Abstract
Importance
Current data identifying COVID-19 risk factors lack standardized outcomes and insufficiently control for confounders.Objective
To identify risk factors associated with COVID-19, severe COVID-19, and SARS-CoV-2 infection.Design, setting, and participants
This secondary cross-protocol analysis included 4 multicenter, international, randomized, blinded, placebo-controlled, COVID-19 vaccine efficacy trials with harmonized protocols established by the COVID-19 Prevention Network. Individual-level data from participants randomized to receive placebo within each trial were combined and analyzed. Enrollment began July 2020 and the last data cutoff was in July 2021. Participants included adults in stable health, at risk for SARS-CoV-2, and assigned to the placebo group within each vaccine trial. Data were analyzed from April 2022 to February 2023.Exposures
Comorbid conditions, demographic factors, and SARS-CoV-2 exposure risk at the time of enrollment.Main outcomes and measures
Coprimary outcomes were COVID-19 and severe COVID-19. Multivariate Cox proportional regression models estimated adjusted hazard ratios (aHRs) and 95% CIs for baseline covariates, accounting for trial, region, and calendar time. Secondary outcomes included severe COVID-19 among people with COVID-19, subclinical SARS-CoV-2 infection, and SARS-CoV-2 infection.Results
A total of 57 692 participants (median [range] age, 51 [18-95] years; 11 720 participants [20.3%] aged ≥65 years; 31 058 participants [53.8%] assigned male at birth) were included. The analysis population included 3270 American Indian or Alaska Native participants (5.7%), 7849 Black or African American participants (13.6%), 17 678 Hispanic or Latino participants (30.6%), and 40 745 White participants (70.6%). Annualized incidence was 13.9% (95% CI, 13.3%-14.4%) for COVID-19 and 2.0% (95% CI, 1.8%-2.2%) for severe COVID-19. Factors associated with increased rates of COVID-19 included workplace exposure (high vs low: aHR, 1.35 [95% CI, 1.16-1.58]; medium vs low: aHR, 1.41 [95% CI, 1.21-1.65]; P < .001) and living condition risk (very high vs low risk: aHR, 1.41 [95% CI, 1.21-1.66]; medium vs low risk: aHR, 1.19 [95% CI, 1.08-1.32]; P < .001). Factors associated with decreased rates of COVID-19 included previous SARS-CoV-2 infection (aHR, 0.13 [95% CI, 0.09-0.19]; P < .001), age 65 years or older (aHR vs age <65 years, 0.57 [95% CI, 0.50-0.64]; P < .001) and Black or African American race (aHR vs White race, 0.78 [95% CI, 0.67-0.91]; P = .002). Factors associated with increased rates of severe COVID-19 included race (American Indian or Alaska Native vs White: aHR, 2.61 [95% CI, 1.85-3.69]; multiracial vs White: aHR, 2.19 [95% CI, 1.50-3.20]; P < .001), diabetes (aHR, 1.54 [95% CI, 1.14-2.08]; P = .005) and at least 2 comorbidities (aHR vs none, 1.39 [95% CI, 1.09-1.76]; P = .008). In analyses restricted to participants who contracted COVID-19, increased severe COVID-19 rates were associated with age 65 years or older (aHR vs <65 years, 1.75 [95% CI, 1.32-2.31]; P < .001), race (American Indian or Alaska Native vs White: aHR, 1.98 [95% CI, 1.38-2.83]; Black or African American vs White: aHR, 1.49 [95% CI, 1.03-2.14]; multiracial: aHR, 1.81 [95% CI, 1.21-2.69]; overall P = .001), body mass index (aHR per 1-unit increase, 1.03 [95% CI, 1.01-1.04]; P = .001), and diabetes (aHR, 1.85 [95% CI, 1.37-2.49]; P < .001). Previous SARS-CoV-2 infection was associated with decreased severe COVID-19 rates (aHR, 0.04 [95% CI, 0.01-0.14]; P < .001).Conclusions and relevance
In this secondary cross-protocol analysis of 4 randomized clinical trials, exposure and demographic factors had the strongest associations with outcomes; results could inform mitigation strategies for SARS-CoV-2 and viruses with comparable epidemiological characteristics.Free full text

Clinical and Demographic Factors Associated With COVID-19, Severe COVID-19, and SARS-CoV-2 Infection in Adults
Key Points
Question
What clinical and demographic factors are associated with rates of COVID-19, severe COVID-19, and SARS-CoV-2 infection?
Findings
In this secondary analysis of 57692 participants randomized to the placebo groups of 4 COVID-19 vaccine phase 3 efficacy trials, exposure risks, demographics (age and race), and evidence of previous infection had the strongest associations with study outcomes.
Meaning
These findings could inform public health policy pertaining to prioritization for vaccination and risk mitigation efforts.
Abstract
Importance
Current data identifying COVID-19 risk factors lack standardized outcomes and insufficiently control for confounders.
Objective
To identify risk factors associated with COVID-19, severe COVID-19, and SARS-CoV-2 infection.
Design, Setting, and Participants
This secondary cross-protocol analysis included 4 multicenter, international, randomized, blinded, placebo-controlled, COVID-19 vaccine efficacy trials with harmonized protocols established by the COVID-19 Prevention Network. Individual-level data from participants randomized to receive placebo within each trial were combined and analyzed. Enrollment began July 2020 and the last data cutoff was in July 2021. Participants included adults in stable health, at risk for SARS-CoV-2, and assigned to the placebo group within each vaccine trial. Data were analyzed from April 2022 to February 2023.
Exposures
Comorbid conditions, demographic factors, and SARS-CoV-2 exposure risk at the time of enrollment.
Main Outcomes and Measures
Coprimary outcomes were COVID-19 and severe COVID-19. Multivariate Cox proportional regression models estimated adjusted hazard ratios (aHRs) and 95% CIs for baseline covariates, accounting for trial, region, and calendar time. Secondary outcomes included severe COVID-19 among people with COVID-19, subclinical SARS-CoV-2 infection, and SARS-CoV-2 infection.
Results
A total of 57692 participants (median [range] age, 51 [18-95] years; 11
720 participants [20.3%] aged ≥65 years; 31
058 participants [53.8%] assigned male at birth) were included. The analysis population included 3270 American Indian or Alaska Native participants (5.7%), 7849 Black or African American participants (13.6%), 17
678 Hispanic or Latino participants (30.6%), and 40
745 White participants (70.6%). Annualized incidence was 13.9% (95% CI, 13.3%-14.4%) for COVID-19 and 2.0% (95% CI, 1.8%-2.2%) for severe COVID-19. Factors associated with increased rates of COVID-19 included workplace exposure (high vs low: aHR, 1.35 [95% CI, 1.16-1.58]; medium vs low: aHR, 1.41 [95% CI, 1.21-1.65]; P
<
.001) and living condition risk (very high vs low risk: aHR, 1.41 [95% CI, 1.21-1.66]; medium vs low risk: aHR, 1.19 [95% CI, 1.08-1.32]; P
<
.001). Factors associated with decreased rates of COVID-19 included previous SARS-CoV-2 infection (aHR, 0.13 [95% CI, 0.09-0.19]; P
<
.001), age 65 years or older (aHR vs age <65 years, 0.57 [95% CI, 0.50-0.64]; P
<
.001) and Black or African American race (aHR vs White race, 0.78 [95% CI, 0.67-0.91]; P
=
.002). Factors associated with increased rates of severe COVID-19 included race (American Indian or Alaska Native vs White: aHR, 2.61 [95% CI, 1.85-3.69]; multiracial vs White: aHR, 2.19 [95% CI, 1.50-3.20]; P
<
.001), diabetes (aHR, 1.54 [95% CI, 1.14-2.08]; P
=
.005) and at least 2 comorbidities (aHR vs none, 1.39 [95% CI, 1.09-1.76]; P
=
.008). In analyses restricted to participants who contracted COVID-19, increased severe COVID-19 rates were associated with age 65 years or older (aHR vs <65 years, 1.75 [95% CI, 1.32-2.31]; P
<
.001), race (American Indian or Alaska Native vs White: aHR, 1.98 [95% CI, 1.38-2.83]; Black or African American vs White: aHR, 1.49 [95% CI, 1.03-2.14]; multiracial: aHR, 1.81 [95% CI, 1.21-2.69]; overall P
=
.001), body mass index (aHR per 1-unit increase, 1.03 [95% CI, 1.01-1.04]; P
=
.001), and diabetes (aHR, 1.85 [95% CI, 1.37-2.49]; P
<
.001). Previous SARS-CoV-2 infection was associated with decreased severe COVID-19 rates (aHR, 0.04 [95% CI, 0.01-0.14]; P
<
.001).
Conclusions and Relevance
In this secondary cross-protocol analysis of 4 randomized clinical trials, exposure and demographic factors had the strongest associations with outcomes; results could inform mitigation strategies for SARS-CoV-2 and viruses with comparable epidemiological characteristics.
Introduction
SARS-CoV-2 infection and COVID-19 remain a significant global health challenge.1,2 Despite the development of safe and effective vaccines, globally, billions of people remain unvaccinated. Greater understanding of risk factors for infection and severe disease can guide future vaccine uptake prioritization strategies and therapeutic allocation policies.
Prospective studies have demonstrated that higher risk of SARS-CoV-2 infection is associated with demographic and behavioral cofactors.3,4,5,6,7,8,9,10,11,12,13,14,15,16,17,18 In contrast, the associations of underlying medical conditions with infection acquisition are less well-defined, although retrospective reports have reported diabetes and obesity were associated with increased risk.7,19,20,21,22,23,24 Prospective studies have identified both demographic characteristics and comorbidities as risk factors associated with severe COVID-19.25,26,27,28,29,30,31
Much of the data defining risk for either acquisition of SARS-CoV-2 or severe COVID-19 come from studies that relied on surveys, registries, or electronic medical records. These data often lack a harmonized definition of outcomes, and none used active surveillance. Moreover, high-quality data regarding risk factors for broadly symptomatic COVID-19 are sparse, despite clear, long-term impacts of disease.32,33
The COVID-19 Prevention Network (CoVPN) was formed by the US National Institutes of Health to conduct phase 3 vaccine clinical trials.34 Four randomized, controlled, efficacy trials were conducted with harmonized protocols beginning in 2020.35,36,37,38 The placebo recipients from these trials afford a large, diverse, multinational cohort. Using these detailed prospective data, and controlling for trial, region, and time in the pandemic, we sought to identify independent risk factors associated with COVID-19, severe COVID-19, any SARS-CoV-2 infection, and subclinical SARS-CoV-2 infection.
Methods
Study Design and Setting
We performed a secondary cross-protocol analysis of participant-level data from the blinded phase of 4 randomized, placebo-controlled, phase 3 COVID-19 vaccine efficacy trials. Local or central institutional review board and ethics committee approvals were obtained by each site participating in the 4 trials.35,36,37,38 All participants provided written informed consent to participate in the trials.
We assessed data from participants in the placebo groups of the COVE/CoVPN3001 (ClinicalTrials.gov identifier: NCT04470427; mRNA1273, Moderna), AZD1222 (ChAdOx1 nCoV-19/CoVPN3002; ClinicalTrials.gov identifier: NCT04516746; AZD1222, AstraZeneca), ENSEMBLE /CoVPN3003 (ClinicalTrials.gov identifier: NCT04505722; Ad26.COV2.S, Janssen), and PREVENT-19/CoVPN3004 (ClinicalTrials.gov identifier: NCT04611802; NVX-CoV2373, Novavax) trials (Figure 1), hereafter, referred to as the Moderna, AstraZeneca, Janssen, and Novavax cohorts. Individual study protocols describing study design have been previously published.35,36,37,38
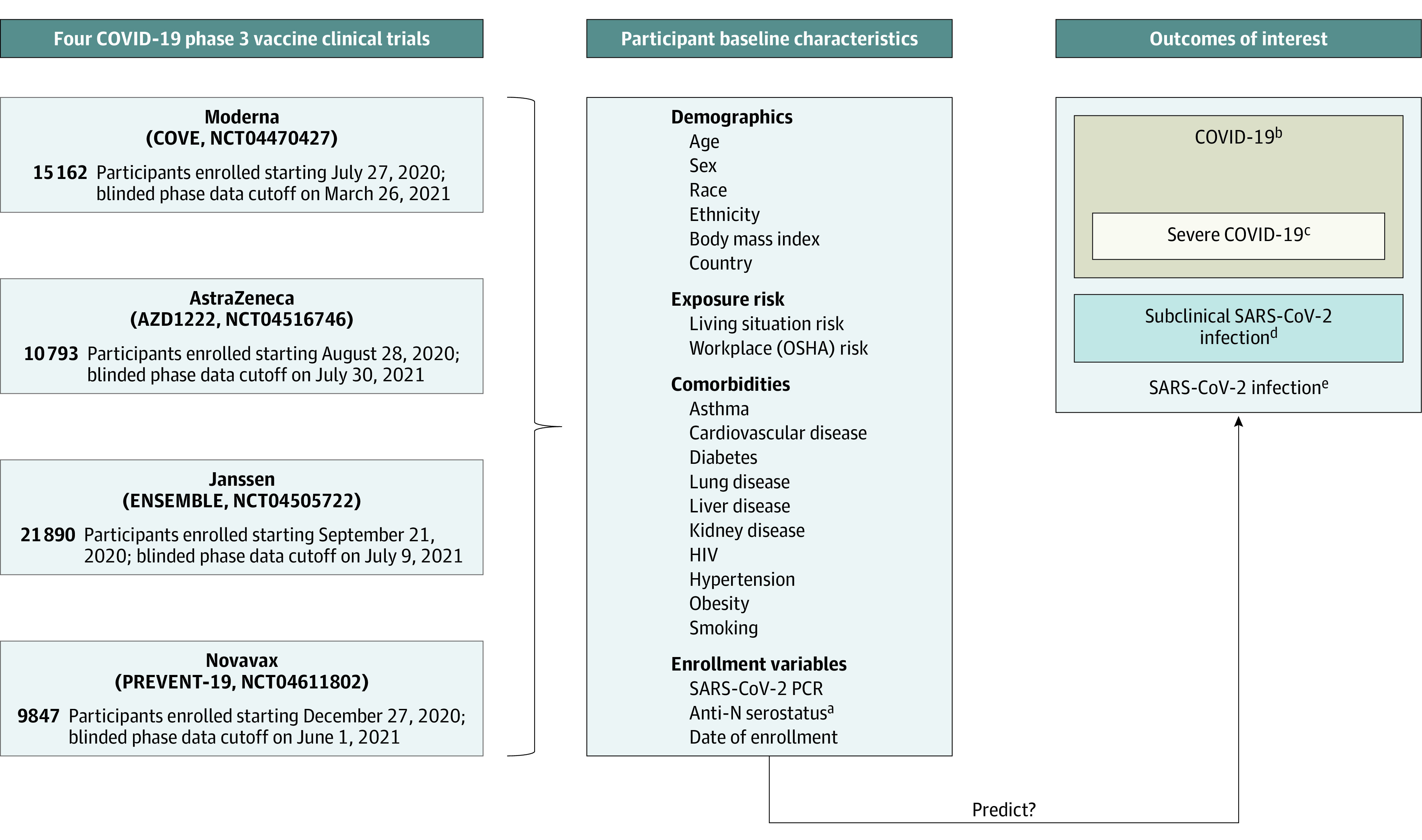
aAnti-N: Binding antibodies against SARS-CoV-2 nucleocapsid protein.
bDetermined by a harmonized definition across studies with minor differences (eTable 1 in Supplement 1).
cDefined per Centers for Disease Control and Prevention criteria (see eTable 1 in Supplement 1).
dDetection of SARS-CoV-2 on reverse transcription–polymerase chain reaction testing and/or anti-N seroconversion (baseline seronegative to seropositive) detected at designated study visits among participants who never met the definition for COVID-19. Limited to participants in per-protocol analysis.
eMeeting the end point of COVID-19 or meeting the subclinical SARS-CoV-2 infection end point. Limited to participants in per-protocol analysis.
Major eligibility criteria were age at least 18 years, in stable health, and at risk for SARS-CoV-2 infection.39 This analysis constitutes data accrued through the blinded, precrossover phases of the trials from placebo recipients who received at least the first study injection.
Trial start dates and blinded precrossover phase cutoff dates are presented in Figure 1. Participants were enrolled from 8 countries, with enrollment beginning in July 2020 and the last blinded phase data cutoff in July 2021.
Study End Points
Study end points diagnosed at least 1 day after the first placebo administration were included and censored at the earliest date of drop out, unblinding, crossover, receipt of outside vaccination, or blinded phase data cutoff. The primary end point was time to COVID-19, defined with minor differences across studies as positive SARS-CoV-2 reverse transcriptase–polymerase chain reaction (RT-PCR) test result and systemic and/or respiratory symptoms (eTable 1 in Supplement 1).39 The severe COVID-19 end point was defined per Centers for Disease Control and Prevention criteria, including shortness of breath at rest or respiratory distress, respiratory rate of at least 30 breaths per minute, heart rate of at least 125 beats per minute, oxygen saturation 93% or less on room air, respiratory or multiorgan failure, intensive care unit admission, or death (eTable 1 in Supplement 1).
The subclinical SARS-CoV-2 infection end point was defined as having positive SARS-CoV-2 RT-PCR test result or antinucleocapsid protein (anti-N) test result, but not meeting the definition for the primary (symptomatic) COVID-19 end point among participants whose test results were negative for SARS-CoV-2 at baseline. The testing schedule is shown in eTable 2 in Supplement 1.
The any SARS-CoV-2 infection end point was defined as meeting the primary COVID-19 end point or meeting the subclinical SARS-CoV-2 infection end point among participants whose test results were negative for SARS-CoV-2 at baseline. Each of the 4 trials included anti-N testing at designated visits; the Moderna and Novavax cohorts also included SARS-CoV-2 RT-PCR testing regardless of symptoms at designated visits (eTable 2 in Supplement 1). Additional analyses were performed to identify variables associated with COVID-19 rates among participants meeting the any SARS-CoV-2 infection end point and to identify variables associated with severe COVID-19 rates among participants meeting the COVID-19 end point.
Baseline Characteristics
We evaluated 23 pre-enrollment variables as potential risk factors for study outcomes. These variables included demographic characteristics: age (≥65 vs 18-64 years), sex assigned at birth, race, ethnicity, body mass index (BMI; calculated as weight in kilograms divided by height in meters squared), and region (SARS-CoV-2 epidemiological characteristics in South America, North America, or South Africa, where trials were conducted); comorbid conditions: asthma, cardiovascular disease, hypertension, diabetes, smoking, obesity (defined as BMI ≥30), lung disease, liver disease, kidney disease, HIV, and number of comorbidities; and SARS-CoV-2 exposure risk or history: Occupational Safety and Health Administration risk category (high, medium, and low), living situation risk score (very high, high, medium, and low), evidence of previous SARS-CoV-2 infection (defined as positive SARS-CoV-2 PCR test result or anti-N serostatus) at screening or enrollment, and calendar date of enrollment. Race and ethnicity were collected by self report and categorized as American Indian or Alaska Native, Asian, Black or African American, White, other, and multiple. Indigenous people from South America were classified together with the American Indian or Alaska Native US and Mexico demographic according to the US Food and Drug definition (ie, a person having origins in any of the original peoples of North and South America [including Central America] and who maintains tribal affiliation or community attachment). Participants also had the option to select other race without specifying. Ethnicity was categorized as Hispanic or Latino or not Hispanic or Latino. Race and ethnicity were included in analysis to describe participant demographic characteristics. Definitions and derivations of these variables are provided in the eMethods and eTable 3 in Supplement 1.
Statistical Analysis
The COVID-19 and severe COVID-19 end points were evaluated with the full cohort of participants who received the first placebo administration. The any SARS-CoV-2 infection and subclinical SARS-CoV-2 infection end points analyses were restricted to the per-protocol population of participants with negative SARS-CoV-2 test results at baseline who received all planned placebo injections. Instantaneous hazard estimates over calendar time were obtained by smoothing the increments of the Nelson-Aalen estimator for the cumulative hazard function and plotted with global epidemiologic trends using information from the World Health Organization (WHO)40 and GISAID.41 Missing data were imputed by the median for continuous variables and by the most frequent category for categorical variables. Univariate and multivariate analyses generated adjusted hazard ratios (aHRs) adjusted for study and region as stratification variables (reflecting SARS-CoV-2 epidemiological characteristics in North America, South America, and South Africa) to account for potentially different baseline hazard functions across trials and regions. Survival random forest, as implemented in the randomForestSRC package in R statistical software version 4.1.0 (R Project for Statistical Computing), was used to rank the relative importance of covariates, without imposing any parametric assumptions on the association between the risk factors and the study outcomes, while accounting for potential correlations among the risk factors.42 Univariate and multivariate Cox proportional hazard regression models, with and without 2-way interaction terms for age (18-64 vs ≥65 years) or ethnicity and each of the other covariates, were used to estimate the associations of baseline covariates using both calendar and study time scales. Given the similarity of the results, only calendar time models are presented. The Cox proportional hazard assumption was evaluated for each risk factor considered in the models.43 The model assumption results for the primary COVID-19 end point are shown in eFigure 1 in Supplement 1. False-discovery rate–based P value adjustment was applied within each end point for all univariate analyses. The final multivariate Cox models with and without interactions were chosen by the Akaike information criterion in a stepwise algorithm. The final multivariate Cox model with 2-way interactions kept interaction terms with P<
.01 for reliability of interpretation. Consequently, not all variables that were significant in the univariate analysis were included in the multivariate model. P
<
.01 was considered statistically significant. All analyses were performed using R software version 4.1.0. Data were analyzed from April 2022 to February 2023.
Results
Demographics
Across the 4 studies, 57692 participants were randomized and received placebo injections. Median (range) age was 51 (18-95) years; 31
058 (53.8%) were male, and 11
720 participants (20.3%) were aged 65 years or older (Table). The Novavax study enrolled the youngest population, with 12.6% of placebo participants aged 65 years or older.
Table.
Characteristic | Study participants, No. (%) | ||||
---|---|---|---|---|---|
Moderna (n![]() ![]() ![]() | AstraZeneca (n![]() ![]() ![]() | Janssen (n![]() ![]() ![]() | Novavax (n![]() ![]() | Total (n![]() ![]() ![]() | |
Age, y | |||||
Median (range) | 52 (18-95) | 51 (18-93) | 52 (18-94) | 47 (18-90) | 51 (18-95) |
≥65 | 3751 (24.7) | 2436 (22.6) | 4298 (19.6) | 1235 (12.5) | 11![]() |
18-64 | 11![]() | 8357 (77.4) | 17![]() | 8612 (87.5) | 45![]() |
BMI | |||||
Median (range) | 28.1 (10.3-72.7) | 27.7 (14.9-71.7) | 27 (13.3-82.6) | 28 (10.5-71.7) | 27.5 (10.3-82.6) |
Missing | 85 (0.6) | 113 (1.0) | 18 (0.1) | 39 (0.4) | 255 (0.4) |
Sex at birth | |||||
Female | 7106 (46.9) | 4789 (44.4) | 9907 (45.3) | 4828 (49.0) | 26![]() |
Male | 8056 (53.1) | 6004 (55.6) | 11![]() | 5019 (51.0) | 31![]() |
Intersexa | NA | NA | 4 (<0.1) | NA | 4 (<0.1) |
Country | |||||
Argentina | NA | NA | 1498 (6.8) | NA | 1498 (2.6) |
Brazil | NA | NA | 3635 (16.6) | NA | 3635 (6.3) |
Chile | NA | 729 (6.8) | 570 (2.6) | NA | 1299 (2.3) |
Colombia | NA | NA | 2123 (9.7) | NA | 2123 (3.7) |
Mexico | NA | NA | 241 (1.1) | 588 (6.0) | 829 (1.4) |
Peru | NA | 491 (4.5) | 885 (4.0) | NA | 1376 (2.4) |
South Africa | NA | NA | 3289 (15.0) | NA | 3289 (5.7) |
United States | 15![]() | 9573 (88.7) | 9649 (44.1) | 9259 (94.0) | 43![]() |
Raceb | |||||
American Indian or Alaska Native | 121 (0.8) | 428 (4.0) | 2060 (9.4) | 661 (6.7) | 3270 (5.7) |
Asian | 739 (4.9) | 482 (4.5) | 686 (3.1) | 416 (4.2) | 2323 (4.0) |
Black or African American | 1531 (10.1) | 892 (8.3) | 4262 (19.5) | 1164 (11.8) | 7849 (13.6) |
White | 11![]() | 8523 (79.0) | 12![]() | 7381 (75.0) | 40![]() |
Other | 326 (2.2) | 21 (0.2) | 47 (0.2) | 12 (0.1) | 406 (0.7) |
Multiple | 318 (2.1) | 257 (2.4) | 1248 (5.7) | 159 (1.6) | 1982 (3.4) |
Missing | 129 (0.9) | 190 (1.8) | 744 (3.4) | 54 (0.5) | 1117 (1.9) |
Ethnicity | |||||
Hispanic or Latino | 3108 (20.5) | 2451 (22.7) | 9964 (45.5) | 2155 (21.9) | 17![]() |
Not Hispanic or Latino | 11![]() | 8202 (76.0) | 11![]() | 7669 (77.9) | 39![]() |
Missing | 136 (0.9) | 140 (1.3) | 559 (2.6) | 23 (0.2) | 858 (1.5) |
Comorbid conditions | |||||
Asthma | 1429 (9.4) | 1149 (10.6) | 1073 (4.9) | 942 (9.6) | 4593 (8.0) |
Cardiovascular disease | 4728 (31.2) | 3079 (28.5) | 5797 (26.5) | 2392 (24.3) | 15![]() |
Diabetes | 1567 (10.3) | 1096 (10.2) | 1968 (9.0) | 998 (10.1) | 5629 (9.8) |
History of smoking | 274 (1.8) | 2059 (19.1) | 404 (1.8) | 3103 (31.5) | 5840 (10.1) |
HIV | 96 (0.6) | 171 (1.6) | 621 (2.8) | 65 (0.7) | 953 (1.7) |
Hypertension | 4517 (29.8) | 2924 (27.1) | 5544 (25.3) | 2325 (23.6) | 15![]() |
Kidney disease | 82 (0.5) | 62 (0.6) | 123 (0.6) | 64 (0.6) | 331 (0.6) |
Liver disease | 107 (0.7) | 209 (1.9) | 180 (0.8) | 74 (0.8) | 570 (1.0) |
Lung disease | 870 (5.7) | 1347 (12.5) | 1330 (6.1) | 1446 (14.7) | 4993 (8.7) |
Obesity | 5871 (38.7) | 3883 (36.0) | 6326 (28.9) | 3799 (38.6) | 19![]() |
≥2 Comorbid conditions | 5613 (37.0) | 4559 (42.2) | 6903 (31.5) | 4089 (41.5) | 21![]() |
≥3 Comorbid conditions | 3284 (21.7) | 2671 (24.7) | 3813 (17.4) | 2463 (25.0) | 12![]() |
≥4 Comorbid conditions | 1198 (7.9) | 1198 (7.9) | 1297 (5.9) | 1267 (12.9) | 4960 (8.6) |
Living conditionc | |||||
Very high risk | 441 (2.9) | 1560 (14.5) | 1470 (6.7) | 155 (1.6) | 3626 (6.3) |
High risk | 1302 (8.6) | 1665 (15.4) | 4083 (18.7) | 398 (4.0) | 7448 (12.9) |
Medium risk | 10![]() | 1976 (18.3) | 6367 (29.1) | 1297 (13.2) | 20![]() |
Low risk | 2477 (16.3) | 5592 (51.8) | 9970 (45.5) | 7997 (81.2) | 26![]() |
Workplace risk of exposure to SARS-CoV-2d | |||||
High | 5314 (35.1) | 2975 (27.6) | 660 (3.0) | 886 (9.0) | 9835 (17.0) |
Medium | 3219 (21.2) | 4485 (41.6) | 276 (1.3) | 3209 (32.6) | 11![]() |
Low | 0 | 3208 (29.7) | 20![]() | 5752 (58.4) | 29![]() |
Missing or other | 6629 (43.7)e | 125 (1.2) | 27 (0.1) | 0 | 6781 (11.8) |
Baseline SARS-CoV-2 status | |||||
Positive RT-PCR test result | 95 (0.6) | 7 (0.1) | 127 (0.6) | 107 (1.1) | 336 (0.6) |
Positive serostatus | 303 (2.0) | 293 (2.7) | 2103 (9.6) | 623 (6.3) | 3322 (5.8) |
Overall positive statusf | 337 (2.2) | 300 (2.8) | 2208 (10.1) | 691 (7.0) | 3536 (6.1) |
Abbreviations: BMI, body mass index (calculated as weight in kilograms divided by height in meters squared); RT-PCR, reverse transcriptase–polymerase chain reaction.
Overall, 3270 participants (5.7%) were American Indian or Alaska Native, 7849 participants (13.6%) were Black or African American, 17678 participants (30.6%) were Hispanic or Latino, and 40
745 participants (70.6%) were White. Most participants were enrolled in the US (43
643 participants [75.6%]), followed by Brazil (3635 participants [6.3%]), South Africa (3289 participants [5.7%]), Colombia (2123 participants [3.7%]), Argentina (1498 participants [2.6%]), Peru (1376 participants [2.4%]), Chile (1299 participants [2.3%]), and Mexico (829 participants [1.4%]). Demographic characteristics were largely consistent across the 4 trials; the Janssen study contributed the most diverse population racially, ethnically, and geographically (Table).
Clinical Characteristics at Enrollment
Comorbid conditions among placebo recipients were distributed similarly across trials and included cardiovascular disease (15996 participants [27.7%]), diabetes (5629 participants [9.8%]), kidney disease (331 participants [0.6%]), liver disease (570 participants [1.0%]), lung disease (4993 participants [8.7%]), obesity (19
879 participants [34.5%]), HIV (953 participants [1.7%]), history of smoking (5840 participants [10.1%]), and hypertension (15
310 participants [26.5%]). Across trials, 21
164 participants (36.7%) had 2 or more comorbidities, 12
231 participants (21.2%) had 3 or more comorbidities, and 4960 participants (8.6%) had 4 or more comorbidities. Most participants had negative results on baseline anti-N antibody and SARS-CoV-2 RT-PCR testing (54
156 participants [93.9%]).
Exposure Risk
A low-risk living condition was most common (26036 participants [45.1%]), followed by medium (20
582 participants [35.7%]), high (7448 participants [12.9%]), and very high (3626 participants [6.3%]). Living condition risk varied by study, reflecting regional differences of the individual trial populations. A lower workplace exposure risk was most common (29
887 participants [51.8%]), followed by medium (11
189 participants [19.4%]) and high (9131 participants [15.8%]) workplace exposure risk. Participants in the Janssen trial had the lowest workplace exposure risks.
Incidence Rates by Study Outcome
Median (range) blinded precrossover follow-up time was 3.8 (0-11.1) months. Across the 4 trials, 2559 placebo recipients developed COVID-19, with an incidence rate of 13.9% (95% CI, 13.3%-14.4%); 367 placebo recipients developed severe COVID-19, with an incidence rate of 2.0% (95% CI, 1.8%-2.2%) (eTable 4 in Supplement 1). In the per-protocol cohort, 3774 placebo recipients developed any SARS-CoV-2 infection, with an incidence rate of 24.3% (95% CI, 23.5%-25.1%); 1612 placebo recipients developed subclinical infection, with an incidence rate of 10.3% (95% CI, 9.8%-10.9%). We plotted the smoothed hazard estimates over calendar time for COVID-19 in each trial (Figure 2; eFigure 2 in Supplement 1). To put our data into context, epidemiological trends for the regions of the trials are presented using information from the WHO40 and GISAID.41 Smoothed hazard peaks largely aligned with case trends in the countries contributing data to each study. The trials enrolled primarily during pandemic waves with the ancestral and Alpha variants. However, trials also included participants with infections with Beta, Delta, Epsilon, Gamma, Mu, and Lambda variants (Figure 2; eFigure 2 and eTable 5 in Supplement 1).
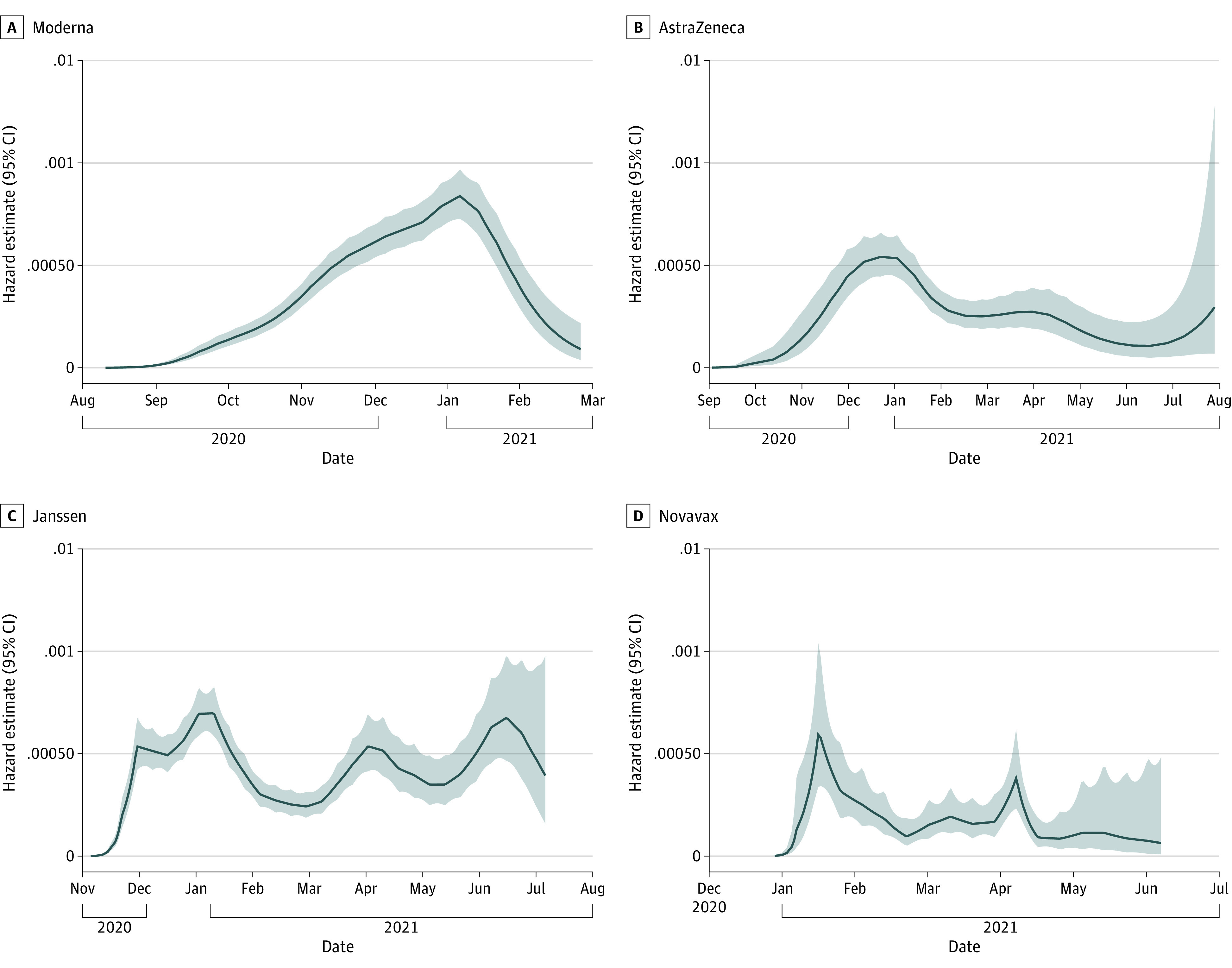
Smoothed hazard estimates are plotted over calendar time for the COVID-19 endpoint in each trial. Corresponding epidemiological trends within the countries contributing data to the trials, including number of cases and emergence of viral variants, are presented in eFigure 2 in Supplement 1.
Variables Associated with Study Outcomes
Univariate Cox regression model results are included in eFigure 3 and eFigure 4 in Supplement 1. In the multivariate Cox regression models, covariates associated with an increased rate of COVID-19 included workplace exposure risk (high vs low risk: aHR, 1.35 [95% CI, 1.16-1.58]; medium vs low risk: aHR, 1.41 [95% CI, 1.21-1.65]; overall P<
.001), living condition risk (very high vs low risk: aHR, 1.41 [95% CI, 1.21-1.66]; medium vs low risk: aHR, 1.19 [95% CI, 1.08-1.32]; overall P
<
.001), and BMI (aHR per 1-unit increase, 1.02 [95% CI, 1.01-1.03]; P
<
.001) (Figure 3). Covariates associated with a decreased rate of COVID-19 included age 65 years or older (aHR vs <65 years, 0.57 [95% CI, 0.50-0.64]; P
<
.001), Black or African American race (aHR vs White race, 0.79 [95% CI, 0.68-0.92]; overall P
=
.002), evidence of previous SARS-CoV-2 infection at enrollment (aHR, 0.13 [95% CI, 0.09-0.19]; P
<
.001), hypertension (aHR, 0.78 [95% CI, 0.66-0.93]; P
=
.004), and history of smoking (aHR, 0.76 [95% CI, 0.63-0.92]; P
=
.005).
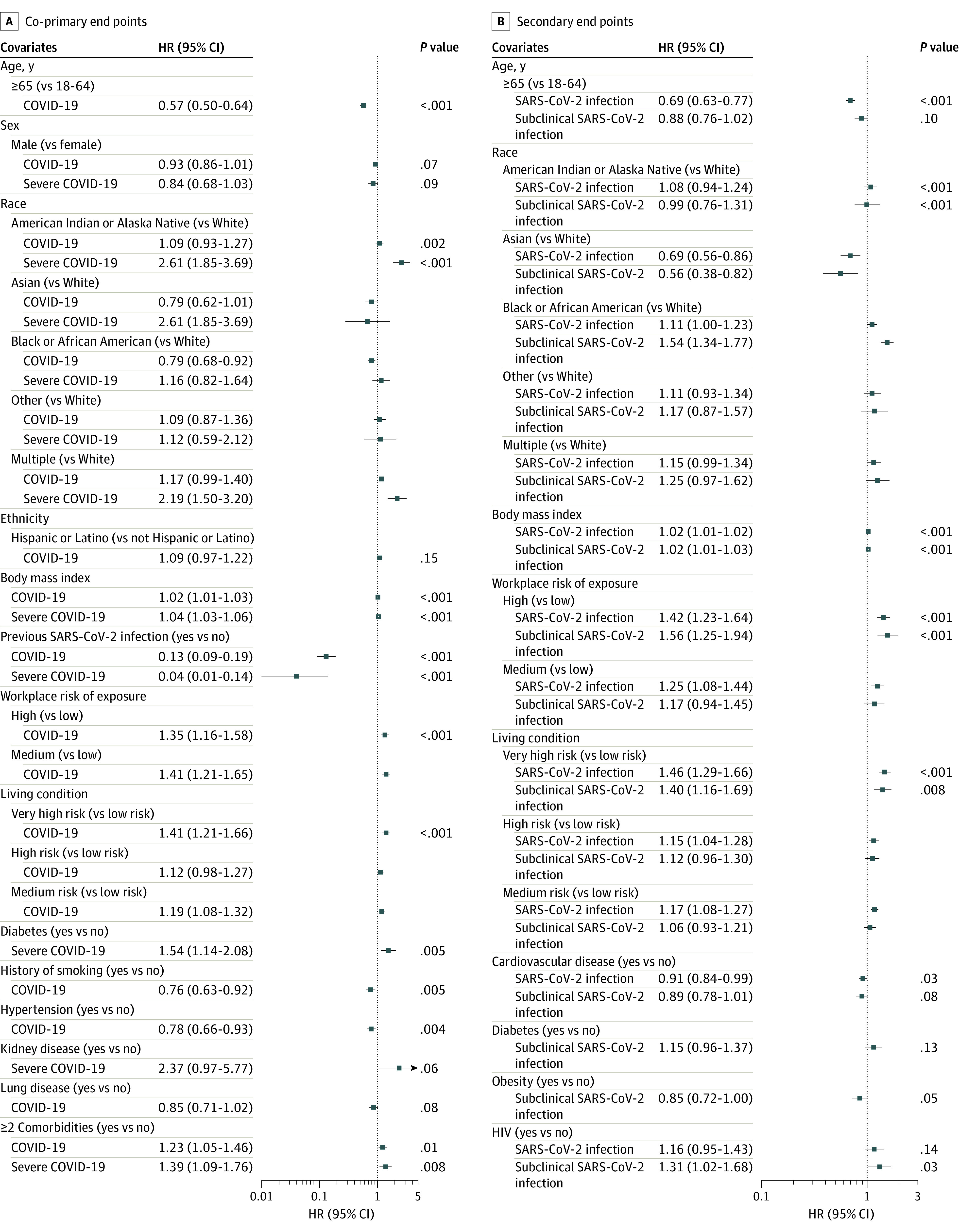
All models adjusted for study (Moderna, AstraZeneca, Janssen, Novavax), Region (South America, North America, South Africa) and calendar time to account for potentially different baseline hazard functions across studies, regions, and time. P=
.01 was considered statistically significant.
We then considered these covariates for the severe COVID-19 end point. Covariates associated with an increased rate of severe COVID-19 included American Indian or Alaska Native race (aHR vs White race, 2.61 [95% CI, 1.85-3.69]) and multiple races (aHR vs White race, 2.19 [95% CI, 1.50-3.20]; overall P<
.001), higher BMI (aHR per 1-unit increase, 1.04 [95% CI, 1.03-1.06]; P
<
.001), diabetes (aHR, 1.54 [95% CI, 1.14-2.08]; P
=
.005), and having at least 2 comorbidities (aHR vs none, 1.39 [95% CI, 1.09-1.76]; P
=
.008). Previous SARS-CoV-2 infection at enrollment was associated with a decreased rate of severe COVID-19 (aHR, 0.04 [95% CI, 0.01-0.14]; P
<
.001).
In an analysis limited to participants meeting the COVID-19 end point, an increased rate of severe COVID-19 was associated with age at least 65 years (aHR vs <65 years, 1.75 [95% CI, 1.32-2.31]; P<
.001), American Indian or Alaska Native race (aHR vs White race, 1.98 [95% CI, 1.38-2.83]), Black or African American race (aHR vs White race, 1.49 [95% CI, 1.03-2.14]), multiple races (aHR vs White race, 1.81 [95% CI, 1.21-2.69]; overall P for race
=
.001), BMI (aHR per 1-unit increase, 1.03 [95% CI, 1.01-1.04]; P
=
.001), and diabetes (aHR, 1.85 [95% CI, 1.37-2.49]; P
<
.001) (eFigure 5 and eFigure 6 in Supplement 1).
We next assessed covariates associated with rates of subclinical SARS-CoV-2 infection among participants in the per-protocol cohort. Covariates associated with an increased rate of subclinical SARS-CoV-2 infection included Black or African American race (aHR vs White race, 1.54 [95% CI, 1.34-1.77]; overall P<
.001); higher BMI (HR per 1-unit increase, 1.02 [95% CI, 1.01-1.03]; P
<
.001), high workplace exposure risk (aHR vs low risk, 1.56 [95% CI, 1.25-1.94]; overall P
<
.001), and very high–risk living situation (aHR vs low risk, 1.40 [95% CI, 1.16-1.69]; P
=
.008). Asian race was associated with a lower rate of subclinical SARS-CoV-2 infection compared with White race (aHR, 0.56 [95% CI, 0.38-0.82]; P
<
.001).
We next analyzed the data across all participants in the per-protocol cohort meeting the any SARS-CoV-2 infection end point (COVID-19 or subclinical SARS-CoV-2 infection). Covariates associated with an increased rate of any SARS-CoV-2 infection included higher BMI (HR per 1-unit increase, 1.02 [95% CI, 1.01-1.02]; P<
.001); workplace exposure risk (high vs low risk: aHR, 1.42 [95% CI, 1.23-1.64]; medium vs low risk: aHR, 1.25 [95% CI, 1.08-1.44]; overall P
<
.001); living situation risk (very high vs low risk: aHR, 1.46 [95% CI, 1.29-1.66]; high vs low risk: aHR, 1.15 [95% CI, 1.04-1.28]; medium vs low risk: aHR, 1.17 [95% CI, 1.08-1.27]; overall P
<
.001), and Black or African American race (aHR vs White race, 1.11 [95% CI, 1.00-1.23]; P
<
.001).
However, among participants meeting the any SARS-CoV-2 infection definition, race was significantly associated with a decreased rate of developing COVID-19 (American Indian or Alaska Native vs White race: aHR, 0.63 [95% CI, 0.54-0.75]; Black or African American vs White race: aHR, 0.56 [95% CI, 0.47-0.67]; multiracial vs White race: aHR, 0.71 [95% CI, 0.59-0.88]; overall P<
.001) (eFigure 7 and eFigure 8 in Supplement 1).
The relative strength of association of covariates with each end point was assessed using a variable importance ranking analysis. Consistent with the Cox regression models, evidence of previous SARS-CoV-2 infection at enrollment, regional differences in SARS-CoV-2 epidemiological characteristics (eFigure 2 in Supplement 1), race, age, living condition, and BMI were top variables associated with COVID-19 rates; for severe COVID-19, evidence of previous SARS-CoV-2 infection at enrollment and region were top variables (Figure 4).
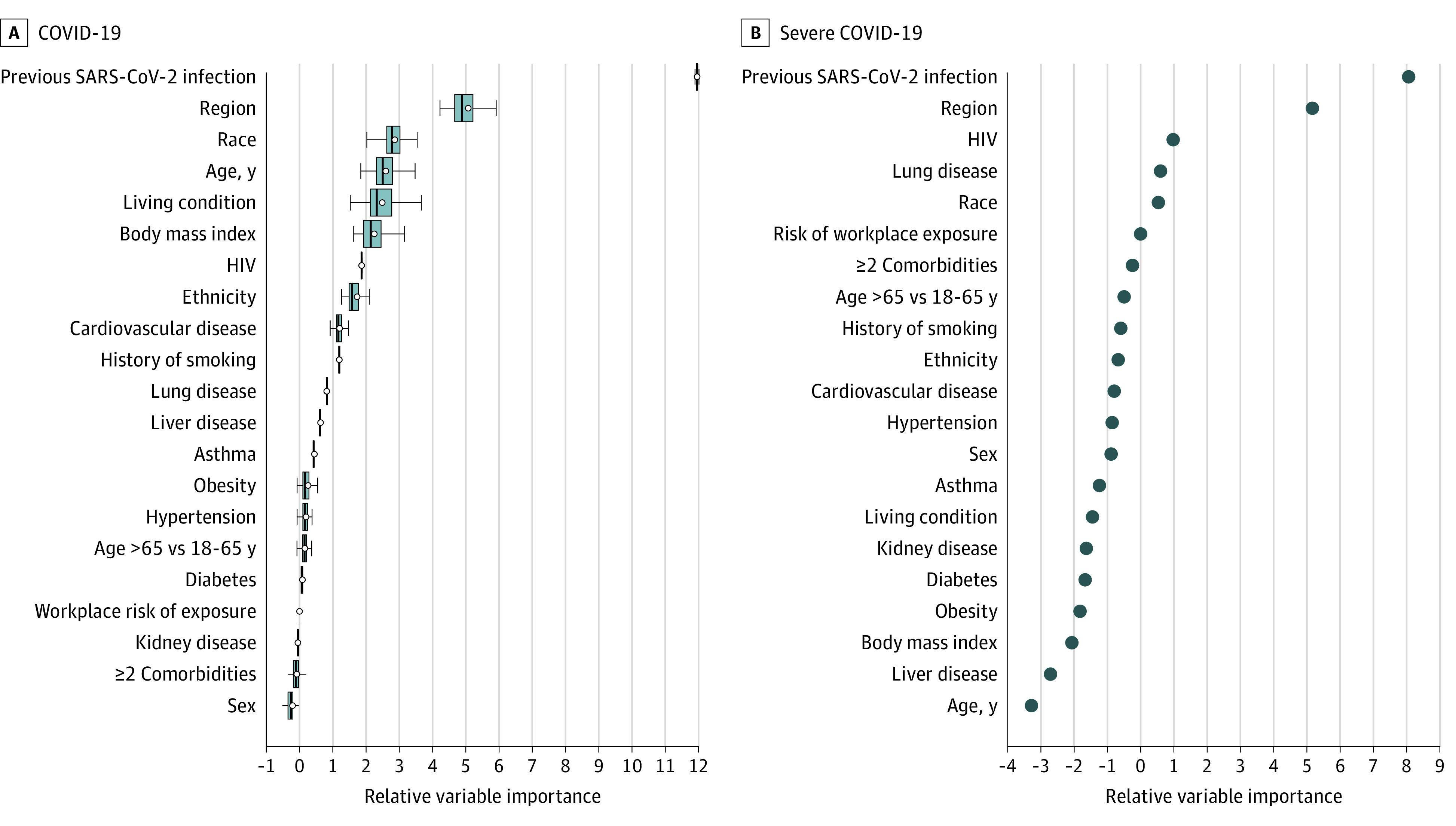
Survival random forest was used to rank variables associated with rates of study outcomes. This analysis included both the covariates and stratification variables considered in the Cox models. Age is presented as both a continuous variable in years and stratified as 18 to 64 years vs 65 years or older. The default splitting rule (log-rank) and the default number of covariates randomly selected (square root of the total number of covariates) for each split of 1000 trees were used. The y-axis lists the variables that were included in the construction of the survival random forest. The x-axis ranks the strength of association, where variables to the left of the 0 are considered of negligible importance and variables to the right of 0 demonstrated increasing strength of association with the study outcomes. To calculate the relative variable importance of each variable, values of the given variable that were not used in the construction of a specific survival tree in the ensemble were permuted and the resulting estimation error was compared to that obtained without the permutation. The difference between the 2 estimation errors was then aggregated over all trees in the ensemble as the variable importance measure for the given variable. A subsampling approach was used to estimate the variance of the variable importance and for constructing confidence intervals. A, Ranking of variables associated with the COVID-19 end point, with circles indicating variable importance estimates. The midlines indicate medians; boxes, IQRs of estimates based on the subsampling approach. The whiskers extend to the most extreme estimates that are no more than 1.5 times the width of the box or if no estimate meets this criterion, to the estimate extremes. B, Ranking of variables associated with the severe COVID-19 end point. For this end point, all variables had a relatively small number for cases, thereby prohibiting confidence interval estimates.
As age and ethnicity, 2 demographic characteristics of COVID-19 research focus, were associated with COVID-19 rates in the univariate analyses, we examined their interactions with other covariates and found significant interactions between age and workplace exposure risk and between evidence of previous SARS-CoV-2 infection and ethnicity. High workplace exposure was associated with an increased rate of COVID-19 among adults aged 18 to 64 years but a decreased rate among adults 65 years or older (eFigure 9 in Supplement 1). The inverse association of older age with COVID-19 rates lessened in strength as workplace exposure risk decreased (high exposure risk aHR, 0.32 [95% CI, 0.24-0.43]; medium exposure risk aHR, 0.59 [95% CI, 0.47-0.74]; low exposure risk aHR, 0.69 [95% CI, 0.58-0.81]; P for interaction<
.001). Evidence of previous SARS-CoV-2 infection at enrollment demonstrated a stronger association with a decreased rate of COVID-19 among Hispanic or Latino (aHR, 0.08 [95% CI, 0.04-0.14]) compared with participants who were not Hispanic or Latino (aHR, 0.23 [95% CI, 0.15-0.36]; P for interaction
=
.004).
Discussion
In this secondary cross protocol analysis of 4 randomized clinical trials, we present the largest, most diverse, global cohort with prospective active surveillance follow-up to our knowledge, enabling precise outcome ascertainment and identification of variables associated with COVID-19 rates. We noted high incidence of SARS-CoV-2 infection in approximately 4 months of follow-up, highlighting the remarkable infectivity of the virus and providing sufficient events for a robust risk assessment. Risk factors identified in this analysis can inform mitigation strategies for SARS-CoV-2 and viruses with comparable epidemiological characteristics. In addition, the characterization of these risk factors will increase efficiency in future analyses of this unique data set.
The sequential rollout of the 4 clinical trials allowed evaluation over different pandemic waves and diverse SARS-CoV-2 strains, affording generalizable results by capturing infections during periods of circulation of the ancestral strain, B.1.1.7 (Alpha variant), B.1.617.2 (Delta variant), B.1.351 (Beta variant), P.1 (Gamma variant), and C.37 (Lambda variant).44
In our analysis, hazard of COVID-19 was associated with workplace exposure risks and living condition risks and inversely associated with previous SARS-CoV-2 infection. Additionally, region had a strong association with hazard of COVID-19, which may reflect differences in study population and local mitigation practices, force of infection during the follow-up period, or the circulating variant.14,15 In this study, we confirm previous findings reporting associations of infection rates with exposure risk and baseline serostatus,14,15 and we offer additional insights, showing that prior infection and ongoing exposure risks at the individual, household, and regional level were associated not only with infection but also disease.
Some of our findings diverge from previously reported observations. For example, large prospective studies have found male sex was associated with SARS-CoV-2 acquisition and severe disease,17,45 which we did not find. Second, Black or African American race was associated with a higher rate of any SARS-CoV-2 infection and subclinical infection but a lower rate of COVID-19. This could reflect selection bias in our study population related to socioeconomic status or health care–seeking behavior of trial participants. Consistent with other reports, in the analysis of severe COVID-19 among participants with COVID-19, Black or African American race was associated with severe disease, as was American Indian or Alaska Native race. This striking finding for American Indian or Alaska Native participants has been previously reported46,47,48 with SARS-CoV-2 and other respiratory viruses49,50 and highlights the need to elucidate the mechanism behind the association.
Previous studies have demonstrated increased risk for severe COVID-19 among older individuals,31 which is consistent with other respiratory viruses, like influenza and respiratory syncytial virus.51,52,53 In our analysis, age had a strong inverse association with any SARS-CoV-2 infection and COVID-19, with the strongest inverse association among those with the highest workplace exposure risk. These findings likely reflect stricter adherence to social distancing and masking and lower social activity by older participants. This association was not seen with severe COVID-19, suggesting that a lower rate of infection due to behavioral adjustment obscured a truly higher rate of severe disease in older adults as was seen in our ad hoc analysis of severe disease rates restricted to participants with COVID-19.
In contrast to other reports that have shaped Centers for Disease Control and Prevention and WHO guidelines for prevention and treatment,5,18,21 in this study, specific comorbidities did not drive COVID-19 rates. Rather, we found inverse associations for COVID-19 with smoking and hypertension. Previous studies of the association of smoking with COVID-19 outcomes have yielded conflicting results, while hypertension emerged as a perceived risk factor for COVID-19 early in the pandemic, potentially influencing the behavior of study participants. As these associations did not persist when assessing COVID-19 risk only among participants with SARS-CoV-2 infection, their link with COVID-19 outcomes remains unclear. Regarding severe COVID-19, diabetes was the sole comorbidity associated with an increased rate; although we did find that having at least 2 comorbidities was associated with a 40% increased rate of severe disease. Overall, our data suggest preexisting immunity, demographics and exposure risks were the strongest variables associated with disease rates.
Limitations
This study has some limitations. It is unclear how infection with specific variants (eg, Omicron) not captured in our study or vaccination would moderate identified risk factors. There were also differences in the severe COVID-19 definition for each trial, warranting additional study of the severe cases within this cohort. The relatively short follow-up time limits understanding of the durability of associations. Any infection and subclinical infection analyses were limited by lack of frequent molecular or anti-N testing, and emerging evidence of variable N antibody responses.54 Differentiation between symptomatic and subclinical infection depended on self-report, which may vary by demographics. In addition, although our analyses considered aggregated study- and region-specific associations of the risk factors, there could still be heterogeneity across the trials (eg, percentage of participants with smoking history) that was not adequately accounted for in the final multivariate models. Furthermore, ascertainment of comorbidities also relied on self-report, excepting obesity, which was measured by BMI. Variable access to health care among participants may have limited self-awareness of comorbid conditions. These considerations suggest a role for individualized risk stratification.
Conclusions
In this secondary cross-protocol analysis, we conducted a detailed assessment of variables associated with rates of COVID-19, severe disease, any SARS-CoV-2 infection, and subclinical SARS-CoV-2 infection obtained systematically from the largest clinical trial cohort enrolled in diverse regions of the world with follow-up spanning multiple waves of the pandemic. Results offer generalizable and precise identification of risk factors and may inform future vaccination policy as SARS-CoV-2 becomes endemic to human populations. Results may also help identify populations at risk in the setting of potential future pandemics.
Notes
Supplement 1.
eMethods.
eFigure 1. Cox Proportional Hazard Scaled Schoenfeld Residual Plots for Each Risk Factor
eFigure 2. Smoothed Hazard Estimates of COVID-19 in Trial Placebo Participants Plotted by Calendar Time and Epidemiologic Trends
eFigure 3. Univariate Cox Proportional Hazard Regression Models for the COVID-19 and Severe COVID-19 Coprimary End Points
eFigure 4. Univariate Cox Proportional Hazard Regression Models for the Subclinical SARS-CoV-2 and Any SARS-CoV-2 Secondary End Points
eFigure 5. Univariate Cox Proportional Hazard Regression Model for the Severe COVID-19 End Point Among Participants With COVID-19
eFigure 6. Multivariate Cox Proportional Hazard Regression Model for the Severe COVID-19 End Point Among Participants With COVID-19
eFigure 7. Univariate Cox Proportional Hazard Regression Model for the COVID-19 End Point Among Participants With SARS-CoV-2
eFigure 8. Multivariate Cox Proportional Hazard Regression Model for the COVID-19 End Point Among Participants With SARS-CoV-2
eFigure 9. Multivariate Cox Proportional Hazard Regression Models With 2-Way Interaction Terms for the COVID-19 End Point
eTable 1. Study Case Definitions
eTable 2. Schedule of Antinucleocapsid Protein Testing Across Protocols
eTable 3. CDC Risk Factors for Severe Disease
eTable 4. Study End Points Stratified by Trial
eTable 5. Variant Counts From COVID-19 Cases
Supplement 2.
Members of the COVID-19 Prevention Network (CoVPN)
References
Full text links
Read article at publisher's site: https://doi.org/10.1001/jamanetworkopen.2023.23349
Read article for free, from open access legal sources, via Unpaywall:
https://jamanetwork.com/journals/jamanetworkopen/articlepdf/2807188/theodore_2023_oi_230691_1688585743.66757.pdf
Citations & impact
Impact metrics
Citations of article over time
Alternative metrics

Discover the attention surrounding your research
https://www.altmetric.com/details/151342991
Article citations
Factors associated with SARS-CoV-2 infection among people living with HIV: Data from the Balearic cohort (EVHIA).
PLoS One, 19(8):e0308568, 07 Aug 2024
Cited by: 0 articles | PMID: 39110761 | PMCID: PMC11305541
Health Status and COVID-19 Epidemiology in an Inland Region of Portugal: A Retrospective Study.
Int J Environ Res Public Health, 21(8):1033, 06 Aug 2024
Cited by: 0 articles | PMID: 39200643 | PMCID: PMC11353782
Safety and feasibility of third-party cytotoxic T lymphocytes for high-risk patients with COVID-19.
Blood Adv, 8(15):4113-4124, 01 Aug 2024
Cited by: 0 articles | PMID: 38885482 | PMCID: PMC11345373
COVID-19 Vaccine Efficacy in Participants With Weakened Immune Systems From 4 Randomized Controlled Trials.
Clin Infect Dis, 79(2):364-374, 01 Aug 2024
Cited by: 0 articles | PMID: 38598658
Dysphagia Is an Underrecognized Risk Factor for Viral Pneumonia Severity.
Dysphagia, 27 Apr 2024
Cited by: 0 articles | PMID: 38676776
Go to all (8) article citations
Data
Data behind the article
This data has been text mined from the article, or deposited into data resources.
BioStudies: supplemental material and supporting data
Clinical Trials (4)
- (1 citation) ClinicalTrials.gov - NCT04611802
- (1 citation) ClinicalTrials.gov - NCT04516746
- (1 citation) ClinicalTrials.gov - NCT04505722
- (1 citation) ClinicalTrials.gov - NCT04470427
Similar Articles
To arrive at the top five similar articles we use a word-weighted algorithm to compare words from the Title and Abstract of each citation.
SARS-CoV-2 Viral Load in the Nasopharynx at Time of First Infection Among Unvaccinated Individuals: A Secondary Cross-Protocol Analysis of 4 Randomized Trials.
JAMA Netw Open, 7(5):e2412835, 01 May 2024
Cited by: 1 article | PMID: 38780941 | PMCID: PMC11117088
Risk Factors for Hospitalization, Mechanical Ventilation, or Death Among 10 131 US Veterans With SARS-CoV-2 Infection.
JAMA Netw Open, 3(9):e2022310, 01 Sep 2020
Cited by: 241 articles | PMID: 32965502 | PMCID: PMC7512055
Folic acid supplementation and malaria susceptibility and severity among people taking antifolate antimalarial drugs in endemic areas.
Cochrane Database Syst Rev, 2(2022), 01 Feb 2022
Cited by: 12 articles | PMID: 36321557 | PMCID: PMC8805585
Review Free full text in Europe PMC
Assessment of the Inclusion of Racial/Ethnic Minority, Female, and Older Individuals in Vaccine Clinical Trials.
JAMA Netw Open, 4(2):e2037640, 01 Feb 2021
Cited by: 101 articles | PMID: 33606033 | PMCID: PMC7896193
Review Free full text in Europe PMC
Funding
Funders who supported this work.
BLRD VA (1)
Grant ID: I01 BX000207
NCATS NIH HHS (1)
Grant ID: UL1 TR002378
NIAID NIH HHS (18)
Grant ID: UM1 AI069424
Grant ID: UM1 AI069470
Grant ID: UM1 AI148450
Grant ID: UM1 AI154468
Grant ID: UM1 AI069481
Grant ID: UM1 AI069494
Grant ID: UM1 AI069412
Grant ID: UM1 AI069534
Grant ID: U01 AI069476
Grant ID: UM1 AI068614
Grant ID: UM1 AI069476
Grant ID: P30 AI045008
Grant ID: UM1 AI068635
Grant ID: UM1 AI148576
Grant ID: U01 AI069470
Grant ID: UM1 AI148575
Grant ID: UM1 AI148685
Grant ID: UM1 AI148689