Abstract
Free full text
Schisandrin B ameliorates non‐alcoholic liver disease through anti‐inflammation activation in diabetic mice
Associated Data
Abstract
Type 2 diabetes mellitus (T2DM) is a metabolic risk factor associated with non‐alcoholic liver disease (NAFLD). Schisandrin B (Sch B) is a promising agent for NAFLD. However, the actions of Sch B on diabetes‐associated NAFLD and the underlying mechanisms are not characterized. This study aimed to assess whether Sch B has beneficial effects on T2DM‐associated NAFLD. Sch B (50mg/kg, gavage) was administrated to C57BL/KSJ db/db mice for 2
weeks. Body weight, liver weight, blood glucose, and insulin resistance were measured. Serum lipid level and liver function were detected using the biochemistry analyzer. Quantitative Real‐Time PCR assay was used to evaluate mRNA levers of lipid metabolism genes. Terminal‐deoxynucleoitidyl Transferase Mediated Nick End Labeling (TUNEL) staining was performed to measure apoptosis in the liver. Pathological analysis and immunohistochemistry assessment were used to analyze hepatic steatosis and inflammatory infiltration. Sch B supplementation significantly decrease body weight, related liver weight, blood glucose, and serum insulin, and improved insulin resistance in db/db mice. Sch B obviously corrected NAFLD phenotypes including lipid deposition, steatohepatitis, and high levels of hepatic enzymes and serum lipid. In addition, mRNA levels of Sterol response element‐bind protein 1c (SREBP‐1c), fatty acid synthetase (Fasn), and acetyl‐CoA carboxylase (ACC) were markedly downregulated by Sch B treatment. TUNEL‐positive cells were also decreased by Sch B. Furthermore, Sch B inhibited the Kupffer cells, IL‐1β, and TNF‐α infiltration to the liver. Sch B ameliorated insulin resistance and lipid accumulation under high glucose conditions, which was partly associated with its inhibition of apoptosis and anti‐inflammatory actions.
1. INTRODUCTION
Non‐alcoholic fatty liver disease (NAFLD) corresponds to the presence of hepatic steatosis and inflammatory infiltration in the absence of heavy alcohol consumption. It is commonly believed that NAFLD is strongly correlated with clinic features of metabolic syndrome (Filippatos et al., 2021; Hazer et al., 2020). The prevalence of NAFLD dramatically rising that is in the range of 11.5%–46% in the general population, indicative of the magnitude of the medical and the economic burden globally (Antunes et al., 2021). Nearly 80% patients with type 2 diabetes mellitus (DM), obesity, insulin resistance, or hyperlipidemia have NAFLD (Filippatos et al., 2021; Hazer et al., 2020; Leoni et al., 2018). Type 2 DM, characterized by insulin resistance, promotes lipolysis in peripheral tissue and results in release of free fatty acids which could be store in the liver. This is one of the critical causes for development of hepatic steatosis (Shields et al., 2009). Current data show that DM is an independent risk factor for hepatocellular carcinoma (Streba et al., 2015). Therefore, early drug intervention treatment of NAFLD patient with concerning complications is important for reducing morbidity.
Traditional Chinese medicinal herbs have a long history in using of safeguarding health that may offer a potential manner for prevention and cure of NAFLD. Schisandrin B (Sch B), an active dibenzooctadiene lignan, is abundant in the traditional Chinese medicine of fruit of Schisandra chinensis (FSC) (Nasser et al., 2020). It has been well recognized that Sch B presents various of biological functions including antihyperlipidemic, antioxidant, anti‐endoplasmic reticulum stress, as well as anti‐inflammation (Chu et al., 2011; Zhang et al., 2019). It has been reported that Sch B decreases apoptotic neurons and inflammatory signaling molecules in ischemic stroke rats (Fan et al., 2020). Recently, the protective effect of Sch B on metabolic diseases has also been largely demonstrated both in vitro and in vivo (Chu et al., 2011; Mak & Ko, 1997; Pan et al., 2008). For example, Sch B dose‐dependently inhibits steatosis in L02 hepatocytes induced by free fatty acids (FFAs), in part through the suppression of sterol response element‐bind protein‐1 (SREBP‐1) and adipose differentiation‐related protein (Chu et al., 2011). Paralleling the results showed in vitro study, administrated with Sch B at the dose of 50–200mg/kg/d reduces hepatic total cholesterol (TC) and triglyceride (TG) in cholesterol/bile salt induced hypercholesterolaemia mice model (Pan et al., 2008). Similarly, in streptozotocin‐induced diabetic rats, Sch B protects against carbon tetrachloride‐mediated hepatotoxicity (Mak & Ko, 1997). Although, the role of Sch B in hepatic steatosis has been evaluated, the effective action and specific mechanisms of this lignan underlying T2DM‐related NAFLD are poorly understood.
The pathogenesis of NAFLD has been described based on the “multiple hit” theory, which includes insulin resistance, inflammation, apoptosis, and even genetic factors (Di Sessa et al., 2019). The critical hallmark of NAFLD is chronic inflammation which results from the expression of pro‐inflammatory cytokines stimulated by overload of free fatty acids (FFAs) in the liver (Cusi, 2012). It is well‐established that lipid deposition in the liver can induce IL‐1β and TNF‐α expression, which in turn promotes inflammatory cells infiltration, and activates downstream signaling pathways, thus leading to the development of insulin resistance and NAFLD (Tilg, 2010). Clinic evidence demonstrate that circulating level of TNF‐α is highly related to the degree of liver fibrosis in non‐alcoholic steatohepatitis (NASH) patients (Lesmana et al., 2009). It has been demonstrated that Sch B suppressed the upregulated protein levels of TNF‐α, IL‐1β, MMP‐2, and MMP‐9 in focal ischemic cerebral (Lee et al., 2012). It is consistent with the evidence that Sch B protects against dextran sulfate sodium‐induced inflammatory bowel damage in mice via NF‐κB and MAPK signaling (Liu et al., 2015). Therefore, Sch B may be promising in anti‐inflammatory therapy for NAFLD.
In this study, we used db/db diabetic mice with Sch B supplementation for 2weeks. We planned to explore the role of Sch B on diabetic‐associated NAFLD and inflammatory mechanism in vivo.
2. MATERIALS AND METHODS
2.1. Animals
Male, 10‐week‐old C57BL/KSJ db/m mice and C57BL/KSJ db/db mice were purchased from the Laboratory Animal Center of Hangzhou Medical College (Hangzhou, China). All animals allowed to acclimate for 2weeks before the experiments. The mice were bred and housed in an air‐conditioned environment with 12
h light–dark cycle, and were provided with a standard chow. The animals were free access to food and water in Hangzhou Medical College Animal Core. All studies involving animals were performed in accordance with the National Institutes of Health Guide for the Care and Use of Laboratory Animals, and approved by the Ethics Committee of Laboratory Animal Care and Welfare, Hangzhou Medical College (NO. 20200196).
2.2. Animal model and sampling
Blood samples adopted from the tail of mice were monitored using a commercial glucose monitoring system (MAJOR, Taiwan). Db/db mice, whose blood glucose levels greater than 11mmol/L were considered as diabetic mice. The mice were randomly divided into four groups (n
= 6): db/m mice group (control); db/m
+
Sch B group with 50
mg/kg Sch B (Selleck, Shanghai, China) for subsequent 2
weeks; db/db group; and db/db
+
Sch B group (50
mg/kg, 2
weeks). The dose and period were chosen according to before report with minor modification (Kwan et al., 2015). The Sch B was dissolved with DMSO (DMSO final concentration
<
0.5%), and each mouse was administrated 0.2 ml solution. The db/m group and db/db group was given an equal volume of DMSO solution. It was freshly configurated each time. Body weight and blood glucose were recorded after drug treatment of 2‐week.
At the end of administration, all mice were euthanized with phenobarbital sodium (40mg/kg, i.p.). Blood was collected from mice eyes. Samples were still standing for 1 h at room temperature and for 2 h at 4°C, then centrifuged with 3000×
g at 4°C for 15
min. The supernatant was obtained as serum. The livers were quickly excised, cleaned carefully, blotted dry, then measured the weight of tissues. One piece of livers was stored at −80°C for biochemical assays and Oil Red O staining, and another piece was fixed in 10% buffered formaline for pathological analysis. At each independent experiment, the protocol was performed at least three times.
2.3. Metabolic parameters and inflammatory cytokines determination
The concentrations of serum insulin, IL‐1β and TNF‐α were measured with ELISA kits (Insulin: SHIBAYAGI, Shibukawa, Japan; IL‐1β and TNF‐α: R&D Systems, MN) as previously described (Jiang et al., 2017). In addition, serum alanine aminotransferase (ALT) and aspartate aminotransferase (AST) levels were determined using an automatic biochemistry analyzer (Backman, Miami, FL) according to the manufacturer's instruction. TC and TG both in serum and tissues were quantified with commercial kits (Cayman Chemical, Ann Arbor, MI).
2.4. Glucose tolerance test and insulin tolerance test
GTT and ITT were performed to evaluate the insulin resistance according to previously reported (Zhou et al., 2016). Mice were deprived of chow overnight before GTT assay, and were fasted for 4 h prior to ITT assay. For GTT, mice received intraperitoneal injection of glucose (2 g/kg), and blood was harvested from the tail at 0, 15, 30, 60, and 90min, respectively. For ITT, mice were subjected to intraperitoneal injection of insulin (2 U/kg), and blood were collected at 0, 15, 30, 60, and 90
min, respectively. The values of blood glucose were measured with glucose monitoring system.
2.5. Histological analysis
For the pathology examination, the fixed liver tissues were embedded in paraffin and cut into a thin section (5 μm). Then samples were stained with hematoxylin and eosin (H&E) for histology score. To visualize lipid deposition, the frozen liver section was used to Oil Red O staining. The samples were cut into 5 μm thin sections, and were stained with Oil Red O reagent for 10 min. Images were taken at 200× using a microscope (Leica Microsystems, Wetzlar, Germany).
The severity of steatosis, ballooning, and lobular inflammation in the livers were semiquantitatively analyzed by a blinded histopathologist according to before described method (Kleiner et al., 2005). The analysis of microvesicular steatosis used a scale of 0–3, where 0 referred to less than 5% of steatosis, 1 referred to less than 33% of steatosis, 2 referred to less than 66% of steatosis, 3 referred to more than 66% of steatosis. The evaluation of ballooning used a scale of 0–2: 0 referred to no sign of hepatocyte ballooning, 1 referred to several ballooning cells in the liver, 2 referred to mass ballooning cells. In addition, the evaluation criterion of infiltration of the lobular inflammatory cells was as follow: 0 referred to none of the lobular inflammatory cells, 1 referred to 0–2 inflammatory cells, 2 referred to 2–4 inflammatory cells, and 3 referred to more than 4 inflammatory cells. The sum of the above scores were defined as a NAFLD activity score, which 0–2 was identified as no symptom for NASH, 3–4 was identified as uncertain, more than 5 was identified as likely NASH.
2.6. Isolation of RNA and quantitative real‐time PCR
Total RNA of isolated hepatic tissues was purified using the RNAiso Plus (Takara, Dalian, China) according to the manufacturer's protocol. The isolated RNA (2 μg) was then reversed‐transcribed to cDNA using the PrimeScript™ RT Reagent Kit (Takara). Quantitative Real‐Time PCR assay was implemented with a SYBR Premix‐Ex Tag Kit (Takara) and an ABI PRISM 7700 Sequence Detection System (Applied Biosystems, Carlsbad). All samples were measured in duplicate. The mRNA level comparison was calculated using the 2–ΔΔCt method.
2.7. Terminal deoxynucleotidyl transferase dUTP nick end labeling (TUNEL)assay
To examine the apoptotic cells in the liver tissues, a TUNEL assay kit (Wuhan Boster Biological Technology, Wuhan, China) was applied. The procedure was performed according to the manufacturer's instructions. Briefly, the slides were incubated with 1% TUNEL reaction mixture for 1 h at room temperature, and were rinsed three times with phosphate buffer saline (PBS). Images were taken at high magnification (200×) with a microscope (Leica Microsystems). TUNEL‐positive cells in three randomly areas were quantitated in each slide.
2.8. Immunohistochemical staining
Samples of liver tissues were excised, and fixed in 10% formalin for 24h at room temperature. Liver tissues were embedded in paraffin, cut into 5 μm thin sections, and subsequently deparaffinization, rehydration, antigen retrieval and 5% normal goat serum blocking for 4 h at room temperature. The slides were incubated in specific primary antibodies for 4 h, and followed by PBS washing for three times. Then, slides were incubated with horseradish peroxidase‐conjugated secondary antibodies for 2 h, and visualized using substrate diaminobenzidine. The following primary antibodies were used: CD68 (ab125212, Abcam, 1:800 dilution), F4/80 (LS‐C96373‐100, Lifespan, 1:800 dilution), IL‐1β (SRP8033, Sigma, 1:800 dilution), TNF‐α (ab6671, Abcam, 1:1000 dilution). One sample from each mouse was detected, and three high‐power fields at 200× were analyzed for each slide using a microscope (Leica). The number of positive cells were counted.
2.9. Statistical analyses
All values are expressed as mean±
SEM with six mice each group to ensure their reliability. Statistical analysis was performed using GraphPad Software Prism‐8. Data of two groups were analyzed with Student's t‐test, and three and four groups were analyzed with Newman–Keuls multiple comparison test. In each test, the conventional analysis of variance (F test) was applied to the ranks instead of the original data. The statistical significance was considered as P less than .05.
3. RESULTS
3.1. Improvements of body weight, relative liver weight, and insulin resistance by Sch B treatment in db/db mice
As expected, body weight in db/db mice was significantly increased compared with db/m mice (56.92±
0.38
g vs. 25.48
±
0.29
g, P
<
.05; Figure 1a). Although Sch B administrated did not change the body weight in db/m mice, it significantly reduced the body weight gain in db/db mice (54.17
±
0.53
g vs. 56.92
±
0.38
g, P
<
.05; Figure 1a). Similarly, the percentages of liver weight to body weight were higher in db/db mice than in the db/m mice (4.14
±
0.03% vs. 3.70
±
0.01%, P
<
.05; Figure 1b). Oral administration of Sch B did not alter the relative liver weight in db/m mice, but it significantly decreased this value in db/db mice (4.04
±
0.02% vs. 4.14
±
0.03%, P
<
.05; Figure 1b). Furthermore, db/db diabetic mice displayed greatly high blood glucose and serum insulin level, which were suppressed by Sch B (Blood glucose: 12.92
±
5.88 vs. 16.24
±
6.83
mmol/L, P
<
.05; Figure 1c; Insulin: 6.93
±
0.38 vs. 8.57
±
0.22
ng/ml, P
<
.05; Figure 1d). There were no significant differences of blood glucose and insulin concentrations in db/m mice with or without Sch B treatment (P
>
.05; Figure 1c,d). GTT and ITT assays were applied to further detect insulin resistance. As show in Figure 1e, db/db diabetic mice had a higher blood glucose level in GTT assay, while the blood glucose was partly inhibited by Sch B administration (P
<
.05). Calculation of area under curve (AUC) verified this result (P
<
.05; Figure 1f). In line with these results, Sch B administration also improved the injury of insulin sensitivity in db/db mice (P
<
.05; Figure 1g,h). There were no significant differences of both GTT and ITT assays in db/m mice with or without Sch B treatment (P
>
.05; Figure 1e–h).
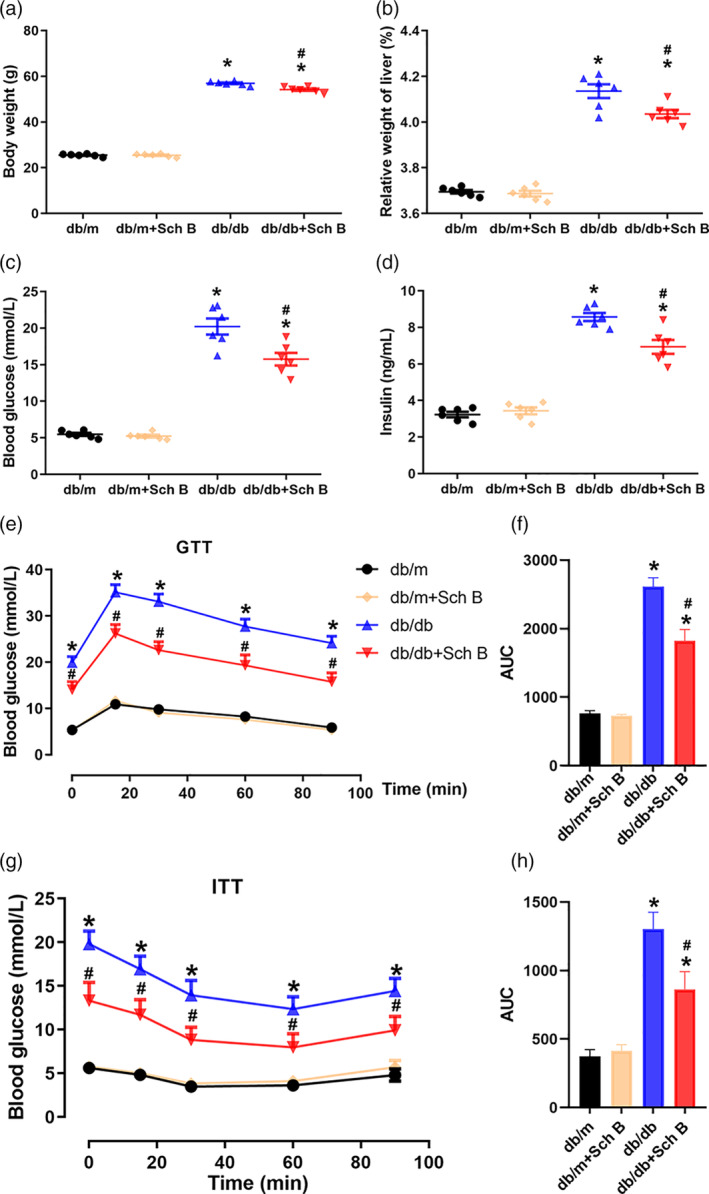
Improvements of body weight, relative liver weight, and insulin resistance by Sch B treatment in db/db mice. Ten‐week‐old db/m mice and db/db mice were administrated with Sch B (50mg/kg/day) or normal saline by gavage. The administration period lasted for 2
weeks. Body weight (a), relative weight of liver (b), blood glucose (c), and serum insulin (d) were detected after Sch B treatment. GTT assay (e) and ITT (g) assay were used to measure blood glucose at different time‐points. (f, h) The corresponding area under curve (AUC) was calculated. The data are presented as mean
±
SEM, n
= 6, *P
<
.05 versus db/m, #
P
<
.05 versus db/db
3.2. Effects of Sch B treatment on hepatic pathology and hepatic function in db/db mice
HE staining and histology scores were used to analyze the steatosis and steatohepatitis in diabetic mice. As illustrated in Figure 2a–d, lipid droplets extensively accumulated in the liver tissues of db/db mice, but that Sch B decreased this accumulation. To further evaluate the histological injury in the liver, the scores were marked by a blinded histopathologist. Steatosis, hepatocyte ballooning, and inflammation scores were remarkably increased in db/db mice (P<
.05; Figure 2e–g), consequently, NAFLD activity score was elevated compared with db/m mice (P
<
.05; Figure 2h). Sch B treatment reversed these abnormalities in db/db mice (P
<
.05). In addition, db/db diabetic mice displayed obvious liver damage reflected by elevations of serum ALT and AST (ALT: 74.5
±
2.8 U/L vs. 24.2
±
2.1 U/L, P
<
.05; Figure 2i; AST: 157.3
±
4.6 U/L vs. 55.0
±
3.3 U/L, P
<
.05; Figure 2j), which were restored by Sch B treatment (ALT: 54.3
±
2.2 U/L vs. 74.5
±
2.8 U/L, P
<
.05; Figure 2i; AST: 104.5
±
3.5 U/L vs. 157.3
±
4.6 U/L, P
<
.05; Figure 2j). There were no significant differences of both scores of hepatic pathology and hepatic function in db/m mice with or without Sch B administration (P
>
.05; Figure 2e–j).
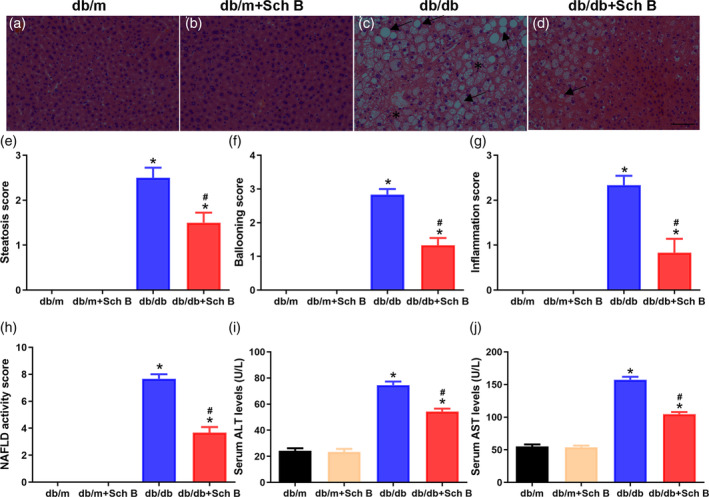
Improvements of hepatic pathology and hepatic function by Sch B treatment in db/db mice. (a–d) representative images of hepatic H&E staining (magnification, 200×). The black arrow pointed to the lipid droplet and asterisk was used to draw inflammatory cells. Scale bar, 100μm. Histopathological analysis of steatosis (e), hepatocyte ballooning (f), lobular inflammation (g), and the total NAFLD activity (h). Changes of serum ALT (i), and AST (j) were detected. The data are presented as mean
±
SEM, n
= 6, *P
<
.05 versus db/m, #
P
<
.05 versus db/db
3.3. Effects of Sch B treatment on hepatic steatosis in db/db mice
Oil Red O staining showed amount of lipid deposited in the liver in diabetic mice compared with db/m mice Figure 3a–d). Oral administration of Sch B was largely attenuated hepatic steatosis in db/db mice. Furthermore, serum and liver tissues levels of TC and TG were significantly increased in db/db mice compared with db/m mice (TC in serum: 115.8±
2.1 vs. 56.8
±
1.6 mg/dL, P
<
.05; Figure 3e; TG in serum: 116.8
±
2.5 vs. 75.5
±
2.3 mg/dL, P
<
.05; Figure 3f; TC in liver: 6.23
±
0.10 vs. 2.53
±
0.09
mg/g, P
<
.05; Figure 3g; TG in liver: 9.06
±
0.21 vs. 5.09
±
0.13
mg/g, P
<
.05; Figure 3h), which were effectively reduced by Sch B in db/db mice (TC in serum: 75.6
±
2.0 vs. 115.8
±
2.1 mg/dL, P
<
.05; Figure 3e; TG in serum: 89.1
±
2.0 vs. 116.8
±
2.5 mg/dL, P
<
.05; Figure 3f; TC in liver: 3.95
±
0.06 vs. 6.23
±
0.10 mg/g, P
<
.05; Figure 3g; TG in liver: 6.42
±
0.18 vs. 9.06
±
0.21
mg/g, P
<
.05; Figure 3h). These results were supported by an amelioration of mRNA levels of Sterol response element‐bind protein 1c (SREBP‐1c), fatty acid synthetase (Fasn), and acetyl‐CoA carboxylase (ACC) in db/db mice receiving Sch B (SREBP‐1c: 1.47
±
0.02 vs. 3.02
±
0.04, P
<
.05; Fasn: 1.41
±
0.02 vs. 1.61
±
0.03, P
<
.05; ACC: 1.64
±
0.04 vs. 2.27
±
0.03, P
<
.05; Figure 3i). There were no significant differences on hepatic steatosis, serum lipid concentrations, and mRNA levels of SREBP‐1c, Fasn, and ACC between db/m mice with Sch B group and db/m mice without Sch B group (P
>
.05).
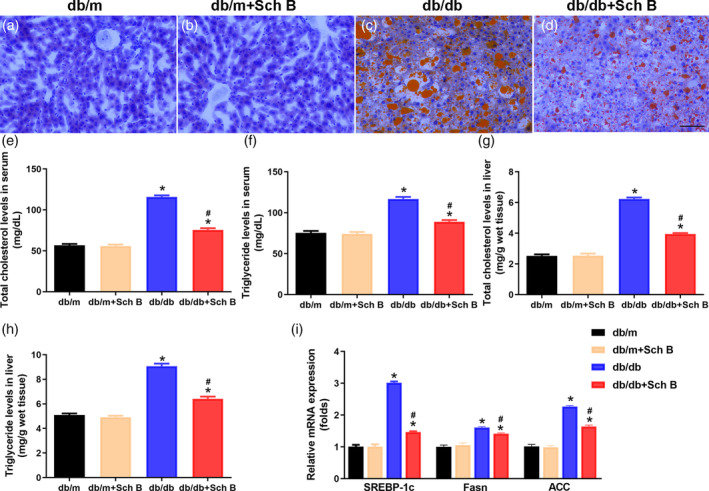
Attenuation of hepatic steatosis by Sch B treatment in db/db mice. (a–d) Representative images of oil red O staining (magnification, 200×). Scale bar, 100μm. Levels of total cholesterol in serum (e), triglyceride in serum (f), total cholesterol in livers (g), and triglyceride in livers (h) were measured. The data are presented as mean
±
SEM, n
= 6, *P
<
.05 versus db/m, #
P
<
.05 versus db/db
3.4. Sch B suppresses hepatocellular apoptosis in db/db mice
To further evaluate the influence of Sch B on liver injury in diabetic mice, TUNEL staining was used to detect the level of hepatocellular apoptosis. In db/db mice group, the liver tissues appeared significant apoptosis, reflected by more TUNEL‐positive nuclei than db/m mice group (P<
.05; Figure 4a,b,d). As expected, db/db mice with Sch B group obviously suppressed TUNEL‐positive cells when compared to db/db mice group (P
<
.05; Figure 4b–d).
3.5. Sch B alleviates inflammatory infiltration in liver tissues in db/db mice
Inflammation is involved in diabetic and lipid metabolism disorders (Kleiner et al., 2005), therefore, inflammatory infiltration in liver tissues were measured. Immunohistochemical staining for CD68 and F4/80 showed that accumulated Kupffer cells were markedly increased in liver tissues of db/db mice compared with db/m mice, while Sch B treatment significantly reduced the number of Kupffer cells in liver in db/db mice (P<
.05; Figure 5a–f,m). In line with these results, immunohistochemical staining for IL‐1β and TNF‐α indicated that the levels of inflammatory cytokines were obviously upregulated in db/db mice compared with db/m mice (P
<
.05; Figure 5g–m). In addition, concentrations of IL‐1β and TNF‐α were also significantly increased in the liver tissues of db/db mice (P
<
.05; Figure 5n). There was also a significant downregulation of IL‐1β and TNF‐α expression in liver tissues by Sch B treatment (P
<
.05; Figure 5g–n).
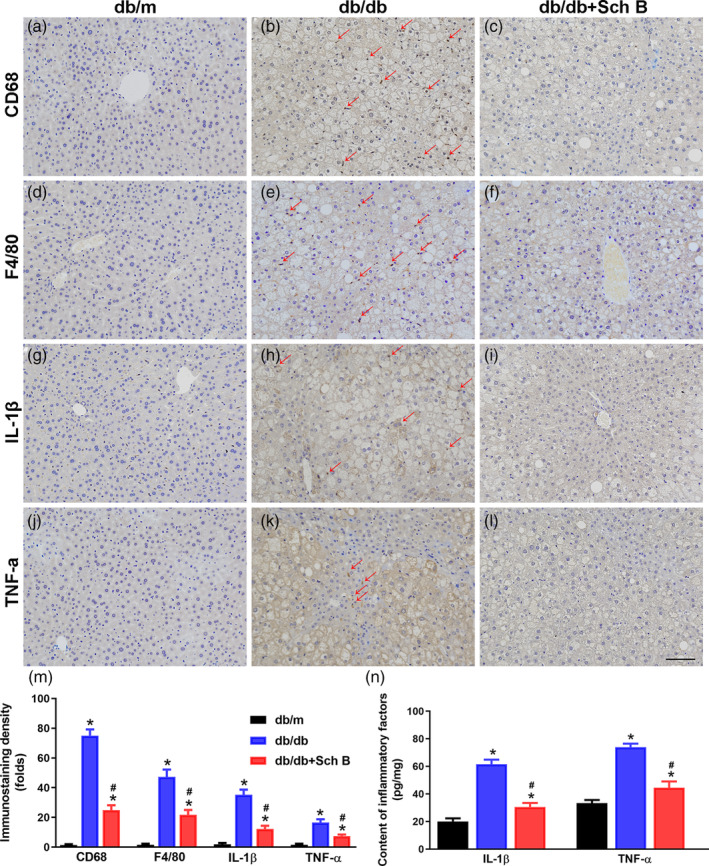
Alleviation of inflammatory infiltration by Sch B treatment in the liver tissues of db/db mice. Liver sections were stained with CD68 (a‐c), F4/80 (d‐f), IL‐1β (g‐i), or TNF‐α (j‐l) to monitor inflammatory infiltration (magnification, 200×). Scale bar, 100μm. (m) the bar graph showed that Sch B treatment significantly downregulated the infiltration of inflammatory cells and inflammatory cytokines in the liver tissues. (n) Concentrations of IL‐1β and TNF‐α in the liver tissues were measured using ELISA kits. The data are presented as mean
±
SEM, n
= 6, *P
<
.05 versus db/m, #
P
<
.05 versus db/db
4. DISCUSSION
The principal findings arose from the current study. First, the supplementation of Sch B significantly lowered blood glucose and improved insulin resistance in diabetic mice. Second, Sch B remarkedly ameliorated steatosis, steatohepatitis and apoptosis in the liver in db/db mice. Furthermore, oral supplementation of Sch B downregulated Kupffer cells infiltrating and IL‐1β and TNF‐α expressions in the liver.
It is well‐established that NAFLD is the manifestation of metabolic syndrome in the liver, therefore, it is closely associated with obesity, dyslipidemia, insulin resistance, and T2DM (Filippatos et al., 2021; Hazer, 2020). The insulin resistance contributes to the progress of NAFLD through promoting the storage of FFAs in the liver (Shields et al., 2009). It has been reported that liver stellate cells incubated with high glucose or insulin overstimulated indirectly caused hepatic fibrosis (Paradis, 2001). NAFLD prevalence is dramatically increased in insulin resistance population (Brunt, 2005). So far, lifestyle modification is regarded as the first‐line interventions for NAFLD (Younossi et al., 2018). There is limited in terms of drug therapeutic options for diabetes mellitus‐related NAFLD.
The Sch B is deemed to be beneficial for health, partly due to a broad spectrum of biological and pharmacological activities (Nasser et al., 2020). Recently, this compound has been identified to directly bind to peroxisome proliferator activator receptor gamma (PPARG) and protect against hepatic fibrosis through a network analysis (Xing et al., 2018). Likewise, the hepatoprotection afforded by Sch B is considered to be attributed to regulation of the hepatic (glutathione) GSH antioxidant enzymes (Ip et al., 1995). Moreover, Sch B alleviated hyperglycemia‐induced renal injury, such as fibrosis and renal cell apoptosis in type 1 diabetic rats (Mou et al., 2019). These results suggested that Sch B play a critical role in protect metabolic homeostasis. However, it is not known whether Sch B is involved in hepatoprotective effects in T2DM‐associated NAFLD.
The db/db leptin receptor mutant mice are recognized as T2DM mice model (Han, Deng, et al., 2017). These mice gain weight and develop to insulin resistance (Han, Tao, et al., 2017). Several studies have shown that db/db mice also develop to NAFLD presenting obviously hepatic steatosis and hepatic dysfunction (Su et al., 2016). Thus, db/db mice were applied for studying the pathophysiological mechanisms underlying T2DM‐associated NAFLD. In the present study, we found that Sch B possessed the ability to lower blood glucose, and improve insulin resistance in db/db mice. More importantly, Sch B alleviated lipid accumulation, steatohepatitis, and apoptosis, hallmarks of NAFLD, in db/db diabetic mice.
SREBP‐1c is a master regulator for fatty acid biosynthesis. Once it activated can promote the levels of Fasn and ACC, which also involved in de novo lipid synthase. Suppression of SREBP‐1c obviously reversed hepatic steatosis in obese mice (Sekiya et al., 2003). Besides, both mRNA and protein levels of SREBP‐1c and Fasn were upregulated in the liver of type 1 diabetes mellitus rats (Wang et al., 2013). In line with these results, we found that Sch B weakened mRNA expressions of SREBP‐1c, Fasn, and ACC in the liver tissues of db/db mice. This result suggests that the effects of Sch B on hepatic steatosis under diabetic conditions may partly through inhibition of these lipid metabolism‐related genes.
Growing evidence suggests that inflammation seems to be the most critical mechanism related to the pathogenesis of NAFLD (Katsarou et al., 2020; Lefere & Tacke, 2019). Excessive lipid accumulation in the liver is closely association with hepatocellular injury and Kupffer activation that triggers the inflammatory activation which further deteriorating liver disease (Katsarou et al., 2020). Several pro‐inflammatory adipokines such as IL‐1β and TNF‐α are reported to participate in all stages of NAFLD development (Tan et al., 2016). In addition, the activation of inflammatory response contributes to insulin resistance and mitochondrial dysfunction (Zangara et al., 2021). Interesting, the anti‐inflammation property of Sch B is related to protect against diabetic nephropathy (Mou et al., 2019), sepsis (Xu et al., 2018), and hepatocyte damage (Liu et al., 2021). It has been reported that Sch B plays a protective effect in a lipopolysaccharide‐induced sepsis model through suppressing toll‐like receptor 4 (TLR4)/NF‐κB/myeloid pathway signaling in rats (Xu et al., 2018). One mechanism of Sch B against liver damage is downregulation of p65 phosphorylation and inhibition of Kupffer cells in bilirubin metabolism disorder mice (Liu et al., 2021). In the current study, our results showed that Kupffer cell were recruited to the liver, and pro‐inflammatory cytokines were produced under diabetic condition. The supplementation of Sch B reduced Kupffer cell accumulation in the liver together with downregulation the infiltration of IL‐1β and TNF‐α in db/db mice. These data indicate that Sch B protects against NAFLD in db/db mice associating with suppression of the inflammatory response.
5. CONCLUSION
In summary, this study investigated the pharmacological effects of Sch B in type 2 diabetic mice, revealing that inhibition of liver inflammation by Sch B contributed to the amelioration in insulin resistance and lipid accumulation. Therefore, the inhibition of apoptosis and anti‐inflammation activity of Sch B may be a promising target for prevention of T2DM‐related NAFLD.
CONFLICT OF INTEREST
The authors declare no conflicts of interests.
ACKNOWLEDGMENTS
This study was supported by the Science and Technology Plan Guiding Project of Hangzhou City (Grant No. 20191231Y163), the Science and Technology Plan Project of Xiaoshan District (Grants No. 2019225), and the Science and Technology Plan Guiding Project of Xiaoshan District (Grant No. 2020313). The funders had no role in study design, data collection and analysis, decision to publish, or preparation of the manuscript.
Notes
Ma R., Zhan Y., Zhang Y., Wu L., Wang X., & Guo M. (2022). Schisandrin B ameliorates non‐alcoholic liver disease through anti‐inflammation activation in diabetic mice. Drug Development Research, 83, 735–744. 10.1002/ddr.21905 [Europe PMC free article] [Abstract] [CrossRef] [Google Scholar]
Ruojia Ma and Yike Zhan contributed equally to this study.
Funding information Science and Technology Plan Guiding Project of Hangzhou City, Grant/Award Number: 20191231Y163; Science and Technology Plan Guiding Project of Xiaoshan District, Grant/Award Number: 2020313; Science and Technology Plan Project of Xiaoshan District, Grant/Award Number: 2019225
DATA AVAILABILITY STATEMENT
The data that support the findings of this study are available on request from the corresponding author. The data are not publicly available due to privacy or ethical restrictions.
REFERENCES
- Antunes C., Azadfard M., Hoilat G. J., & Gupta M. (2021). Fatty liver. StatPearls Publishing. [Google Scholar]
- Brunt E. M. (2005). Nonalcoholic steatohepatitis: Pathologic features and differential diagnosis. Seminars in Diagnostic Pathology, 22(4), 330–338. [Abstract] [Google Scholar]
- Chu J., Wang H., Ye Y., Chan P. K., Pan S. Y., Fong W. F., & Yu Z. L. (2011). Inhibitory effect of schisandrin B on free fatty acid‐induced steatosis in L‐02 cells. World Journal of Gastroenterology, 17(19), 2379. [Europe PMC free article] [Abstract] [Google Scholar]
- Cusi K. (2012). Role of obesity and lipotoxicity in the development of nonalcoholic steatohepatitis: Pathophysiology and clinical implications. Gastroenterology, 142(4), 711–725. [Abstract] [Google Scholar]
- Di Sessa A., Cirillo G., Guarino S., Marzuillo P., & Miraglia Del Giudice E. (2019). Pediatric non‐alcoholic fatty liver disease: Current perspectives on diagnosis and management. Pediatric Health, Medicine and Therapeutics, 10, 89–97. [Europe PMC free article] [Abstract] [Google Scholar]
- Fan X. J., Elkin K., Shi Y. W., Zhang Z. H., Cheng Y. Q., Gu J. X., & Ji X. M. (2020). Schisandrin B improves cerebral ischemia and reduces reperfusion injury in rats through TLR4/NF‐κB signaling pathway inhibition. Neurological Research, 42(8), 693–702. [Abstract] [Google Scholar]
- Filippatos T. D., Alexakis K., Mavrikaki V., & Mikhailidis D. P. (2021). Nonalcoholic fatty pancreas disease: Role in metabolic syndrome, “Prediabetes,” diabetes and atherosclerosis. Digestive Diseases and Sciences. [Epub ahead of print]. [Abstract] [Google Scholar]
- Han X., Deng Y., Yu J., Sun Y., Ren G., Cai J., & Jiang G. (2017). Acarbose accelerates wound healing via Akt/eNOS signaling in db/db mice. Oxidative Medicine and Cellular Longevity, 2017, 1–11. [Europe PMC free article] [Abstract] [Google Scholar]
- Han X., Tao Y., Deng Y., Yu J., Sun Y., & Jiang G. (2017). Metformin accelerates wound healing in type 2 diabetic db/db mice. Molecular Medicine Reports, 16(6), 8691–8698. [Europe PMC free article] [Abstract] [Google Scholar]
- Hazer I., Hilmi O. K., Murat Y., & Zeynep E. (2020). The association of hypolipidemia and hyperlipidemia with non‐alcoholic fatty liver disease in obese children. Turkish Archives of Pediatrics, 55(3), 263–269. [Europe PMC free article] [Abstract] [Google Scholar]
- Ip S., Poon M., Wu S., Che C., Ng K., Kong Y., & Ko K. (1995). Effect of Schisandrin B on hepatic glutathione antioxidant system in mice: Protection against carbon tetrachloride toxicity. Planta Medica, 61(5), 398–401. [Abstract] [Google Scholar]
- Jiang G., Han X., Tao Y., Deng Y., Yu J., Cai J., & Sun Y. (2017). Metformin ameliorates insulitis in STZ‐induced diabetic mice. PeerJ, 5, 155. [Europe PMC free article] [Abstract] [Google Scholar]
- Katsarou A., Moustakas I. I., Pyrina I., Lembessis P., Koutsilieris M., & Chatzigeorgiou A. (2020). Metabolic inflammation as an instigator of fibrosis during non‐alcoholic fatty liver disease. World Journal of Gastroenterology, 26(17), 1993–2011. [Europe PMC free article] [Abstract] [Google Scholar]
- Kleiner D. E., Brunt E. M., Van Natta M., Behling C., Contos M. J., Cummings O. W., & Nonalcoholic Steatohepatitis Clinical Research Network . (2005). Design and validation of a histological scoring system for nonalcoholic fatty liver disease. Hepatology, 41(6), 1313–1321. [Abstract] [Google Scholar]
- Kwan H. Y., Niu X., Dai W., Tong T., Chao X., Su T., & Yu Z. L. (2015). Lipidomic‐based investigation into the regulatory effect of Schisandrin B on palmitic acid level in non‐alcoholic steatotic livers. Scientific Reports, 5, 9114. [Europe PMC free article] [Abstract] [Google Scholar]
- Lee T. H., Jung C. H., & Lee D. (2012). Neuroprotective effects of Schisandrin B against transient focal cerebral ischemia in Sprague–Dawley rats. Food and Chemical Toxicology, 50(12), 4239–4245. [Abstract] [Google Scholar]
- Lefere S., & Tacke F. (2019). Macrophages in obesity and non‐alcoholic fatty liver disease: Crosstalk with metabolism. JHEP Reports, 1(1), 30–43. [Europe PMC free article] [Abstract] [Google Scholar]
- Leoni S., Tovoli F., Napoli L., Serio I., Ferri S., & Bolondi L. (2018). Current guidelines for the management of non‐alcoholic fatty liver disease: A systematic review with comparative analysis. World Journal of Gastroenterology, 24(30), 3361–3373. [Europe PMC free article] [Abstract] [Google Scholar]
- Lesmana C. R. A., Hasan I., Budihusodo U., Gani R. A., Krisnuhoni E., Akbar N., & Lesmana L. A. (2009). Diagnostic value of a group of biochemical markers of liver fibrosis in patients with non‐alcoholic steatohepatitis. Journal of Digestive Diseases, 10(3), 201–206. [Abstract] [Google Scholar]
- Liu D., Yu Q., Li Z., Zhang L., Hu M., Wang C., & Liu Z. (2021). UGT1A1 dysfunction increases liver burden and aggravates hepatocyte damage caused by long‐term bilirubin metabolism disorder. Biochemical Pharmacology, 190, 592. [Abstract] [Google Scholar]
- Liu W., Liu Y., Wang Z., Yu T., Lu Q., & Chen H. (2015). Suppression of MAPK and NF‐kappa B pathways by schisandrin B contributes to attenuation of DSS‐induced mice model of inflammatory bowel disease. Pharmazie, 70(9), 598–603. [Abstract] [Google Scholar]
- Mak D. H., & Ko K. M. (1997). Alterations in susceptibility to carbon tetrachloride toxicity and hepatic antioxidant/detoxification system in streptozotocin‐induced short‐term diabetic rats: Effects of insulin and Schisandrin B treatment. Molecular and Cellular Biochemistry, 175(1–2), 225. [Abstract] [Google Scholar]
- Mou Z., Feng Z., Xu Z., Zhuang F., Zheng X., Li X., & Liang G. (2019). Schisandrin B alleviates diabetic nephropathy through suppressing excessive inflammation and oxidative stress. Biochemical and Biophysical Research Communications, 508(1), 243–249. [Abstract] [Google Scholar]
- Nasser M. I., Zhu S., Chen C., Zhao M., Huang H., & Zhu P. (2020). A comprehensive review on Schisandrin B and its biological properties. Oxidative Medicine and Cellular Longevity, 2020, 1–13. [Europe PMC free article] [Abstract] [Google Scholar]
- Pan S., Dong H., Zhao X., Xiang C., Fang H., Fong W., & Ko K. (2008). Schisandrin B from Schisandra chinensis reduces hepatic lipid contents in hypercholesterolaemic mice. Journal of Pharmacy and Pharmacology, 60(3), 399–403. [Abstract] [Google Scholar]
- Paradis V. (2001). High glucose and hyperinsulinemia stimulate connective tissue growth factor expression: A potential mechanism involved in progression to fibrosis in nonalcoholic steatohepatitis. Hepatology, 34(4), 738–744. [Abstract] [Google Scholar]
- Sekiya M., Yahagi N., Matsuzaka T., Najima Y., Nakakuki M., Nagai R., & Shimano H. (2003). Polyunsaturated fatty acids ameliorate hepatic steatosis in obese mice by SREBP‐1 suppression. Hepatology, 38(6), 1529–1539. [Abstract] [Google Scholar]
- Shields W. W., Thompson K. E., Grice G. A., Harrison S. A., & Coyle W. J. (2009). The effect of metformin and standard therapy versus standard therapy alone in nondiabetic patients with insulin resistance and nonalcoholic steatohepatitis (NASH): A pilot trial. Therapeutic Advances in Gastroenterology, 2(3), 157–163. [Europe PMC free article] [Abstract] [Google Scholar]
- Streba L. A. M., Vere C. C., Rogoveanu I., & Streba C. T. (2015). Nonalcoholic fatty liver disease, metabolic risk factors, and hepatocellular carcinoma: An open question. World Journal of Gastroenterology, 21(14), 4103. [Europe PMC free article] [Abstract] [Google Scholar]
- Su M., He Y., Li Q., & Zhu B. (2016). Efficacy of acetylshikonin in preventing obesity and hepatic steatosis in db/db mice. Molecules, 21(8), 976. [Europe PMC free article] [Abstract] [Google Scholar]
- Tan Q., Hu J., Yu X., Guan W., Lu H., Yu Y., & Tang Z. (2016). The role of IL‐1 family members and Kupffer cells in liver regeneration. BioMed Research International, 2016, 1–6. [Europe PMC free article] [Abstract] [Google Scholar]
- Tilg H. (2010). The role of cytokines in non‐alcoholic fatty liver disease. Digestive Diseases, 28(1), 179–185. [Abstract] [Google Scholar]
- Wang W., Wang C., Ding X., Pan Y., Gu T., Wang M., & Kong L. D. (2013). Quercetin and allopurinol reduce liver thioredoxin‐interacting protein to alleviate inflammation and lipid accumulation in diabetic rats. British Journal of Pharmacology, 169(6), 1352–1371. [Europe PMC free article] [Abstract] [Google Scholar]
- Xing X., Chen S., Li L., Cao Y., Chen L., Wang X., & Zhu Z. (2018). The active components of Fuzheng Huayu formula and their potential mechanism of action in inhibiting the hepatic stellate cells viability‐a network pharmacology and transcriptomics approach. Frontiers in Pharmacology, 9, 525. [Europe PMC free article] [Abstract] [Google Scholar]
- Xu J., Lu C., Liu Z., Zhang P., Guo H., & Wang T. (2018). Schizandrin B protects LPS‐induced sepsis via TLR4/NF‐κB/MyD88 signaling pathway. American Journal of Translational Research, 10(4), 1155–1163. [Europe PMC free article] [Abstract] [Google Scholar]
- Younossi Z., Anstee Q. M., Marietti M., Hardy T., Henry L., Eslam M., & Bugianesi E. (2018). Global burden of NAFLD and NASH: Trends, predictions, risk factors and prevention. Nature Reviews Gastroenterology & Hepatology, 15(1), 11–20. [Abstract] [Google Scholar]
- Zangara M. T., Johnston I., Johnson E. E., & McDonald C. (2021). Mediators of metabolism: An unconventional role for NOD1 and NOD2. International Journal of Molecular Sciences, 22(3), 1156. [Europe PMC free article] [Abstract] [Google Scholar]
- Zhang H., Chen Q., Dahan A., Xue J., Wei L., Tan W., & Zhang G. (2019). Transcriptomic analyses reveal the molecular mechanisms of schisandrin B alleviates CCl4‐induced liver fibrosis in rats by RNA‐sequencing. Chemico‐Biological Interactions, 309, 8675. [Abstract] [Google Scholar]
- Zhou C., Yang X., Hua X., Liu J., Fan M., Li G., & Miao C. Y. (2016). Hepatic NAD (+) deficiency as a therapeutic target for non‐alcoholic fatty liver disease in ageing. British Journal of Pharmacology, 173(15), 2352–2368. [Europe PMC free article] [Abstract] [Google Scholar]
Full text links
Read article at publisher's site: https://doi.org/10.1002/ddr.21905
Read article for free, from open access legal sources, via Unpaywall:
https://onlinelibrary.wiley.com/doi/pdfdirect/10.1002/ddr.21905
Citations & impact
Impact metrics
Citations of article over time
Article citations
The implications of FASN in immune cell biology and related diseases.
Cell Death Dis, 15(1):88, 25 Jan 2024
Cited by: 4 articles | PMID: 38272906 | PMCID: PMC10810964
Review Free full text in Europe PMC
Simultaneous Extraction and Determination of Lignans from Schisandra chinensis (Turcz.) Baill. via Diol-Based Matrix Solid-Phase Dispersion with High-Performance Liquid Chromatography.
Molecules, 28(18):6448, 05 Sep 2023
Cited by: 0 articles | PMID: 37764224 | PMCID: PMC10535609
Network pharmacology-based analysis of Resinacein S against non-alcoholic fatty liver disease by modulating lipid metabolism.
Front Nutr, 10:1076569, 14 Feb 2023
Cited by: 2 articles | PMID: 36866057 | PMCID: PMC9971728
MicroRNAs in kidney injury and disease.
Nat Rev Nephrol, 18(10):643-662, 16 Aug 2022
Cited by: 52 articles | PMID: 35974169
Review
Schisandrin B ameliorates non-alcoholic liver disease through anti-inflammation activation in diabetic mice.
Drug Dev Res, 83(3):735-744, 19 Dec 2021
Cited by: 5 articles | PMID: 34927282 | PMCID: PMC9299884
Similar Articles
To arrive at the top five similar articles we use a word-weighted algorithm to compare words from the Title and Abstract of each citation.
[Effect of Danlou Tablets on alleviating hepatic adipogenesis, inflammatory response, and insulin resistance in db/db mice].
Zhongguo Zhong Yao Za Zhi, 47(12):3320-3327, 01 Jun 2022
Cited by: 0 articles | PMID: 35851126
Melatonin ameliorates hepatic steatosis by inhibiting NLRP3 inflammasome in db/db mice.
Int J Immunopathol Pharmacol, 35:20587384211036819, 01 Jan 2021
Cited by: 7 articles | PMID: 34399601 | PMCID: PMC8375339
Gracilaria chorda subcritical water ameliorates hepatic lipid accumulation and regulates glucose homeostasis in a hepatic steatosis cell model and obese C57BL/6J mice.
J Ethnopharmacol, 320:117395, 11 Nov 2023
Cited by: 2 articles | PMID: 37952731
Schisandrin B: A Double-Edged Sword in Nonalcoholic Fatty Liver Disease.
Oxid Med Cell Longev, 2016:6171658, 26 Oct 2016
Cited by: 20 articles | PMID: 27847552 | PMCID: PMC5101399
Review Free full text in Europe PMC