Abstract
Free full text

Acute Plasmodium chabaudi chabaudi Malaria Infection Induces Antibodies Which Bind to the Surfaces of Parasitized Erythrocytes and Promote Their Phagocytosis by Macrophages In Vitro
Abstract
CBA/Ca mice infected with 5 × 104 Plasmodium chabaudi chabaudi AS-parasitized erythrocytes experience acute but self-limiting infections of relatively short duration. Parasitemia peaks (~40% infected erythrocytes) on day 10 or 11 and is then partially resolved over the ensuing 5 to 6 days, a period referred to as crisis. How humoral and cellular immune mechanisms contribute to parasite killing and/or clearance during crisis is controversial. Humoral immunity might be parasite variant, line, or species specific, while cellular immune responses would be relatively less specific. For P.c. chabaudi AS, parasite clearance is largely species and line specific during this time, which suggests a primary role for antibody activity. Accordingly, acute-phase plasma (APP; taken from P.
c. chabaudi AS-infected mice at day 11 or 12 postinfection) was examined for the presence of parasite-specific antibody activity by enzyme-linked immunosorbent assay. Antibody binding to the surface of intact, live parasitized erythrocytes, particularly those containing mature (trophozoite and schizont) parasites, was demonstrated by immunofluorescence in APP and the immunoglobulin G (IgG)-containing fraction thereof. Unfractionated APP (from P.
c. chabaudi AS-infected mice), as well as its IgG fraction, specifically mediated the opsonization and internalization of P.
c. chabaudi AS-parasitized erythrocytes by macrophages in vitro. APP from another parasite line (P.
c. chabaudi CB) did not mediate the same effect against P.
c. chabaudi AS-parasitized erythrocytes. These results, which may represent one mechanism of parasite removal during crisis, are discussed in relation to the parasite variant, line, and species specificity of parasite clearance during this time.
An estimated 500 million clinical cases of malaria occur each year. Of these, only 1 million to 2 million, mostly young children, develop complicated and/or severe malaria and die. Of the remaining cases, many will be primary (possibly acute) infections in nonimmune individuals and will be treated, with various degrees of success, with antimalarial drugs. Yet others of these individuals must be capable of controlling potentially dangerous levels of parasitemia, in primary infections, in the absence of chemotherapy. Populations of areas endemic for malaria may be almost continuously exposed to infected mosquitoes during the transmission seasons. Even as they recover from their primary infections, many individuals are susceptible to reinfection and become semi-immune during successive infection episodes. It may take many years to establish protective hyperimmunity capable of preventing clinical disease (1, 4; see also reference 6). The basis of this continued susceptibility and the exact nature of mediators controlling primary parasitemia are central in any analysis of immunity to malaria.
Malaria parasites demonstrate extensive antigenic diversity and undergo antigenic variation. Immunity to malaria in a range of hosts, including humans, is markedly parasite species, line, and variant specific, although a degree of cross-resistance is seen in some cases (10, 16, 20). These are factors which may partly explain the observed susceptibility to reinfection in humans. As such, they represent important considerations in the host-parasite interaction in human malaria and also in the design and application of effective vaccines. Study of the dynamics and relative efficacy of specific and cross-reactive immune responses occurring during primary infection and reinfection is therefore particularly relevant. Analysis of infections with the rodent malaria Plasmodium chabaudi chabaudi has allowed sophisticated modeling of this situation under laboratory conditions. Thus, (i) inbred mice infected with the AS cloned line of P.c. chabaudi experience acute but self-limiting infections (6), (ii) P.
c. chabaudi AS is antigenically diverse and undergoes antigenic variation during a single infection (3, 13), and (iii) immunity to the parasite has been demonstrated to include variant-, line-, and species-specific components (7, 20).
Immunity to malaria in various experimental animal hosts has been shown clearly to be both B- and T-cell dependent (14, 28). More recent studies of P. chabaudi infections in mice with genetic or experimentally induced lesions of their immune system suggested that parasite clearance after first peak parasitemia (crisis) is B-cell independent (25–27). These authors proposed that T-cell-activated macrophages secrete mediators which are directly cytotoxic to intraerythrocytic parasites. Such activity might well be parasite variant, line, or species specific at the T-cell (induction) level but would be relatively nonspecific at the macrophage (effector) level. Studies of P.c. chabaudi AS-infected (immunologically intact) mice, when animals were superinfected with homologous or heterologous parasites 1 or 2 days into crisis, clearly showed line or species specificity of parasite clearance (7, 20). These results suggested that the mediators of crisis were specific in nature and that nonspecific cell-mediated mechanisms cannot (alone) account for the massive parasite removal that occurs at this time. Later work identified a potent antiparasitic activity in plasma taken from P.
c. chabaudi AS-infected mice during early crisis. Thus, when P.
c. chabaudi AS-parasitized erythrocytes (PE) were preincubated with such plasma in vitro and then injected back into reporter mice, the inoculum demonstrated markedly reduced infectivity in a parasite line-specific manner (8). In this report, we (i) demonstrate that plasma from mice undergoing crisis contains antibody which binds specifically to the surface of homologous PE and (ii) investigate the ability of such antibody to opsonize these cells for phagocytosis, since there is strong evidence that mononuclear cell phagocytic activity may play an important role in the clearance of parasites during infection (22, 23, 29).
MATERIALS AND METHODS
Parasites and mice.
CBA/Ca mice and P.c. chabaudi AS and CB parasites were maintained and prepared as previously described (6). The parasites were originally obtained as cloned lines from the WHO Registry of Standard Malaria Parasites, University of Edinburgh.
Preparation of APP and NP.
A group of mice were infected intraperitoneally with 5 × 104 PE, and their parasitemia was monitored by light microscopy of tail blood films stained with Giemsa’s reagent. A control group was injected intraperitoneally with Krebs saline containing glucose (KGS) (6). On days 11 to 12 postinfection (approximately 1 to 2 days after peak parasitemia), mice from both the infected and the control (sham-infected) groups were bled into 200 μl of KGS containing 25 U of heparin per ml at 4°C. The blood was then centrifuged (2,000 × g at 4°C for 1 to 2 min), and the plasma was removed and snap frozen in liquid nitrogen. Acute-phase plasma (APP) was always obtained on days 11 to 12 postinfection, and normal plasma (NP) was obtained from the sham-infected mice. In some experiments, plasma samples obtained on sequential days of infection were used; in the figures for these experiments, the relevant axis is labeled with the corresponding days of infection where plasma samples were taken.
Plasma fractionation.
A protein G-Sepharose 4 Fast Flow column was washed at 4°C with 300 ml of phosphate-buffered saline (PBS) followed by 30 ml of binding buffer (0.02 M sodium phosphate [pH 7.0]); 6 ml of NP or APP was loaded onto the column, followed by 30 ml of binding buffer. The unbound non-immunoglobulin G (IgG) fraction was collected, and the IgG-rich protein retained on the column was then eluted with 30 ml of 1.0 M glycine-HCl (pH 2.7). Both fractions were dialyzed against PBS and concentrated to the initial volume of plasma, using Centriprep 3 concentrators (Amicon).
Enzyme-linked immunosorbent assay (ELISA).
P.c. chabaudi AS-infected CBA/Ca mice (40% parasitemia) were bled into KGS-heparin at 4°C to provide PE. The blood was passed through a CF11 cellulose powder (Whatman, Maidstone, United Kingdom) column to remove leukocytes and then washed three times with KGS by centrifugation at 750 × g for 15 min at 4°C. The final cell pellet was resuspended to 5 ml in KGS, and approximately 3 μl of 10% (wt/vol) saponin in KGS was added to lyse the erythrocyte membranes. After centrifugation at 18,000 × g for 5 min at 4°C, the supernatant was removed, the pellets were lysed with 3 to 4 volumes of a detergent buffer (1% Triton X-100, 5 mM EDTA, 100 mM Tris-HCl [pH 8.0]) and centrifuged at 200,000 × g for 5 min at 4°C, and the supernatant was retained. Each well of a 96-well microtiter plate was coated with 50 μl of an appropriate predetermined dilution of this PE antigen in coating buffer (100 mM Tris-HCl [pH 8.0]). After overnight incubation at 4°C, the plates were washed with Tris-buffered saline–0.05% Tween 20 and blocked with BLOTTO (Tris-buffered saline, 0.05% Tween 20, 5% dried milk) for 2 h at room temperature. Serial dilutions in BLOTTO of NP and plasma from different days of a P.
c. chabaudi AS infection were then added. After incubation at room temperature for 2 h, the plates were washed three times as described above. An anti-mouse Ig affinity-purified biotin-conjugated antibody (The Binding Site Co., Birmingham, United Kingdom) was then added (in different experiments, conjugated antibodies directed against different mouse Ig isotypes were also used). The plates were incubated for 40 min at 37°C, washed six times, and exposed to streptavidin-conjugated alkaline phosphatase (Sigma) for 40 min at 37°C. The plates were then washed as described above, incubated for 15 min with substrate buffer (10 mM diethanolamine [pH 9.5], 0.5 mM Mg Cl2), exposed to the substrate p-nitrophenyl phosphate (Sigma), and read in a Titertek Multiskan MCC/340 reader with a 405-nm filter. All samples were tested in triplicate, and background values were obtained by using 96-well microtiter plates where only coating buffer (without antigen) was added, and the binding of antibody from each sample was assayed as described above. These values were subtracted from the respective values obtained for anti-PE binding.
Surface immunofluorescence antibody assay.
PE and nonparasitized erythrocytes (E) from infected and noninfected CBA/Ca mice, respectively, were washed three times by centrifugation in KGS containing 1% (wt/vol) bovine serum albumin (KGS-B), and 1 μl of the final cell pellet was placed in a 0.5-ml tube. Ten microliters of homologous APP (from a P.c. chabaudi AS infection), heterologous APP (from a P.
c. chabaudi CB infection), or NP diluted in KGS-B (1:4 to 1:10) was added, and the cells were gently resuspended before incubation at 37°C for 30 to 60 min. Anti-mouse IgG fluorescein isothiocyanate (FITC)-conjugated antibody (Sigma) was added to a final concentration of 25 μg/ml, and incubation was continued for 15 to 30 min at 37°C. Between each addition and following the final incubation, the cells were washed twice with ice-cold KGS-B. The final pellet was resuspended in 1 ml of KGS-B, and each sample was then analyzed in a Becton Dickinson FACStar Plus fluorescence-activated cell sorter (FACS), using an Innava 90 argon ion laser at 488 nm. Using predetermined counting parameters for forward scatter (cell size for mouse E) and fluorescence intensity, a total of 10,000 events were recorded. The data were analyzed by using FACSplot analysis software.
Phagocytosis assay.
Macrophages were obtained from each of the CBA/Ca mice by peritoneal lavage with 3 to 4 ml of ice-cold RPMI 1640 medium supplemented with 5 U of heparin per ml. An erythrocyte-free leukocyte preparation of 1 × 106 to 2 × 106 cells/ml was usually obtained. One milliliter of this suspension was added to Leighton tissue culture tubes (Wheaton 358231) containing coverslips, gassed with 7% CO2–5% O2–88% N2, and incubated for 1 to 2 h at 37°C. Cells nonadherent to the coverslips were removed by washing the coverslips in situ with RPMI 1640 medium. One milliliter of RPMI 1640 containing 10% (vol/vol) fetal calf serum (FCS) was added to the adherent cells; the tubes were gassed as described above and incubated at 37°C for 2 h. During this period, aliquots of P.c. chabaudi AS PE (108 PE/ml containing mature trophozoites or schizonts at 40 to 50% parasitemia) or E in RPMI 1640–10% FCS were incubated with homologous APP, heterologous APP, NP, KGS, or homologous APP fractions at 37°C for 1 h at 70 to 80 rpm (G24 environmental incubator shaker; New Brunswick Scientific Co., Edison, N.J.). The cells were then pelleted by centrifugation, washed three times with RPMI 1640–10% FCS, and resuspended in the same medium; 1 ml was added to each Leighton tube. After a further incubation for 1 h, nonadherent or noningested PE and E were removed by gentle aspiration, and the coverslips were washed three times with 1 ml of PBS. Noningested but adherent PE and E were then lysed by a brief (20-s) treatment with cold distilled water, followed by an additional wash with 1 ml of PBS. This treatment had no detrimental effects on the integrity of the macrophages. The adherent cells were then fixed with methanol and stained with Giemsa’s reagent, and the numbers of internalized PE and E were assessed by light microscopy and quantified as described in the legend to Fig. Fig.66.
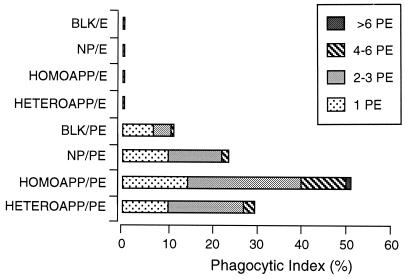
Phagocytosis of PE preincubated with homologous or heterologous APP (HOMOAPP or HETEROAPP). PE containing mature parasites, and E, were incubated with NP, APP, or KGS (BLK) and then exposed to macrophages in vitro. The results are presented as the phagocytic index (percentage of macrophages with PE inside) for the different treatments and are further broken down by the numbers of cells inside individual macrophages.
Statistical analyses.
Statistical analyses were performed by using Student’s t test, with P < 0.05 considered to be significant.
RESULTS
Kinetics of anti-PE antibody production during P.
c. chabaudi AS infection.
The binding activity of antibody in plasma during P.c. chabaudi AS infection was assayed by ELISA against preparations of total PE lysate. Total (parasite binding) Ig levels were examined in plasma samples collected between days 4 and 16 of a P.
c. chabaudi AS infection initiated with 5 × 104 PE (Fig. (Fig.1,1, Total Ig). By the use of mouse Ig isotype-specific second antibodies, it was possible to differentiate the parasite-specific antibody binding by Ig isotype (Fig. (Fig.1,1, IgM, IgG1, and IgG2a). As early as day 4 postinfection, antibody binding was detectable and enhanced relative to NP (P < 0.01), increased between days 4 and 11, peaked at day 12 (as the mice went into crisis), and then gradually decreased. By day 16 postinfection (as the parasitemia was almost resolved), antibody levels were still higher than those observed in NP (P < 0.01). In the Ig isotype-specific ELISAs, IgM reactivity against PE increased, initially quickly (significant difference observed between NP and infected mouse plasma on day 6 postinfection [P < 0.01]) and then more gradually, between days 7 and 11, peaked at day 12, and then decreased quickly. The level of IgG1 reactivity against PE antigen preparations increased very gradually, with significant differences observed only by day 8 postinfection (P < 0.01), peaked at day 12 postinfection, and then decreased. For IgG2a, the pattern observed was very similar to that for IgG1.

ELISA analysis of binding properties of antibody present during P.c. chabaudi AS infection. CBA/Ca mice were infected with 5 × 104 PE, and the course of parasitemia was monitored (
). Plasma samples from the days indicated were collected and tested against PE antigen preparation, measured by ELISA for total Ig, IgM, IgG1, and IgG2a and expressed as the mean absorbance reading. Antibody levels were also measured in NP. All data are expressed as means of three independent experiments ± standard deviation.
Surface immunofluorescence detects antibody binding to PE.
Antibody binding to the surface of infected or noninfected cells was measured by immunofluorescence using E or PE containing either young trophozoites or mature trophozoites and schizonts. The cells were treated with NP or APP, and antibody binding was detected by using FITC-conjugated anti-mouse antibody and FACS analysis. In these assays, as in the phagocytosis assay, PE were harvested from heavily infected mice at 2 to 3 days postinfection. The binding of antibody from APP to the surface of PE was higher than that of antibody from NP (Fig. (Fig.2).2). Thus, 13.9% of mature trophozoite- or schizont-infected cells incubated with APP fluoresced (Fig. (Fig.2F),2F), compared with 2.1% of these cells incubated with NP (Fig. (Fig.2E).2E). The capacity of antibody, present in APP, to bind to PE containing young parasites (ring forms or immature trophozoites) was lower than for PE containing mature trophozoites and schizonts (3.4 and 13.9%, respectively [Fig. 2D and F]). The percentage of infected cells in the two populations was the same (40% ± 2%). Only a low (2.3%) level of antibody binding to uninfected cells was detectable (Fig. (Fig.2B).2B). We used the same system to study antibody binding to the surface of PE, using samples obtained on sequential days of infection. Although antibody binding levels were elevated between days 7 and 16 relative to NP, this difference was significant only between days 11 and 16 (Fig. (Fig.3).3). The binding to the PE surface of different Ig isotypes contained in APP or NP was also measured. Compared with NP antibody, APP-IgM, -IgG1, -IgG2a, and -IgG2b isotype binding was higher, but no difference was observed for IgG3 (Fig. (Fig.4).4).
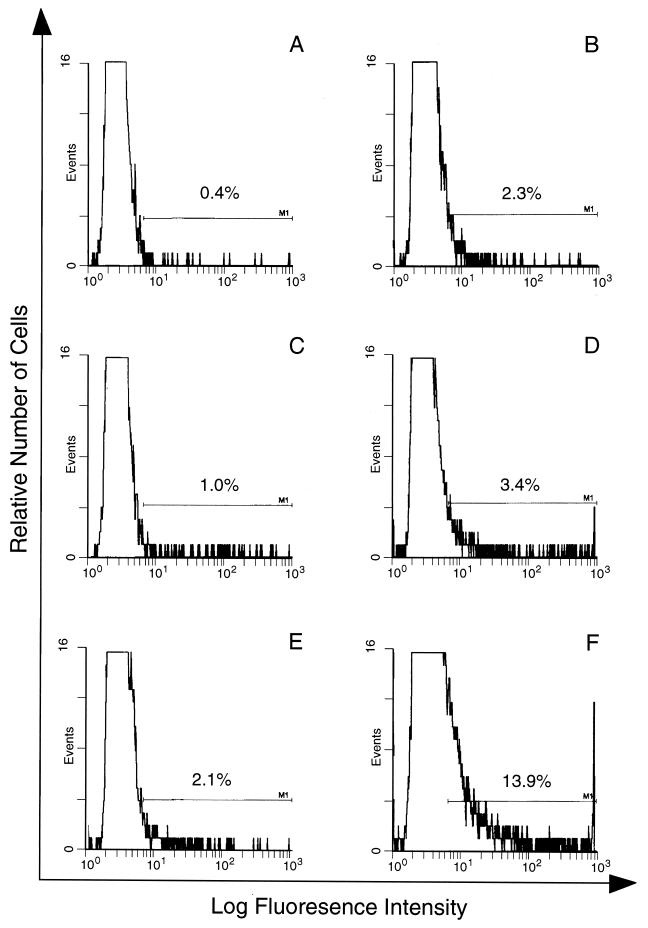
Immunofluorescence analysis of antibody in APP binding to the surface of PE and E. E (A and B) or PE containing either young parasites (C and D) or mature parasites (E and F) were incubated with NP (A, C, and E) or APP (B, D, and F), and antibody binding to the cell surface was quantified in duplicate samples by FACS analysis. The proportion of cells within the predetermined window of positive fluorescence is indicated. The window was determined by using E or PE incubated with KGS alone instead of a plasma sample.
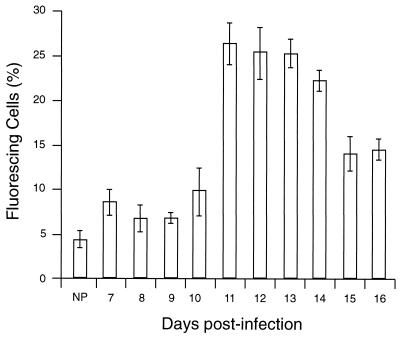
Kinetics of antibody activity during a P.c. chabaudi AS infection analyzed by surface immunofluorescence. Plasma samples from the days indicated were collected and tested against intact live PE in triplicate samples. Antibody binding to the PE surface was also measured in NP. The results represent the percentage of positive PE and were determined by using a window defined by PE incubated with KGS alone instead of plasma. All data are expressed as means of three independent experiments ± standard deviation.
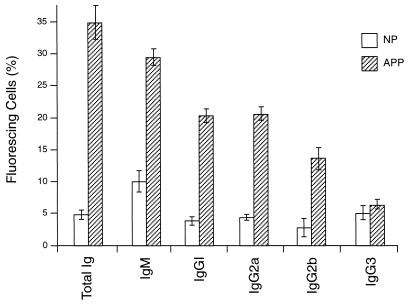
Immunofluorescence analysis of anti-P.c. chabaudi AS antibody subclasses from APP binding specifically to the surface of PE. PE containing mature parasites were incubated with NP or APP, and the antibody isotype binding to the cell surface was identified by immunofluorescence and quantified in triplicate samples by FACS analysis. The results represent the percentage of positive PE and were determined by using a window defined by PE incubated with KGS alone instead of plasma. All data are expressed as means of three independent experiments ± standard deviation.
Antibody binding to the surface of PE is parasite line specific.
Antibody binding to the surface of PE or E was measured as described above, using P.c. chabaudi AS PE containing mature trophozoites and schizonts. In this experiment, cells were treated with NP, homologous APP (from P.
c. chabaudi AS infection), or heterologous APP (from P.
c. chabaudi CB infection). The binding of antibody from homologous APP to the surface of PE was higher than that of antibody from heterologous APP (9.6 and 2.4%, respectively [Fig. 5D and F]). The difference is statistically significant (P < 0.01) and is not merely due to reduced antigen expression on P.
c. chabaudi CB PE, as APP from CB-infected mice effectively labels homologous PE (results not shown).
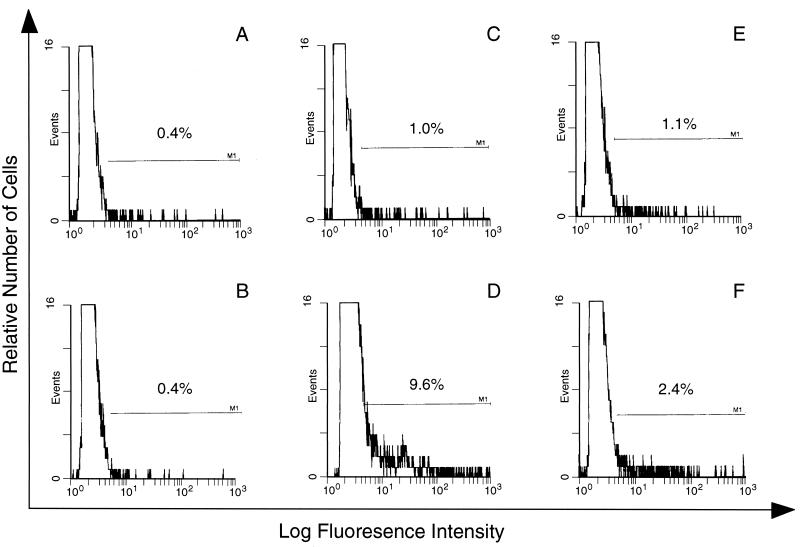
Immunofluorescence analysis of the line specificity of APP antibody. E (A, C, and E) or PE (B, D, and F) were incubated with NP (A and B), homologous anti-P.c. chabaudi AS APP (C and D), and heterologous anti-P.
c. chabaudi CB APP (E and F), and antibody binding was detected in triplicate samples. The window was determined by using E or PE incubated with KGS alone instead of plasma.
Homologous APP-mediated opsonization leads to phagocytosis of P.
c. chabaudi AS-infected cells by macrophages.
FACS analysis of cells incubated in APP indicated the presence of antibody which specifically recognized antigens on the surface of intact infected cells. This result does not define whether antibody has a role in the clearance of these infected cells during crisis. In the in vitro phagocytosis assay, incubation of PE in homologous APP resulted in significantly enhanced internalization (P < 0.01) compared to incubation in NP (52 and 24%, respectively [Fig. 6]) or heterologous APP (52 and 29%, respectively [Fig. 6]). No significant difference was found between internalization of PE incubated with NP and heterologous APP. Neither APP nor NP promoted phagocytosis of noninfected erythrocytes from either normal or infected mice, and a significant difference (P < 0.01) was found in the comparison between E and PE samples.
IgG in APP mediates opsonization of infected cells.
Fractionation of plasma on protein G produced two fractions. The fraction which specifically bound to the column contained IgG, and unbound material contained IgM (data not shown). IgG binding to the surface of PE was then determined after the cells were first treated with NP, APP, or the two protein G fractions and then exposed to FITC-conjugated anti-mouse IgG. The IgG-containing fraction of APP was the only fraction showing significant binding to the surface of PE, and the percentage of fluorescent cells in this sample was not significantly different from that in the sample treated with unfractionated APP (results not shown). After fractionation of plasma on protein G, only the IgG fraction of APP was capable of promoting phagocytosis of PE (Fig. (Fig.7).7).
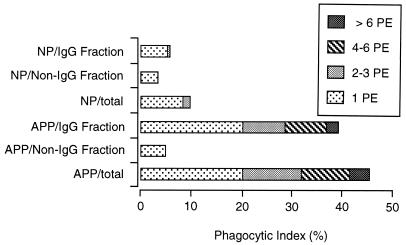
Phagocytosis of PE preincubated with protein G-fractionated APP fractions. PE containing mature parasites were incubated with NP, APP, or their protein G fractions and then exposed to macrophages in vitro. The results are presented as the phagocytic index (percentage of macrophages with PE inside) for the different treatments. Analysis by t test showed no significant difference between the APP and the IgG fraction obtained from it.
DISCUSSION
The induction of both antibody and cell-mediated responses by infection with the malaria parasite is well documented (12, 14, 18). In some individuals, these responses act to control parasitemia. However, the importance of each of these mechanisms in the dramatic decrease of parasitemia observed during crisis remains unclear. Antibody-mediated opsonization of PE, followed by their internalization and subsequent destruction by macrophages in the spleen and/or liver, might represent one of the most likely mechanisms to explain the resolution of parasitemia observed during crisis. As antibody-mediated targeting is very specific, this activity may explain the observed parasite species and line specificity of resolution. Antiparasite mediators produced by T-cell-activated macrophages capable of damaging intraerythrocytic parasites, but lacking the targeting specificity intrinsic to antibody, would be less likely to produce such specific parasite clearance.
We have analyzed the antiparasite properties of APP in P.c. chabaudi AS-infected mice and show that this activity is, at least partly, antibody dependent. ELISA showed that antibody is produced early in the P.
c. chabaudi AS infection, and surface immunofluorescence demonstrated the presence of antibody in APP reacting specifically with the surface of infected cells by day 11 postinfection. In the ELISA, antibody binding was not line specific and cross-reacted between P.
c. chabaudi AS and CB antigen preparations (results not shown). In contrast, antibody binding in the surface immunofluorescence assay was parasite line specific. That immunoassays using disrupted or nonintact PE antigen do not reflect the specificity intrinsic to the in vivo biological activity of antibody in this system has been noted previously (9). Antigen expression or detection at the surface of live intact malaria-infected E is relatively parasite maturation stage dependent. Although the parasite cell cycle in P.
c. chabaudi is highly synchronous, mature parasite forms are less evident in the peripheral blood due to sequestration. This might explain the relatively low (maximum of 13.9%) numbers of positive PE in the surface immunofluorescence assay compared to the level of parasitemia in the cell population used (40 to 50%).
We also found a correlation between antibody binding to the erythrocyte surface and phagocytosis in vitro, using either unfractionated plasma or the IgG fraction obtained from it. The phagocytic activity was not stimulated by the fraction depleted of IgG (but containing IgM). Antibody binding to the surface of a small proportion of noninfected cells was detected by surface immunofluorescence, but these cells were not phagocytosed by macrophages. To be sure that these cells were not phagocytosed and destroyed very quickly, the phagocytosis assay was performed for different periods of time (such as 10, 15, 20, 30, and 45 min). Even under these conditions, uninfected E from either infected or normal mice were not seen inside macrophages (results not shown).
These studies indicate that infection of mice with P.c. chabaudi AS induced antibody against the surface of PE and that this antibody is capable of opsonizing these cells for phagocytosis. A role for macrophages and phagocytosis in the host response to malaria was proposed over 100 years ago (23). Observations (mainly histological) made by Taliaferro and Cannon (22) indicated that in experimental infections using canaries and monkeys, there was an initial rise in parasitemia during which parasites were slowly phagocytosed, primarily in the spleen and to a lesser extent in the liver and bone marrow. Similar observations have been made in spleens of rats infected with P. berghei (29). We have histological evidence which supports the above findings for P.
c. chabaudi-infected mice (results not shown).
The antibody subclasses produced in response to infection are of particular relevance, since different antibody isotypes may have distinct biological functions. Macrophage receptors, recognizing the Fc region of antibody, are involved in a number of cellular functions, of which the ingestion of IgG-coated particles is fundamental in defense against bacteria and parasites (reviewed in reference 15). In the rat, IgG1 is most efficient for opsonization of erythrocytes for phagocytosis by macrophages, while IgG2a is more efficient in mediating antibody-dependent cytotoxicity (15). In the mouse, however, the situation seems not to be so clear, as here different isotypes of antibody are involved in the process of phagocytosis (17). In the surface immunofluorescence assay, we identified antibody isotypes present in APP which recognize the surface antigens of PE. IgM, IgG1, IgG2a, and IgG2b binding to PE were found in APP at levels above those in NP. However, no difference in the level of IgG3 binding was observed between NP and APP. Using a similar experimental model, Taylor Robinson (24) detected appreciable levels of IgG1 by ELISA only at day 20 postinfection, with a maximal level detectable during the recrudescence of parasitemia. However, our results show that significant levels of IgG1 as early as day 8 postinfection can be detected by ELISA.
Many studies have now shown that B cells and both Th1 and Th2 CD4 T cells play an important role in immunity to erythrocytic malaria. The results of many studies, particularly those using immunocompromised or genetically modified mice, suggest that control of primary peak parasitemia is B-cell independent and dominated by Th1 cells, while control of the recrudescence is B-cell and Th2 cell dependent (11, 24, 25). However, exactly when and why there is the switch from predominantly Th1 to predominantly Th2 activity and the exact roles of these activities in crisis and eventual elimination of parasitemia are still not clear. What does seem clear in the systems described above is that infected mice can, at least partially, control initial parasite replication during crisis in the absence of B cells. Our results provide evidence that as early as 1 to 2 days after peak parasitemia, specific mechanisms (detecting infected cells versus noninfected cells or homologous line versus heterologous line) act, possibly to damage parasites but certainly to target PE for clearance. We also show that antibody seems to play a central role in the PE clearance during this period. The apparent discrepancies in all these findings might be explained by assuming that in the absence of one (or several) immune mechanisms, other compensatory responses become more important.
This study has identified potentially protective antibody in the plasma of mice infected with P.c. chabaudi AS at the time of crisis. Future experiments will be designed to investigate this activity with respect to (i) whether it demonstrates variant specificity, (ii) the in vivo mechanism of specific parasite clearance observed as the primary peak parasitemia is resolved, (iii) the selection or induction of new parasite variants which may occur during crisis (3), and (iv) the nature of the antigens on the parasitized cell surface. In this context, the presence of erythrocyte membrane protein 1 (5) and var gene (2, 19, 21) homologs in P.
c. chabaudi AS needs to be investigated. If these goals can be accomplished, then the P.
c. chabaudi AS model will be a powerful system in which many more aspects of the host-parasite interaction in malaria can be analyzed in detail.
ACKNOWLEDGMENTS
We thank Chris Atkins for help with the FACS analysis, Dipna Joshi for help with the plasma fractionation, Keiran O’Dea for valuable discussion and advice, and Pradeep Patnaik and Sue Fleck for critical reading of this manuscript.
Maria M. Mota is supported by a PRAXIS XXI fellowship (BD 2665/94), JNICT, Portugal.
REFERENCES
Articles from Infection and Immunity are provided here courtesy of American Society for Microbiology (ASM)
Full text links
Read article at publisher's site: https://doi.org/10.1128/iai.66.9.4080-4086.1998
Read article for free, from open access legal sources, via Unpaywall:
https://iai.asm.org/content/iai/66/9/4080.full.pdf
Free after 4 months at iai.asm.org
http://iai.asm.org/cgi/content/full/66/9/4080
Free after 4 months at iai.asm.org
http://iai.asm.org/cgi/reprint/66/9/4080
Free to read at iai.asm.org
http://iai.asm.org/cgi/content/abstract/66/9/4080
Citations & impact
Impact metrics
Citations of article over time
Smart citations by scite.ai
Explore citation contexts and check if this article has been
supported or disputed.
https://scite.ai/reports/10.1128/iai.66.9.4080-4086.1998
Article citations
Using two phases of the CD4 T cell response to blood-stage murine malaria to understand regulation of systemic immunity and placental pathology in Plasmodium falciparum infection.
Immunol Rev, 293(1):88-114, 01 Jan 2020
Cited by: 6 articles | PMID: 31903675 | PMCID: PMC7540220
Review Free full text in Europe PMC
Sticking for a Cause: The Falciparum Malaria Parasites Cytoadherence Paradigm.
Front Immunol, 10:1444, 27 Jun 2019
Cited by: 40 articles | PMID: 31316507 | PMCID: PMC6610498
Review Free full text in Europe PMC
The Contribution of Co-signaling Pathways to Anti-malarial T Cell Immunity.
Front Immunol, 9:2926, 14 Dec 2018
Cited by: 5 articles | PMID: 30631323 | PMCID: PMC6315188
Review Free full text in Europe PMC
A bite to fight: front-line innate immune defenses against malaria parasites.
Pathog Glob Health, 112(1):1-12, 29 Jan 2018
Cited by: 7 articles | PMID: 29376476 | PMCID: PMC6056835
Review Free full text in Europe PMC
P2X7 receptor drives Th1 cell differentiation and controls the follicular helper T cell population to protect against Plasmodium chabaudi malaria.
PLoS Pathog, 13(8):e1006595, 31 Aug 2017
Cited by: 35 articles | PMID: 28859168 | PMCID: PMC5597262
Go to all (49) article citations
Similar Articles
To arrive at the top five similar articles we use a word-weighted algorithm to compare words from the Title and Abstract of each citation.
Antibody recognition of rodent malaria parasite antigens exposed at the infected erythrocyte surface: specificity of immunity generated in hyperimmune mice.
Infect Immun, 69(4):2535-2541, 01 Apr 2001
Cited by: 15 articles | PMID: 11254617 | PMCID: PMC98189
Susceptibility of the different developmental stages of the asexual (schizogonic) erythrocyte cycle of Plasmodium chabaudi chabaudi to hyperimmune serum, immunoglobulin (Ig)G1, IgG2a and F(ab')2 fragments.
Parasite Immunol, 23(11):587-597, 01 Nov 2001
Cited by: 19 articles | PMID: 11703810
Trafficking of Plasmodium chabaudi adami-infected erythrocytes within the mouse spleen.
Proc Natl Acad Sci U S A, 93(10):4595-4599, 01 May 1996
Cited by: 39 articles | PMID: 8643449 | PMCID: PMC39322
A dual role for B cells in Plasmodium chabaudi chabaudi (AS) infection?
Res Immunol, 145(6):412-419, 01 Jul 1994
Cited by: 12 articles | PMID: 7899705
Review
Funding
Funders who supported this work.
Medical Research Council (1)
Malaria parasite proteins and erythrocyte invasion
Anthony Holder, MRC National Inst for Medical Research
Grant ID: MC_U117532067