Abstract
Free full text

The L-type calcium channel blocker nifedipine impairs extinction, but not reduced contingency effects, in mice
Abstract
We recently reported that fear extinction, a form of inhibitory learning, is selectively blocked by systemic administration of L-type voltage-gated calcium channel (LVGCC) antagonists, including nifedipine, in mice. We here replicate this finding and examine three reduced contingency effects after vehicle or nifedipine (40 mg/kg) administration. In the first experiment, contingency reduction was achieved by adding USs to the training protocol (degraded contingency), a phenomenon thought to be independent of behavioral inhibition. In the second experiment, contingency reduction was achieved by varying the percentage of CS-US pairing, a phenomenon thought to be weakly dependent on behavioral inhibition. In the third and fourth experiments, contingency reduction was achieved by adding CSs to the training protocol (partial reinforcement), a phenomenon thought to be completely dependent on behavioral inhibition. We found that none of these reduced contingency effects was impaired by nifedipine. In a final experiment, we found that extinction conducted 1 or 3 h post-acquisition, but not immediately, was LVGCC-dependent. Taken together, the results suggest that reduced contingency effects and extinction depend on different molecular mechanisms and that LVGCC dependence of behavioral inhibition develops with time after associative CS-US learning.
Pavlovian fear conditioning in rodents has been widely used in the laboratory setting to model human fear and anxiety, as well as excitatory learning and memory processes (Fendt and Fanselow 1999; Maren 2001; Ohman and Mineka 2001; Fanselow and Gale 2003; LeDoux 2003). Fear conditioning is achieved by temporally pairing an initially neutral conditional stimulus (CS), such as a tone, with an aversive unconditional stimulus (US), usually a mild footshock. Rats show robust fear responding to a CS with as little as one CS-US pairing (Fanselow 1990). Extinction of conditional fear, the progressive weakening of the fear response by repeated presentations of the CS alone, has been an important model in the development of behavior therapy for human anxiety disorders (Wolpe 1969; Wolpe and Rowan 1988; Craske 1999; Myers and Davis 2002) and is the prototypical procedure for inducing inhibitory learning (Davis et al. 2003; Delamater 2004).
While the molecular and cellular mechanisms of fear acquisition have been intensely studied for decades, the mechanisms of fear extinction are only beginning to be unraveled. For instance, lesion and local infusion studies suggest that the amygdala (Falls et al. 1992; Quirk et al. 1997; Lu et al. 2001; Marsicano et al. 2002; Royer and Pare 2002; Walker et al. 2002; Davis et al. 2003; Ledgerwood et al. 2003; Lin et al. 2003a,b,c) and prefrontal cortex (Morgan et al. 1993; Quirk et al. 2000; Herry and Garcia 2002; Milad and Quirk 2002; Santini et al. 2004; Shah et al. 2004) participate in the learning and retention of fear extinction. Additionally, both local infusion and systemic behavioral pharmacology studies have identified important roles in extinction for the NMDA-type glutamate receptor (Falls et al. 1992; Baker and Azorlosa 1996; Li et al. 1998; Santini et al. 2001; Tang et al. 2001; McKay et al. 2002; Walker et al. 2002; Lin et al. 2003c), the cannabinoid CB1 receptor (Marsicano et al. 2002; Suzuki et al. 2004), the adrenergic system (Mason and Iversen 1977; Mason and Fibiger 1979; Berman and Dudai 2001; Cain et al. 2004), calcineurin (Lin et al. 2003b), and MAP-kinase (Berman and Dudai 2001; Lu et al. 2001; Lin et al. 2003c). Although these molecules participate in the long-term retention of fear extinction, we recently reported that systemic administration of the L-type voltage-gated calcium channel (LVGCC) antagonists, nifedipine and nimodipine, prevent both short- (within 1 h) and long-term (24 h) fear extinction (Cain et al. 2002). This finding supports a crucial role for LVGCCs in the acquisition of fear extinction.
Most extinction procedures involve three phases: (1) fear acquisition, where every CS is paired with a US (100% contingency); (2) fear extinction, where CSs are never paired with a US (0% contingency); and (3) a final test of CS-elicited fear. Psychologists divide learning into excitatory learning, which involves the formation and strengthening of new responses to a cue or CS, and inhibitory learning, which decreases such responding (Domjon 1998). The dominant view of extinction learning presumes that an excitatory CS-US association is formed during acquisition of conditioned fear, while an independent inhibitory CS-US association is formed during extinction, which ultimately compete during the final CS test to produce reduced fear (Myers and Davis 2002). Our recent findings suggest that this type of inhibitory learning is dependent on LVGCCs.
Many other Pavlovian procedural manipulations can result in reduced responding to the CS, and not all are thought to be the result of inhibitory learning. In contingency reduction protocols, acquisition itself occurs with <100% contingency (CSs and USs are not always paired). Such protocols have been a driving force in the development of learning theory (Miller and Matzel 1988b). Reduced contingency effects can be achieved with any of the following three manipulations: (1) keeping the number of CS-US pairings constant while adding USs (degraded contingency) (Rescorla 1968; Singh and Banerji 1986); (2) keeping the total number of CSs and USs constant while varying the percentage of CS-US pairing (reduced temporal overlap); or (3) keeping the number of CS-US pairings constant while varying the number of CSs (partial reinforcement) (Siegel 1977; Singh and Banerji 1986; Pearce et al. 1997). Thus, nifedipine may be a valuable tool for testing whether or not these various contingency reduction effects share a common physiological mechanism with extinction. A shared mechanism would be consistent, for instance, with scalar expectation theory (SET) (Gallistel and Gibbon 2000). However, associative theory predicts different mechanisms for the different protocols (Rescorla and Wagner 1972).
The experiments in this paper were designed to achieve two goals. The first was to replicate and extend our finding that systemic nifedipine prevents cue fear extinction. The second was to elucidate the role of LVGCCs in contingency evaluation. The results should thus shed light on whether extinction shares a common LVGCC-dependent mechanism with any or all of the protocols that reduce CS-US contingency.
Results
Cue fear extinction with nonassociative controls
We previously reported that systemic administration of nifedipine (40 mg/kg, subcutaneous) prevents both short- and long-term cue fear extinction (Cain et al. 2002). In this experiment we replicate and extend this finding (Fig. 1). A 3-d procedure was used to replicate the extinction blockade. Three groups of mice received five CS-US pairings on day 1. The next day all three groups were injected and placed in the novel extinction context. Two of the groups (Paired-Extinction-Vehicle and Paired-Extinction-Nifedipine) were presented with 60 nonreinforced CS presentations to induce extinction. The third group (Paired-No Extinction-Vehicle) served as a control for nonspecific effects of time and context exposure on retained fear and received no CS presentations. On the third day, each group was returned to the extinction context and presented with a single CS to probe cue fear. In addition to the paired groups, two pairs of nonassociative controls were included in this experiment. CS-only groups received the same 3-d procedure as the Paired-Extinction groups except that only CSs were delivered during the first session. US-only groups received the same 3-d procedure as the Paired-Extinction groups except that only USs were delivered during the first session.
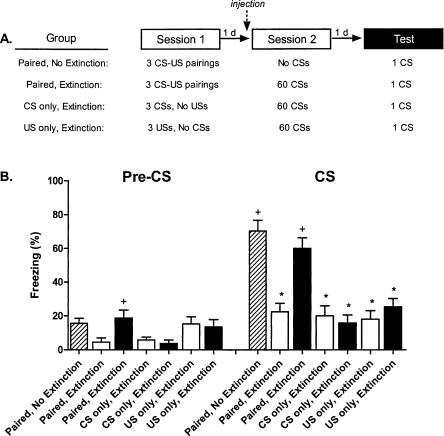
Nifedipine blocks cue fear extinction without increasing freezing in nonassociative control groups. (A) Experimental design (8-12 mice/group). (B) Freezing 1 d after extinction sessions during a 2-min acclimation period (Pre-CS) followed by a test CS (white bars, vehicle treatment; black bars, nifedipine treatment). No Extinction control mice were injected with vehicle and placed in the extinction context on day 2, but not presented with any CSs. (*) p < 0.05 vs. Paired-No Extinction; (+) p < 0.05 vs. Paired-Extinction-Vehicle.
As previously, cue fear extinction was blocked by nifedipine (F(6,77) = 19.7, p < 0.01). Freezing was generally low for all groups prior to the single CS test on day 3; however, the Paired-Extinction-Nifedipine group froze slightly more than the Paired-Extinction-Vehicle group (p < 0.05). Long-term extinction was evident (Paired-No Extinction-Vehicle vs. Paired-Extinction-Vehicle, p < 0.01), and nifedipine blocked this extinction (Paired-Extinction-Vehicle vs. Paired-Extinction-Nifedipine, p < 0.01). There was no indication that CS-only or US-only groups froze more following the extinction procedure, regardless of drug treatment (vs. Paired-No Extinction-Vehicle, p-values < 0.01; vehicle vs. nifedipine, p-values > 0.05). Thus, as we have seen before, nifedipine blocks CS extinction, while showing no interaction with pseudoconditioning or sensitization.
Contingency reduction: Adding USs to training protocol
We first examined the effect of nifedipine on contingency reduction by adding USs to a standard training protocol (degraded contingency) (Fig. 2). All groups of mice received three CS-US pairings after injections of vehicle or nifedipine on day 1 of the experiment. Mice in the 100% groups received no additional USs during the session. Mice in the 50% groups received three additional USs during the session. Mice in the 10% groups received 27 additional USs during the session. Then, 1 d after the acquisition sessions, all mice were subjected to a cue fear test in a novel context (two CS presentations) and a context fear test in the acquisition context (5-min exposure).
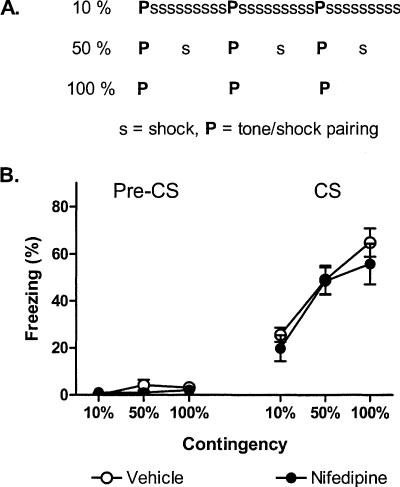
Reduced contingency effects achieved by adding USs to the training protocol are insensitive to nifedipine treatment. (A) Experimental design (eight mice/group). Mice were injected with vehicle (open circles) or nifedipine (40 mg/kg, filled circles) and subjected to either a 10%, 50%, or 100% contingent acquisition procedure (see Materials and Methods for details). (B) Freezing 1 d after acquisition during a 2-min acclimation period (Pre-CS) and two test CSs.
For the cue fear tests, freezing was low and statistically similar for all groups prior to the CS presentations (F(5,42) = 0.9). The reduced contingency protocols significantly reduced freezing to the CS. Mice in the 50% and 10% groups froze less than mice in the 100% groups (F(2,42) = 20.1, p < 0.01 for Contingency) (Fig. 2B). Nifedipine treatment had no effect on freezing at any level of contingency (F(2,42) = 0.3, p = 0.78 for the Contingency × Drug interaction). For the context fear tests, freezing was similar for all groups regardless of the contingency protocol used or drug treatment (F(2,42) = 2.7, p = 0.08 for Contingency; F(1,42) = 0.7, p = 0.43 for Drug; F(2,42) = 2.2, p = 0.12 for the Contingency × Drug interaction) (Table 1).
Table 1.
Context freezing for reduced contingency Experiments 1-3
Method of contingency reduction
| Training contingency (%)
| Nifedipine (mg/kg)
| Freezing
| |
---|---|---|---|---|
Mean | SEM | |||
Add USs to training protocol (Fig. 2) | 100 | 0 | 28.8 | 8.1 |
100 | 40 | 14.0 | 6.4 | |
50 | 0 | 34.0 | 7.3 | |
50 | 40 | 47.7 | 10.3 | |
10 | 0 | 24.6 | 6.7 | |
10 | 40 | 42.3 | 10.8 | |
Vary percentage of CS-US pairing (Fig. 3) | 100 | 0 | 23.8 | 4.5 |
100 | 40 | 34.2 | 7.3 | |
50 | 0 | 41.5 | 9.8 | |
50 | 40 | 40.4 | 11.9 | |
10 | 0 | 38.6 | 6.2 | |
10 | 40 | 41.9 | 13.6 | |
Add CSs to training protocol (Fig. 4) | 100 | 0 | 11.9 | 3.2 |
100 | 40 | 11.9 | 2.5 | |
50 | 0 | 18.1 | 7.5 | |
50 | 40 | 13.8 | 4.9 | |
10 | 0 | 15.0 | 4.0 | |
10 | 40 | 23.1 | 7.0 |
No statistically significant drug effects or contingency effects.
Contingency reduction: Varying the percentage of CS-US pairing
We next examined the effect of nifedipine on contingency reduction by varying the percentage of CS-US pairing (Fig. 3). All groups of mice received 10 CSs and 10 USs after injections of either vehicle or nifedipine on day 1 of the experiment. Mice in the 100% groups received 10 CS-US pairings and no additional CSs or USs. Mice in the 50% groups received five CS-US pairings, five CS-alone presentations, and five US-alone presentations. Mice in the 10% groups received one CS-US pairing, nine CS-only presentations, and nine US-only presentations. Then, 1 d after the acquisition sessions, all mice were subjected to a cue fear test in a novel context (two CS presentations) (Fig. 3) and a context fear test in the acquisition context (5-min exposure).
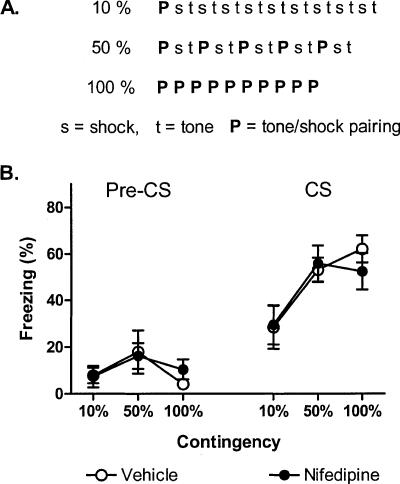
Reduced contingency effects achieved by varying the percentage of CS-US pairing are insensitive to nifedipine treatment. (A) Experimental design (seven to eight mice/group). Mice were injected with vehicle (open circles) or nifedipine (40 mg/kg, filled circles) and subjected to either a 10%, 50%, or 100% contingent acquisition procedure (see Materials and Methods for details). (B) Freezing 1 d after acquisition during a 2-min acclimation period (Pre-CS) and two test CSs.
For the cue fear tests, freezing was low and statistically similar for all groups prior to the CS presentations (F(5,42) = 1.1). Reduced contingency protocols significantly reduced freezing. Mice in the 50% and 10% groups froze less than mice in the 100% groups (F(2,41) = 8.5, p < 0.01 for Contingency) (Fig. 3B). Nifedipine treatment had no effect on freezing at any level of contingency (F(2,41) = 0.4, p = 0.68 for the Contingency × Drug interaction). For the context fear tests, freezing was similar for all groups regardless of the contingency protocol used or drug treatment (F(2,38) = 1.1, p = 0.34 for Contingency; F(1,38) = 0.3, p = 0.58 for Drug; F(2,38) = 0.2, p = 0.80 for the Contingency × Drug interaction) (Table 1).
Contingency reduction: Adding CSs to training protocol
We next examined the effect of nifedipine on contingency reduction achieved by adding CSs to a standard training protocol (partial reinforcement) (Fig. 4). All groups of mice received three CS-US pairings after injections of vehicle or nifedipine on day 1 of the experiment. Mice in the 100% groups received no additional CSs during the session. Mice in the 50% groups received three additional CSs during the session. Mice in the 10% groups received 27 additional CSs during the session. Then, 1 d after the acquisition sessions, all mice were subjected to a cue fear test in a novel context (two CS presentations) and a context fear test in the acquisition context (5-min exposure).
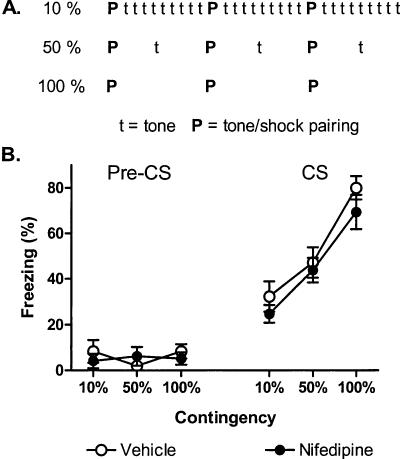
Reduced contingency effects achieved by adding CSs to the training protocol (partial reinforcement) are insensitive to nifedipine treatment. (A) Experimental design (eight mice/group). Mice were injected with vehicle (open circles) or nifedipine (40 mg/kg, filled circles) and subjected to either a 10%, 50%, or 100% contingent acquisition procedure (see Materials and Methods for details). (B) Freezing 1 d after acquisition during a 2-min acclimation period (Pre-CS) and two test CSs.
For the cue fear tests, freezing was low for all groups prior to the CS presentations (F(5,42) = 0.5). Reduced contingency protocols significantly reduced freezing to the CS. Mice in the 50% and 10% groups froze less than mice in the 100% groups (F(2,42) = 30.4, p < 0.01 for Contingency) (Fig. 4B). Nifedipine treatment had no effect on freezing at any level of contingency (F(2,42) = 0.2, p = 0.83 for the Contingency × Drug interaction). For the context fear tests, freezing was again similar for all groups regardless of the contingency protocol used or drug treatment (F(2,42) = 1.0, p = 0.39 for Contingency; F(1,42) = 0.1, p = 0.77 for Drug; F(2,42) = 0.8, p = 0.48 for the Contingency × Drug interaction) (Table 1).
Contingency reduction: Further examination of partial reinforcement effects
The results of the first partial reinforcement experiment were somewhat surprising. Partial reinforcement effects are often explained by an extinction-like process that is triggered by CS-alone presentations (Rescorla and Wagner 1972; Mackintosh 1975; Gibbon et al. 1980; Pearce and Hall 1980; Wagner 1981; Gallistel and Gibbon 2000). For this reason, we expected the partial reinforcement effect to be blocked by nifedipine, since nifedipine prevents extinction (Fig. 1). In order to further investigate the role of LVGCCs in partial reinforcement, we designed a second, more extensive, experiment. This experiment increased the number of CS-alone presentations to resemble the extinction study. It also contained groups to address whether or not the order of CS presentations, relative to pairings, was important in determining LVGCC dependence. All groups of mice were injected with drug or vehicle and subjected to three CS-US pairings on day 1. Mice in the 100% groups received no additional CSs during the session. Mice in the 50% groups received three additional CSs during the session. Mice in the 5% groups received 57 additional CSs during the session. There were three separate 5% conditions and three separate 100% conditions. Mice in the 5% conditions received their pairings as the first three trials in the session (5%-first), the last three trials in the session (5%-last), or three trials mixed in with the CS presentations (5%-mixed). Control (100%) groups were run for each of these conditions with CS-US pairings occurring with the same temporal spacing as in their corresponding partial reinforcement groups. Then, 1 d later, all mice were subjected to a cue fear test (two CS presentations) (Fig. 5).
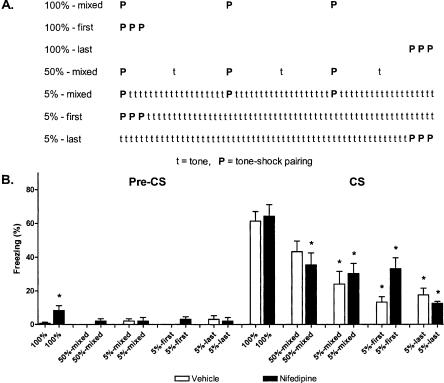
Several partial reinforcement effects are insensitive to nifedipine treatment. (A) Experimental design (8-12 mice/group). Mice were injected with vehicle (open bars) or nifedipine (40 mg/kg, filled bars) and subjected to either a 5%, 50%, or 100% contingent acquisition procedure. Three 5% contingent conditions examined the importance of trial order in determining the LVGCC dependence of partial reinforcement (see Materials and Methods for details). (B) Freezing 1 d after acquisition during a 2-min acclimation period (Pre-CS) and two test CSs. (*) p < 0.05 vs. 100%-Vehicle.
There were no statistically significant differences in freezing among the three control groups (100%-mixed, 100%-first, 100%-last; F(2,21) = 1.1, p = 0.36), and to simplify data analyses and presentation, these groups were combined into a single control group (100%). For the cue fear test, freezing was low for all groups prior to the CS presentations. The reduced contingency protocols significantly reduced freezing (F(9,110) = 13.6, p < 0.01) (Fig. 5B); all 5% groups, regardless of drug treatment, froze significantly less than the 100%-Vehicle group (p-values < 0.01). For the 50% groups, only the nifedipine-treated mice froze less than the 100%-Vehicle controls (p < 0.01). Nifedipine treatment failed to affect freezing in any of the conditions (p-values > 0.05 vs. vehicle-treated mice), even in the 5%-first groups, where there was a small trend toward increased freezing for nifedipine-treated mice.
Extinction time course
The previous two experiments indicated that nifedipine fails to block partial reinforcement effects, even when all CS-alone presentations occur after CS-US pairings, like extinction. Since nifedipine consistently blocks extinction when pairings and CS presentations are separated by a day (Fig. 1; Cain et al. 2002), we hypothesized that extinction of newly acquired fear is LVGCC-independent while extinction of consolidated fear is LVGCC-dependent. To test this hypothesis, we designed an extinction experiment in which the extinction session began immediately after acquisition, 1 h after acquisition, or 3 h after acquisition. Mice in the three conditions were injected with vehicle or nifedipine 50 min prior to their extinction session. Then, 1 d after extinction, all mice were returned to the extinction context for a test of cue fear (two CS presentations).
For the cue fear test, freezing was low for all groups prior to the CS presentations; however, the 1-h-vehicle and 3-h-vehicle groups froze less than the immediate-vehicle group (p-values < 0.05). Freezing differed between the groups during the final test session (F(5,42) = 7.0, p < 0.01; Figure 6). Extinction was less effective when CS presentations began immediately after acquisition; mice in the 1-h-vehicle and 3-h-vehicle groups froze significantly less than mice in the immediate-vehicle group (p-values < 0.05). Nifedipine blocked extinction in the 1-h and 3-h conditions (p-values < 0.01 vs. vehicle), but not in the immediate condition (p > 0.05).
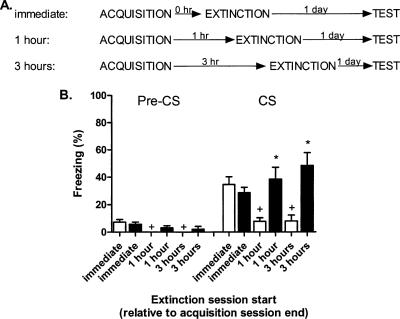
Extinction begun 1 or 3 h post-acquisition, but not immediately, is nifedipine-sensitive. (A) Experimental design (eight mice/group). Mice were subjected to cue fear acquisition followed by extinction in a different context either 0, 1, or 3 h later. Mice were injected with vehicle or nifedipine (40 mg/kg) 50 min prior to extinction. (B) Freezing 1 d after extinction during a 2-min acclimation period (Pre-CS) and two test CSs (white bars, vehicle treatment; black bars, nifedipine treatment). (*) p < 0.05 vs. Vehicle; (+) p < 0.05 vs. Immediate-Vehicle.
Discussion
We recently reported that systemic administration of nifedipine completely blocks both short- (within session) and long-term (1 d later) fear extinction learning in mice (Cain et al. 2002). The same treatment failed to alter fear acquisition or expression, suggesting that nifedipine selectively impaired fear extinction. In Experiment 1, nifedipine was again found to block long-term extinction completely (Fig. 1). Additionally, nifedipine did not increase freezing in two nonassociative control groups, ruling out the possibility that nifedipine treatment interacts with stimulus presentations during the extinction procedure to increase freezing in mice apart from a CS-US association. Thus, these results confirm that systemic LVGCC blockade selectively impairs extinction of conditional fear in mice. This finding was recently replicated by another laboratory (Suzuki et al. 2004). Since extinction is widely believed to result from associative inhibitory learning, we hypothesized that LVGCCs may be fundamentally involved in inhibitory learning (Barad et al. 2004).
We chose to examine the role of LVGCCs in contingency evaluation learning because of the apparent similarity to extinction and because this learning may involve associative inhibition (i.e., the Rescorla-Wagner Model) (Rescorla and Wagner 1972; Wagner and Rescorla 1972). The results of the contingency experiments are conclusive (Figs. (Figs.2,2, ,3,3, ,4,4, ,5).5). Adding USs or CSs or reducing the percentage of CS-US pairing all reduce conditional responding to the CS, and nifedipine fails to block any of these reductions. It may be unsurprising that degrading contingency or reducing the percentage of CS-US pairing is nifedipine-insensitive, since associative theory predicts that they are partially or entirely due to a blocking-like effect rather than to inhibitory learning (Kamin 1969). It is surprising, however, that partial reinforcement effects are LVGCC-independent. Like extinction, partial reinforcement relies only on CS-alone presentations to achieve a decrement in responding, and CS-alone presentations should theoretically lead to associative inhibition with our protocols (Rescorla and Wagner 1972; Mackintosh 1975; Gibbon et al. 1980; Pearce and Hall 1980; Wagner 1981; Gallistel and Gibbon 2000). While other theories have somewhat different interpretations of inhibitory learning, they generally state that partial reinforcement and extinction are explained by the same mechanism (Amsel 1958; Mackintosh 1975; Gibbon et al. 1980; Pearce and Hall 1980; Gallistel and Gibbon 2000; Bouton and Sunsay 2001, 2003). In a follow-up experiment designed to make the partial reinforcement procedure more closely resemble our extinction procedure (more CSs; all pairings first) (Fig. 5), we obtained the same result; partial reinforcement is nifedipine-insensitive, regardless of the order of pairings relative to CS-alone presentations. Together, these findings suggest that within-session contingency manipulations resulting in a response decrement to the CS operate through an LVGCC-independent mechanism, unlike extinction. They also indicate that LVGCC blockers like nifedipine do not generally increase fear or block all learning characterized by reduced responding.
It should be noted that the results of the context fear tests in the first three contingency experiments (Figs. (Figs.2,2, ,3,3, ,4)4) were also unexpected. In each experiment we expected, based on associative theory, that mice subjected to 10% contingent procedures would show more context freezing than those subjected to 100% contingent procedures. This was especially true in the degraded contingency experiment, in which 10% mice received 27 more shocks than 100% mice. However, we found no significant differences in context freezing related to contingency or drug treatment in any of the experiments (Table 1). It is possible that in long sessions these mice extinguish context fear during the US-free periods. Some of our own indirect evidence suggests that these C57/bl6 mice extinguish context fear faster than cue fear (Cain et al. 2002). However, other investigators have also failed to find increases in context freezing with more CS-alone presentations in experiments designed to reveal them (Maes and LoLordo 1996), and we have been unable to find any observations of this expected reciprocal increase in context conditioning with degraded CS contingency. Thus, while the orderly pattern of decreasing response to the cue with reduced contingency in our experiments suggests that cue competition should have taken place, it was not expressed as context freezing.
While there are reasonable explanations for reduced contingency effects achieved by adding USs to the training protocol that do not rely on associative inhibition, it is more difficult to explain why extinction and partial reinforcement should rely on a different process. One major distinction between our extinction experiment (Fig. 1) and the 5%-first partial reinforcement group (Fig. 5) was the delay between acquisition trials and extinction trials. In the extinction experiment, CS-alone trials occurred one day after the CS-US pairings, while in the partial reinforcement experiment the CS-alone trials occurred immediately after the CS-US pairings. Thus, we hypothesized that extinction of a well-consolidated fear memory requires LVGCCs, but extinction of a newly acquired fear memory does not.
To test this hypothesis, we subjected separate groups of mice to cue fear acquisition and conducted extinction either immediately after the acquisition session, or 1 or 3 h later. We found that nifedipine only blocked fear extinction when it occurred 1 or 3 h after acquisition. Nifedipine-treated mice given CS-alone trials immediately after acquisition extinguished the same as vehicle-treated mice. Thus, the data support our hypothesis that the LVGCC dependence of extinction is related to the maturity or consolidation of the acquisition memory.
Although unexpected, these findings strongly suggest that reduced contingency effects, regardless of the protocol used, do not depend on LVGCC activity. We propose that contingency reducing manipulations, including extinction, act to weaken the excitatory CS-US association in an LVGCC-independent manner when the newly acquired fear memory is not consolidated, perhaps through depotentiation or an LTD-like process. This possibility is supported by recent behavioral (Lin et al. 2003a,b,c) and electrophysiological (Bauer et al. 2001) reports. However, extinction of well-consolidated fear memories may lead to LVGCC-dependent associative inhibition, which acts to block fear expression without altering the excitatory memory. Also in support of this concept, we have found no published reports of “spontaneous recovery” or increase in fear with time following reduced contingency protocols including partial reinforcement, suggesting that the reduced fear is not a result of “fragile” associative inhibition that competes with excitation. However, LVGCC-dependent extinction does dissipate with time (spontaneous recovery) (Baum 1988), context change (renewal) (Bouton and King 1983), and US exposure (reinstatement) (Rescorla and Heth 1975), suggesting that the original excitatory memory remains intact after extinction and is inhibited.
Some of our findings are not predicted by prominent learning theories. Associative theory (Rescorla and Wagner 1972) makes the explicit prediction that extinction and partial reinforcement result in reduced responding via the same inhibitory process generated on CS-alone trials. However, our data indicate that extinction conducted more than 1 h after acquisition relies on an LVGCC-dependent process, while partial reinforcement does not. Although other theories of conditioning and extinction place more emphasis on CS-processing (Mackintosh 1975), timing (Gallistel and Gibbon 2000), or responding (Miller and Matzel 1988a), they do not predict the mechanistic discrepancy between extinction and partial reinforcement delineated here, and none explains the mechanistic transformation of extinction in the hours following acquisition. While behavioral examination of learning theory has proven invaluable in driving conditioning research, we expect that biological manipulations will provide important data for future theoretical consideration.
Materials and Methods
Subjects
Naive 12-20-wk-old C57/bl6 male mice (Taconic) were housed four per cage, maintained on a 12-h light/12-h dark schedule, and allowed free access to food and water. All testing was conducted during the light phase in illuminated testing rooms following protocols approved by UCLA's Institutional Animal Care and Use Committee.
Drug
The LVGCC antagonist nifedipine (40 mg/kg; Sigma) was sonicated into 100% Cremophor EL (BASF). PBS was added to make the final vehicle 10% Cremophor/90% PBS, and the mixture was again sonicated. This nifedipine dose was partly a suspension, and care was taken to thoroughly mix the drug prior to injecting. Mice were injected subcutaneously 50 min prior to behavioral testing (10 mL/kg). Drug dose and pretreatment time were chosen based on studies conducted in our laboratory (Cain et al. 2002; Barad et al. 2004) and on previous reports of systemic administration in rodents (Janicki 1988; Larkin 1992).
Conditioning apparatus
Two contexts (A and B), in separate rooms, were used for all behavioral testing. Shuttle box compartments (#ENV-010MC; Med Associates) measuring 20.3 × 15.9 × 21.3 cm served as context A, and conditioning boxes (#ENV-008; Med Associates) measuring 30.5 × 24.1 × 21 cm served as context B. Both contexts A and B had transparent front and back walls and stainless steel grid floors (3.2 mm diameter, 8 mm centers). Context A was wiped down before testing with 10% ethanol and context B with 10% methanol. Individual video cameras were mounted in the ceiling of each chamber and connected via a quad processor to a standard VCR and monitor for videotaping and scoring of freezing. Grid floors were connected to a scrambled shock source (ENV-412 and ENV-413; Med Associates). Auditory stimuli (ANL-926; Med Associates) were delivered via a speaker in the chamber wall. Delivery of stimuli was controlled with a PC and Med-PC software through a SmartCTL Interface System (DIG-716; Med Associates). Background white noise was maintained at 62 dB throughout behavioral testing.
Behavioral testing: General
In all experiments, fear acquisition was conducted in context A. Cue fear extinction and final tests of cue fear were conducted in context B. Context fear tests were conducted in context A. In all experiments the CSs were 2-min white-noise presentations (80 dB), and USs were 0.7 mA × 2-sec scrambled footshocks. Freezing behavior was rated by an experienced investigator, who was blinded to treatment classification of the mice, using a 5-sec instantaneous time sampling technique (12 observances/min).
Cue fear extinction with nonassociative controls (Fig. 1)
The experiment comprised three phases: training, context B exposure, and a final test of cue fear. Each phase was separated by 1 d to allow for memory consolidation. For training, all groups of mice were naive and in the drug-free state. Three groups of mice were subjected to CS-US pairings (paired), two groups received only CSs (CS-only), and two groups received only USs (US-only). Mice in the paired condition received five CSs with coterminating USs (2-min intertrial interval, ITI), and 2-min periods preceded and followed the pairings. Mice in the CS-only groups received five CSs on the same schedule as the paired groups, but without any US presentations (sensitization procedure). Mice in the US-only groups received five USs on the same schedule as the paired groups, but without any CSs (pseudoconditioning procedure). Freezing during the third training CS was rated for paired mice in order to match them into three equivalent treatment groups prior to session 2. Mice in the CS-only and US-only groups were divided into four separate groups. The resultant groups were as follows: Paired-No Extinction-Vehicle, Paired-Extinction-Vehicle, Paired-Extinction-Nifedipine, CS-only-Extinction-Vehicle, CS-only-Extinction-Nifedipine, US-only-Extinction-Vehicle, and US-only-Extinction-Nifedipine. Then, 1 d after training, all mice were injected, placed in context B 50 min later, and allowed to acclimate for 2 min. With the exception of the No Extinction group, all mice were subjected to the extinction protocol (60 nonreinforced CS presentations, 5-sec ITI). No Extinction control mice remained in the chambers for an equivalent period of time, but received no CS presentations. All mice were returned to context B 1 d after session 2, allowed to acclimate for 2 min, and then subjected to a single CS presentation.
Contingency reduction: Adding USs to training protocol (Fig. 2)
The experiment comprised three phases: Fear acquisition, cue fear testing, and context fear testing. Fear acquisition and fear testing were separated by 1 d to allow for memory consolidation. After vehicle or drug injections, separate groups of naive mice were placed in context A and subjected to one of three fear acquisition protocols: 100% contingency, 50% contingency, or 10% contingency. Each protocol presented three CS-US pairings to the mice with the same interpairing interval (1378 sec), beginning 2 min after entry to the chambers. For the pairings, US presentations always began 118 sec after CS onset and terminated with the CS. The session duration and number of CSs was the same for all groups; only the number and timing of USs varied between the protocols. The 100% groups received only the three CS-US pairings. For the 50% groups, an additional US was presented after each pairing (three total), either 460, 689, or 918 sec after the pairing. For the 10% groups, nine additional USs were presented after each pairing (27 total) beginning 120 sec after the pairing with an average inter-US interval of 138 sec (range 108-168 sec). Each protocol ended with a 2-min stimulus-free period. The following day, all mice were subjected to a cue fear test and a context fear test, separated by 3 h with the order of testing counterbalanced. For the cue fear test, mice were placed in context B, allowed to acclimate for 2 min, and presented with two CSs (2-min ITI). For the context fear test, mice were returned to context A for 5 min.
Contingency reduction: Varying degree of CS-US pairing (Fig. 3)
The experiment comprised three phases: Fear acquisition, cue fear testing, and context fear testing. Fear acquisition and fear testing were separated by 1 d to allow for memory consolidation. After vehicle or drug injections, separate groups of naive mice were placed in context A and subjected to one of three fear acquisition protocols: 100% contingency, 50% contingency, or 10% contingency. Each protocol presented the mice with 10 CSs and 10 USs. The CS presentations always began 2 min after entry to the chamber, and a 2-min interval separated all CSs and concluded the session. The session duration, number of US presentations, and number of CS presentations was the same for all groups; only the temporal spacing of US delivery varied between the protocols. In the 100% groups, all USs were temporally paired with CSs, with USs occurring either 38, 78, or 118 sec after CS onset in a pseudorandom order. In the 50% groups, five of the USs were paired with CSs and were given either 38, 78, or 118 sec after CS onset. The remaining five US presentations occurred during the inter-CS intervals either 15, 30, or 45 sec prior to a CS presentation. In the 10% groups, only the first trial was a CS-US pairing (118 sec CS-onset to US-onset delay). The remaining nine USs were presented during the inter-CS intervals either 15, 30, or 45 sec prior to a CS presentation. Only one US was ever presented in the intervals between CSs. The following day, all mice were subjected to a cue fear test and a context fear test, separated by 3 h with the order of testing counterbalanced. For the cue fear test, mice were placed in context B, allowed to acclimate for 2 min, and presented with two CSs (2-min ITI). For the context fear test, mice were returned to context A for 5 min.
Contingency reduction: Adding CSs to training protocol (Fig. 4)
The experiment comprised three phases: fear acquisition, cue fear testing, and context fear testing. Fear acquisition and fear testing were separated by 1 d to allow for memory consolidation. After vehicle or drug injections, separate groups of naive mice were placed in context A and subjected to one of three fear acquisition protocols: 100% contingency, 50% contingency, or 10% contingency. Each protocol presented the mice three CS-US pairings with the same interpairing interval (1380 sec), beginning 2 min after entry to the chambers. For the pairings, US presentations always began 118 sec after CS onset and terminated with the CS. The session duration and number of USs were the same for all groups; only the number and timing of CSs varied between the protocols. The 100% groups received only the three CS-US pairings. For the 50% groups, an additional CS was presented after each pairing (three total), 630 sec after the pairing. For the 10% groups, nine additional CSs were presented after each pairing (27 total) beginning 30 sec after the pairing with an inter-CS interval of 30 sec. Each protocol ended with a 2-min stimulus-free period. The following day, all mice were subjected to a cue fear test and a context fear test, separated by 3 h with the order of testing counterbalanced. For the cue fear test, mice were placed in context B, allowed to acclimate for 2 min, and presented with two CSs (2-min ITI). For the context fear test, mice were returned to context A for 5 min.
Contingency reduction: Further investigation of partial reinforcement effects (Fig. 5)
A more extensive partial reinforcement experiment was designed to mimic some of the extinction parameters and to examine the importance of trial order in determining LVGCC dependence. The experiment comprised two phases: fear acquisition and cue fear testing. Fear acquisition and fear testing were separated by 1 d to allow for memory consolidation. After vehicle or drug injections, separate groups of naive mice were placed in context A and subjected to 100% contingency, 50% contingency, or 5% contingency protocols. For all of the training protocols, mice were subjected to three CS-US pairings where the US coterminated with the CS, during a session of 7735 sec in duration. However, there were three different 100% protocols and three different 5% protocols. For the 100%-mixed groups, pairings began 2 min after entry to the chambers and were separated by a 2380-sec ITI. For the 100%-first groups, pairings began 2 min after entry to the chambers and were separated by a 5-sec ITI. For the 100%-last groups, pairings began 7245 sec after entry to the chambers and were separated by a 5-sec ITI. For the 50%-mixed groups, pairings occurred on the same schedule as the 100%-mixed groups with three additional CSs presented 1130 sec after each pairing. For the 5%-mixed groups, pairings occurred on the same schedule as the 100%-mixed and 50%-mixed groups, with 19 additional CSs presented after each pairing (5-sec inter-CS interval for all CSs in the session). For the 5%-first protocol, designed to resemble extinction procedures, pairings occurred on the same schedule as the 100%-first groups, with 57 additional CSs presented after the last pairing (5-sec inter-CS interval for all CSs in the session). For the 5%-last protocol, designed to resemble latent inhibition procedures, pairings occurred on the same schedule as the 100%-last groups, with 57 additional CSs preceding the first pairing (5-sec inter-CS interval for all CSs in the session). Each protocol ended with a 2-min stimulus-free period. Then, 1 d later, all mice were placed in context B and presented with two CSs (2-min ITI) after a 2-min acclimation period to probe cue fear.
Extinction time course (Fig. 6)
Three phases comprised the experiment: cue fear acquisition, cue fear extinction, and cue fear testing. Acquisition and extinction occurred on the same day, and testing occurred the next day. All groups of mice were subjected to the same acquisition, extinction, and testing procedures. For acquisition, mice were placed in context A, allowed to acclimate for 2 min, and presented with three CS-US pairings (CSs and USs coterminating, 2-min ITI). For extinction, mice were placed in context B, allowed to acclimate for 2 min, and presented with 45 CSs (5-sec ITI). For the day 2 test session, all mice were returned to context B, allowed to acclimate for 2 min, and presented with two CSs (2-min ITI). Mice in all groups were injected 50 min prior to the extinction session. Although this required injection before conditioning in the immediate extinction experiment, our previous data indicate that fear acquisition is not affected by nifedipine treatment (Cain et al. 2002). Drug treatment and the delay between acquisition and extinction sessions differed between the groups. Mice in the Immediate groups began the extinction session immediately after the acquisition session. Mice in the 1-h and 3-h groups began extinction 1 or 3 h after acquisition, respectively.
Statistical analyses
Percent freezing scores were calculated by dividing the instances of freezing observed by the total number of observations and multiplying by 100. All data represent mean freezing percentages (±SEM) for groups of mice during specified time bins. Pre-CS means and CS means (Figs. (Figs.11 and and5)5) were analyzed with one-way ANOVA and post hoc Dunnett's Test comparisons (when main effects were significant). Experiments of a 3 × 2 design (Figs. (Figs.2,2, ,3,3, and and4;4; Table 1) were analyzed with two-way ANOVA. Differences were considered significant if p < 0.05.
Acknowledgments
The authors thank Steve Kushner, Aaron Blaisdell, and Ravikumar Ponnusammy for helpful suggestions and comments on this manuscript. This work was supported by grants from the West Coast College of Biological Psychiatry, NARSAD, and the NIMH to M.B. and by an NIH NRSA grant to C.C.
References
- Amsel, A. 1958. The role of frustrative nonreward in noncontinuous reward situations. Psychol. Bull. 55: 102-119. [Abstract] [Google Scholar]
- Baker, J.D. and Azorlosa, J.L. 1996. The NMDA antagonist MK-801 blocks the extinction of Pavlovian fear conditioning. Behav. Neurosci. 110: 618-620. [Abstract] [Google Scholar]
- Barad, M., Blouin, A.M., and Cain, C.K. 2004. Like extinction, latent inhibition of conditioned fear in mice is blocked by systemic inhibition of L-type voltage-gated calcium channels. Learn. Mem. 11: 536-539. [Abstract] [Google Scholar]
- Bauer, E.P., LeDoux, J.E., and Nader, K. 2001. Fear conditioning and LTP in the lateral amygdala are sensitive to the same stimulus contingencies. Nat. Neurosci. 4: 687-688. [Abstract] [Google Scholar]
- Baum, M. 1988. Spontaneous recovery from the effects of flooding (exposure) in animals. Behav. Res. Ther. 26: 185-186. [Abstract] [Google Scholar]
- Berman, D.E. and Dudai, Y. 2001. Memory extinction, learning anew, and learning the new: Dissociations in the molecular machinery of learning in cortex. Science 291: 2417-2419. [Abstract] [Google Scholar]
- Bouton, M.E. and King, D.A. 1983. Contextual control of the extinction of conditioned fear: Tests for the associative value of the context. J. Exp. Psychol. Anim. Behav. Process 9: 248-265. [Abstract] [Google Scholar]
- Bouton, M.E. and Sunsay, C. 2001. Contextual control of appetitive conditioning: Influence of a contextual stimulus generated by a partial reinforcement procedure. Q J. Exp. Psychol. B 54: 109-125. [Abstract] [Google Scholar]
- ____. 2003. Importance of trials versus accumulating time across trials in partially reinforced appetitive conditioning. J. Exp. Psychol. Anim. Behav. Process 29: 62-77. [Abstract] [Google Scholar]
- Cain, C.K., Blouin, A.M., and Barad, M. 2002. L-type voltage-gated calcium channels are required for extinction, but not for acquisition or expression, of conditional fear in mice. J. Neurosci. 22: 9113-9121. [Abstract] [Google Scholar]
- ____. 2004. Adrenergic transmission facilitates extinction of conditional fear in mice. Learn. Mem. 11: 179-187. [Europe PMC free article] [Abstract] [Google Scholar]
- Craske, M.G. 1999. Anxiety disorders: Psychological approaches to theory and treatment. Westview Press, Boulder, CO.
- Davis, M., Walker, D.L., and Myers, K.M. 2003. Role of the amygdala in fear extinction measured with potentiated startle. Ann. NY Acad. Sci. 985: 218-232. [Abstract] [Google Scholar]
- Delamater, A.R. 2004. Experimental extinction in Pavlovian conditioning: Behavioural and neuroscience perspectives. Q J. Exp. Psychol. B 57: 97-132. [Abstract] [Google Scholar]
- Domjon, M. 1998. The principles of learning and behavior, pp. 67, 74. Brooks/Cole, Pacific Grove, CA.
- Falls, W.A., Miserendino, M.J., and Davis, M. 1992. Extinction of fear-potentiated startle: Blockade by infusion of an NMDA antagonist into the amygdala. J. Neurosci. 12: 854-863. [Abstract] [Google Scholar]
- Fanselow, M.S. 1990. Factors governing one-trial contextual conditioning. Anim. Learn. Behav. 18: 264-270. [Google Scholar]
- Fanselow, M.S. and Gale, G.D. 2003. The amygdala, fear, and memory. Ann. NY Acad. Sci. 985: 125-134. [Abstract] [Google Scholar]
- Fendt, M. and Fanselow, M.S. 1999. The neuroanatomical and neurochemical basis of conditioned fear. Neurosci. Biobehav. Rev. 23: 743-760. [Abstract] [Google Scholar]
- Gallistel, C.R. and Gibbon, J. 2000. Time, rate, and conditioning. Psychol. Rev. 107: 289-344. [Abstract] [Google Scholar]
- Gibbon, J., Farrell, L., Locurto, C.M., Duncan, H.J., and Terrace, H.S. 1980. Partial reinforcement in authoshaping with pigeons. Anim. Learn. Behav. 8: 45-59. [Google Scholar]
- Herry, C. and Garcia, R. 2002. Prefrontal cortex long-term potentiation, but not long-term depression, is associated with the maintenance of extinction of learned fear in mice. J. Neurosci. 22: 577-583. [Abstract] [Google Scholar]
- Janicki, P.K., Siembab, D., Paulo, E.A., and Krzascik, P. 1988. Single dose kinetics of nifedipine in rat plasma and brain. Pharmacology 36: 183-187. [Abstract] [Google Scholar]
- Kamin, L. 1969. Selective association and conditioning. In Fundamental issues in associative conditioning (eds. N. Mackintosh and W. Honig), pp. 42-64. Dalhousie University Press, Halifax, Nova Scotia, Canada.
- Larkin, J.G., Thompson, G.G., Scobie, G., Forrest, G., Drennan, J.E, and Brodie, M.J. 1992. Dihydropyridine calcium antagonists in mice: Blood and brain pharmacokinetics and efficacy against pentylenetetrazol seizures. Epilepsia 33: 760-790. [Abstract] [Google Scholar]
- Ledgerwood, L., Richardson, R., and Cranney, J. 2003. Effects of D-cycloserine on extinction of conditioned freezing. Behav. Neurosci. 117: 341-349. [Abstract] [Google Scholar]
- LeDoux, J. 2003. The emotional brain, fear, and the amygdala. Cell Mol. Neurobiol. 23: 727-738. [Abstract] [Google Scholar]
- Li, H., Weiss, S.R., Chuang, D.M., Post, R.M., and Rogawski, M.A. 1998. Bidirectional synaptic plasticity in the rat basolateral amygdala: Characterization of an activity-dependent switch sensitive to the presynaptic metabotropic glutamate receptor antagonist 2S-α-ethylglutamic acid. J. Neurosci. 18: 1662-1670. [Abstract] [Google Scholar]
- Lin, C.H., Lee, C.C., and Gean, P.W. 2003a. Involvement of a calcineurin cascade in amygdala depotentiation and quenching of fear memory. Mol. Pharmacol. 63: 44-52. [Abstract] [Google Scholar]
- Lin, C.H., Yeh, S.H., Leu, T.H., Chang, W.C., Wang, S.T., and Gean, P.W. 2003b. Identification of calcineurin as a key signal in the extinction of fear memory. J. Neurosci. 23: 1574-1579. [Abstract] [Google Scholar]
- Lin, C.H., Yeh, S.H., Lu, H.Y., and Gean, P.W. 2003c. The similarities and diversities of signal pathways leading to consolidation of conditioning and consolidation of extinction of fear memory. J. Neurosci. 23: 8310-8317. [Abstract] [Google Scholar]
- Lu, K.T., Walker, D.L., and Davis, M. 2001. Mitogen-activated protein kinase cascade in the basolateral nucleus of amygdala is involved in extinction of fear-potentiated startle. J. Neurosci. 21: RC162. [Abstract] [Google Scholar]
- Mackintosh, N. 1975. A theory of attention: Variations in the associability of stimuli with reinforcement. Psychol. Rev. 82: 276-298. [Google Scholar]
- Maes, J.H.R. and LoLordo, V.M. 1996. The effect of a discrete signal on context conditioning: Assessment by preference and freezing tests. Learn. Motiv. 27: 428-450. [Abstract] [Google Scholar]
- Maren, S. 2001. Neurobiology of Pavlovian fear conditioning. Annu. Rev. Neurosci. 24: 897-931. [Abstract] [Google Scholar]
- Marsicano, G., Wotjak, C.T., Azad, S.C., Bisogno, T., Rammes, G., Cascio, M.G., Hermann, H., Tang, J., Hofmann, C., Zieglgansberger, W., et al. 2002. The endogenous cannabinoid system controls extinction of aversive memories. Nature 418: 530-534. [Abstract] [Google Scholar]
- Mason, S.T. and Fibiger, H. 1979. Noradrenaline, fear and extinction. Brain Res. 165: 47-56. [Abstract] [Google Scholar]
- Mason, S.T. and Iversen, S.D. 1977. Effects of selective forebrain noradrenaline loss on behavioral inhibition in the rat. J. Comp. Physiol. Psychol. 91: 165-173. [Abstract] [Google Scholar]
- McKay, B.E., Lado, W.E., Martin, L.J., Galic, M.A., and Fournier, N.M. 2002. Learning and memory in agmatine-treated rats. Pharmacol. Biochem. Behav. 72: 551-557. [Abstract] [Google Scholar]
- Milad, M.R. and Quirk, G.J. 2002. Neurons in medial prefrontal cortex signal memory for fear extinction. Nature 420: 70-74. [Abstract] [Google Scholar]
- Miller, R.R. and Matzel, L.D. 1988a. The comparator hypothesis: A response rule for the expression of associations. In The psychology of learning and motivation (ed. G.H. Bower), pp. 51-92. Academic Press, San Diego, CA
- ____. 1988b. Contingency and relative associative strength. In Contemporary learning theories: Pavlovian conditioning and the status of traditional learning theory (eds. S.B. Klein and R.R. Mowrer), pp. 61-84. Lawrence Erlbaum Associates, Hillsdale, NJ.
- Morgan, M.A., Romanski, L.M., and LeDoux, J.E. 1993. Extinction of emotional learning: Contribution of medial prefrontal cortex. Neurosci. Lett. 163: 109-113. [Abstract] [Google Scholar]
- Myers, K.M. and Davis, M. 2002. Behavioral and neural analysis of extinction. Neuron 36: 567-584. [Abstract] [Google Scholar]
- Ohman, A. and Mineka, S. 2001. Fears, phobias, and preparedness: Toward an evolved module of fear and fear learning. Psychol. Rev. 108: 483-522. [Abstract] [Google Scholar]
- Pearce, J.M. and Hall, G. 1980. A model for Pavlovian learning: Variations in the effectiveness of conditioned but not unconditioned stimuli. Psychol. Rev. 87: 532-552. [Abstract] [Google Scholar]
- Pearce, J.M., Redhead, E.S., and Aydin, A. 1997. Partial reinforcement in appetitive Pavlovian conditioning with rats. Q J. Exp. Psychol. B 50: 273-294. [Abstract] [Google Scholar]
- Quirk, G.J., Armony, J.L., and LeDoux, J.E. 1997. Fear conditioning enhances different temporal components of tone-evoked spike trains in auditory cortex and lateral amygdala. Neuron 19: 613-624. [Abstract] [Google Scholar]
- Quirk, G.J., Russo, G.K., Barron, J.L., and Lebron, K. 2000. The role of ventromedial prefrontal cortex in the recovery of extinguished fear. J. Neurosci. 20: 6225-6231. [Abstract] [Google Scholar]
- Rescorla, R.A. 1968. Probability of shock in the presence and absence of CS in fear conditioning. J. Comp. Physiol. Psychol. 66: 1-5. [Abstract] [Google Scholar]
- Rescorla, R.A. and Heth, C.D. 1975. Reinstatement of fear to an extinguished conditioned stimulus. J. Exp. Psychol. Anim. Behav. Process 1: 88-96. [Abstract] [Google Scholar]
- Rescorla, R.A. and Wagner, A.R. 1972. A theory of Pavlovian conditioning: Variations in the effectiveness of reinforcement and nonreinforcement. In Classical conditioning II (eds. A.H. Black and W.F. Prokasy), pp. 64-99. Appleton-Century-Crofts, New York.
- Royer, S. and Pare, D. 2002. Bidirectional synaptic plasticity in intercalated amygdala neurons and the extinction of conditioned fear responses. Neuroscience 115: 455-462. [Abstract] [Google Scholar]
- Santini, E., Muller, R.U., and Quirk, G.J. 2001. Consolidation of extinction learning involves transfer from NMDA-independent to NMDA-dependent memory. J. Neurosci. 21: 9009-9017. [Abstract] [Google Scholar]
- Santini, E., Ge, H., Ren, K., Pena de Ortiz, S., and Quirk, G.J. 2004. Consolidation of fear extinction requires protein synthesis in the medial prefrontal cortex. J. Neurosci. 24: 5704-5710. [Abstract] [Google Scholar]
- Shah, A.A., Sjovold, T., and Treit, D. 2004. Selective antagonism of medial prefrontal cortex D4 receptors decreases fear-related behaviour in rats. Eur. J. Neurosci. 19: 3393-3397. [Abstract] [Google Scholar]
- Siegel, S. 1977. Morphine tolerance acquisition as an associative process. J. Exp. Psychol. Anim. Behav. Process. 3: 1-13. [Abstract] [Google Scholar]
- Singh, M. and Banerji, M. 1986. Interference in conditioning by CS-alone and US-alone trials. Psychol. Studies 31: 108-112. [Google Scholar]
- Suzuki, A., Josselyn, S.A., Frankland, P.W., Masushige, S., Silva, A.J., and Kida, S. 2004. Memory reconsolidation and extinction have distinct temporal and biochemical signatures. J. Neurosci. 24: 4787-4795. [Abstract] [Google Scholar]
- Tang, Y.P., Wang, H., Feng, R., Kyin, M., and Tsien, J.Z. 2001. Differential effects of enrichment on learning and memory function in NR2B transgenic mice. Neuropharmacology 41: 779-790. [Abstract] [Google Scholar]
- Wagner, A.R. 1981. SOP: A model of automatic memory processing in animal behavior. In Information processing in animals: Memory mechanisms (eds. N.E. Spear and R.R. Miller), pp. 5-47. Erlbaum, Hillsdale, NJ.
- Wagner, A.R. and Rescorla, R.A. 1972. Inhibition in Pavlovian conditioning. In Inhibition and learning (eds. R.A. Boakes and M.S. Halliday), pp. 301-336. Academic Press, New York.
- Walker, D.L., Ressler, K.J., Lu, K.T., and Davis, M. 2002. Facilitation of conditioned fear extinction by systemic administration or intra-amygdala infusions of D-cycloserine as assessed with fear-potentiated startle in rats. J. Neurosci. 22: 2343-2351. [Abstract] [Google Scholar]
- Wolpe, J. 1969. The practice of behavior therapy. Pergamon Press, New York.
- Wolpe, J. and Rowan, V.C. 1988. Panic disorder: A product of classical conditioning. Behav. Res. Ther. 26: 441-450. [Abstract] [Google Scholar]
Articles from Learning & Memory are provided here courtesy of Cold Spring Harbor Laboratory Press
Full text links
Read article at publisher's site: https://doi.org/10.1101/lm.88805
Read article for free, from open access legal sources, via Unpaywall:
http://learnmem.cshlp.org/content/12/3/277.full.pdf
Citations & impact
Impact metrics
Article citations
Weaker situations: Uncertainty reveals individual differences in learning: Implications for PTSD.
Cogn Affect Behav Neurosci, 23(3):869-893, 22 Mar 2023
Cited by: 0 articles | PMID: 36944865
Review
Reducing shock imminence eliminates poor avoidance in rats.
Learn Mem, 27(7):270-274, 15 Jun 2020
Cited by: 7 articles | PMID: 32540916 | PMCID: PMC7301752
Making translation work: Harmonizing cross-species methodology in the behavioural neuroscience of Pavlovian fear conditioning.
Neurosci Biobehav Rev, 107:329-345, 12 Sep 2019
Cited by: 25 articles | PMID: 31521698 | PMCID: PMC7822629
Review Free full text in Europe PMC
Mechanisms of fear learning and extinction: synaptic plasticity-fear memory connection.
Psychopharmacology (Berl), 236(1):163-182, 10 Nov 2018
Cited by: 30 articles | PMID: 30415278 | PMCID: PMC6374177
Review Free full text in Europe PMC
Prevention of post-concussion-like symptoms in patients presenting at the emergency room, early single eye movement desensitization, and reprocessing intervention versus usual care: study protocol for a two-center randomized controlled trial.
Trials, 19(1):555, 12 Oct 2018
Cited by: 0 articles | PMID: 30314512 | PMCID: PMC6186089
Go to all (44) article citations
Similar Articles
To arrive at the top five similar articles we use a word-weighted algorithm to compare words from the Title and Abstract of each citation.
Like extinction, latent inhibition of conditioned fear in mice is blocked by systemic inhibition of L-type voltage-gated calcium channels.
Learn Mem, 11(5):536-539, 01 Sep 2004
Cited by: 11 articles | PMID: 15466304
L-type voltage-gated calcium channels are required for extinction, but not for acquisition or expression, of conditional fear in mice.
J Neurosci, 22(20):9113-9121, 01 Oct 2002
Cited by: 85 articles | PMID: 12388619 | PMCID: PMC6757698
Rethinking the role of L-type voltage-gated calcium channels in fear memory extinction.
Learn Mem, 15(5):324-325, 25 Apr 2008
Cited by: 7 articles | PMID: 18441290
L-type voltage-gated calcium channels in conditioned fear: a genetic and pharmacological analysis.
Learn Mem, 15(5):326-334, 25 Apr 2008
Cited by: 29 articles | PMID: 18441291 | PMCID: PMC2364604