Abstract
Free full text

Nitric Oxide-Dependent Production of cGMP Supports the Survival of Rat Embryonic Motor Neurons Cultured with Brain-Derived Neurotrophic Factor
Abstract
Trophic factor deprivation induces neuronal nitric oxide synthase (NOS) and apoptosis of rat embryonic motor neurons in culture. We report here that motor neurons constitutively express endothelial NOS that helps support the survival of motor neurons cultured with brain-derived neurotrophic factor (BDNF) by activating the nitric oxide-dependent soluble guanylate cyclase. Exposure of BDNF-treated motor neurons to nitro-l-arginine methyl ester (l-NAME) decreased cell survival 40–50% 24 hr after plating. Both low steady-state concentrations of exogenous nitric oxide (<0.1 μm) and cGMP analogs protected BDNF-treated motor neurons from death induced by l-NAME. Equivalent concentrations of cAMP analogs did not affect cell survival. Inhibition of nitric oxide-sensitive guanylate cyclase with 2 μm1H-[1,2,4]oxadiazolo[4,3-a]quinoxalin-1-one (ODQ) reduced the survival of BDNF-treated motor neurons by 35%. cGMP analogs also protected from ODQ-induced motor neuron death, whereas exogenous nitric oxide did not. In all cases, cell death was prevented with caspase inhibitors. Our results suggest that nitric oxide-stimulated cGMP synthesis helps to prevent apoptosis in BDNF-treated motor neurons.
Nitric oxide (NO) production has been implicated in the induction of neuron death by glutamate (Dawson et al., 1991, 1993; Strijbos et al., 1996) and in the pathogenesis of a variety of neurodegenerative diseases (Beckman, 1991; Varner and Beckman, 1994), including Alzheimer’s disease (Good et al., 1996;Smith et al., 1997), multiple sclerosis (Sherman et al., 1993), and amyotrophic lateral sclerosis (Beckman et al., 1993; Abe et al., 1995,1997; Chou et al., 1996a,b). Ventral root avulsion in the rat results in the induction of nitric oxide synthase (NOS) and motor neuron loss 6 weeks after treatment (Wu, 1993). Inhibition of NOS activity or prevention of NOS expression by brain-derived neurotrophic factor (BDNF) improves motor neuron survival after ventral root avulsion (Wu and Li, 1993; Novikov et al., 1995, 1997).
Motor neuron survival in culture is highly dependent on trophic factor supply (Henderson et al., 1993, 1994; Hughes et al., 1993; Pennica et al., 1996). Trophic factor deprivation of cultured motor neurons stimulates de novo synthesis of neuronal NOS, increases nitrotyrosine immunoreactivity (Estévez et al., 1998), and induces apoptosis after 18–24 hr (Milligan et al., 1995; Pennica et al., 1996; Estévez et al., 1998). Inhibition of nitric oxide synthesis supports the survival of trophic factor-deprived motor neurons for up to 3 d. Production of nitric oxide by (Z)-1-[2-(2-aminoethyl)-N-(2-ammonio-ethyl)amino] diazen-1-ium-1,2-diolate (DETA-NONOate) reverses protective effects of NOS inhibitors against apoptosis induced by trophic factor deprivation. In contrast, extracellular NO production does not affect the number of motor neurons surviving either in the presence or absence of trophic factors (Estévez et al., 1998), showing that nitric oxide itself is not sufficient to initiate apoptosis.
Nitric oxide also plays an important role in cell signaling in the nervous system and is implicated in synaptic plasticity (Garthwaite, 1991; Dinerman et al., 1994; O’Dell et al., 1994; Zhuo et al., 1994;Boulton et al., 1995; Garthwaite and Boulton, 1995; Kantor et al., 1996). Nitric oxide is known to contribute to spinal motor neuron maturation stimulated by supraspinal inputs (Kalb and Agostini, 1993).
It has been proposed that nitric oxide toxicity involves its reaction with superoxide to form the strong oxidant peroxynitrite (Beckman, 1991; Beckman et al., 1993: Dawson et al., 1993; Lipton et al., 1993;Troy et al., 1996; Estévez et al., 1998), although its protective and regulatory effects may be attributable to either stimulation of cGMP synthesis by the soluble guanylate cyclase (Garthwaite and Boulton, 1995; Farinelli et al., 1996) or S-nitrosylation of the NMDA receptor and probably other cellular targets (Lipton et al., 1993;Stamler et al., 1997). Activation of the soluble guanylate cyclase is a major signaling pathway activated by nitric oxide (Ignarro, 1989;Garthwaite and Boulton, 1995). Early studies showed that cultured spinal cord neurons produce cGMP (Weill, 1982). Furthermore, cGMP analogs prevented spinal cord motor neuron apoptosis during the period of programmed cell death (Weill and Greene, 1984), suggesting a role for cGMP in motor neuron survival and differentiation. We report that nitric oxide-stimulated cGMP synthesis helps prevent apoptosis of motor neuron cultures in the presence of BDNF, suggesting that low levels of nitric oxide can have a role in the survival of motor neurons.
MATERIALS AND METHODS
Materials. Monoclonal antibodies to p75 low-affinity neurotrophin receptor and to Islet-1/2 were obtained from the culture medium of the MC192 (Chandler et al., 1984) and 4D5 (Ericson et al., 1992; Tsuchida et al., 1994) hybridoma cells obtained from C. E. Henderson (Institut National de la Santé et de la Recherche Médicale Unité 382, Developmental Biology Institute of Marseille, Marseille, France) and the Developmental Studies Hybridoma Bank (Iowa City, IA), respectively. Polyclonal antibodies to neuronal and endothelial NOS were from Transduction Laboratories (Lexington, KY) and a generous gift from B. Mayer (Karl-Franzes-Universität Graz, Graz, Austria). Monoclonal antibodies to endothelial NOS were kindly provided by T. Michel (Harvard Medical School, Boston, MA). Affinity-purified anti-mouse IgG was from Cappel (Durham, NC). Cy3- and FITC-conjugated goat anti-mouse and anti-rabbit secondary antibodies were obtained from Jackson ImmunoResearch (West Grove, PA). Recombinant mouse BDNF was a gift of R. W. Scott and J. D. Hirsch (Cephalon, Inc., West Chester, PA). Culture media, serum, insulin, and antibiotics were from Life Technologies (Grand Island, NY). The NO donor DETA-NONOate, the guanylate cyclase inhibitor 1 H-[1,2,4]oxadiazolo[4,3-a]quinoxalin-1-one (ODQ), and 8-bromo and 8-(4-chlorophenylthio) analogs of cGMP and cAMP were from Alexis Biochemicals (San Diego, CA). The caspase inhibitor Ac-YVAD-CHO was from Calbiochem (San Diego, CA), and caspase inhibitors z-VAD-fmk and Ac-DEVD-CHO were from Alexis Biochemicals (San Diego, CA). Other reagents used were from Sigma (St. Louis, MO).
Motor neuron purification and culture. Purified motor neurons were prepared from rat embryonic day 15 spinal cord by combination of metrizamide gradient centrifugation and immunopanning with the monoclonal antibody IgG192 against the p75 low-affinity neurotrophin receptor, as described previously (Henderson et al., 1995;Estévez et al., 1998). Motor neurons were cultured in L15 media supplemented with 0.63 mg/ml sodium bicarbonate, 5 μg/ml insulin, 0.1 mm putrescine, 0.1 mg/ml conalbumin, 30 nmsodium selenite, 20 nm progesterone, 20 mmglucose, 100 IU/ml penicillin, 100 μg/ml streptomycin, and 2% horse serum. Before plating, culture dishes and slides were precoated with polyornithine–laminin. Cultures maintained for 24 hr in the presence of BDNF were mainly composed of large neurons with long-branched neurites. More than 94% of the cells showed immunofluorescence for the motor neuron markers p75 neurotrophin receptor (Yan and Johnson, 1988;Henderson et al., 1993; Estévez et al., 1998) and Islet-1/2 (Henderson et al., 1993; Tsuchida et al., 1994; Estévez et al., 1998).
Determination of mRNA expression. Total RNA from 50,000 motor neurons plated on 60 mm dishes was isolated using Trizol (Life Technologies) according to manufacturer’s instructions, reverse-transcribed with a reverse transcription-PCR (RT-PCR) kit (Stratagene, La Jolla, CA), and amplified with the GeneAmp PCR reagent kit (Perkin-Elmer, Norwalk, CT) (1 cycle at 91°C for 5 min, 54°C for 5 min, followed by 30 cycles at 91°C for 1 min; 1 cycle at 54°C for 1 min; 1 cycle at 72°C for 2 min; and a final cycle of 72°C for 10 min). Sense and antisense primers were for endothelial NOS 5′-TACGGAGCAGCAAATCCAC and 5′-CAGGCTGCAGTCCTTTGATC-3′ as described byShaul et al. (1995). These endothelial NOS primers did not yield a detectable product using the neuronal NOS cDNA as a template. Furthermore, the results of a search for the primer sequences in the National Institutes of Health BLAST indicate that the only homologous sequence contained in the library corresponds to the rat endothelial NOS. The products of the PCR were separated by electrophoresis in a 2% agarose gel and visualized in a UV transilluminator after staining with ethidium bromide. Glyceraldehyde-3-phosphate dehydrogenase (GAPDH) mRNA was used to normalize the levels of RNA (Estévez et al., 1998).
Immunofluorescence. Cultures were fixed for 15 min with a combination of 4% paraformaldehyde and 0.1% glutaraldehyde in PBS on ice. Then the cells were rinsed three times with PBS, permeabilized with 0.1% Triton X-100 for 15 min, blocked for 1 hr with 10% goat serum and 2% bovine serum albumin in PBS, incubated with the primary antibody overnight at 4°C, rinsed three times with PBS, incubated with FITC- or Cy3-conjugated secondary antibody for 30 min at room temperature, rinsed again three times with PBS, fixed with 4% paraformaldehyde, and rinsed with PBS. After a brief rinse in double-distilled water, the cells were mounted in a SlowFade-Light antifade kit (Molecular Probes, Eugene, OR). Primary antibodies were used at a dilution of 1:100 for supernatant from 4D5 hybridoma against Islet-1/2 and 5 μg/ml for the IgG 192 to p75 neurotrophin receptor. Rabbit polyclonal antibody against neuronal NOS from Transduction Laboratories was used at a 1:100 dilution. Antibodies to endothelial NOS included a monoclonal provided by T. Michel used at a 1:200 dilution and two different polyclonal antibodies obtained from B. Mayer and Transduction Laboratories used at 1:400 and 1:100 dilutions, respectively. Similar results were obtained with all three antibodies to endothelial NOS, but the best results were obtained using the polyclonal antibody obtained from B. Mayer. Controls used included omission of the primary antibody or nonpermeabilizing cells when using antibodies directed against the intracellular antigens endothelial NOS and Islet-1/2.
Determination of motor neuron survival. Motor neuron survival was quantified 24 hr and 3 d after plating by counting all healthy neurons with neurites longer than four soma diameters under phase contrast in a 1 cm2 field in the center of the dish, as described previously (Henderson et al., 1993; Pennica et al., 1996; Estévez et al., 1998). Motor neurons considered viable by this method also stained with the vital dye fluorescein diacetate. Most of the degenerating neurons considered nonviable by this method showed morphological characteristics of apoptosis, including disintegration of the neurites and soma shrinkage (see Fig. Fig.2).2). Nuclear condensation and fragmentation were visualized by terminal deoxynucleotidyl transferase-mediated biotinylated UTP nick end labeling as described previously (Estévez et al., 1998). To compare the results of different experiments, the number of cells attached with neurites 4–5 hr after plating in the presence of BDNF was taken as 100% survival and was ~50–60% of the cells initially plated (Henderson et al., 1993; Estévez et al., 1998).
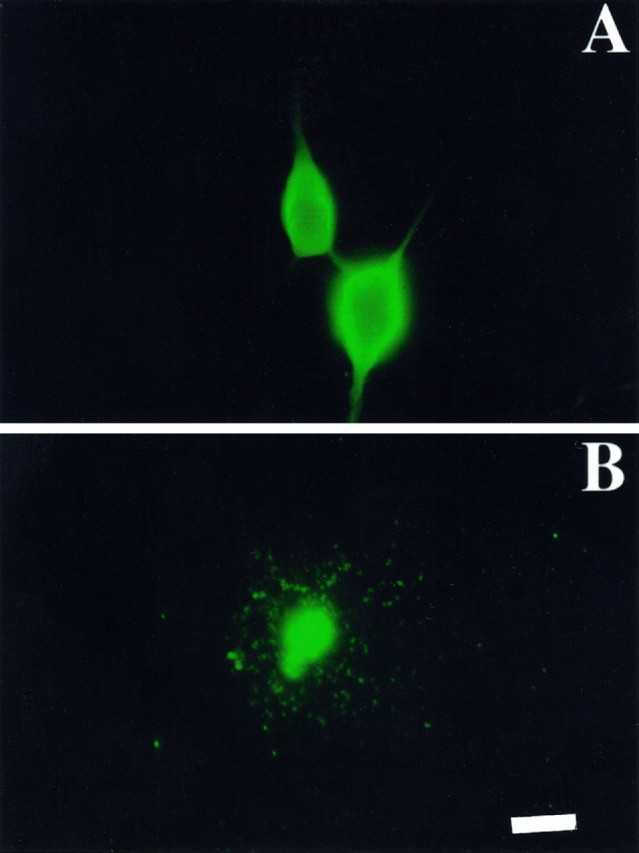
Endothelial NOS immunoreactivity in cultured motor neurons. Fluorescence micrographs of motor neurons cultured for 24 hr with 100 pg/ml BDNF (A) or without trophic factors (B) and stained using antibodies to endothelial NOS. Staining was performed as described in Materials and Methods. Similar results were obtained using two other antibodies. Omission of the primary antibody and nonpermeabilized cells revealed no detectable stain. Scale bar, 25 μm.
RESULTS
Motor neurons in culture express endothelial NOS
BDNF-treated motor neurons expressed endothelial NOS mRNA (Fig.(Fig.1)1) and were immunoreactive for endothelial NOS protein 24 hr after plating (Fig.(Fig.2).2). Both mRNA (Fig. (Fig.1)1) and immunoreactivity (Fig. (Fig.2)2) for endothelial NOS were also present after trophic factor deprivation, which causes motor neurons to undergo apoptosis (Milligan et al., 1995; Estévez et al., 1998). In contrast, mRNA and protein immunoreactivity for neuronal NOS were found only after trophic factor deprivation and were not detectable in BDNF-treated neurons, as reported previously (Estévez et al., 1998). Endothelial NOS immunoreactivity was localized predominately in cytoplasmic regions of the soma of BDNF-treated motor neurons. Less immunoreactivity was found in the neurites. In contrast, endothelial NOS immunoreactivity in trophic factor-deprived cells was disordered because of the loss of nuclear and cellular structure during apoptosis. These results showed that motor neurons constitutively express both the messenger and the protein for endothelial NOS.
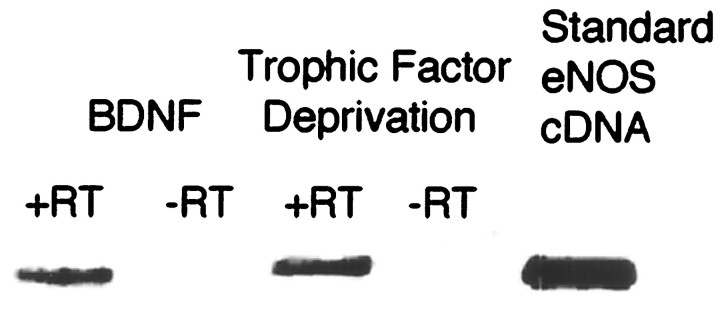
Rat embryonic motor neurons in culture express endothelial NOS mRNA. Total RNA from 50,000 motor neurons was extracted 24 hr after plating. Half of the RNA was incubated with reverse transcriptase (+RT) before PCR amplification, whereas the other half was amplified without RT (−RT). The amplification product for the GAPDH was used to standardize the quantities of sample used in the gels. A cDNA (100 pg) from an endothelial NOS vector was used as the standard, and the specificity of the primers was assessed using a similar quantity of neuronal NOS cDNA (data not shown).
Inhibition of nitric oxide synthesis initiates BDNF-treated motor neuron death
Inhibition of nitric oxide production by l-NAME supported trophic factor-deprived motor neuron survival (Fig.(Fig.33A) but stimulated the death of >40% of the BDNF-treated neurons 24 hr after plating (Fig.(Fig.33B). Low steady-state concentrations of NO (<100 nm) produced by 20 μm DETA-NONOate were measured in the culture medium, as described previously (Beckman and Conger, 1995; Estevez et al., 1998). Production of low steady-state concentrations of exogenous nitric oxide by 20 μmDETA-NONOate prevented BDNF-treated motor neuron death induced byl-NAME for 24 hr (Fig. (Fig.3)3) and for up to 3 d (data not shown) but did not affect motor neuron survival in either the presence or absence of BDNF (Fig. (Fig.3).3). The inactive stereoisomer nitro-d-arginine methyl ester (d-NAME) did not modify neurotrophin-treated motor neuron survival (Fig. (Fig.33B). These results suggested that l-NAME induced motor neuron death by inhibiting nitric oxide production.
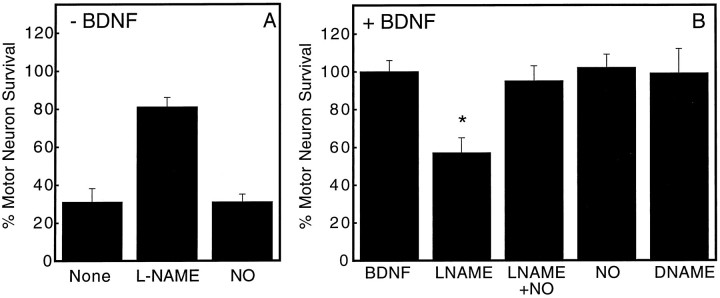
Effect of NOS inhibitors on BDNF-dependent motor neuron survival. Motor neuron survival was determined 24 hr after plating by counting neurons with neurites longer than four soma diameters, as described in Materials and Methods. Motor neurons were cultured with (A) or without (B) 100 pg/ml BDNF plus 1 mml-NAME (LNAME), 1 mmd-NAME (DNAME), 20 μmDETA-NONOate (NO), or the indicated combinations. The steady-state concentration of NO produced by 20 μmDETA-NONOate under our experimental conditions was 100 nm, as described previously (Beckman and Conger, 1995; Estévez et al., 1998). Different from BDNF, *p < 0.001. Values are the mean ± SD of at least three experiments performed in duplicate.
Most of the cells considered nonviable showed apoptotic morphology (Fig. (Fig.22B). To further determine whetherl-NAME inhibited neurite growth or induced apoptosis,l-NAME toxicity on BDNF-treated motor neuron cultures was challenged using the following: Ac-YVAD-CHO, a selective caspase I inhibitor that prevents cultured motor neuron apoptosis (Milligan et al., 1995; Estévez et al., 1998); zVAD, a general caspase inhibitor; and Ac-DEVD-CHO, a selective caspase III inhibitor (for review, see Nicholson and Thornberry, 1997). Motor neuron death induced by NOS inhibition was prevented by all three caspase inhibitors for 24 hr (Fig. (Fig.4),4), suggesting thatl-NAME induced apoptosis in BDNF-treated motor neurons rather than inhibited differentiation. Similar results were obtained after 3 d, but the fresh caspase inhibitors had to be added every 24 hr (data not shown). The protection by caspase inhibitors suggests that the change in morphology was attributable to motor neuron death by apoptosis rather than inhibition of differentiation.
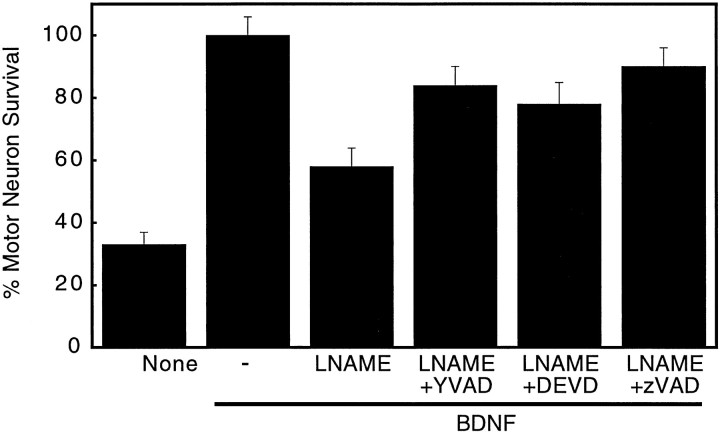
Inhibition of caspase activity preventsl-NAME-induction of BDNF-treated motor neuron death. Motor neuron survival was determined 24 hr after plating, as indicated in Figure Figure3.3. Cultures were exposed to 100 pg/ml BDNF alone or with the indicated combinations of 1 mml-NAME (LNAME), 10 μm Ac-YVAD-CHO (LNAME+YVAD), 50 μmzVAD-fmk (LNAME+zVAD), and 30 μm Ac-DEVD-CHO (LNAME+DEVD). Values are the mean ± SD of two experiments performed in duplicate.
cGMP supports motor neuron survival
The cGMP analogs 8-Br-cGMP and 8-CPT-cGMP abolished the toxic effects of l-NAME on BDNF-treated motor neurons (Table(Table1).1). The protective effect of 8-Br-cGMP was dependent on the concentration, with an apparent EC50of 30 μm, and was maximal at ~100 μm(Fig. (Fig.5).5). No protection was provided by 100 μm 8-CPT-cAMP (Table (Table1)1) or 10–1000 μm8-Br-cAMP (Fig. (Fig.5),5), showing that cGMP analogs were not protective by cross-activation of a cAMP-dependent pathway (Jiang et al., 1992;Cornwell et al., 1994).
Table 1.
cGMP analogs prevent l-NAME-induced death of BDNF-treated motor neurons
Treatment | % Motor neuron survival | n |
---|---|---|
BDNF (100 pg/ml) | 100 ± 6 | 8 |
None | 35 ± 9 | 8 |
BDNF + l-NAME (1 mm) | 52 ± 5 | 8 |
BDNF + l-NAME + Br-cGMP (1 mm) | 96 ± 6 | 6 |
BDNF +l-NAME + CPT-cGMP (100 μm) | 94 ± 10 | 6 |
BDNF + l-NAME + Br-cAMP (1 mm) | 50 ± 8 | 4 |
BDNF + l-NAME + CPT-cAMP (100 μm) | 56 ± 8 | 4 |
The percentage of motor neuron survival was determined 24 hr after plating as indicated in Materials and Methods. The number of cells in BDNF at 24 hr was taken as 100%. Values are the mean ± SD of n determinations from at least two independent experiments.

Concentration-dependent prevention ofl-NAME toxicity by 8-Br-cGMP but not by cAMP. Motor neuron survival was determined 24 hr after plating, as described in Figure Figure3.3. ○, Trophic factor deprivation; , BDNF (100 pg/ml) plusl-NAME (1 mm);
, BDNF plusl-NAME plus 8-Br-cGMP; □, BDNF plus l-NAME plus 8-Br-cAMP. Values are the mean ± SD of at least two experiments performed in duplicate.
To further test the role of cGMP production on motor neuron survival, BDNF-treated cultures were exposed to the selective soluble guanylate cyclase inhibitor ODQ (Garthwaite et al., 1996). ODQ (2 μm) did not affect the survival of motor neurons deprived of trophic factors but stimulated ~40% of BDNF-treated motor neurons to die after 24 hr (Fig. (Fig.6).6). After 72 hr, ODQ stimulated the death of 55 ± 7% of the motor neurons cultured with BDNF. Analogs of cGMP abolished the toxic effects of ODQ after 24 hr (Fig. (Fig.6)6) and for up to 3 d (data not shown), whereas extracellular generation of nitric oxide from DETA-NONOate was not protective (data not shown). These results suggest that ODQ increased apoptosis was attributable to selective inhibition of cGMP synthesis by guanylate cyclase.
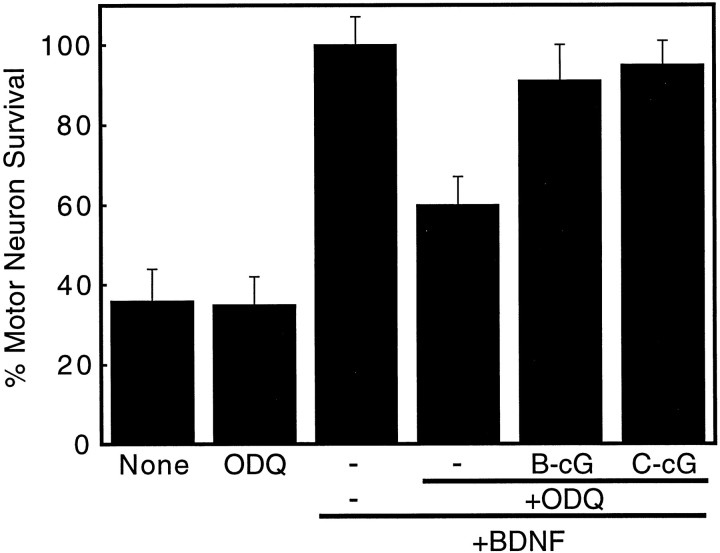
Effect of the inhibition of soluble guanylate cyclase on BDNF-treated motor neuron survival. Motor neuron survival was determined 24 hr after plating, as described in Figure Figure3.3. Motor neurons were cultured with 100 pg/ml BDNF (bars 3–6), 2 μm ODQ (bars 2,4–6), 1 mm 8Br-cGMP (B-cG; bar 5), and 100 μmCTP-cGMP (C-cG; bar 6) in the indicated combinations. Values are the mean ± SD of at least three experiments performed in duplicate.
DISCUSSION
In the present study, we found that nitric oxide produced by the endothelial isozyme of nitric oxide synthase contributed to the survival of motor neurons cultured with BDNF by a cGMP-dependent mechanism. Inhibition of nitric oxide synthesis with l-NAME or cGMP formation with the guanylate cyclase inhibitor ODQ reduced motor neuron survival by 40%. The cell death appeared to result from apoptosis based on morphological criteria and protection with caspase inhibitors. Cell death attributable to NOS inhibition was prevented either by generating low steady-state concentrations of exogenous nitric oxide or by the addition of cGMP analogs. In contrast, cell death induced by the guanylate cyclase inhibitor ODQ was prevented by cGMP analogs but not by exogenous nitric oxide. These results suggest that nitric oxide-dependent activation of soluble guanylate cyclase was necessary for BDNF to support the survival of motor neurons in culture.
Treatment of chick embryos with cGMP analogs prevents programmed cell death of motor neurons (Weill and Greene, 1984), suggesting that cGMP production may be involved in embryonic development. Induction of neuronal NOS is frequently associated with critical developmental periods in the brain. However, Kalb and Agostini (1993) report that differentiation of motor neurons in vivo appears to be influenced by nitric oxide production during a period in which motor neurons are not immunoreactive for neuronal NOS, suggesting that either nitric oxide is produced by neighboring cells or by a different NOS isoform. Our results indicate that embryonic motor neurons in culture constitutively express endothelial NOS. We have reported previously that BDNF-treated motor neurons do not express detectable amounts of neuronal NOS, but it is rapidly induced in BDNF-deprived cultures (Estévez et al., 1998). No detectable immunoreactivity for the inducible macrophage-like nitric oxide synthase could be found in either BDNF-treated or -deprived motor neuron cultures (A. G. Estevez and J. S. Beckman, unpublished observations). Consequently, endothelial NOS was the most likely candidate for stimulating cGMP formation that contributes to BDNF-stimulated survival of motor neurons in culture.
Immunoreactivity for endothelial NOS has been found in adult human motor neurons from amyotrophic lateral sclerosis patients (Chou et al., 1996b; Abe et al., 1997). Endothelial NOS is also reported in rat and mouse hippocampal pyramidal neurons in which it may play a role in the regulation of long-term potentiation (Dinerman et al., 1994; O’Dell et al., 1994; Kantor et al., 1996). Posttranslational modifications of endothelial NOS result in a distinct intracellular localization compared with neuronal NOS. The N terminus of endothelial NOS can be both palmitoylated and myristylated, the hydrophobic tails of which anchor the endothelial NOS to the plasma and Golgi membranes (Busconi and Michel, 1993; Robinson et al., 1995, 1996; Sessa et al., 1995). The activity of endothelial NOS is further modulated in endothelium by binding to caveolin proteins that keep endothelial NOS in an inactive state (Feron et al., 1996; Michel et al., 1997). In contrast, neuronal NOS is a soluble enzyme but contains an additional 20 kDa N-terminal protein domain known as the PDZ domain (PSD-95/disks large/ZO-1 homology domain) that targets neuronal NOS to membrane-associated proteins (Brenman et al., 1997). These interactions can localize neuronal NOS to NMDA receptor in neurons and to cluster near motor neuron endplates in skeletal muscle in which the influx of calcium after channel opening will rapidly activate neuronal NOS. The distinct intracellular localization of endothelial NOS compared with neuronal NOS may result in different physiological regulations and functionsin vivo. Trophic factors such as neurotrophins are known to elevate intracellular calcium levels in neurons (Hegarty et al., 1997;Holm et al., 1997; Jiang et al., 1997) that may be responsible for the activation of endothelial NOS and subsequent stimulation of cGMP production.
The apparent requirement for nitric oxide-stimulated cGMP production for the survival of motor neurons cultured with BDNF was surprising, because we have shown previously that the production of nitric oxide by neuronal NOS can induce motor neuron death in trophic factor-deprived motor neurons (Estévez et al., 1998). The toxicity of nitric oxide in trophic factor-deprived motor neurons appears to result from the simultaneous production of superoxide and nitric oxide that leads to the formation of peroxynitrite (Estévez et al., 1998). Both NOS inhibitors and superoxide scavengers largely prevent motor neuron death attributable to trophic factor deprivation. In addition, motor neurons become immunoreactive for nitrotyrosine, a modification induced by peroxynitrite (Beckman et al., 1994; Beckman, 1996). The protection by NOS inhibitors in BDNF-deprived motor neurons was overcome by generating nitric oxide with 20 μm DETA-NONOate. However, the same concentrations of NO generated under identical conditions from DETA-NONOate were protective in the present study to motor neurons treated with NOS inhibitors. Consequently, nitric oxide either can support the survival of motor neurons by stimulating cGMP synthesis or can induce apoptosis by reacting with superoxide to form peroxynitrite (Estévez et al., 1998).
In parallel with our observations on motor neurons, nitric oxide has been shown to support survival of PC12 cells deprived of trophic factors through a cGMP-dependent mechanism (Farinelli et al., 1996). However, nitric oxide can also be toxic to PC12 cells when superoxide scavenging is impaired with antisense superoxide dismutase (SOD) oligonucleotides (Troy et al., 1996). The toxicity resulting from SOD downregulation has also been attributed to the formation of peroxynitrite in PC12 cells (Troy et al., 1996). Nerve growth factor (NGF) prevents death, induces differentiation of PC12 cells (Greene and Tischler, 1976), and stimulates de novo synthesis of all three isoforms of NOS (Peunova and Enikolopov, 1995). Inhibition of nitric oxide production prevents differentiation of PC12 cells by NGF but does not affect cell survival induced by NGF (Peunova and Enikolopov, 1995; Farinelli et al., 1996). This contrasts with our results with cultured motor neurons in which inhibition of nitric oxide synthesis in the presence of trophic factors led to apoptosis. In both PC12 cells and motor neurons, endogenous production of nitric oxide has important physiological functions mediated by cGMP that improve survival and differentiation. On the other hand, NO can become toxic to motor neurons by forming peroxynitrite when conditions increase cellular superoxide generation, as occurs during trophic factor deprivation.
Footnotes
This work was supported by grants from National Institutes of Health (NIH) (J.S.B.) and the American Amyotrophic Lateral Sclerosis Association (J.S.B.). N.S. was supported in part by the Basic Mechanisms in Lung Disease Training Fellowship Grant HL07533 from NIH. We thank C. E. Henderson from the Developmental Biology Institute of Marseille for the MC192 hybridoma and for helpful suggestions and discussion; R. A. Star from the University of Texas (Dallas, TX) for the generous gift of endothelial NOS cDNA; R. W. Scott and J. D. Hirsch from Cephalon, Inc. (West Chester, PA) for BDNF; B. Mayer from Karl-Franzes-Universität Graz (Graz, Austria) for endothelial NOS antibodies; K. Conger, Y. Zhuang, and Y. Z. Ye from University of Alabama at Birmingham for helpful suggestions and assistance; Q. Li for technical assistance with the RT-PCR; and V. Darley-Usmar for helpful comments on this manuscript.
Correspondence should be addressed to Dr. Alvaro G. Estévez, Department of Anesthesiology, Research Division, University of Alabama at Birmingham, 1900 University Boulevard, THT 958, Birmingham, AL 35233.
REFERENCES
Articles from The Journal of Neuroscience are provided here courtesy of Society for Neuroscience
Full text links
Read article at publisher's site: https://doi.org/10.1523/jneurosci.18-10-03708.1998
Read article for free, from open access legal sources, via Unpaywall:
https://www.jneurosci.org/content/jneuro/18/10/3708.full.pdf
Free after 6 months at www.jneurosci.org
http://www.jneurosci.org/cgi/content/full/18/10/3708
Free to read at www.jneurosci.org
http://www.jneurosci.org/cgi/content/abstract/18/10/3708
Free after 6 months at www.jneurosci.org
http://www.jneurosci.org/cgi/reprint/18/10/3708.pdf
Citations & impact
Impact metrics
Article citations
Mechanisms of nitric oxide in spinal cord injury.
Med Gas Res, 14(4):192-200, 28 Mar 2024
Cited by: 0 articles | PMID: 39073327 | PMCID: PMC11257186
Review Free full text in Europe PMC
The Behavioral and Neurochemical Changes Induced by Boldenone and/or Tramadol in Adult Male Rats.
Neurochem Res, 48(5):1320-1333, 30 Nov 2022
Cited by: 0 articles | PMID: 36449200 | PMCID: PMC10066173
cGMP Signaling in the Neurovascular Unit-Implications for Retinal Ganglion Cell Survival in Glaucoma.
Biomolecules, 12(11):1671, 11 Nov 2022
Cited by: 3 articles | PMID: 36421684 | PMCID: PMC9687235
Review Free full text in Europe PMC
The Complex Mechanisms by Which Neurons Die Following DNA Damage in Neurodegenerative Diseases.
Int J Mol Sci, 23(5):2484, 24 Feb 2022
Cited by: 18 articles | PMID: 35269632 | PMCID: PMC8910227
Review Free full text in Europe PMC
Increased bioavailability of cyclic guanylate monophosphate prevents retinal ganglion cell degeneration.
Neurobiol Dis, 121:65-75, 10 Sep 2018
Cited by: 8 articles | PMID: 30213732 | PMCID: PMC6424569
Go to all (111) article citations
Similar Articles
To arrive at the top five similar articles we use a word-weighted algorithm to compare words from the Title and Abstract of each citation.
Nitric oxide and superoxide contribute to motor neuron apoptosis induced by trophic factor deprivation.
J Neurosci, 18(3):923-931, 01 Feb 1998
Cited by: 231 articles | PMID: 9437014 | PMCID: PMC6792767
Whole brain spheroid cultures as a model to study the development of nitric oxide synthase-guanylate cyclase signal transduction.
Brain Res Dev Brain Res, 125(1-2):99-115, 01 Dec 2000
Cited by: 12 articles | PMID: 11154766
Differential regulation of vasoactive intestinal peptide (VIP) in the dentate gyrus and hippocampus via the NO-cGMP pathway following kainic acid-induced seizure in the rat.
J Mol Neurosci, 42(3):359-369, 06 Apr 2010
Cited by: 4 articles | PMID: 20369387
Tonic inhibitory action by nitric oxide on spontaneous mechanical activity in rat proximal colon: involvement of cyclic GMP and apamin-sensitive K+ channels.
Br J Pharmacol, 127(2):514-520, 01 May 1999
Cited by: 21 articles | PMID: 10385253 | PMCID: PMC1566019