lee, Progenity, Inc, San Diego, CA, USA.
Sharat Singh, Progenity, Inc, San Diego, CA, USA.
Christopher Wahl, Progenity, Inc, San Diego, CA, USA.
Rupert W Leong, School of Medical Sciences, Faculty of Medicine, The University of New South Wales, Sydney, NSW, Australia. Department of Gastroenterology, Concord Repatriation General Hospital, Sydney, NSW, Australia.
Bruce E Sands, Dr. Henry D. Janowitz Division of Gastroenterology, Icahn School of Medicine at Mount Sinai, New York, NY, USA.
Author Contribution
Y.Y.Y., V.C.W., R.P.H., S.N.L., V.Y., J.Shimizu, J.Smith, S.S., C.W., R.W.L., B.E.S., and E.C., contributed to the conceptualization, writing, and review of the article. M.H. and J.S. contributed to the writing and review of the article.
Conflicts of Interest
S.N.L. is an employee of Progenity. V.Y. is an employee of Takdea. J.Shimizu is an employee of Progenity. S.S. is an employee of Progenity. C.W. is an employee of Progenity B.E.S. is a consultant for Progenity. E.C. is a consultant for Progenity. All other authors have nothing to declare.
REFERENCES

Articles from Inflammatory Bowel Diseases are provided here courtesy of Oxford University Press
Full text links
Read article at publisher's site: https://doi.org/10.1093/ibd/izab179
Read article for free, from open access legal sources, via Unpaywall:
https://academic.oup.com/ibdjournal/article-pdf/27/Supplement_2/S17/44160776/izab179.pdf
Citations & impact
Impact metrics
Citations of article over time
Alternative metrics

Discover the attention surrounding your research
https://www.altmetric.com/details/117088953
Article citations
Retinoid orphan receptor gamma t (rorγt) promotes inflammatory eosinophilia but is dispensable for innate immune-mediated colitis.
PLoS One, 19(3):e0300892, 21 Mar 2024
Cited by: 0 articles | PMID: 38512959 | PMCID: PMC10956760
Conference Report: The FutuRE oF MinimalLy InvasivE GI and Capsule DiagnosTics (REFLECT) Nyborg, Denmark, October 2023.
Diagnostics (Basel), 14(5):458, 20 Feb 2024
Cited by: 0 articles | PMID: 38472931 | PMCID: PMC10931071
Smart capsules for sensing and sampling the gut: status, challenges and prospects.
Gut, 73(1):186-202, 07 Dec 2023
Cited by: 7 articles | PMID: 37734912 | PMCID: PMC10715516
Review Free full text in Europe PMC
Research-Based Product Innovation to Address Critical Unmet Needs of Patients with Inflammatory Bowel Diseases.
Inflamm Bowel Dis, 27(suppl 2):S1-S16, 01 Nov 2021
Cited by: 2 articles | PMID: 34791292 | PMCID: PMC8922161
Similar Articles
To arrive at the top five similar articles we use a word-weighted algorithm to compare words from the Title and Abstract of each citation.
Soluble Blood Markers of Mucosal Healing in Inflammatory Bowel Disease: The Future of Noninvasive Monitoring.
Inflamm Bowel Dis, 26(6):961-969, 01 May 2020
Cited by: 9 articles | PMID: 31587036
Review
Rapid fecal calprotectin level assessment and the SIBDQ score can accurately detect active mucosal inflammation in IBD patients in clinical remission: a prospective study.
J Gastrointestin Liver Dis, 23(3):273-278, 01 Sep 2014
Cited by: 16 articles | PMID: 25267955
Persistent Diarrhea in Patients With Crohn's Disease After Mucosal Healing Is Associated With Lower Diversity of the Intestinal Microbiome and Increased Dysbiosis.
Clin Gastroenterol Hepatol, 19(2):296-304.e3, 24 Mar 2020
Cited by: 13 articles | PMID: 32220613 | PMCID: PMC7511440
Development, validation and implementation of an in vitro model for the study of metabolic and immune function in normal and inflamed human colonic epithelium.
Dan Med J, 62(1):B4973, 01 Jan 2015
Cited by: 12 articles | PMID: 25557335
Review
Funding
Funders who supported this work.
Crohn's & Colitis Foundation
Crohn’s & Colitis Foundation
NIDDK NIH HHS (1)
Grant ID: K23 DK129835