Abstract
Free full text

Preventive nasal administration of flagellin restores antimicrobial effect of gentamicin and protects against a multidrug-resistant strain of Pseudomonas aeruginosa
ABSTRACT
The increasing prevalence of multidrug-resistant Pseudomonas aeruginosa (PA) is a significant concern for chronic respiratory disease exacerbations. Host-directed drugs, such as flagellin, an agonist of toll-like receptor 5 (TLR5), have emerged as a promising solution. In this study, we evaluated the prophylactic intranasal administration of flagellin against a multidrug-resistant strain of PA (PAMDR) in mice and assessed the possible synergy with the antibiotic gentamicin (GNT). The results indicated that flagellin treatment before infection decreased bacterial load in the lungs, likely due to an increase in neutrophil recruitment, and reduced signs of inflammation, including proinflammatory cytokines. The combination of flagellin and GNT showed a synergistic effect, decreasing even more the bacterial load and increasing mice survival rates, in comparison to mice pre-treated only with flagellin. These findings suggest that preventive nasal administration of flagellin could restore the effect of GNT against MDR strains of PA, paving the way for the use of flagellin in vulnerable patients with chronic respiratory diseases.
INTRODUCTION
Pseudomonas aeruginosa (PA) is one of the leading causes of hospital-acquired infections globally and the most common pathogen associated with respiratory chronic illnesses, such as cystic fibrosis (CF) (1,–3). Overuse of antimicrobial agents during the past decades has resulted in the emergence of PA-resistant strains, particularly to β-lactams, carbapenems, and aminoglycosides antibiotics (4). Multidrug-resistant (MDR) strains of PA are even harder to eradicate and cause higher mortality rates (5, 6). As a consequence, the World Health Organization (WHO) has included MDR strains of PA in a list of pathogens requiring urgent attention for the development of new therapeutic strategies (7).
Approaches that can enhance and rescue antibiotic efficacy are of significant interest and are increasingly being studied (8). In this context, host-directed molecules in combination with antibiotics are a promising strategy for preventing infections and restoring antibiotic effectiveness. Flagellin, a structural component of the flagellum of Gram-negative bacteria, has emerged as a promising host-directed immunomodulatory drug (9). In particular, the recombinant form of flagellin (flagellin174-400), which lacks its antigenic part, has been described for its immuno-stimulatory capacity and antimicrobial properties against intestinal infections caused by Enterococcus faecium, Clostridium difficile, and Escherichia coli (10,–12) and against respiratory infections caused by Streptococcus pneumoniae (13).
In the airways, the mechanism of action of flagellin174-400 (hereafter only designated as flagellin) is well-described. Flagellin binds to toll-like receptor 5 (TLR5), a pattern recognition receptor (PRR) present in epithelial cells (ECs), alveolar macrophages (AM), conventional dendritic cells (cDCs), and lymphocytes (14). This interaction strongly activates the transcription factor nuclear factor-kappa B (NFκB), mitogen-activated protein kinases (MAPK), and interferon (IFN)-regulatory factor (IRF) pathways, resulting in the transcription of genes encoding several immune mediators (15). This includes chemokines such as CXCL1, CXCL2, CXCL5, CCL2, and CCL20; cytokines such as interleukin-6 (IL-6), granulocyte-macrophage colony-stimulating factor (GM-CSF), and interleukin-1 beta (IL-1β) as well as mucins; and several antimicrobial peptides such as β-defensins (16,–19).
It has been demonstrated that flagellin triggers a short-lived response (peaking 2 h after administration and decaying 2–3 h after the peak) due to strong feedback regulatory mechanisms (20). This controlled response is crucial because it allows the inflammation to resolve toward homeostasis. Interestingly, the same study also shows that a second stimulus with flagellin (either with soluble protein or PA infection) leads to a less intense proinflammatory state, suggesting that a single prophylactic flagellin instillation can reduce the inflammatory response to PA (20).
Previous studies have also shown that nasal administration of flagellin has a synergistic effect with the antibiotic amoxicillin in controlling antibiotic-resistant pneumococcal infections (13). Other data have suggested that prophylactic nasal administration of flagellin can decrease the bacterial load of pigs infected with the P. aeruginosa (PAK) strain (20). However, flagellin has never been tested in combination with an antibiotic to fight PA and was never evaluated against a MDR strain of PA.
Here, using an in vivo mouse model, we show that although prophylactic flagellin alone attenuates infection caused by a MDR strain of PA (PAMDR), its combination with the antibiotic gentamicin (GNT) leads to a strong decrease in bacterial load in the lung and a significant reduction in cell infiltration and inflammatory cytokines. Moreover, mice receiving flagellin in combination with GNT showed a 100% survival rate, a result that GNT alone was unable to provide against PAMDR.
MATERIALS AND METHODS
Microbial strain and culture conditions
P. aeruginosa multidrug-resistant strain was isolated from a clinical patient and kindly provided by Dr. Katy Jeannot (CHRU Besançon). This strain is multidrug-resistant (denominated in this study as PAMDR), including a resistance to gentamicin (MIC >4 µg/mL), and its characterization is provided in Table S1 [done by microbiology service of CHRU Bretonneau (Tours)] and Fig. S1. P. aeruginosa PAO1 strain was kindly provided by Dr. Sophie Bleves (CNRS & Aix-Marseille Université, Laboratoire d’Ingénierie des Systèmes Macromoléculaires (UMR7255), Institut de Microbiologie de la Méditerranée)
Flagellin
The custom-designed flagellin used in the present study, that is, FLAMOD (recombinant flagellin FliC174–400 as described in (21) and harboring one extra amino acid at the N terminus), is derived from Salmonella enterica serovar Typhimurium FliC. It was produced in inclusion bodies in Escherichia coli by the Vaccine Development department at Statens Serum Institut, Denmark. FLAMOD (in this work only denominated flagellin) was purified by filtration and chromatography and resuspended in NaPi buffer (10 mM phosphate, 145 mM NaCl, and polysorbate 80 0.02% (w/v) pH 6.5). The immunostimulatory activity was validated using the HEK-Dual hTLR5 cell assay (Invivogen). The low endotoxin content in the protein preparation was assessed with a Limulus assay (Pyrochrome, kinetic LAL assay from Associates of Cape Cod Inc.).
Susceptibility testing
Broth microdilution method was used for the determination of minimal inhibitory concentration (MIC) of gentamicin against PAMDR. Minimal inhibitory concentrations were determined in accordance with the guidelines of the Clinical and Laboratory Standards Institute (CSLI). Briefly, PA strain was cultured on Tryptic Soy Agar (TSA) plates overnight. Three isolated colonies were suspended in 3 mL of LB medium and grown overnight at 37°C, under agitation (200 rpm). Then, several dilutions of this fresh suspension were prepared and incubated at 37°C for 4 h, under agitation (200 rpm). The suspension with OD600 between 0.3 and 0.6, representing the exponential phase of growth, was centrifuged for 10 min at 3,000 × g. Bacteria were suspended in MH medium to obtain approximately 2 × 105 CFU/mL (CFU for colony forming unit). The inoculum size was verified by plating 5-fold dilutions on TSA plates and incubating overnight at 37°C for CFU counts. Hundred microliters/well of the bacterial suspension were inoculated into 96-well microtiter plate, and 100 µL/well of MH (control) or gentamicin was added in duplicate for each condition. The microtiter plate was incubated in a plate reader (TECAN Infinite 200) for 24 h at 37°C. The absorbance at OD600 was read at 30 min intervals.
Animal care and handling
C57Bl/6 female mice were purchased from the Centre d'Elevage R. Janvier (Le Genest Saint-Isle) and were used at about 8 weeks of age. All procedures were in accordance with the European animal welfare regulations. All mice were housed under specific pathogen-free conditions at the PST “Animaleries” animal facility (Université de Tours) and had access to food and water ad libitum. All experiments complied with the French government’s ethical and animal experiment regulations (APAFIS#201604071220401 .V2-4885 and 2016111512369894 V3 - 7590).
Mice were treated with 10 µg of recombinant flagellin (FLAMOD) 24 h before infection with 5 × 105 or 8 × 105 CFU/mouse of PAMDR by the nasal route (Fig. 1A). One hour post-infection, mice were treated by intraperitoneal injection (i.p.) with 15 mg/kg of gentamicin purchased from Sigma Aldrich (Sant-Quentin Fallavier). The choice of gentamicin dose was based on previous experiments in vivo, showing that a single dose of 15 mg/kg i.p. was the minimum dose required to decrease PAMDR bacterial load (in mice infected with 105 CFU/mouse). Sixteen hours after challenge with PAMDR, mice were sacrificed, and airways were washed four times with 0.5 mL of phosphate buffered saline (PBS) for bronchioalveolar lavage (BAL) collection. After centrifugation at 400 × g for 5 min, BAL fluids were stored at −80°C for subsequent measurement of inflammatory mediators, and pellets were recovered in PBS 2% fetal bovine serum (FBS). The pellet was resuspended and filtered, and erythrocytes were discarded using a red blood cell lysis buffer. Leukocytes were counted and analyzed by flow cytometry. After BAL recovery, the lungs were perfused with 10 mL PBS injected into the heart. The lungs were photographed for inflammation assessment based on lung morphology and color. The right lungs were homogenized with 1 mL of PBS using the Gentle MACSTM Octo Dissociator (Miltenyi Biotech), and 100 µL of lung suspension was plated for bacterial count.
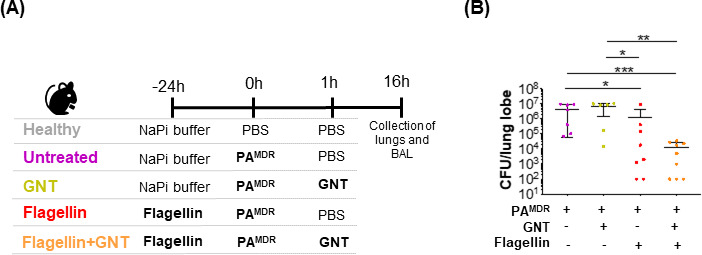
Timeline for the treatment and infection of mice and bacterial load 16 h post-infection. (A) Tweenty-four hours before infection mice in group “untreated” (n = 7) and “GNT” (n = 6) received NaPi buffer and mice in groups “flagellin” (n = 8) and “flagellin + GNT” (n = 9) received FliC (10 µg), resuspended in NaPi buffer, by the nasal route. At T0, all animals (with the exception of controls in “healthy” group) were intranasally infected with 5 × 105 CFU of a MDR strain of P. aeruginosa. One hour after infection, mice in group GNT and flagellin + GNT received GNT 15 mg/Kg by intraperitoneal injection. Healthy mice were used as control (group “healthy”). All mice were sacrificed 16 h p.i.. (B) Colony forming units (CFU)/lung lobe were counted in all infected mice. Each symbol represents an individual animal in two independent experiments. Differences between groups were analyzed with Mann-Whitney test. Data are reported as mean ± SEM. *P < 0.05, **P < 0.01, ***P < 0.001, ****P < 0.0001
Analysis of synergy between flagellin and gentamicin
The effect of treatments (standalone flagellin, standalone GNT, or combination flagellin +GNT) against PAMDR was quantified as the percentage of bacterial growth (%growth), corresponding to the ratio of the mean bacterial load in the lungs of infected and treated mice to that of infected and untreated mice (control). For example, the effect of treatment A was calculated as follows: %growth[A] = (mean CFU[A]/mean CFU[control]) ×100. The predicted additive effect (or predicted %growth) of combination treatment was calculated as described previously (22). Briefly, the predicted %growth of a treatment combining compounds A and B is the product of the experimentally defined %growth values for each standalone treatment (predicted %growth[A + B] = %growth[A] × %growth[B]). If the experimental %growth for the combination treatment is lower or higher than the predicted %growth, then the two drugs are synergistic or antagonistic, respectively. When the experimental and predicted %growth values are identical, the two drugs’ effects are additive.
Flow cytometry analysis
Bronchioalveolar lavage from all mice was dispensed into round-bottomed 96-well plates and were centrifuged at 500 g at 4°C for 5 min. Samples were further stained using specific antibodies and appropriate isotype controls. To identify total leukocytes, neutrophils, total dendritic cells, and alveolar macrophages, cells were stained with a lineage cocktail containing anti-CD45 (APCVio770 - clone 30-f11, BD cat.no. 45–04551-82), anti-CD11b (PE - clone M1/70, BD cat.no. 553311), anti-CD11c (PEVio770 - (clone N418, Bio cat.no. 117318), anti-Ly6G (APC - clone 1A8, BD cat.no. 5605599), anti- SiglecF (VioBlue - clone E50-2440, BD cat.no. 565934), anti-MHCII (PerCP - clone AF6-120.1, BD cat.no. 46–5320-82), and LIVE/DEAD Fixable Aqua Dead Cell Stain Kit (Thermo Fisher Scientific, cat.no. L34966). Flow cytometry data were acquired on a MACSQuant Analyzer, and analyses were performed using the VenturiOne software according to the gating strategy described in Fig. S2.
ELISA
BAL was centrifuged for 5 min at 500 g, and the supernatants were stored at −80°C. DuoSet ELISA (Mouse IL-6, IL-1β, TNF-α, and GM-CSF) was performed according to the manufacturer’s (R&D Systems) instructions.
Statistical analysis
Sample size estimation was based on previous animal studies conducted by our group using P. aeruginosa-infection mice model. There was no randomization or blinding procedure. Statistical analyses were performed using GraphPad Prism. Data are reported as mean ± SEM. Statistical values, including the number of replicates and the statistical test used, can be found in the figure legends. *P < 0.05, **P < 0.005, ***P < 0.0005, ****P < 0.0001.
RESULTS
The combination of preventive flagellin and the antibiotic gentamicin displays synergistic therapeutic activity against a MDR strain of P. aeruginosa
To evaluate the impact of preventive intranasal flagellin in combination with the antibiotic gentamicin against PAMDR, we compared mice that received both prophylactic flagellin (24 h before infection) and GNT 1 h post-infection (“flagellin +GNT group”) with groups of mice that received either prophylactic flagellin alone (“flagellin group”) or mice only treated with GNT (“GNT group”) or infected non-treated mice (“untreated group”). We also considered animals that were neither treated nor infected as healthy (“Healthy group”) (see Fig. 1A). We assessed bacterial load and inflammatory status in the BAL fluids of these mice 16 h post-infection (i.e., 40 h after flagellin administration).
As expected, infected untreated mice presented a high bacterial load in the lungs 16 h after PAMDR challenge (4.5 × 106 CFU ±1.7×106) (see Fig. 1B). Although GNT treatment was not able to significantly decrease the bacterial load of infected mice, prophylactic administration of flagellin significantly reduced PAMDR CFU (P < 0.05). Interestingly, when mice received the combination of flagellin and GNT, they had much lower bacterial CFU (a 400-fold reduction compared with infected non-treated mice; P < 0.001). This result suggests a significant therapeutic advantage for the combination treatment compared with flagellin or GNT treatments alone.
When two drugs are administered in combination, their effect may have an additive mode of action or they may work in synergy to improve the host’s condition. Hence, the interaction between two drugs is classified as synergistic when the total effect of the two drugs combined is greater than that predicted by their individual potencies. Using the equation described in the Materials and Methods, the expected percentage of bacterial growth when combining flagellin and GNT should be 9.4% (55% growth for GNT x 17% growth for flagellin = 9.4%). However, flagellin +GNT combination therapy led to only 0.2% of PAMDR growth compared with the control (infected untreated mice). Since the experimental percentage of growth is lower than the theoretically predicted value, there is a synergistic effect between flagellin and GNT in controlling P. aeruginosa growth.
Preventive intranasal administration of flagellin decreases proinflammatory cytokines and cell infiltration but increases neutrophils and dendritic cells in the BAL of PAMDR-infected mice
Using the same experimental design as described above, we examined the inflammatory status of mice. We analyzed the macroscopic aspect of their lungs (Fig. 2A), immune cell populations recruitment (Fig. 2B), and the secretion of proinflammatory cytokines in BAL (Fig. 2C), at 16 h post-infection, for all experimental groups.
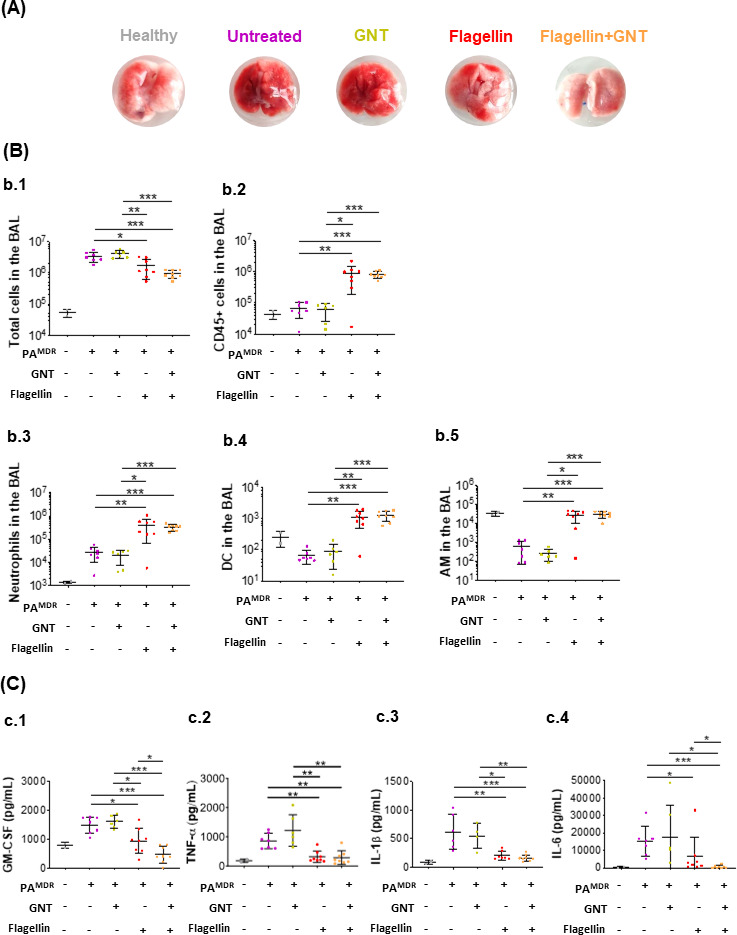
Macroscopic aspect of lungs, immune cell population recruitment, and the secretion of proinflammatory cytokines in BAL. Tweenty-four hours before infection with 5 × 105 CFU of a MDR P. aeruginosa strain, mice were treated or not with flagellin (10 µg) intranasally. Then, 1 h post-infection, mice were treated intraperitoneally or not with Gentamicin (GNT, at 15 mg/Kg body weight). All mice were sacrificed 16 h p.i.. Lungs were perfused for visual assessment of inflammation (A), and BAL fluids were collected to determine the number of immune and inflammatory cells by flow cytometry (B), and the levels of GM-CSF, TNF-α, IL-1β, and IL-6 (C). All data are represented as the mean ± SEM and are cumulative of two independent experiments. The number of mice per group is indicated by symbols [gray (n = 2), purple (n = 7), green (n = 6), red (n = 8), and orange (n = 8)]. Statistical analysis was performed using the Mann-Whitney test. *P < 0.05, **P < 0.01, ***P < 0.001, .****P < 0.0001
Figure 2A shows the difference between representative lungs of a mouse from each group. As expected, untreated mice infected with PAMDR showed a higher extent of gross pathology and hemorrhage. Interestingly, mice only treated with GNT experienced similar macroscopic lung pathology than did the untreated mice. Although animals pre-treated with flagellin before infection exhibited a clear reduction in hemorrhage, the reduction was even more reduced in animals that received the dual therapy of flagellin and GNT.
The total number of cells and leukocytes (CD45+) in the BAL (Fig. 2B.1 and 2B.2, respectively) was analyzed by flow cytometry using the gating strategy described in Fig. S2. We observed that animals treated with flagellin, with or without GNT, presented significantly fewer cells in the BAL at 16 h post-infection, compared with infected non-treated or those only treated with GNT (P < 0.05 for group flagellin vs group untreated and P < 0.001 for group flagellin + GNT vs group GNT). Interestingly, among the cells present in the BAL, significantly more were CD45 +immune cells for the animals that received preventive flagellin (P < 0.01 for group flagellin vs group untreated and P < 0.001 for group flagellin + GNT vs group GNT).
According to the study of Fougeron et al. (23), the number of neutrophils and total DCs in the lungs of mice remain elevated for approximately 72 h after being challenged nasally with flagellin. In this context, we investigated both immune cell populations (neutrophils identified as CD45+CD11b+Ly6G+ and total DCs as CD45+CD11c+SiglecF-MHCII+) in the BAL (Fig. 2B.3 and 2B.4, respectively). As expected, P. aeruginosa infection induced neutrophil recruitment to the airways. The mucosal delivery of flagellin (in combination or not with GNT) induced an even higher increase in the number of neutrophils (P < 0.01 for group flagellin vs group untreated and P < 0.001 for group flagellin + GNT vs group GNT). Flagellin instillation also caused an increase in total DCs (P < 0.01 for group flagellin vs group untreated and P < 0.001 for group flagellin + GNT vs group GNT). Regarding AM (identified as CD45+CD11c+SiglecF+; Fig. 2B.5), infection with PAMDR decreased the number of this cell population in BAL (a 5-fold reduction compared with the non-infected group) and treatment with the antibiotic GNT was not enough to revert such drop. Pre-treatment with flagellin (in combination or not with GNT) preserved the level of AM (P < 0.01 for group flagellin vs group untreated and P < 0.001 for group flagellin + GNT vs group GNT).
Sixteen hours after challenge with PAMDR, infected non-treated animals presented high levels of IL-6, tumor necrosis factor alpha (TNF-α), GM-CSF, and IL-1β 16 h post-infection (Fig. 2C). Although GNT-treated mice also presented high proinflammatory cytokines, mice that received flagellin (in combination or not with GNT) showed a significant reduction for all tested cytokines (P < 0.01 or P < 0.001). Thus, flagellin pretreatment reduced the expression of proinflammatory cytokines triggered by PAMDR infection. The combination of flagellin and GNT fully restores the level of pro-inflammatory mediators released from the lungs of PAMDR -infected mice to a homeostatic basal level.
Combination of prophylactic flagellin and therapeutic gentamicin protects mice against a lethal dose of a MDR strain of P. aeruginosa
We evaluated the effectiveness of the flagellin + GNT combination treatment in protecting mice against a lethal PAMDR infection. To this end, we monitored mice survival over 60 h post-bacterial infection (Fig. 3A). As anticipated for a MDR strain of PA, most infected non-treated mice succumbed to the infection within 24 h (Fig. 3B). Similarly, the mice only treated with GNT showed similar susceptibility to bacterial infection. However, prophylactic flagellin treatment alone was sufficient to significantly protect the mice against the PA infection (80% survival for group “flagellin” vs 20% survival for group “GNT”, P < 0.001). Moreover, the combination of prophylactic flagellin with therapeutic GNT resulted in full protection compared with non-treated animals (Fig. 3B).
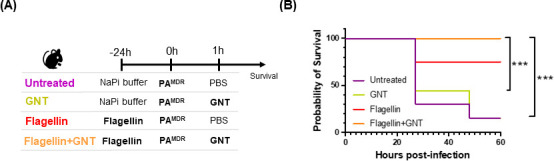
Timeline for treatment and infection of mice and survival study. (A) Tweenty-four hours before infection, C57Bl/6 mice received flagellin (10 µg) [red (n = 5) and orange (n = 7) groups] resuspended in NaPi buffer or only NaPi buffer [purple (n = 7) and green (n = 7) groups] by the nasal route. At T0, all animals were intranasally infected with 8 × 105 CFU of a MDR strain of P. aeruginosa. One hour after infection, mice received GNT 15 mg/kg by intraperitoneal injection (green and orange groups) or PBS (purple and red groups). (B) Animal survival was monitored daily. All data are represented are cumulative of two independent experiments. Statistical analysis was performed using the log-rank (Mantel-Cox) test. *P < 0.05, **P < 0.01, ***P < 0.001, .****P < 0.0001
DISCUSSION
In the present study, we used an in vivo mouse model to investigate the effect of prophylactic nasal administration of flagellin in combination, or not, with the antibiotic GNT against a MDR strain of P. aeruginosa (PAMDR). We demonstrated that (i) a single prophylactic respiratory administration of flagellin was sufficient to decrease bacterial load in the lungs, and when combined with GNT 1 h post-infection, this reduction was even more accentuated, which suggests a synergistic effect between the two treatments; (ii) pre-treatment with flagellin, in combination or not with GNT, reduced inflammation but increased the number of neutrophils, total DCs, and maintained AM levels in the BAL, 16 h post-infection.; and (iii) combination of prophylactic flagellin and GNT 1 h post-infection led to 100% survival, contrary to the control groups.
Several studies have shown that nasal administration of flagellin can reduce bacterial load in an animal infection model (11, 17, 24, 25). For example, the study from Muñoz N., et al. demonstrated that respiratory treatment with flagellin leads to S. pneumoniae clearance in the lungs and promotes survival of infected mice. They attributed such results to increased neutrophil infiltration into the airways as a result of strong chemokine secretion by flagellin-stimulated ECs. Our findings are consistent with such studies. We observed that animals pre-treated with flagellin 24 h before infection, receiving or not GNT 1 h after challenge, presented significantly less PAMDR bacterial load and higher neutrophil counts in the BAL, compared with infected non-treated mice or only treated with GNT. Neutrophils play a critical role in combating infections through their antimicrobial functions. Particularly in the context of P. aeruginosa, it has been demonstrated that early neutrophil influx to the respiratory airways is essential for the clearance of the pathogen (12, 26, 27). Accordingly, we hypothesize that the rise in neutrophil counts in flagellin-treated mice could play a role in the decrease of P. aeruginosa CFU in those same mice. The secretion of antimicrobial compounds by ECs (11, 17) may also contribute to the reduction of PAMDR. To fully comprehend the effect of antimicrobial effectors in our model, additional experiments should be carried out.
While standalone flagellin was effective in clearing PAMDR, our study showed that the combination of prophylactic flagellin and therapeutic GNT confers an additional advantage. Mice treated with this combination showed an even greater reduction in lung bacterial load compared with those treated with flagellin alone, suggesting a synergy between the antibiotic and flagellin. Since we did not find any significant difference in the number of immune cell populations, particularly neutrophils, between mice treated with flagellin alone and those treated with flagellin +GNT, the observed additional advantage in the combination group may be attributed to the direct antibiotic’s impact despite GNT’s limited efficacy against PAMDR when used alone. We hypothesize that the prophylactic nasal administration of flagellin may have contributed not only to the recruitment of phagocytes to the site of infection but also to the secretion of multiple antimicrobial peptides, which in turn led to the elimination of, at least, some of the bacteria present in the respiratory tract. When GNT was administered, 1 h after infection, less viable bacteria in the flagellin-regulated lung mucosa enabled the antibiotic to efficiently clear most of the remaining bacteria.
Thus, our work is reminiscent of previous studies showing that a host-directed immunomodulator can contribute to the clearance of a pathogen, facilitating the action of an antibiotic (13, 28,–31). Secoeudesma sesquiterpenes lactone A (SESLA) is an anti-inflammatory molecule proven to modulate NFκB and PI3K/Akt signaling pathways (31). When in simultaneous combination with the antibiotic meropenem, it promotes bacterial clearance and survival in mice infected with carbapenem-resistant Klebsiella pneumoniae. Recently, a similar strategy was found to be efficacious in the context of intestinal infections with Salmonella Typhimurium (28). Indeed, the combination of systemic administration of ciprofloxacin and a TLR4 or TLR9 agonist was able to further reduce the number of antibiotic-tolerant Salmonella in the gut and the associated draining lymph nodes, compared with standalone treatments (28). Another study also showed that the simultaneous combination of flagellin and the antibiotic amoxicillin, administered 12 h post-infection, can provide additional protection by effectively eliminating a resistant strain of S. pneumoniae (13).
A host-directed immunomodulator, when paired with an antibiotic, should also promote a balanced inflammatory response and confer long-lasting immunity (32, 33). In our study, respiratory administration with flagellin 24 h before challenge, either alone or in combination with GNT, effectively reduced excessive local inflammation caused by PAMDR infection. Mice receiving the combination of flagellin and GNT exhibited even lower tissue hemorrhage, a decrease in total cell infiltration in the BAL, and a return of proinflammatory cytokine levels to steady state by 16 h post-infection. These findings are in alignment with a recent investigation conducted by López-Gálvez et al. (20). Using airway epithelial cells and a pig model, their research demonstrated that flagellin serves as an immunostimulant molecule capable of eliciting a transient proinflammatory reaction. This proinflammatory stimulus plays a pivotal role in initiating an efficient host immune response toward a forthcoming pathogen. However, because flagellin’s proinflammatory stimulus is short-lived and the molecule itself is also rapidly degraded within the airway mucosa, a few hours after its administration, there is no longer inflammation driven by flagellin. They also show that particularly in the context of P. aeruginosa infection, a first prophylactic stimulus with flagellin is enough to attenuate P. aeruginosa-triggered inflammatory response. Although the detailed mechanism is still unclear, López-Gálvez et al. (20) suggest that epigenetic changes or trained immunity caused by flagellin (also present in P. aeruginosa flagellum) may also contribute to restraining inflammation upon P. aeruginosa challenge (34, 35). For example, the research of Bigot and colleagues demonstrated that bronchial epithelial cells pre-exposed with flagellin modify their inflammatory response to a second stimulus due to epigenetic regulation (35). The observed decreased inflammatory response could also be related to TLR5 signaling desensitization due to a decrease in receptor availability or tachyphylaxis, as observed when repeated doses of TLR ligands are administered to mice (36). In addition, it has been shown that flagellin treatment increased the concentration of TNFAIP3. This key negative regulator of the innate immune response can decrease the signalling of both TLR4 and TLR5 pathways, thereby modifying the ability of airway epithelial cells to respond to a second stimulation by P. aeruginosa (37, 38).
Our findings also demonstrate that preventive administration of flagellin can enhance the preservation of AM, which were diminished following P. aeruginosa infection. Such apparent reduction has also been previously reported in the context of influenza and SARS-Cov-2 infection (39, 40). Although we have yet to fully elucidate the precise mechanisms underlying this decrease in our PAMDR infection model, our data strongly suggest that nasal flagellin administration effectively mitigates AM reduction. The advantages of preserving AM in the context of respiratory infections are vast (41). These cells are the first to encounter pathogens in the alveoli. After an antigen exposure, AM become activated and not only are able to internalize and kill pathogens but also produce several proinflammatory cytokines and chemokines that recruit neutrophils to the site of infection, helping with clearance. Thus, flagellin-dependent AM preservation may contribute to the reduction of P. aeruginosa infections.
It has been shown that total DCs, which include monocyte derived-DCs and conventional DCs, are increased in the airway mucosa after stimulus with flagellin. Although we did not discriminate DC subtypes in the current study, we also observed an increase in these immune cell populations in animals that were nasally challenged with flagellin, 24 h before infection (receiving or not GNT 1 h post-infection). These results may indicate possible long-lasting immunity driven by prophylactic nasal flagellin against a MDR strain of P. aeruginosa, although further studies would have to be conducted to confirm such a hypothesis.
Finally, the beneficial synergy between flagellin and gentamicin was evidenced in a survival study. The combination between flagellin and GNT protected all infected mice, which was not observed in any other group. The observed survival outcome may be attributed to the synergistic effects of flagellin and the restoration of GNT effectiveness, involving several mechanisms as follows: (i) flagellin enhances the influx of neutrophils into the lungs, (ii) these cells aid in bacterial clearance, (iii) the reduced bacterial burden increases the antibiotic-to-bacteria ratio, thereby restoring the efficacy of GNT, and (iv) flagellin’s prophylactic effect mitigates excessive inflammation in the lungs, ultimately contributing to improved host survival. Such results highlight the advantageous synergy between the two molecules and underline the importance of investigating such combination further. Moreover, our work extends the current knowledge about the beneficial effect of flagellin as host-directed immunomodulator to MDR strains of P. aeruginosa, which present a significant challenge for patients with chronic respiratory conditions and limited treatment options. Although more research is required to confirm the feasibility of prophylactic flagellin administration in patients, this study provides a basis for a novel dual therapy against P. aeruginosa in the context of increasing antimicrobial resistance.
ACKNOWLEDGMENTS
The authors are very grateful to Dr. Christophe Paget and the Ph.D. student Camille David for the fruitful discussions on flow cytometry analysis. The authors would also like to thank the Animal Facility of the University of Tours, the Vaccine Development department of Statens Serum Institut (Denmark) for the manufacture and supply of FLAMOD, and Mara Baldry for her support as a scientific manager of the FAIR project.
The work was funded by the project FAIR from the European Union’s Horizon 2020 research and innovation program under grant agreement No 847786.
A.R.M., A.C., J.-C.S., V.H., and M.S.-T. designed the study. A.R.M., A.C., D.F., V.V., and B.B. performed the experiments. A.R.M., A.C., V.H., and M.S.-T. wrote the manuscript. All authors analyzed the data, contributed to the article, and approved the submitted version.
SUPPLEMENTAL MATERIAL
The following material is available online at https://doi.org/10.1128/aac.01361-23.
Supplemental material Figure S1
aac.01361-23-s0001.docx:Growth curve of PAMDR in the presence or absence of gentamicin antibiotic.
Supplemental material Figure S2
aac.01361-23-s0002.docx:Gating strategy used for flow cytometry analysis.
Supplemental material Table S1
aac.01361-23-s0003.docx:Characterization of PAMDR clinical strain by the microbiology department of the Bretonneau University Hospital (Tours, France).
ASM does not own the copyrights to Supplemental Material that may be linked to, or accessed through, an article. The authors have granted ASM a non-exclusive, world-wide license to publish the Supplemental Material files. Please contact the corresponding author directly for reuse.
REFERENCES
Articles from Antimicrobial Agents and Chemotherapy are provided here courtesy of American Society for Microbiology (ASM)
Similar Articles
To arrive at the top five similar articles we use a word-weighted algorithm to compare words from the Title and Abstract of each citation.
Proof-of-Principle Study in a Murine Lung Infection Model of Antipseudomonal Activity of Phage PEV20 in a Dry-Powder Formulation.
Antimicrob Agents Chemother, 62(2):e01714-17, 25 Jan 2018
Cited by: 37 articles | PMID: 29158280 | PMCID: PMC5786808
Evaluation of chlorogenic acid and carnosol for anti-efflux pump and anti-biofilm activities against extensively drug-resistant strains of Staphylococcus aureus and Pseudomonas aeruginosa.
Microbiol Spectr, 12(9):e0393423, 24 Jul 2024
Cited by: 0 articles | PMID: 39046262 | PMCID: PMC11370622
Silver nanoparticle with potential antimicrobial and antibiofilm efficiency against multiple drug resistant, extensive drug resistant Pseudomonas aeruginosa clinical isolates.
BMC Microbiol, 24(1):277, 26 Jul 2024
Cited by: 0 articles | PMID: 39060955 | PMCID: PMC11282727
Funding
Funders who supported this work.
European Union's Horizon 2020 research and innovation programme (1)
Grant ID: 847786
European Union's Horizon 2020 research and innovation programme (1)
Grant ID: 847786