Abstract
Free full text

SARS‐CoV‐2 infection and lytic reactivation of herpesviruses: A potential threat in the postpandemic era?
Abstract
The outbreak of the severe acute respiratory syndrome coronavirus 2 (SARS‐CoV‐2), which is the causative pathogen for the coronavirus disease 2019 (COVID‐19) pandemic, has greatly stressed our healthcare system. In addition to severe respiratory and systematic symptoms, several comorbidities increase the risk of fatal disease outcomes, including chronic viral infections. Increasing cases of lytic reactivation of human herpesviruses in COVID‐19 patients and vaccinated people have been reported recently. SARS‐CoV2 coinfection, COVID‐19 treatments, and vaccination may aggravate those herpesvirus‐associated diseases by reactivating the viruses in latently infected host cells. In this review, we summarize recent clinical findings and limited mechanistic studies regarding the relationship between SARS‐CoV‐2 and different human herpesviruses that suggest an ongoing potential threat to human health in the postpandemic era.
1. INTRODUCTION
Human herpesviruses are a family of DNA viruses with a relatively large double‐stranded, linear DNA genome and represent a class of the most common infectious pathogens for humans, responsible for many human diseases. 1 Currently, eight herpesviruses members have been identified, including herpes simplex virus types 1 (HSV‐1) and 2 (HSV‐2), Varicella‐zoster virus (VZV or HHV‐3), Epstein–Barr virus (EBV or HHV‐4,), Cytomegalovirus (HCMV or HHV‐5), HHV‐6, HHV‐7, and Kaposi's sarcoma‐associated herpesvirus (KSHV or HHV‐8). 2 Almost 100% of the adult population in the world is infected with at least one herpesviruses during their life. 3 All herpesviruses have two alternative life‐cycle programs after primary infection, latent and lytic phases, which are essential for the maintenance of viral infections and the process of diseases. 4 In general, latency is the primary status in which a virus persists in infected cells with only a limited number of viral proteins being expressed. 5 , 6 However, viral lytic reactivation usually induces high expression of most viral genes, amplification of viral DNA, and release of mature virions. 7 , 8 , 9 Although the mechanisms of viral reactivation in cells are well described, how various stresses such as immunosuppression, inflammatory disorders, and coinfections with other pathogens promote viral reactivation is not completely understood. 10 , 11
According to the data released by Johns Hopkins Coronavirus Resource Center (https://coronavirus.jhu.edu/), SARS‐CoV‐2 has infected over 0.45 billion people and accounts for over six million deaths. Four prominent variants (Alpha, Beta, Delta, and Omicron) have emerged since the beginning of the pandemic. 12 The SARS‐CoV‐2 virus is a single‐strand ribonucleic acid (RNA) virus belonging to the family Coronaviridae and is transmitted from person to person mainly by air. 13 SARS‐CoV‐2 is comprised of four main structural proteins, nucleocapsid protein (N), spike protein (S), envelope glycoprotein (E), and membrane protein (M). 14 Respiratory epithelial cells and alveolar cells of the respiratory system are the initial sites for SARS‐CoV‐2 infection, which is mediated by the interaction of viral S proteins with the host cellular receptor, angiotensin‐converting enzyme 2 (ACE2). 15 Importantly, viral components have been observed in multiple organs and body fluids 16 indicating that SARS‐CoV‐2 infection may affect other pre‐existing diseases.
Increasing data show that SARS‐CoV‐2 infection aggravates pre‐existing comorbidities, including cancer and other infectious diseases. 17 , 18 , 19 For example, a recent review of the global evidence found that the risk of adverse COVID‐19 outcomes is highly associated with several pre‐existing conditions including diabetes, obesity, heart failure, chronic obstructive pulmonary disease dementia, liver cirrhosis, and active cancer. 17 Another study showed that hospitalized COVID‐19 patients with a history of cancer had a worse prognosis and a higher mortality rate (29.4%) than those without cancer (10.2%). 20 Interestingly, SARS‐CoV‐2 or COVID‐19 may act as a direct regulator or an unexpected participator in the lytic reactivation of human herpesviruses. Clinical symptoms related to the lytic reactivation of herpesviruses were significantly more common in patients who were coinfected with SARS‐CoV‐2, especially those with severe COVID‐19 requiring hospitalization in an intensive care unit (ICU), and in COVID‐19 vaccinated people. For example, a retrospective study of 100 patients with severe COVID‐19 found that 56.1% of patients suffered viral reactivation of herpesvirus at 10 days, including 12% for HSV, 58% for EBV, and 19% for HCMV, respectively. 21 Additional studies related to SARS‐CoV‐2 induced reactivation of herpesviruses and potential mechanisms underlying this reactivation are reviewed below.
2. SARS‐CoV‐2 INFECTION AND KSHV
KSHV is the etiologic agent for several malignancies, including Kaposi's sarcoma (KS), primary effusion lymphoma(PEL), and multicentric Castleman disease (MCD), which are mostly seen in immunocompromised patients or organ transplantation receipts.
22
We recently reported that ectopic expression of SARS‐CoV‐2 encoded N or S proteins, two major structural proteins, induced KSHV lytic reactivation and production of infectious virions in KSHV+
lymphoma cells.
23
We further showed that the expression of ACE2, the receptor for SARS‐CoV‐2, was upregulated in AIDS‐KS tissues relative to normal skin tissues.
23
These results suggest the possibility that SARS‐CoV‐2 coinfection can induce KSHV reactivation in COVID‐19 patients. In support of this possibility, a recent case study reported that a female patient with a history of KS without active skin lesions had a recurrence of KS after hospitalization for COVID‐19.
24
Furthermore, transmission electron microscope analysis provided solid evidence for both SARS‐CoV‐2 and KSHV viruses in this patient's specimen. The authors speculated that SARS‐CoV‐2 stimulates a hyper‐inflammatory status which may contribute to KSHV reactivation, replication, and ultimately the recurrence of KS. Similarly, a case of disseminated KS in an immunocompetent patient after hospitalization for COVID‐19 was recently reported.
25
The authors of this study postulated that interleukin‐6 (IL‐6) activity and steroid‐induced immunodeficiency played a major role in KS emergence. Finally, a study of 104 patients in South Africa, where there is a high prevalence of herpesviruses infections, reported that coinfection with KSHV but not EBV might be associated with the severity of clinical outcomes following COVID‐19.
26
In addition to viral coinfection, anti‐COVID‐19 therapies, including azithromycin, nafamostat mesylate, and remdesivir are associated with activating different cellular signaling pathways leading to viral lytic reactivation.
23
,
27
Azithromycin, an antibiotic for treatment of bacterial infections, effectively upregulated mitogen‐activated protein kinases (MAPKs) signaling activities to mediate lytic reactivation. Nafamostat mesylate, a synthetic serine protease inhibitor, regulated the Nuclear factor kappa B pathway to induce lytic reactivation.
23
Remdesivir, an Food and Drug Administration (FDA)‐approved anti‐COVID‐19 drug, significantly activated lytic reactivation of not only KSHV but also EBV, from virus‐associated lymphoma cells.
27
The AMPK and signal transducer and activator of transcription 3 (STAT3) pathways were shown to contribute to remdesivir‐induced KSHV reactivation, while EBV reactivation may be associated with the p38 MAPK and STAT3 pathways.
28
,
29
,
30
,
31
In contrast, molnupiravir, another FDA‐approved anti‐COVID‐19 drug, had minimal effects on KSHV and EBV reactivation, even at cytotoxic concentrations, and significantly decreased the expression of EBV lytic genes from EBV+
lymphoma cells undergoing spontaneous lytic replication.
27
Together, these data strongly suggest that the coinfection with KSHV and SARS‐CoV‐2 and certain drugs used to treat COVID‐19 may facilitate the pathogenesis and progression of diseases associated with each virus (Figure 1).
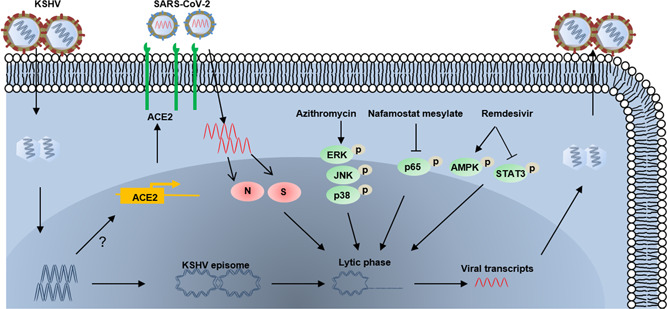
Schematic diagram of potential mechanisms for Kaposi's sarcoma‐associated herpesvirus (KSHV) reactivation by SARS‐CoV‐2 coinfection or anti‐COVID‐19 drugs. The arrows and bars represent the activation and inhibition, respectively. Notably, the mechanisms of KSHV regulation of angiotensin‐converting enzyme 2 expression remain unknown. N, nucleocapsid protein; S, spike protein.
3. SARS‐CoV‐2 INFECTION AND EBV
EBV infection can cause several human malignancies, including Hodgkin's lymphoma, Burkitt's lymphoma, diffuse large B cell lymphoma, natural killer (NK)/T cell lymphomas, and nasopharyngeal carcinoma.
32
Like other herpesviruses, the life cycle of EBV includes latent and lytic phases, which are involved in viral transmission and genome maintenance.
33
,
34
Increasing data support an association between EBV reactivation and SARS‐CoV‐2/COVID‐19. Early in the COVID‐19 pandemic, several reports described cases of EBV and SARS‐CoV‐2 coinfection in patients.
35
,
36
Garcia‐Martinez et al. reported reactivation of EBV with high viral loads in blood and plasma after SARS‐CoV‐2 infection in a 19‐year‐old French female patient with no other relevant clinical history.
37
A large survey of 50419 samples from the United States collected between March and August 2020 showed much higher levels of EBV coinfection (2.13%) in the SARS‐CoV‐2 positive population compared to the coinfection rates of HSV (0.11%) and HCMV (0.07%).
38
A correlation between EBV reactivation and SARS‐CoV‐2 infection was confirmed in a clinical investigation of 67 COVID‐19 patients from Wuhan, China in which it was reported that 55.2% of patients with a history of EBV infection had a positive test for EBV Viral capsid antigen immunoglobulin M, an indicator for EBV reactivation, within 2 weeks of SARS‐CoV‐2 infection.
39
There are several reports that indicate EBV reactivation may contribute to the severity of COVID‐19 infection. For example, COVID‐19 patients with EBV coinfection had a higher risk of fever symptoms and higher levels of C‐reactive protein, aspartate aminotransferase, and corticosteroid use. 39 Another study from China reported that the mortality rate of COVID‐19 patients with EBV reactivation (29.4%, 5/17) was higher than that of patients without EBV infection (8.1%, 9/111). 40 Similarly, a study from France confirmed critically ill patients with COVID‐19 in the ICU were prone to develop lytic reactivation of herpesviruses, including EBV, CMV, and HHV‐6. It was observed that 82% of such patients suffered EBV reactivation, while the incidence rates of CMV and HHV‐6 reactivations were 15% and 22%, respectively. The authors concluded that EBV reactivation occurred early after ICU admission, and was associated with longer ICU length‐of‐stay. 41 It has also been observed 42 that 88.3% of patients (91/103) tested positive for EBV DNA in whole blood samples and that the positive rates of EBV DNA in ICU patients were 95.2% (40/42) higher than 83.6% (51/61) of patients in a sub‐intensive care unit (SICU). Moreover, the levels of viral DNA in ICU patients were higher than those of SICU patients. Interestingly, there was a significant loss of NK and CD8+ T cells in COVID‐19 patients, which paralleled the severity of infection, and this reduction was significantly correlated with the presence of EBV DNA. 42 Another study suggested that EBV reactivation induced by COVID‐19‐related inflammation was likely the main cause of chronic COVID syndrome in COVID‐19 patients; although the underlying mechanisms were not defined. 43
Recent studies have begun to address the mechanisms underlying the interactions between SARS‐CoV‐2 and EBV. Because of the effects of long COVID‐19 44 and EBV reactivation 45 on mitochondrial functions that are important to host immune response, Nunn et al. suggested that EBV reactivation in patients with long COVID might represent a second mitochondrial “hit” that promotes occurrence of longer‐term symptoms. 46 Another recent study reported a similar finding of significant levels of EBV viremia in critically ill COVID‐19 patients (78% of COVID‐19 patients vs. 44.4% of non‐COVID‐19 patients) and associated the high prevalence of EBV reactivation in COVID‐19 patients with the inflammatory factor, IL‐6. 41 In a different mechanistic study, Verma et al. indicated a role for lytic infection of EBV in increasing the susceptibility of SARS‐CoV‐2 infection of human oral epithelium by upregulating ACE2 expression. 47 This involved Zta, an EBV lytic activator, directly acting on methylated promoters to increase ACE2 expression thereby enhancing SARS‐CoV‐2 infectivity (Figure 2). These results suggest that inhibiting EBV replication with antivirals may decrease SARS‐CoV‐2 infectivity. There is also a case report of a 43‐year‐old Chinese female with chronic EBV infection developing hemophagocytic lymphohistiocytosis (HLH) after receiving the inactivated COVID‐19 vaccination. 48 Importantly, there were high levels of EBV virus and IgG antibodies, which are the most common trigger for HLH, in this patient's samples suggesting that the development of HLH in this patient was associated with acute immune‐stimulation of COVID‐19 vaccination under the chronic EBV infection condition. Together, these reports suggest a potential interaction between these two viruses and the impacts on related disease progression in patients.
4. SARS‐CoV‐2 INFECTION AND HSVS
HSVs are categorized into two major types, HSV‐1 and HSV‐2, reflecting oral herpes and genital herpes, respectively. HSV infections are prevalent worldwide, with an estimated 491.5 million people infected with HSV‐2 and over 3752 million with HSV‐1 based on a WHO investigation in 2016.
49
Several clinical reports and other studies indicate the prevalence of SARS‐CoV‐2 and HSV coinfection may promote HSV‐associated diseases in COVID‐19 patients by inducing viral reactivation. A French study involving 38 COVID‐19 patients described Herpesviridae (HSV and/or HCMV) pulmonary reactivations. Of the 38 patients, 18 (47%) presented with at least one viral pulmonary reactivation, nine with HSV reactivation alone, two with CMV reactivation alone, and seven with co‐reactivation.
50
Patients with Herpesviridae reactivation had a significantly longer duration of mechanical ventilation than those without Herpesviridae reactivation. Another cross‐sectional study involving 80 patients with mild‐to‐moderate COVID‐19 without treatment found that 35% (28/80) of patients had one or more HSV infections.
51
Most respondents (78%) reported a single HSV reactivation, 14.29% had two attacks, and 7.14% experienced three attacks. Compared to previous non‐COVID‐19‐related HSV reactivation, the COVID‐19‐related attacks were more severe in 12 (42.85%) patients. Interestingly, 10 (35.71%) patients developed an initial symptomatic HSV attack during COVID‐19 infection, indicating a possible association between COVID‐19 and primary HSV infection or reactivation. In addition, several studies reported potential lytic reactivation of HSV by COVID‐19 treatment or vaccination. In one retrospective cohort study from Italy, although treatment with a recombinant monoclonal antibody to the IL‐6 receptor, tocilizumab, effectively reduced the risk of invasive mechanical ventilation or death, 13% (24/179) of patients treated with this antibody presented new infections relative to 4% (14/365) of patients treated with standard care alone (p<
0.0001).
52
Interestingly, four patients in the tocilizumab group experienced HSV reactivation, of which one died after receiving high‐dose glucocorticoids, while there were none in the standard care group. Another study from the United Kingdom described an 82‐year‐old man with a history of herpes simplex keratitis who presented with recurrence after receiving a COVID‐19 vaccine (AstraZeneca),
53
implying the rare potential for HSV reactivation following the vaccination.
5. SARS‐CoV‐2 INFECTION AND VZV
VZV infection usually causes herpes zoster (shingles) and varicella (chickenpox). 54 This herpesvirus infection is prone to establishing latency in dorsal‐root or cranial‐nerve ganglia. 55 Although there are available vaccines against VZV, some stressors, for example, fever, immunosuppression, and inflammatory may induce its reactivation. 56 It recently has been reported that SARS‐CoV‐2 and its vaccines may represent a trigger for VZV reactivation. Previous clinical observation reported after getting COVID‐19, some patients suffered various cutaneous manifestations, ranging from erythematous rash to chickenpox‐like/urticarial eruption, including herpes varicella zoster‐induced skin diseases. 57 , 58 , 59 Two recent publications reported specific COVID‐19‐associated varicella‐like exanthema, which may be associated with VZV reactivation. 60 , 61 Fernandez‐Nieto et al. also reported 15 cases of COVID‐19 hospitalized patients with typical clinical skin manifestation, including HSV‐associated zoster and VZV‐associated zoster. 62 Elsaie et al. reported two COVID‐19 patients who first presented with herpes varicella‐zoster lesions, suggesting VZV reactivation should be considered an alarming sign for possible COVID‐19, especially during the pandemic. 63 Maldonado et al. reported that a 25‐year‐old woman had itchy vesicular lesions associated with varicella‐zoster infection and a subsequent positive diagnosis of SARS‐CoV‐2. 64 Although this patient had an immunocompetent system, she suffered from a worsening condition even though she was treated with paracetamol and topical calamine powders. Ferreira et al. also presented a clinical case of a 39‐year‐old man with co‐infection of SARS‐CoV‐2 and VZV who suffered the left facial herpes zoster affecting the three divisions of the trigeminal nerve, 65 which is probably related to viral inflammatory responses. Tartari et al. observed four elderly COVID‐19 patients showed a decrease in CD3+/CD8+ elements in circulating blood, providing another possible mechanism of VZV reactivation. 66 These reports together indicate a potential role of SARS‐CoV‐2 co‐infection in VZV reactivation.
Several case reports describe herpes zoster induced by VZV reactivation following vaccination with either inactivated or messenger RNA (mRNA) COVID‐19 vaccines. A cross‐sectional investigation from Spain collected 405 patients with cutaneous reactions after vaccination with the BNT162b2 (Pfizer‐BioNTech; 40.2%), mRNA‐1273 (Moderna; 36.3%) and AZD1222 (AstraZeneca; 23.5%), and found the reactivation of VZV and HSV accounted for 13.8% of reactions.
67
Yalici‐Armagan et al. reported an unusual case of VZV reactivation in a 78‐year‐old man after receiving the inactivated COVID‐19 vaccine.
68
This patient suffered from some severe and painful pimple‐like lesions but no other symptoms such as fever, dyspnea, or cough 5 days after the inactivated COVID‐19 vaccine. Eid et al. presented another case of VZV reactivation in a 79‐year‐old man after receiving the mRNA COVID‐19 vaccine.
69
This patient suffered from a skin eruption over the right thigh in a dermatomal distribution, and the condition improved after antiviral treatment. Foster et al. also described two young patients under 20‐year‐old with acute lymphoblastic leukemia who presented with VZV reactivation following BNT162b2 vaccination.
70
Similarly, five cases of VZV reactivation in Spain were reported from 3007 persons vaccinated with the BNT162b2 mRNA COVID‐19 vaccine, who were immunocompetent without any abnormality.
71
Furer et al. assessed the safety of the BNT162b2 vaccine by comparing VZV reactivation in patients with autoimmune inflammatory rheumatic diseases (AIIRD) (n=
491) and controls (n
=
99) in Israel.
72
Their data indicated that six patients with AIIRD presented VZV reactivation compared to none in controls, and antiviral treatment showed a positive effect on VZV‐related symptoms. Although a series of cases reported the possible relationship between VZV reactivation and COVID‐19 vaccines, several groups provided epidemiological data to refute the promoted effect of vaccines on herpes virus‐associated diseases. Broth‐Nissimov et al. investigated the prevalence of herpes viruses in 103 paired samples of immunocompetent patients before and after receiving BNT162b2 vaccination. Their data showed no increase in oropharyngeal reactivation of herpesviruses, including VZV and all other HHVs except KSHV.
73
Birabaharan et al. presented a retrospective cohort study, including 1
306
434 persons who received a dose of the mRNA COVID‐19 vaccine. However, they were unable to observe the difference in the relative risk of herpes zoster in the 28 days after index events within vaccinated persons compared to the control cohorts.
74
Therefore, their data suggest mRNA COVID‐19 vaccination is not associated with increased rates of VZV reactivation. However, their analysis is limited by potential misclassification bias, which is inherent in using diagnostic codes. Still, these studies indicate a possible connection between COVID‐19 vaccines and VZV reactivation.
6. SARS‐CoV‐2 INFECTION AND HCMV
HCMV infection has a prevalence of 60%–70% of adults in developed countries and almost 100% in developing countries. 75 Although HCMV infection‐induced symptoms are mild in healthy people, the congenital infection of HCMV can lead to severe organ disease and even death in immunocompromised or immunosuppressed patients, including HIV‐infected patients, organ transplant recipients, and newborn infants. 76 Moreover, like other human herpesviruses, HCMV reactivation is commonly reported in critically ill patients and promotes morbidity and mortality. 77 Not surprisingly, its reactivation has been reported in individuals with severe COVID‐19 and is potentially associated with SARS‐CoV‐2 coinfection.
A clinical investigation including 38 COVID‐19 patients in France found that among 47% (18/38) of patients who presented with lymphopenia and the reactivation of herpesviruses, 2 of these patients had HCMV reactivation. 50 Another study including 257 laboratory‐confirmed COVID‐19 patients in China indicated that 1.2% of patients had HCMV reactivation, while 20.2% and 3.1% for EBV and HSV reactivation, respectively. 78 A retrospective single‐center cohort study from Germany, including 117 patients with severe COVID‐19 in ICU, found that 9% of patients presented HCMV reactivations, of which 55% were detected in patients under systemic corticosteroid treatment, 79 suggesting that critically ill patients with COVID‐19 are at high risk for HCMV reactivations. Another study from France also observed that 15% (5/34) of patients with severe COVID‐19 in the ICU suffered HCMV reactivation. 41 A previous clinical observation reported five patients at a median age of 68.2 years (range 61–78) with a coinfection of SARS‐CoV‐2 and HCMV. 80 All patients were admitted to ICU in the context of respiratory failure due to severe COVID‐19 and developed HCMV reactivation symptoms during the stay. Thus, the authors speculate that immune suppression caused by severe COVID‐19 may represent a primary reason for HCMV reactivation. Amaral et al. described a case of coinfection in a 62‐year‐old Caucasian male patient with hypoxemia, lung parenchyma, and prolonged fever. 81 This patient then developed invasive HCMV colitis, which was cured after receiving treatment with Ganciclovir, an antiviral drug against HCMV. They thought HCMV reactivation might be associated with SARS‐CoV‐2 coinfection and COVID‐19 treatment, such as glucocorticoids, anti‐IL‐6, and other immunobiological therapies. Interestingly, Basic‐Jukic et al. studied the potential benefit of hyperimmune anti‐HCMV globulin for treating renal transplant recipients with COVID‐19. 82 Their results strongly suggest using HCMV‐specific immunoglobulin to treat COVID‐19 patients with the immunocompromised state and HCMV reactivation.
7. SARS‐CoV‐2 INFECTION AND HHV‐6/HHV‐7
HHV‐6 and HHV‐7 are two closely related members of the Roseolovirus genus of the Herpesviridae family. 83 The primary infection of both viruses commonly occurs in infants or young children and then establishes latency that persists throughout life. A large majority of the general adult population is latently infected with both viruses. Reactivation of both viruses commonly causes Pityriasis rosea (PR), an acute, self‐limiting exanthematous inflammatory skin disease. Lytic reactivation of latent HHV‐6 and HHV‐7 can be induced in the conditions of immunosuppression or under stress. Several clinical investigations reported PR and PR‐like eruptions in COVID‐19 patients, which may represent a cutaneous manifestation of COVID‐19 infection, although virology tests for HHV‐6 or HHV‐7 were not performed. 84 , 85 , 86 , 87 In addition, Hu et al. reported a case of HHV‐6B reactivation in a 70‐year‐old patient with COVID‐19. 88 They confirmed the presence of nucleic acid of SARS‐CoV‐2, HHV‐6B, and HSV‐1 in the eye swabs from these patients using next‐generation sequencing technology. Drago et al. also reported a 16‐year‐old patient with pityriasis rosea following COVID‐19. 89 This patient was further found positive for HHV‐6, HHV‐7, and EBV in serology, and detectable viral DNA of HHV‐6 and EBV but not HHV‐7. Furthermore, Simonnet et al. reported 7 cases of HHV‐6 reactivation by investigating 34 patients with COVID‐19 under ICU stay. 41 Although PR or PR‐like eruptions were reported in patients after receiving different COVID‐19 vaccines, 90 the evidence of reactivation of HHV‐6 and HHV‐7 following vaccination has not been provided in the literature. Together, these data have provided clinical clues of HHV‐6/HHV‐7 lytic reactivation in some COVID‐19 patients.
8. CONCLUSION AND PROSPECT
Increasing data have indicated the potential roles of SARS‐CoV‐2 coinfection, COVID‐19 treatments, or vaccination in the reactivation of human herpesviruses. However, most of these studies come from clinical or retrospective investigations, therefore, the underlying mechanisms remain largely unclear. Nevertheless, several possible mechanisms are probably involved. First, the transcripts or proteins of SARS‐CoV‐2 can directly induce herpesviruses' reactivation by interacting with herpesvirus elements or regulating host factors involved in reactivation‐related cellular signaling pathways. Second, one of the key characteristics of SARS‐CoV‐2 infection is causing the cytokine storm, which is especially noteworthy in severely ill COVID‐19 patients. One or more of these cytokines/chemokines, including IL‐6 and TNF‐α, 91 could induce herpesvirus reactivation. 92 , 93 Third, immunosuppression in COVID‐19 patients may contribute to herpesviruses reactivation. SARS‐CoV‐2 infection can result in lymphopenia, especially in severe COVID‐19 patients, that results from hyperinflammation exhausting T cells and virus infecting T cells to interfere with T cell expansion. 94 Significant loss of NK and CD8+ T cells may cause herpesvirus infections and reactivation. 95 , 96 Fourth, the pandemic caused psychological stresses that may contribute to herpesviruses reactivation. Increasing data have confirmed COVID‐19 results in several psychological disorders, including stress, anxiety, and depression. A systematic review of recent literature showed the prevalence of stress, anxiety, and depression obtained as 29.6%, 31.9%, and 33.7% in COVID‐19 patients, respectively. 97 In addition, we assume that different herpesviruses may share some similar mechanisms (e.g., certain cellular signaling pathways) for reactivation by SARS‐CoV‐2 coinfection, although still requiring further investigation.
Because several human herpesviruses, such as KSHV, and EBV, are oncogenic, it is necessary to establish an appropriate follow‐up or surveillance system for COVID‐19 patients even after their full recovery (or some vaccinated personnel). This is especially important for immunocompromised people with herpesvirus infection because they may have a higher risk of developing severe COVID‐19 and developing virus‐associated cancers.
AUTHOR CONTRIBUTIONS
Jungang Chen, Jiao Song, Lu Dai, and Zhiqiang Qin wrote the manuscript; Steven R. Post edited the manuscript and provided critical input.
ACKNOWLEDGMENTS
This study was supported by NIH R01CA228166, R03DE031978, the Arkansas Bioscience Institute, the major research component of the Arkansas Tobacco Settlement Proceeds Act of 2000. Funding sources had no role in study design, data collection and analysis, decision to publish, or preparation of the manuscript.
Notes
Chen J, Song J, Dai L, Post SR, Qin Z. SARS‐CoV‐2 infection and lytic reactivation of herpesviruses: a potential threat in the postpandemic era? J Med Virol. 2022;94:5103‐5111. 10.1002/jmv.27994 [Europe PMC free article] [Abstract] [CrossRef] [Google Scholar]
DATA AVAILABILITY STATEMENT
All the data shown in this paper are available from the corresponding authors upon reasonable request.
REFERENCES
Full text links
Read article at publisher's site: https://doi.org/10.1002/jmv.27994
Read article for free, from open access legal sources, via Unpaywall:
https://www.ncbi.nlm.nih.gov/pmc/articles/PMC9350099
Citations & impact
Impact metrics
Citations of article over time
Alternative metrics

Discover the attention surrounding your research
https://www.altmetric.com/details/131918630
Smart citations by scite.ai
Explore citation contexts and check if this article has been
supported or disputed.
https://scite.ai/reports/10.1002/jmv.27994
Article citations
Human herpesvirus reactivation and its potential role in the pathogenesis of post-acute sequelae of SARS-CoV-2 infection.
Geroscience, 29 Aug 2024
Cited by: 0 articles | PMID: 39207648
Herpesviridae and Atypical Bacteria Co-Detections in Lower Respiratory Tract Samples of SARS-CoV-2-Positive Patients Admitted to an Intensive Care Unit.
Microorganisms, 12(4):714, 31 Mar 2024
Cited by: 0 articles | PMID: 38674658 | PMCID: PMC11051806
Precision nutrition to reset virus-induced human metabolic reprogramming and dysregulation (HMRD) in long-COVID.
NPJ Sci Food, 8(1):19, 30 Mar 2024
Cited by: 2 articles | PMID: 38555403 | PMCID: PMC10981760
Review Free full text in Europe PMC
Viral DNAemia and DNA Virus Seropositivity and Mortality in Pediatric Sepsis.
JAMA Netw Open, 7(2):e240383, 05 Feb 2024
Cited by: 1 article | PMID: 38407904 | PMCID: PMC10897747
Reactivation of Kaposi's sarcoma-associated herpesvirus (KSHV) by SARS-CoV-2 in non-hospitalised HIV-infected patients.
EBioMedicine, 100:104986, 02 Feb 2024
Cited by: 3 articles | PMID: 38306893 | PMCID: PMC10850403
Go to all (12) article citations
Similar Articles
To arrive at the top five similar articles we use a word-weighted algorithm to compare words from the Title and Abstract of each citation.
Shedding Light on the Effect of Natural Anti-Herpesvirus Alkaloids on SARS-CoV-2: A Treatment Option for COVID-19.
Viruses, 12(4):E476, 23 Apr 2020
Cited by: 13 articles | PMID: 32340120 | PMCID: PMC7232216
COVID-19 infection and vaccines: potential triggers of Herpesviridae reactivation.
An Bras Dermatol, 98(3):347-354, 10 Feb 2023
Cited by: 4 articles | PMID: 36803914 | PMCID: PMC9915050
Review Free full text in Europe PMC
SARS-CoV-2 proteins and anti-COVID-19 drugs induce lytic reactivation of an oncogenic virus.
Commun Biol, 4(1):682, 03 Jun 2021
Cited by: 27 articles | PMID: 34083759 | PMCID: PMC8175744
COVID-19: a conundrum to decipher.
Eur Rev Med Pharmacol Sci, 24(10):5830-5841, 01 May 2020
Cited by: 8 articles | PMID: 32495923
Funding
Funders who supported this work.
NCI NIH HHS (1)
Grant ID: R01 CA228166
NIDCR NIH HHS (1)
Grant ID: R03 DE031978