Abstract
Free full text

Noninvasive Stereotactic Radiotherapy for PADN in an Acute Canine Model of Pulmonary Arterial Hypertension
Visual Abstract
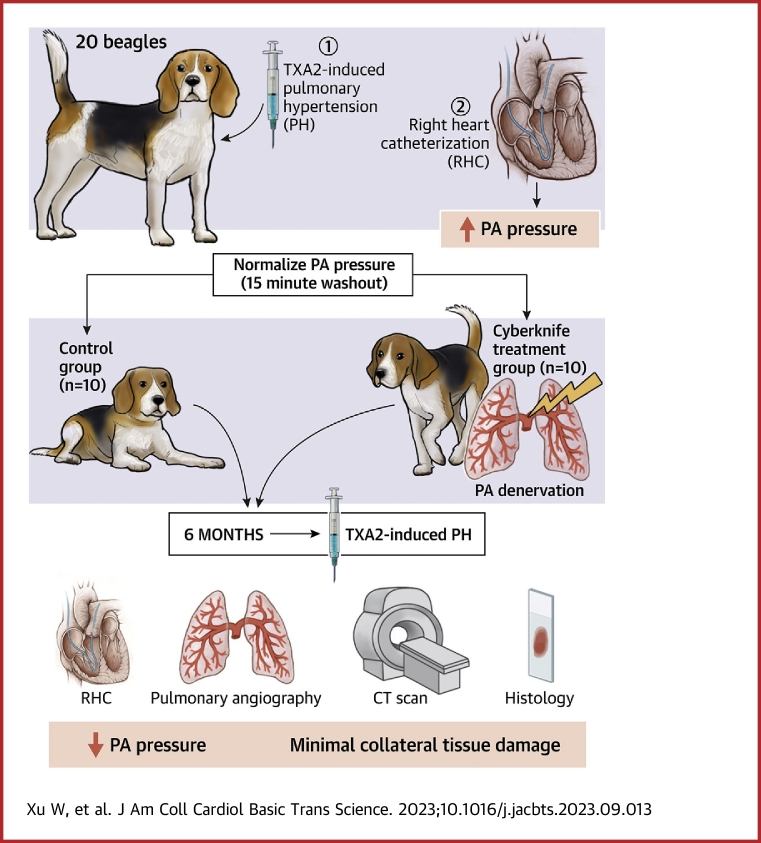
Summary
This study assesses the feasibility, safety, and effectiveness of noninvasive stereotactic body radiotherapy (SBRT) as an approach for pulmonary artery denervation in canine models. SBRT with CyberKnife resulted in reduced mean pulmonary artery pressure, pulmonary capillary wedge pressure, and pulmonary vascular resistance, and insignificantly increased cardiac output. In comparison to the control group, serum norepinephrine levels at 1 month and 6 months were significantly lower in the CyberKnife group. Computed tomography, pulmonary angiography, and histology analysis revealed that SBRT was associated with minimal collateral damage.
Pulmonary hypertension (PH) is a rare but severe disease defined by a resting mean pulmonary artery pressure (mPAP) of ≥20 mm Hg. PH is divided into 5 groups based on similar pathophysiological causes, clinical manifestations, hemodynamic traits, and therapeutic therapy. Pulmonary arterial hypertension (PAH) is the first group characterized by progressive pulmonary artery proliferation and increased right ventricular (RV) pressure.1,2 Targeted medications have been shown to increase functional capacity and hemodynamics and to decrease hospital admissions; however, their impact on mortality is less clear, and residual risk is still significant.3 These therapies focus on the endothelin, nitric oxide, and prostacyclin pathways.4 Reversing the progressive remodeling of the pulmonary artery and RV in patients with PAH is difficult. Given that PAH is progressive, has a high death rate, and has a high hospitalization rate, a novel treatment option is urgently needed.
Pulmonary sympathetic nerve (SN) hyperactivity contributes to the emergence and progression of PAH. Numerous SNs have been widely dispersed in the pulmonary artery and the surrounding connective tissue with indications of enhanced SN firing and circulating catecholamines in both PAH patients and animal models.5, 6, 7 Because of this, various minimally invasive pulmonary artery denervation (PADN) –based approaches, including radiofrequency, ultrasound, and transthoracic PADN, have been developed to destroy afferent and efferent nerves embedded within the pulmonary artery wall.6,8, 9, 10 However, the range of SN histological injury caused by radiofrequency approaches was nevertheless constrained because of the influence of limited penetration distance, intensity of radiofrequency ablation energy, anatomic distribution of SNs, and the requirement of experienced intervention experts.6 Moreover, the late occurrence of pulmonary artery stenosis after radiofrequency-based PADN may be reported based on renal artery denervation (RDN) experience.11 Furthermore, it is necessary to confirm the efficacy of intravascular ultrasonography methods for PADN. Radiofrequency and intravascular ultrasound approaches are also limited by the potential for adverse endothelial lesions and vascular architecture. Surgical procedures are thought to denervate the pulmonary artery more comprehensively but are highly invasive.
A noninvasive stereotactic body radiotherapy (SBRT) technology called CyberKnife can precisely administer large doses of radiation to body targets while causing minimum damage to the normal adjacent tissue.12 CyberKnife has been used for tumor treatments and in other fields, such as the cardiovascular system. SBRT can be used to treat atrial fibrillation (AF) and ventricular tachycardia. Studies have examined the pathological alterations in irradiated lesions in a canine model as well as the viability of isolating the left atrial posterior wall and pulmonary vein using SBRT. This study provides a theoretical basis for the application of SBRT in the treatment of AF.13 Most recently, a limited number of patients with malignant arrhythmias such as ventricular tachycardia and AF have been successfully treated with SBRT in a safe and effective manner.14, 15, 16 Further clinical evaluation to define safety and efficacy in larger populations of patients is needed due to the small number of cases.
SBRT can also be used to treat hypertension. Treatment for hypertension with RDN has produced positive outcomes. However, the traditional catheter-based radiofrequency technique has a higher incidence of restenosis. In animal models, the renal artery SNs have been successfully ablated using stereotactic irradiation.17 SBRT can be a noninvasive alternative to catheter ablation for RDN.
SBRT has been widely used and achieved good results in treating cardiovascular diseases. To date, few studies have investigated the potential value of noninvasive stereotactic radiotherapy for PADN. Therefore, the present study investigates the feasibility, effectiveness, and safety of SBRT as an approach for PADN in an acute canine model of pulmonary hypertension.
Methods
This study was approved by the Animal Use and Management Ethics Committee of the First Affiliated Hospital of Harbin Medical University. The use of animals and all procedures was in agreement with the Guide for the Care and Use of Laboratory Animals, 8th Edition.18 All dogs were raised in the animal center of the First Affiliated Hospital of Harbin Medical University where have a temperature-controlled room at 23 °C under a 12-hour light-dark cycle. All dogs were housed in individual cages and received a standard laboratory diet and filtered water.
Animal Preparation
To evaluate the effects of PADN on PAH, the initial hemodynamic test was performed in which 20 beagle dogs weighing between 10 and12 kg were randomly assigned into 2 groups (a control group [n = 10] and a CyberKnife group [n = 10]) that received radiation doses of 45 Gy. All animals were initially anesthetized by intravenous injection of Nembutal (0.03 mg/kg). Then, they were maintained via intravenous propofol (3 mg/kg) and isoflurane (2% to 3% in 100% O2 via the endotracheal tube). In both groups, venous blood samples were obtained to detect blood norepinephrine (NE) at baseline, 1 month, and 6 months. Pulmonary artery hemodynamic indexes were also found. The concentration of serum NE was measured using an enzyme-linked immunosorbent assay kit (Jian Cheng). At the end of the experimental procedure, all animals were sacrificed, and all lung tissues with pulmonary arterial (PA) vasculature were stored at –80 °C for pathological and molecular research.
Hemodynamic Measurements
After the dog was anesthetized, an 8-F sheath was inserted into the femoral vein, and a Swan-Ganz catheter (Edwards Lifesciences) was positioned at the PA. Then, the thermodilution cardiac output (CO), the heart rate, the right atrial systolic pressure, the right ventricular systolic pressure (RVSP), the pulmonary capillary wedge pressure (PCWP), and the systolic/diastolic and mPAP were measured and calculated via pulmonary vascular resistance: (PVR) = (mPAP – PCWP)/CO. Acute canine models of PH were created by intravenous infusion of a thromboxane A2 agonist (TXA2, U-46619, Cayman) following the measurement of fundamental hemodynamic indices (Figure 1). The dose of infused TXA2 was increased at 5-minute intervals in accordance with the defined protocol (10 μg/mL at 17, 22, 27, 32, and 37 μg/kg/h) based on previous studies.9,19 TXA2 was administered to each animal at the same time and dose. U46619 was administered intravenously, and the dose-dependent elevation of pulmonary artery pressure (PAP) from baseline was significant. Therefore, the mPAP reached its maximum of 25 minutes. To assess the efficacy of PADN, the infusion of TXA2 was repeated after SBRT using the same method.
SBRT Procedure For PADN
The procedural workflow for SBRT is shown in Figure 2. Based on previous studies, we devised a radiotherapy target area that ranged from 2 mm close to the left and right PAs to the center of the major pulmonary artery.8,20 Before PADN, dogs should have a series of computed tomography (CT) scans to identify the target area, including a respiration-correlated 4-dimensional, a native, and a contrast-enhanced CT. In order to exclude the influence of the dog's breathing on the target area, the sum of cardiac and pulmonary motion should be calculated based on the respiration-correlated 4-dimensional CT of dogs. The final target should be expanded to account for motion, setup uncertainty, and delivery uncertainty. The total dose for each dog was 45 Gy. The PADN strategy should ensure that normal surrounding tissue, such as the esophagus, trachea, lungs, and RV, sustain the least amount of damage possible while the target volume receives the maximum amount of dose coverage. SBRT was performed with the CyberKnife (Accuray). Following SBRT, the dogs from the CyberKnife group recovered from the surgery and were looked after for 6 months.
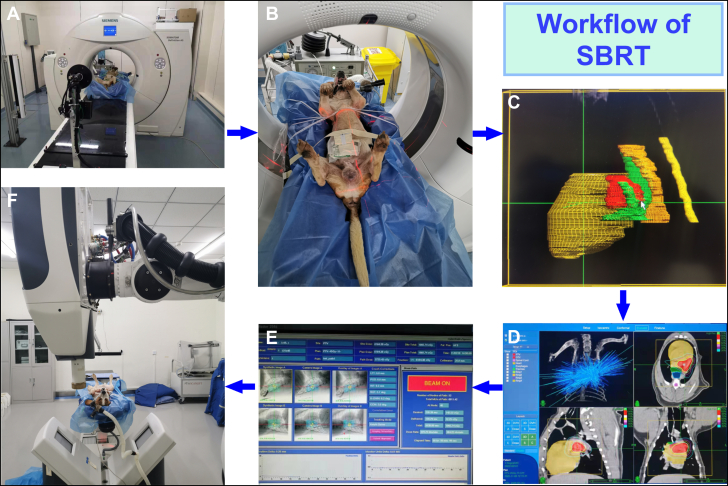
Workflow of Noninvasive SBRT for Pulmonary Artery Denervation
(A) Beagles underwent a series of computed tomography (CT) scans, including native, contrast-enhanced, and respiration-correlated 4-dimensional CT. (B) Based on the CT scan data, the animals were located using lasers and skin markers. (C) The target volume (including the pulmonary artery) was delineated, and the target volume containing motion was generated according to the 4-dimensional CT. The plan target volume was expanded by 2 mm due to mechanical tolerance and setup tolerance. The delineation of organs at risk (OAR) included the heart, trachea, esophagus, spinal cord, and lungs. (D) The prescription dose was 45 Gy. The stereotactic radiosurgery plan was designed to improve the prescription dose coverage of the target volume while minimizing the dose to OAR. The green isodose line corresponds to a 45-Gy prescribed dose. The target area is almost entirely enveloped by 45 Gy whereas the dose of nearby OARs decreases rapidly. (E) Two orthogonal kilovolt x-ray tubes were used for position and image-guided alignment until the error was sub-millimeter and treatment was available. (F) CyberKnife was used for stereotactic body radiotherapy (SBRT).
Terminal CT Examination
A chest contrast-enhanced CT scan and pulmonary angiography were performed 6 months after SBRT to detect potential side effects, including pulmonary artery stenosis, lung damage, or any unpredicted alterations to the surrounding tissues.
Serum NE Determination
After a 12-hour fast, each dog’s venous blood was collected between 7 am and 8 am. Blood samples were collected at baseline, 1 month, and 6 months. They were centrifuged at 3,500 × g for 15 minutes. NE concentration measurement kits were purchased from Tianjin Ruerkang Biological Technology Co, LTD. All procedures followed the manufacturer’s instructions.
Immunohistochemical determination
The dogs' PA, lung tissues, and RV were preserved in 10% formalin and sectioned into 3- to 5-μm-thick sections for paraffinization. The specimens were stained with hematoxylin and eosin (HE) and examined under a light microscope. HE staining was randomly selected from each of the 5 tissue sections and was applied to evaluate the damage to the surrounding tissues of the target area after SBRT.
PAs were dissected and incubated with anti–tyrosine hydroxylase (TH) (Abcam) overnight at 4 °C. After rinsing, the tissue sections were exposed to peroxidase-conjugated goat anti-rabbit immunoglobulin G (1:1000; Abcam) for 10 to 20 minutes at 37 °C. They were then visualized with a 3,3'-diaminobenzidine–based colorimetric method.21,22 The target protein expression was determined by the digital medical image analysis system using the positive cell area density (defined as the positive cell area/total area of the field) (HPISA-1,000, Olympus).
Statistical Analysis
Data are expressed as mean ± SD. Longitudinal differences between groups were evaluated using repeated measures 2-way analysis of variance with Sidak's post hoc correction for multiple pairwise comparisons or unpaired Student t test. P < 0.05 was considered statistically significant. All the analyses were performed with Prism 8 (GraphPad Software).
Results
Twenty beagles were randomly divided into 2 groups including the control group and the CyberKnife group. In the control group, 1 animal suffered an anesthesia accident during a CT scan, and 2 animals died during hemodynamic tests in the CyberKnife group. The remaining animals lived to the endpoint at 6 months.
Acute PH in a canine model
To determine the effect of PADN on PH, an acute canine model of PAH was established that had been very effective in previous studies.9,19 Additionally, we discovered that TXA2 injection could cause a repeatable, dose-dependent increase in PAP (Figure 3). After stopping the TXA2 injection, PAP could recover to normal in 15 minutes.
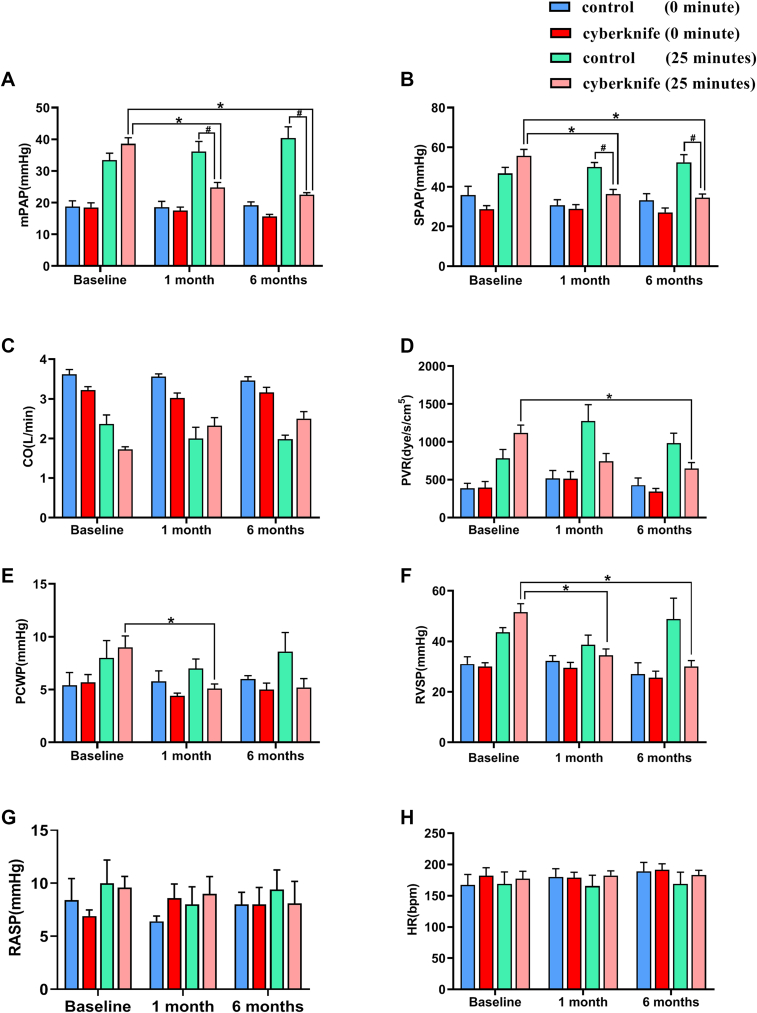
Experimental Study Hemodynamics
(A) Mean pulmonary artery pressure (mPAP) at baseline, 1 month, and 6 months with thromboxane A2 agonist (TXA2) pre- and post-TXA2 procedure for control and CyberKnife-treated animals. (B) Systolic pulmonary artery pressure (SPAP), (C) cardiac output (CO), (D) pulmonary vascular resistance (PVR), (E) pulmonary capillary wedge pressure (PCWP), (F) right ventricular systolic pressure (RVSP), (G) right arterial systolic pressure (RASP), and (H) heart rate (HR) at baseline, 1 month, and 6 months TXA2 agonist pre- and post-TXA2 procedure for control and CyberKnife-treated animals. Data are expressed as mean ± SD and compared with repeated measures analysis of variance. P < 0.05 vs 1 month and 6 months. Control group n = 6. CyberKnife group n = 8.
Design of the stereotactic radiofrequency ablation
Radiofrequency ablation treatment parameters for all dogs are shown in Supplemental Table 1. More than 90% of the planned target volume was covered by the specified dose, with adjacent critical structures — particularly the lungs — experiencing a significant dose falloff. The mean administered dosage to the target tissues was 45 Gy. However, the surrounding target area had a rapid dose decline. The mean ablation target size of bilateral pulmonary arteries was 17.5 ± 2.67 mm. The mean treatment times from beam on to beam off were 85.25 ± 9.05 minutes.
PAP measurements
Right heart catheterization revealed no significant difference in hemodynamics at baseline between the 2 groups (Figure 3). The maximum PAP was attained at 25 minutes after TXA2 injection because the increase in PAP caused by TXA2 was dose-dependent. Similarly, after TXA2 injection, mPAP and systolic pulmonary artery pressure (SPAP) significantly increased in the CyberKnife-treated group and the sham-treated group, reaching their peak levels at 25 minutes. However, compared with the sham group, the mPAP and SPAP were markedly reduced in the CyberKnife group at 1 month and 6 months (Figures 3A and and3B).3B). Similar to PAP, the PVR, PCWP, and RVSP were increased after TXA2 injection and were significantly reduced by CyberKnife treatment at 1 month and 6 months (Figures 3D to 3F). CO was insignificantly decreased during the induction of TXA2 in both groups; SBRT could improve CO (Figure 3C).
SBRT reduces adventitial SN staining
Tyrosine hydroxylase (TH) staining was used to count the SNs in the pulmonary artery adventitia. Expression of the TH protein linked with nerves decreased (Figures 4 and and55).
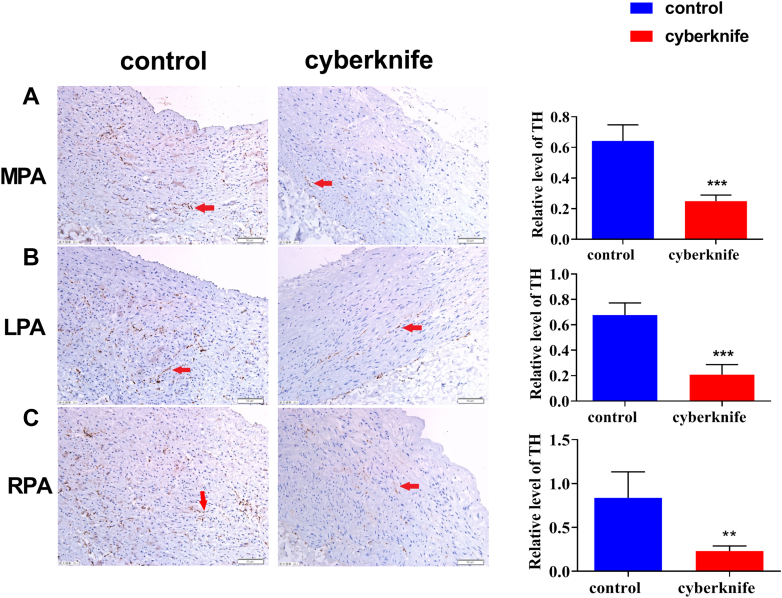
Immunohistochemistry of Pulmonary Artery Nerve
(A) Tyrosine hydroxylase (TH) staining of MPA, (B) LPA, and (C) RPA in control and CyberKnife groups at 6 months. Data are expressed as mean ± SD and compared with unpaired Student t test. P < 0.01.
P < 0.001 vs control groups; n = 6 per group. LPA = left pulmonary artery; MPA = main pulmonary artery; RPA = right pulmonary artery.
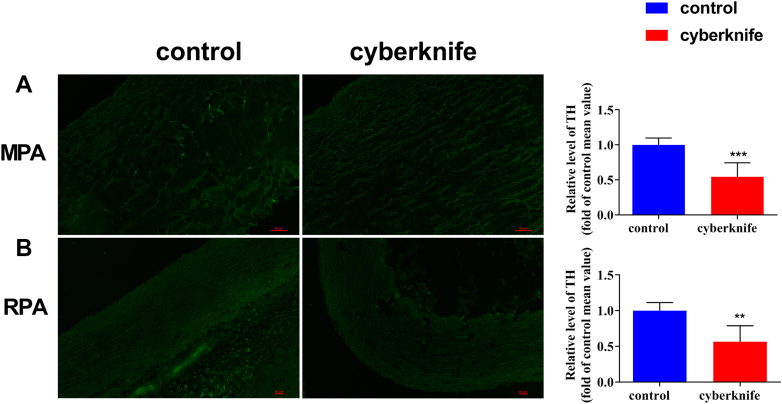
Immunofluorescence of Pulmonary Artery Nerve
(A) Immunofluorescence of MPA in control and CyberKnife groups at 6 months. (B) Immunofluorescence of RPA in control and CyberKnife groups at 6 months. Data are expressed as mean ± SD and compared with unpaired Student t test. P < 0.01,
P < 0.001 vs control groups. n = 6 per group. Abbreviations as in Figure 4.
Sympathetic activity detection
The NE level in the CyberKnife group gradually declined from baseline to 6 months and peaked at 6 months, revealing a significant difference from the control group. In addition, serum NE level was markedly reduced at 6 months compared with that at baseline in the CyberKnife group (Figure 6).
Non–target organ damage assessment
Non–target organ damage was evaluated by histological changes, terminal CT, and pulmonary angiography. HE staining was performed in both groups after animals were sacrificed at 6 months. The CyberKnife group displayed higher inflammatory cell infiltration in the PA and lung tissues than the control group (Figures 7A and and7B).7B). However, there were no differences in RV injury (Figure 7C).
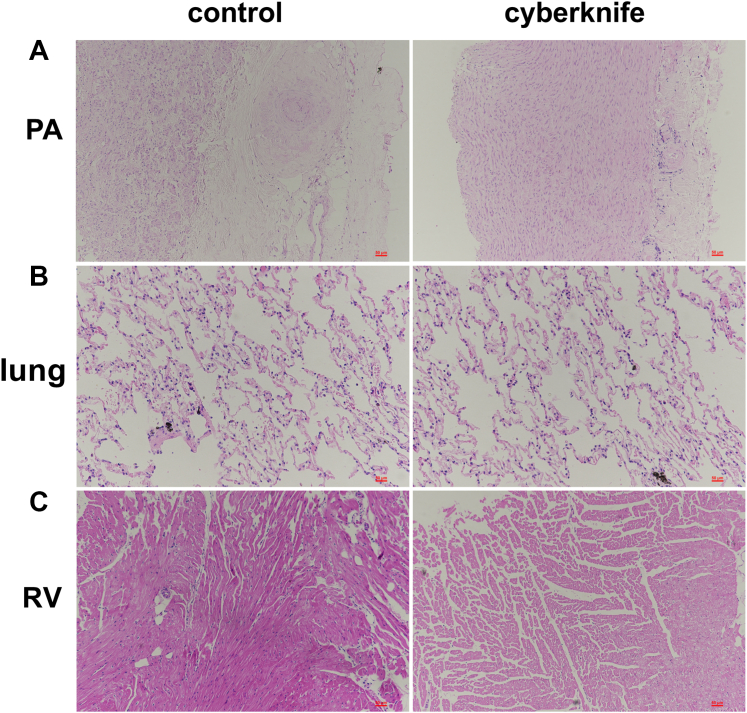
Non–Target Organ Injury
(A) Representative images of pulmonary arteries (PAs), (B) lungs, and (C) right ventricle (RV) were stained with hematoxylin and eosin (HE) in control and CyberKnife groups. HE stain of PA and lungs showed that many inflammatory cells were infiltrated in CyberKnife groups compared with the control group, whereas RV showed no apparent damage.
At baseline and after 6 months in the CyberKnife group, chest CT scans on all beagles were performed. There was no significant surrounding soft tissue damage or lung necrosis at 6 months; only mild to moderate lung texture disorder was observed (Figures 8A and and8B).8B). Meanwhile, pulmonary angiography was performed to assess the degree of PA stenosis. Compared with baseline data, SBRT did not cause PA stenosis (Figures 8C and and8D8D).
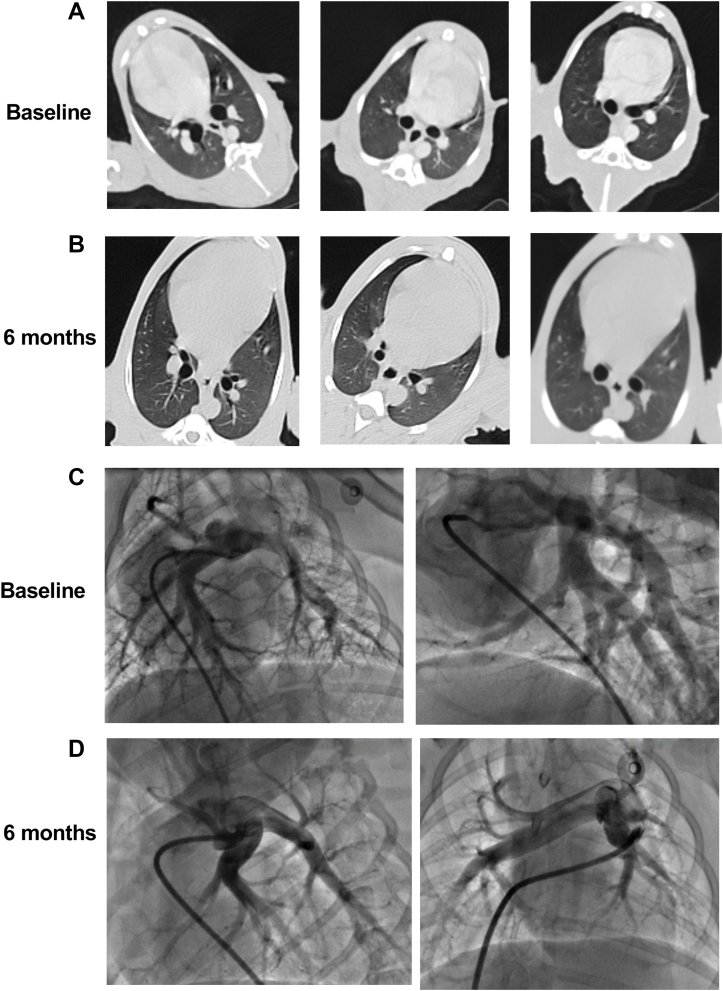
CT and Pulmonary Angiography Evaluation
Representative coronal CT images of the lung at (A) baseline and (B) 6 months in the CyberKnife group. Representative pulmonary angiography images at (C) baseline and (D) 6 months in the CyberKnife group. No significant fibrosis, inflammation, or other changes were observed at the 6-month endpoint. PA stenosis was also not observed 6 months after SBRT in the CyberKnife group. Abbreviations as in Figures 1, ,2,2, and and77.
Discussion
In our study, we have shown that: 1) in this acute canine model of pulmonary hypertension, noninvasive stereotactic radiation for PADN is both feasible and efficacious, and there is evidence of decreased sympathetic activity and complete circumferential PADN; and 2) noninvasive stereotactic radiotherapy for PADN is safe, with the evidence of non-PA stenosis or damage to normal adjacent tissue. This technique may provide an alternative approach for PADN and maybe a new and efficient PAH treatment.
Animal models of PAH
Numerous animal models have been developed to investigate the pathophysiological process of PH. Stenmark et al23 developed an animal model of PH by placing 2-day-old calves at a simulated height of 4,300 m for 2 weeks. The PA circulation of these calves exhibited features resembling chronic PH in newborn infants.23 However, the third kind of pulmonary hypertension, hypoxia-induced PH, may not be linked to the SN. Earlier PADN experiments have shown efficacy using a balloon occlusion model. However, the mechanism by which PH is produced in that model is still debatable; instead of sympathetic hyperactivation, balloon occlusion promotes PAP by activating a pulmo-pulmonary baroreceptor reflex.24
The pathogenesis of PAH involves an SN overactivity leading to an imbalance between pulmonary vasoconstriction and vasodilation, which leads to increases in PAP. TXA2, a powerful antacid that causes platelet aggregation and vasoconstriction, has been shown in experimental and clinical studies to have elevated plasma levels in PAH-affected humans and animals.25,26
Previous studies have found that an intravenous infusion of TXA2 significantly elevated mPAP, SPAP, and PVR.9,19 Therefore, we developed an acute PAH model using intravenous TXA2. In our study, TXA2 significantly elevated mPAP, SPAP, PCWP, and PVR in a dose-dependent manner, and insignificantly decreased CO. One of the main limitations of this model is the only slight reduction in CO. The elevated PAP and PVR in this model are caused by the severe contraction of the pulmonary vessels rather than the progressive remodeling of the pulmonary vessels. TXA2 can cause systemic arterial constriction and lead to a decrease in CO. Together, pulmonary vasoconstriction and decreased CO cause an increase in PCWP. Examining the pressure and hemodynamic changes in the acute phase of the TXA2 model provides valuable insight into the mechanism of PAH despite not completely replicating its pathophysiology.
PADN treatment for PAH
The major pathophysiological mechanisms of PAH include endothelial dysfunction within appropriate vasoconstriction, pulmonary vascular remodeling, and in situ thrombosis.1 Many studies have suggested that the sympathetic nervous system activation plays a critical role in the clinical deterioration of patients with PAH with the evidence of increased muscle SN activity, higher vessel SN endings, circulating plasma noradrenaline levels, and markers of chronic sympathetic activation.5,27, 28, 29, 30 The interaction of these neurohormones with their receptors contributed to the advancement of PAH. Therefore, single hormone receptor blockers cannot delay PAH progression.31 In addition, international PH guidelines also do not recommend beta-blockade treatment for PAH. As a new approach for PAH treatment, PADN has been proven feasible in animal models.9,10 Similar to earlier research, we used a vasoconstriction-based acute model of PAH to show that SBRT for PADN can significantly decrease PAP and PVR while increasing CO.
In addition, some studies have reported that the renin-angiotensin-aldosterone system may also play a vital role in the pathogenesis of PAH.32 Deactivating the sympathetic bundles of the renal nerves could improve the activity of the sympathetic nervous system and positively impact the hemodynamic conditions in patients with PAH. A study has shown that renal denervation technology is ineffective in producing significant acute pulmonary or systemic hemodynamic changes in the TXA2-induced PH model. Additionally, it was found ineffective in reversing the increase in PAP induced by TXA2.33 TXA2 is a direct vasoconstrictor, and renal artery denervation can only inhibit renal artery contraction but does not affect PA contraction.
SBRT for PADN treatment
According to previous research, PADN is effective in animal models of PAH And in patients with PAH.6,8, 9, 10,34,35 Hence, various PADN methods have emerged, including ultrasound-, transthoracic-, and catheter-based radiofrequency PADN. SBRT, a noninvasive treatment for tumors, can be used for PADN. This treatment concentrates radiation energy to the specific target area with sub-millimeter accuracy while minimizing the dose to nearby organs. In this study, we present evidence that, in an acute animal model of PAH, full pulmonary SN injury caused by noninvasive stereotactic pulmonary nerve radiofrequency ablation could reduce PAP.
Multiple techniques for PADN have been developed. Among these technologies, catheter-based PADN has been the most widely studied method. However, some academics have questioned the efficacy of catheter-based percutaneous PADN because they believe that it cannot achieve sufficient denervation.6,36 In a study comparing surgical and catheter-based PADN, Garcia-Lunar36 reported that radiofrequency ablation produced incomplete denervation, with only focal damage to vascular adventitial nerve fibers. Numerous parameters, such as the distribution and depth of the SNs in patients, the thickness of the PA, and the ablation energy, could affect the efficacy of denervation. In contrast, surgical PADN resulted in total PADN with histological evidence of extensive damage to circumferential, 4-quadrant nerve fibers and nerve axons in the PA adventitia. However, it has been shown that surgical PADN produces more thorough denervation than a catheter technique. Moreover, patients with PAH, particularly those with severe heart failure, may not be candidates for general anesthesia and thoracic surgery. SBRT, a noninvasive procedure, can produce complete, circumferential denervation. Therefore, it may be more suitable for PADN.
Increased circulating serum NE levels and markers of chronic sympathetic activation are always used to measure the severity of PAH.29,30 Neurohormonal activity markers are elevated in PAH patients and are associated with adverse clinical events.27 The pulmonary vasculature is highly innervated, releasing and metabolizing >40% of circulating catecholamines.37 Our findings indicated that PADN with SBRT significantly decreased serum NE levels 1 and 6 months after treatment. The result is consistent with earlier studies. Beyond that, we observed histological nerve damage and a marked reduction by TH stain. In contrast to catheter-based PADN, which causes instantaneous cell necrosis, it takes longer for radiation-induced injuries to appear.38 Therefore, we observed that the damage to the pulmonary nerve and reduced serum NE in 6 months was more evident than in 1 month and at baseline.
In addition to the efficiency of PADN, we found radiation-induced damage to the PA and adjacent organs. The present investigation shows the safety of SBRT to the PA and associated organs, including the esophagus, windpipe, lung, and RV, based on histological evidence of intact tissue and the presence of only minor damage on chest CT imaging.
Study limitations
This study provides evidence that SBRT is a realistic, safe, and effective method for treating PADN and suggests a new therapy option for individuals with PAH. However, some limitations must be explained. First, this acute canine model of PH may not accurately represent the clinical features of PH patients. Second, the PAs used in this study may not accurately represent the characteristics of human PAs with PAH, which may react differently to radiation. Last, the optimal dose for PADN has not been determined, and the dose used in this study may cause damage to non–target organs in humans.
Conclusions
SBRT is a feasible, effective, and safe approach for PADN in this acute canine model of PAH, with evidence of a complete, circumferential pulmonary nerve injury, inhibition of sympathetic activity, and minimal damage to adjacent organs. This method is an alternative to catheter-based PADN because it is safer and more effective. However, additional animal studies are required to determine acceptable radiation doses, and additional preclinical experience is needed to optimize the PADN method before it can be implemented in clinical practice.
Funding Support and Author Disclosures
Supported by grants from the State Key Program of National Natural Science Foundation ofChina (No.81830012, No82330014 to Dr Y. Li); National Natural Science Foundation of China (No.82070336 to Dr Y. Li); National Natural Science Foundation of China (No. 81974024 to Dr Gong)Youth Foundation of National Natural Science Foundation of China (No. 82100507 to Dr Zhang). This study was approved by the Animal Use and Management Ethics Committee of the First Affiliated Hospital of Harbin Medical University. The use of animals and all procedures were in agreement with the Guide for the Care and Use of Laboratory Animals (NIH Publication 2011; eighth edition). All other authors have reported that they have no relationships relevant to the contents of this paper to disclose.
Footnotes
The authors attest they are in compliance with human studies committees and animal welfare regulations of the authors’ institutions and Food and Drug Administration guidelines, including patient consent where appropriate. For more information, visit the Author Center.
Appendix
For a supplemental table, please see the online version of this paper.
References
Articles from JACC: Basic to Translational Science are provided here courtesy of Elsevier
Citations & impact
Impact metrics
Alternative metrics

Discover the attention surrounding your research
https://www.altmetric.com/details/160700183
Article citations
Pulmonary Hypertension: Pharmacological and Non-Pharmacological Therapies.
Life (Basel), 14(10):1265, 04 Oct 2024
Cited by: 0 articles | PMID: 39459565 | PMCID: PMC11509317
Review Free full text in Europe PMC
Similar Articles
To arrive at the top five similar articles we use a word-weighted algorithm to compare words from the Title and Abstract of each citation.
Sympathetic innervation of canine pulmonary artery and morphometric and functional analysis in dehydromonocrotaline-induced models after pulmonary artery denervation.
Interact Cardiovasc Thorac Surg, 31(5):708-717, 01 Nov 2020
Cited by: 0 articles | PMID: 33057705
Noninvasive Stereotactic Radiotherapy for Renal Denervation in a Swine Model.
J Am Coll Cardiol, 74(13):1697-1709, 01 Oct 2019
Cited by: 10 articles | PMID: 31558254
Pulmonary Artery Denervation Significantly Increases 6-Min Walk Distance for Patients With Combined Pre- and Post-Capillary Pulmonary Hypertension Associated With Left Heart Failure: The PADN-5 Study.
JACC Cardiovasc Interv, 12(3):274-284, 23 Oct 2018
Cited by: 40 articles | PMID: 30732732
Current status of pulmonary artery denervation.
Front Cardiovasc Med, 9:972256, 03 Oct 2022
Cited by: 1 article | PMID: 36262207 | PMCID: PMC9573987
Review Free full text in Europe PMC
Funding
Funders who supported this work.