Abstract
Free full text

α-Galactosylceramide (KRN7000) suppression of chemical- and oncogene-dependent carcinogenesis
Abstract
Recent studies have revealed significant efficacy of the marine sponge glycolipid, α-galactosylceramide (α-GalCer), in treatment of experimental metastatic cancers, infections, and autoimmune diseases. However, the capacity of α-GalCer to prevent tumor development had never, to our knowledge, been evaluated in mouse models of chemical- and oncogene-dependent carcinogenesis. In this study, we demonstrate that long-term administration of soluble α-GalCer, spanning the time of tumor initiation, inhibits primary tumor formation in three different models: methylcholanthrene-induced sarcomas, mammary carcinomas in Her-2/neu transgenic mice, and spontaneous sarcomas in p53–/– mice. Weekly treatment of mice with α-GalCer maintained lymphoid tissue natural killer cell and T cell activation and elevated serum IFN-γ and IL-4 concentrations. Consistent with the antimetastatic activity of α-GalCer, prevention of methylcholanthrene-induced sarcoma was IFN-γand tumor necrosis factor-related apoptosis-inducing ligand dependent, but not perforin-dependent. Taken together, our results demonstrate that NK1.1+αβTCR+ cell-based immune therapy can inhibit primary tumorigenesis.
KRN7000, a marine sponge glycolipid with a novel α-galactosylceramide (α-GalCer) structure, was found to exhibit potent antitumor activity in a variety of experimental and spontaneous tumor metastasis models (1–7). The antitumor effects of α-GalCer were first shown to be attributable to natural killer (NK) cell activation and the antigen presentation function of dendritic cells (8). α-GalCer was then identified as a ligand presented by CD1d to the invariant Vα14 T cell antigen receptor of mouse NK1.1+αβTCR+ (NKT) cells (6, 9) and the invariant Vα24JαQVβ11 TCR of human NKT cells (10). NKT cells have a range of characteristics that distinguish them from conventional T and NK cells (reviewed in refs. 11 and 12). In particular, upon stimulation, NKT cells have a remarkable capacity to produce immunoregulatory cytokines, including IL-4, IL-10, IL-13, and IFN-γ (11, 13), that can powerfully influence the nature of adaptive immune responses. NKT cells also express a wide variety of cell death-inducing effector molecules and have been demonstrated to kill tumor target cells in vitro. Several studies have demonstrated the importance of NKT cells in immunoregulation, tumor immunity, and the prevention of autoimmune diseases in mice (reviewed in refs. 11 and 14–16).
The antitumor activities of α-GalCer resemble those of IL-12, and a series of in vitro and in vivo studies (17–20) have suggested that the initial steps that follow NKT cell TCR and CD1d/α-GalCer interaction involve IL-12 secretion by the antigen-presenting cell (21). In addition to these immediate events between NKT cells and dendritic cells, there are a series of consequences downstream, some of which result in the antitumor activity of α-GalCer (22–24). α-GalCer administration leads to rapid Th1 and Th2 cytokine production by NKT cells, and the activation of NKT cell IFN-γ production rapidly results in NK cell activation, including further IFN-γ production, cytotoxicity, proliferation, and CD69 expression (25, 26). Early NKT cell IFN-γ production was followed by prolonged (up to 3 days) NK cell IFN-γ production. NKT cell IFN-γ secretion alone was insufficient to mediate a significant antimetastatic effect (18, 23, 24). The sequential production of IFN-γ by both NKT cells and NK cells was absolutely required to reconstitute the antimeta-static activity of α-GalCer (24), and, although the α-GalCer-induced NK cell cytotoxicity in vitro was mostly perforin (pfp)-dependent, the antimetastatic effect of α-GalCer in several tumor models in vivo was not impaired in pfp-deficient mice. Recently, we have demonstrated that the IFN-γ produced by NK cells in α-GalCer-treated mice reduced tumor angiogenesis (27).
Despite the clear activity of α-GalCer against transplanted experimental tumors and their spontaneous metastases, α-GalCer has been reported to have only modest activity against transplanted primary tumors in the mammary gland or s.c. tissue (7). The majority of these studies have involved short-term treatment with α-GalCer for 1–4 weeks. In this study, we use models in which tumor immunity and IFN-γ activity have been demonstrated (28, 29) to provide an evaluation of long-term α-GalCer therapy in the prevention of chemical- and oncogene-dependent carcinogenesis.
Materials and Methods
Mice. Inbred C57BL/6J (B6) WT mice were purchased from The Walter and Eliza Hall Institute (Melbourne) and Charles River Breeding Laboratories. The following gene-targeted mice were bred and maintained at the Peter MacCallum Cancer Centre (Melbourne): B6 p53–/– mice (backcrossed for >20 generations to B6) originally obtained from Alan Harris (The Walter and Eliza Hall Institute); B6 pfp-deficient (B6 pfp–/–) mice (targeted in B6 embryonic stem cells; ref. 30); B6 IFN-γ-deficient (B6 IFN-γ–/–) backcrossed to B6 for 10 generations from Genentech (31); B6 tumor necrosis factor-related apoptosis-inducing ligand (TRAIL)-deficient (B6 TRAIL–/–) backcrossed to B6 for 5 generations (7); and B6 pfp–/– IFN-γ–/– mice bred as described (32). BALB/c mice overexpressing the transforming activated rat Her-2/neu oncogene driven by the mouse mammary tumor virus promoter (BALB-neuT mice) (33), B6 WT, and B6 WT × BALB-neuT F1 (CBf1-neuT) were maintained and bred at the Department of Clinical and Biological Sciences (University of Turin, Italy).
Her-2 Neu Transgenic Mice. Male BALB-neuT mice were mated with B6 WT females, and transgene expressing females were screened by PCR (33). As all 10 mammary glands of CBf1-neuT virgin females display palpable carcinomas within 36 weeks, the mammary pads of these mice were inspected weekly, and data were recorded as tumor incidence and the mean number of tumors per mouse ± SEM. Progressively growing masses of ≥1 mm in mean diameter were regarded as tumors. Growth was monitored until a first tumor exceeded an average diameter of 10 mm, at which time mice were killed for humane reasons. Probability significance of differences in tumor incidence was evaluated by the Fisher's exact test, and differences in tumor/mouse ratios (multiplicity) were evaluated by using a Mann–Whitney U test.
p53 Mutant Mice. A total of five B6 p53–/– founder parent pairs were used to generate progeny, and both vehicle and α-GalCer-treated groups comprised ≈50% male and 50% female mice. All mice were bred and maintained in a specific pathogen-free room of the Peter MacCallum Cancer Institute. Mice used in the study were monitored for health and weighed twice weekly. Any mouse with an abnormality (palpable mass, abdominal distension, weight loss >10%, ruffled fur) was killed, its age was recorded, and a postmortem analysis was performed. Spleen, liver, thymus, and lymph nodes were routinely examined by histology after fixing these tissues in formalin and, on occasion, also fresh frozen. Mean life span ± SEM was calculated, and probability of significance was determined by using a Mann–Whitney U test. The significance of proportions of tumors was calculated by using a Fisher's exact test.
Methylcholanthrene (MCA)-Induced Sarcoma. Groups of 15–20 male mice were inoculated s.c. in the right hind leg with 0.1 ml of corn oil containing 400 μg of MCA (Sigma), and mice were monitored weekly for the development of fibrosarcoma as described (29). The growth of some MCA-induced fibrosarcomas initiated in vehicle-treated or α-GalCer-treated B6 WT mice was monitored weekly and measured by a caliper square along the perpendicular axes of the tumors. The data were recorded as the mean tumor size (cm2, product of the two perpendicular diameters) ± SEM. Tumor-free mice did not develop sarcomas beyond 180 days.
α-GalCer and IL-12 Treatment Protocols. α-GalCer was kindly provided by Kirin Pharmaceutical Research Laboratories (Gunma, Japan) and prepared as described (24). Mice received 2 μg of α-GalCer (unless indicated in the Figure legends) in 0.2 ml i.p. A group of B6 WT mice was also treated weekly with 2 μg of α-GalCer i.p., and their spleen and liver mononuclear cells (MNC) and serum were collected. IL-12 (Genetics Institute, Cambridge, MA) suspended in PBS supplemented with 0.01% mouse serum albumin (Sigma) was administrated i.p. Mice received 5-day courses of 100 ng of IL-12 every 4 weeks from 5 weeks of age until 29 weeks of age.
Flow Cytometric Analysis. MNC were collected 24 h and 7 days after each α-GalCer or vehicle injection, prepared, and phenotyped on a FACSCalibur (Becton Dickinson) by three-color flow cytometry as described (18). All staining reagents [biotinylated isotype-matched control mAbs, anti-CD69 (H1.2F3), anti-CD8 (53-6.7) mAb; PE-conjugated anti-NK1.1 (PK136) mAb, Cy-Chrome-conjugated anti-CD3 mAb (17A2) and FITC-conjugated streptavidin] were obtained from PharMingen.
ELISA. Sera were collected 4 h, 24 h, or 7 days after each i.p. injection (on day 0, 7, 14, 21, or 28) of α-GalCer or vehicle. IFN-γ and IL-4 levels in the serum were evaluated by using specific ELISA kits (OptEIA Mouse ELISA kit, PharMingen) according to the manufacturer's instructions.
Results and Discussion
α-GalCer Protects Mice from Carcinogen-Induced Fibrosarcoma. A number of previous studies have addressed the importance of tumor immunity by inoculating mice with the chemical carcinogen MCA (28, 32, 34, 35). In addition, similar studies have defined an important protective role of both NKT and NK cells (29, 36). A network of events between NKT cells and NK cells has been inferred from further experiments using experimental tumors derived from MCA-inoculated mice (37). Some immunotherapeutic approaches have also been assessed in this carcinogen model, including long-term administration of recombinant IL-12 (38). To evaluate the antitumor activity of α-GalCer in this model, we initially tested the most effective means of weekly treatment by initiating therapy before, at the time of, or after MCA inoculation. As described (35), a dose of 400 μg of MCA was inoculated to ensure that most vehicle-treated B6 WT mice would succumb to sarcoma formation (Fig. 1a). More than 50% of mice that received 2 μg of α-GalCer weekly for 10 weeks after MCA inoculation (days 0–70) remained tumor-free after 250 days (Fig. 1a), demonstrating that α-GalCer could prevent the initiation of sarcoma by MCA. Surprisingly, mice that received α-GalCer from 4 weeks to 14 weeks after MCA inoculation were also similarly protected, suggesting that stimulation of the host immune system immediately after MCA inoculation was not critical to prevent tumor initiation. These data demonstrate that α-GalCer can prevent tumor initiation, and the level of protection compares favorably with IL-12 treatment that has been shown under similar conditions to delay, but not prevent, sarcoma induction (38). In concert with these data, mice that received α-GalCer from only days 0–28 also displayed a significant delay in the onset of MCA-induced sarcoma and some reduction in sarcoma frequency. By contrast, mice that were pretreated with α-GalCer from day –28 through day 0 displayed only a minor delay in the onset of tumor induction and the same incidence of sarcoma as vehicle-treated mice (Fig. 1a).
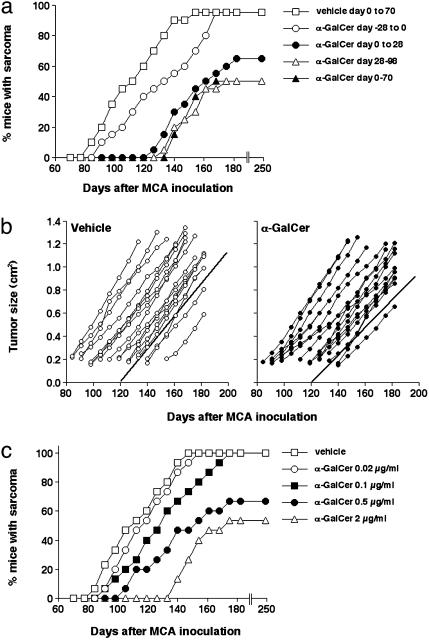
α-GalCer-mediated control of MCA-induced fibrosarcoma. (a) Groups of 20 B6 WT mice were injected s.c. in the hind flank with 400 μg of MCA diluted in 0.1 ml of corn oil. Mice received 2 μg of α-GalCer i.p. as follows: (i) weekly from day –28 to day 0 (where day 0 = day of MCA inoculation); (ii) weekly from day 0 to day 28; (iii) weekly from day 0 to day 70; (iv) weekly from day 28 to day 98. Mice were observed weekly for tumor development over the course of 60–200 days. Tumors >4 mm in diameter and demonstrating progressive growth over 2 weeks were counted as positive. (b) Representative individual fibrosarcomas from the group of 20 B6 WT mice receiving 400 μgof MCA and vehicle (Left) were compared for growth with a similar group of B6 WT mice inoculated with 400 μg of MCA and receiving α-GalCer treatment (2μg of α-GalCer weekly; Right), commencing in each mouse when sarcoma was palpated for the second successive week. Tumor size was measured weekly with a caliper square as the product of two diameters, and results were recorded as the tumor size (cm2). The lines of best fit of each group demonstrate growth rate, are represented by the solid lines, and are not significantly different (P = 0.1649, Mann–Whitney U test). (c) Groups of 15 B6 WT mice were injected s.c. in the hind flank with 400 μg of MCA diluted in 0.1 ml of corn oil and received 0.02–2.0 μg of α-GalCer i.p. (as indicated) weekly from day 0 to day 70.
In a parallel experiment, another group of B6 WT mice were inoculated with 400 μg of MCA, and α-GalCer treatment commenced in each mouse only when sarcoma was palpated for the second successive week. α-GalCer did not significantly reduce the growth of sarcomas in B6 WT mice compared with the growth of sarcomas in untreated B6 WT mice (P = 0.1649), and no tumors regressed in size (Fig. 1b). These data suggest that MCA-induced sarcomas established in WT mice were quite refractory to α-GalCer treatment, a finding in keeping with previous observations of natural immunoselection of resistant tumor variants (29, 39). A dose–response experiment performed with α-GalCer treatment for 10 weeks after MCA inoculation revealed that a dose of α-GalCer as low as 0.5 μg weekly still prevented tumor initiation; however, lower doses were less effective (Fig. 1c).
Given the immunomodulatory properties of α-GalCer, we assessed the activation, cytokine production, and expansion of lymphocyte subsets after single and multiple weekly injections of 2 μg of α-GalCer (Fig. 2). Consistent with previous reports (25), both NK cells and T cells expressed the very early activation antigen CD69 within 24 h of α-GalCer administration (data not shown), and CD69 expression was persistent for at least 7 days after the first and subsequent (up to five) α-GalCer inoculations (Fig. 2a). In particular, the proportion and number of NK cells was elevated in the liver (Fig. 2b) after one α-GalCer treatment, and greater proportions and numbers of CD8+ T cells were observed in the liver after multiple (five) treatments (Fig. 2b). Very similar activation and expansions were obtained for NK cells and CD8+ T cells in the spleen, which is a reflection of the effect of chronic α-GalCer treatment on the systemic immune system (data not shown). Greatly elevated levels of serum IFN-γ and IL-4 are a hallmark of early NKT cell activation, and these cytokines were detected within 4 h of the first α-GalCer treatment (Fig. 2c). NK cells have been shown to produce high levels of IFN-γ several days after NKT cell activation by α-GalCer (18). Interestingly, both IFN-γ and IL-4 remained elevated in the serum for at least 7 days after each subsequent treatment, and levels were further increased with each treatment, consistent with continued NKT cell, NK cell, and T cell activation (Fig. 2c). Overall, these data demonstrate the ability of chronic α-GalCer administration to maintain NK cell and T cell activation, expansion, and cytokine production.
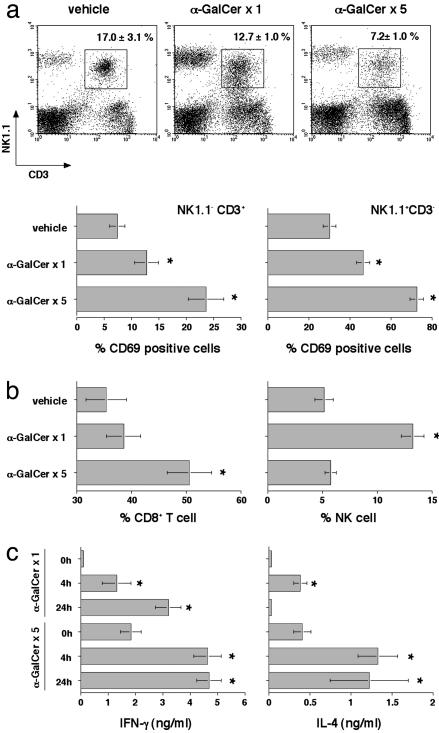
α-GalCer-mediated activation, cytokine production, and expansion of lymphocyte subsets. Groups of three B6 WT mice received either vehicle or 2 μg of α-GalCer i.p. on day 0 (α-GalCer ×1) or weekly from day 0 to day 28 (α-GalCer ×5). (a) Liver MNC were collected 7 days after each α-GalCer or vehicle injection, and the surface phenotype of the MNC was characterized by flow cytometry as indicated, NK1.1 vs. CD3 vs. CD69. (b) The percentage of CD8+ T cells and NK cells in the liver MNC were plotted. Cell numbers (×105) were as follows: CD8+ T cells; vehicle, 2.5 ± 0.3; α-GalCer × 1, 18.7 ± 1.5; α-GalCer × 5, 13.5 ± 1.1; CD3– NK1.1+ cells; vehicle, 1.2 ± 0.2; α-GalCer × 1, 16.1 ± 1.2; α-GalCer × 5, 4.8 ± 0.7; CD3+ NK1.1+ cells; vehicle, 4.1 ± 0.7; α-GalCer × 1, 15.4 ± 1.2; and α-GalCer × 5, 4.8 ± 0.7. (c) Sera were collected before (0 h), or4hor24h after a single (×1) or multiple (×5) i.p. injections of 2 μgof α-GalCer or vehicle. IFN-γ and IL-4 levels in the serum were evaluated by using ELISA, and statistical differences were determined by comparing levels with the 0-h time point for each treatment using a Mann–Whitney U test (*, P < 0.05). Levels of IFN-γ and IL-4 were below detection (<50 pg/ml) in vehicle-treated mice.
To further elucidate the importance of effector molecules in α-GalCer suppression of MCA tumor induction, the 10-week (days 0–70) treatment regimen was examined in mice deficient for TRAIL, pfp, and/or IFN-γ (Fig. 3). Despite the fact that both pfp and IFN-γ are important in natural immunity against MCA-induced sarcoma (32), at a dose of 400 μg of MCA all vehicle-treated mice developed sarcomas with similar kinetics, regardless of genotype. In concert with previous studies in experimental metastasis models (18, 24), the immunotherapeutic activity of α-GalCer critically required IFN-γ, was to some extent dependent on TRAIL, and was not dependent on pfp (Fig. 3). These data suggest a common mechanism of action of α-GalCer in suppression of tumor initiation and metastasis.
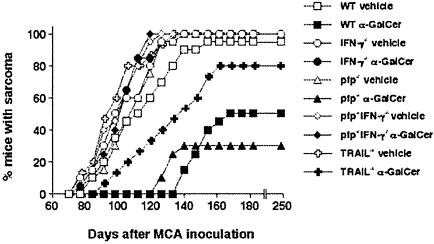
α-GalCer-mediated control of MCA-induced fibrosarcoma by innate immunity and IFN-γ activity. Groups of 15–20 B6 WT, B6 IFN-γ–/–, B6 TRAIL–/–,B6 pfp–/–,orB6pfp–/– IFN-γ–/– mice were injected s.c. in the hind flank with 400 μg of MCA diluted in 0.1 ml of corn oil. Mice received either vehicle or 2 μg of α-GalCer i.p. weekly from day 0 to day 70. All mice were observed weekly for tumor development over the course of 60–200 days. Tumors >4 mm in diameter and demonstrating progressive growth over 2 weeks were counted as positive.
α-GalCer Controls the Onset and Malignancy of Oncogene-Driven Malignancy. The Her-2/neu oncogene is overexpressed in a substantial proportion of human mammary carcinomas, and this overexpression correlates with particular aggressiveness (40). In Her-2/neu transgenic mice (BALB-neuT and CBf1-neuT mice), mammary carcinogenesis progresses through atypical hyperplasia to in situ lobular carcinoma and finally to invasive carcinoma (33), mimicking the progress of the human disease (41). We tested the ability of α-GalCer to delay mammary carcinogenesis by treating CBf1-neuT mice with 2 μg every 4 weeks from 5–29 weeks of age. This regimen was used to compare the efficacy of α-GalCer with that of IL-12, which was tested by using a similar monthly treatment protocol. Although CBf1-neuT mice treated with vehicle developed a palpable tumor in all 10 mammary glands by 36 weeks of age, α-GalCer both delayed the appearance of the first tumor (Fig. 4a) and reduced tumor multiplicity (Fig. 4b). In particular, all vehicle-treated mice developed one tumor by week 25, whereas two α-GalCer-treated mice were still tumor-free at week 31. At 36 weeks, α-GalCer-treated mice presented a mean of only 7.6 mammary glands with tumors. The level of inhibition by α-GalCer compared favorably with the efficacy of IL-12 in this model, in which all IL-12-treated mice had one palpable tumor at 30 weeks (versus 33 weeks for α-GalCer), and at 34 weeks IL-12-treated mice had a mean of 4.8 tumors (versus 6.8 tumors for α-GalCer-treated). Extensive testing of earlier time points and more regular treatment will be required to make a direct comparison of their efficacy. α-GalCer also demonstrated only modest activity against established mammary carcinomas from female BALB/c-neuT transgenic mice when they were transplanted s.c. into female BALB/c WT mice (data not shown).
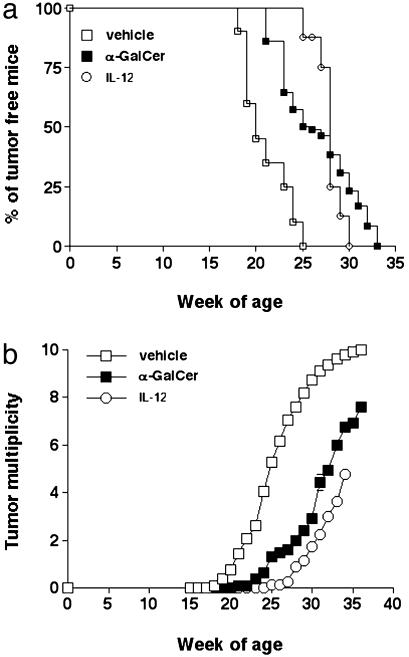
Appearance of the first tumor (a) and tumor multiplicity (b) in CBf1-neuT mice treated with α-GalCer and IL-12. Mice received 2 μg of α-GalCer (n = 13) or vehicle only (n = 20) i.p. at weeks 5, 9, 13, 17, 21, 25, and 29. Alternatively, a group of mice (n = 8) received 100 ng in 0.2 ml of IL-12/day for 5 days at weeks 5, 9, 13, 17, 21, 25, and 29. Progressively growing masses (>1-mm mean diameter) were regarded as tumors. Differences in tumor incidence at 25 weeks were statistically different between vehicle-treated and IL-12 or α-GalCer-treated mice, as evaluated by a Fisher's exact test (P < 0.0004). Tumor multiplicity was statistically different between vehicle-treated and IL-12- or α-GalCer-treated mice at week 25 and beyond, as demonstrated by using a Mann–Whitney U test (P < 0.004).
α-GalCer Controls Specific Malignancies Caused by Tumor Suppressor Loss. p53 mutations are found in 50–55% of all human cancers (42), and humans heterozygous for p53 have been defined in Li Fraumeni families (43). p53 gene-targeted mice are developmentally normal, but they develop a range of different tumors of epithelial, stromal, or hematopoietic origin, depending on their genetic background and whether one or two alleles are mutated (44, 45). In concert with previous studies of C57BL/6 p53–/– mice (46), approximately one-third of vehicle-treated mice developed thymic lymphomas, disseminated lymphomas, or sarcomas, whereas a minority (2 of 44) succumbed to other tumors (Fig. 5). α-GalCer was administered weekly from 4 weeks of age, and of the mice that developed sarcomas (13 of 44), the onset of growth was significantly delayed (192.6 ± 6.1 days) compared with vehicle-treated mice (147.1 ± 8.3 days; P = 0.0005). There was no apparent difference in the types of sarcomas arising in these groups (angiosarcoma, osteosarcoma, leiomyosarcoma). No significant delay in the mean onset of disseminated lymphomas (α-GalCer-treated, 210.1 ± 6.8 days and vehicle-treated, 186.8 ± 10.2 days, P = 0.0848) and thymic lymphomas (α-GalCer-treated, 163.5 ± 11.7 days and vehicle-treated, 158.6 ± 12.6 days) was observed in α-GalCer-treated mice (Fig. 5). It is not yet clear whether inoculated α-GalCer can effectively access the thymus, but it appears that NKT cell and NK cell secretion of IFN-γ is not as effective in suppressing lymphoid tumors compared with localized tumor masses. It is of interest that α-GalCer has considerable activity in preventing or delaying tumor initiation in two different models of spontaneous sarcoma formation. Although previous data support an antiangiogenic activity of α-GalCer through NK cell IFN-γ secretion (27), it remains to be determined whether sarcoma induction is particularly sensitive to control via angiogenesis. Further investigation into the molecular role of IFN-γ in these types of malignancies should be of great interest.
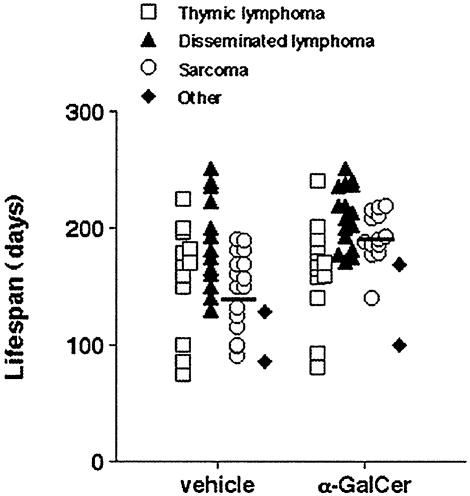
α-GalCer protects mice from spontaneous sarcoma. The appearance of tumors was recorded in groups of 34–35 B6 p53–/– mice receiving either vehicle or 2 μgof α-GalCer i.p. weekly from 4 weeks of age. Mice were evaluated on a weekly basis. When mice were moribund, tumor type (open squares, thymic lymphoma; filled triangles, disseminated lymphoma; open circles, sarcoma; filled diamonds, other tumors) was recorded against the age (in days) at the time of death/autopsy. The mean age of p53–/– mice developing spontaneous sarcoma is depicted by the cross bars, and the statistically later incidences of sarcomas in the α-GalCer-treated group were noted (192.6 ± 6.1 days), compared with vehicle-treated mice (147.1 ± 8.3 days; P = 0.0005, Mann–Whitney U test).
Conclusions
Recent preclinical studies have revealed significant efficacy of the marine sponge glycolipid α-GalCer for treatment of metastatic cancers and infections (6, 7, 47, 48) and for the prevention of autoimmune diseases (14–16). These findings suggest that appropriate stimulation of NKT cells could be exploited for modulation of human diseases. Nevertheless, the capacity of α-GalCer to inhibit tumor initiation had never, to our knowledge, been evaluated in mouse models of chemical- and oncogene-dependent carcinogenesis. In this study we demonstrated that weekly long-term administration of soluble α-GalCer prevented MCA-induced sarcoma and delayed the development of Her-2/neu mammary carcinomas and some spontaneous tumors in p53–/– mice. Although the latter tumors were only delayed in appearance, these examples are all aggressive models of carcinogenesis in which tumor development was completely predestined. Loss of both p53 alleles in all tissues, or expression of the Her-2/neu oncogene in the mammary gland, are two of the most extreme transforming events that can be inflicted on such cells. It is possible that α-GalCer can prevent sarcoma development in some p53+/– mice, but these experiments remain to be performed. A 2-μg dose of α-GalCer exceeds the dose required for optimal NKT cell activation in vivo in a single treatment, although clearly 2 μg weekly was more effective than 0.5 μg weekly in the MCA-induced sarcoma model. Both α-GalCer and IL-12 have dose-limiting toxicities in mice that would be approached when further increasing the treatment dose or frequency to more than once weekly. α-GalCer should be evaluated in additional mouse models of cancer as they become available, but our study has demonstrated the important principle that tumor initiation can be delayed or prevented (depending on the type and strength of the oncogenic event) by early α-GalCer treatment.
Despite previous observations that repeated soluble α-GalCer administration may anergize NKT cell IFN-γ production (49), NK cell and T cell activation was maintained, and the efficacy of weekly α-GalCer injections compared favorably with a variety of different IL-12 regimens used in the same models (33, 38, 50). Importantly, high sustained serum IFN-γ and IL-4 levels were achieved, and these cytokines may have been critical in obtaining protection from spontaneous tumor development (51). The dependence of α-GalCer activity on IFN-γ, but not pfp, suggests that this compound inhibits both tumor initiation and metastasis by similar mechanisms. α-GalCer did not require IL-4 to mediate antimetastatic activity (24); however, it remains to be examined whether chronic elevation of IL-4 levels plays any role in preventing tumor initiation. Future experiments are required to dissect the activated lymphocyte populations that sustain serum IFN-γ and IL-4 levels after chronic α-GalCer administration. Clearly, IFN-γ induction up to 4 weeks after MCA inoculation was still sufficient to suppress tumor development. In many models in which α-GalCer treatment suppresses tumor metastases or growth (e.g., B16 melanoma, Renca renal carcinoma), treatment with recombinant IFN-γ is somewhat effective, but limited in these models by short half-life and toxicity (52, 53). We believe the antitumor activity of α-GalCer cannot be mimicked in chronic treatment models by simple IFN-γ administration, and the cellular context of IFN-γ production is most likely relevant to the efficacy of α-GalCer. Although short-term soluble α-GalCer appears to inhibit metastases by an acute activation of NK cell function, it remains to be determined in long-term studies whether chronic α-GalCer treatment suppresses tumor initiation by NK cell activation and/or cytotoxic T lymphocyte induction. Previous experimental tumor models suggested that α-GalCer stimulated NK cell and CD8+ T cell antitumor activity after NKT cell activation. At this stage, we can only assume that these same effector cells play a role in delaying tumor formation in p53–/– and Her-2/neu transgenic mice after α-GalCer treatment.
α-GalCer is only a surrogate ligand for NKT cells, and the natural ligands that stimulate the antitumor and other activities of these cells remain an important unsolved mystery. Nevertheless, we have shown that powerfully stimulating NKT cells can be a viable means of delaying chemical- and oncogene-driven carcinogenesis. Clinical trials using soluble α-GalCer have commenced in humans with solid tumors (54), and there are several possibilities for the manipulation of NKT cells for tumor immunotherapy in humans. In particular, experimental studies in mice have illustrated that dendritic cells pulsed with α-GalCer more effectively induce antimeta-static activity than simple short-term α-GalCer administration, impressively inhibiting 7-day-established B16 melanoma nodules (49, 55). B16 melanoma is particularly sensitive to NK cells, and it will now be important to compare the efficacy and mechanism of soluble α-GalCer and dendritic cell pulsed α-GalCer in these chemical- and oncogene-dependent carcinogenesis models. Given the aggressive and suppressive functions of these cells, caution must be taken in their application (13). In addition, humans appear to have proportionally fewer NKT cells than mice; therefore, establishing a treatment regimen that similarly activates human NKT cells in patients remains a future challenge.
Acknowledgments
We thank the animal staff at the Peter MacCallum Cancer Centre and Ms. Susanna Massasso at the Department of Clinical and Biological Sciences, University of Turin, for their assistance with the experiments, and Dr. Dale Godfrey for his critical reading of this manuscript. This work was supported by fellowship and project grants from the National Health and Medical Research Council of Australia (to M.J.S.), a Cancer Research Institute post-doctoral fellowship (to Y.H.), the Italian Association for Cancer Research, and the Italian Ministry for University and Scientific and Technological Research.
Notes
This paper was submitted directly (Track II) to the PNAS office.
Abbreviations: α-GalCer, α-galactosylceramide; B6, C57BL/6; MCA, methylcholanthrene; pfp, perforin; TRAIL, tumor necrosis factor-related apoptosis-inducing ligand; NK cell, natural killer cell; NKT cells, NK1.1+αβTCR+ cells; MNC, mononuclear cells.
References
Articles from Proceedings of the National Academy of Sciences of the United States of America are provided here courtesy of National Academy of Sciences
Full text links
Read article at publisher's site: https://doi.org/10.1073/pnas.1630663100
Read article for free, from open access legal sources, via Unpaywall:
http://www.pnas.org/content/100/16/9464.full.pdf
Citations & impact
Impact metrics
Citations of article over time
Alternative metrics
Article citations
T Cell Receptor-Engaging Monoclonal Antibodies Mobilize the Anti-Tumor Functions of Invariant Natural Killer T Cells.
Crit Rev Oncog, 29(1):69-81, 01 Jan 2024
Cited by: 0 articles | PMID: 38421715
Enhanced antitumor efficacy of mesenchymal stem cells expressing cytosine deaminase and 5-fluorocytosine combined with α-galactosylceramide in a colon cancer model.
Am J Cancer Res, 13(6):2439-2451, 15 Jun 2023
Cited by: 2 articles | PMID: 37424825 | PMCID: PMC10326594
Ring Opening of Aziridines by Pendant Silanols Allows for Preparations of (±)-Clavaminol H, (±)-Des-Acetyl-Clavaminol H, (±)-Dihydrosphingosine, and (±)-N-Hexanoyldihydrosphingosine.
Org Lett, 24(33):6202-6207, 11 Aug 2022
Cited by: 7 articles | PMID: 35951966 | PMCID: PMC10017055
Adoptive Immunotherapy With Engineered iNKT Cells to Target Cancer Cells and the Suppressive Microenvironment.
Front Med (Lausanne), 9:897750, 09 May 2022
Cited by: 18 articles | PMID: 35615083 | PMCID: PMC9125179
Review Free full text in Europe PMC
Type 1 Innate Lymphoid Cells Limit the Antitumoral Immune Response.
Front Immunol, 12:768989, 16 Nov 2021
Cited by: 10 articles | PMID: 34868026 | PMCID: PMC8637113
Go to all (82) article citations
Data
Data behind the article
This data has been text mined from the article, or deposited into data resources.
BioStudies: supplemental material and supporting data
Similar Articles
To arrive at the top five similar articles we use a word-weighted algorithm to compare words from the Title and Abstract of each citation.
Critical contribution of IFN-gamma and NK cells, but not perforin-mediated cytotoxicity, to anti-metastatic effect of alpha-galactosylceramide.
Eur J Immunol, 31(6):1720-1727, 01 Jun 2001
Cited by: 125 articles | PMID: 11385616
Effects of cyclosporin A on the activation of natural killer T cells induced by alpha-galactosylceramide.
Transplantation, 83(2):184-192, 01 Jan 2007
Cited by: 6 articles | PMID: 17264815
Enhancement of the synthetic ligand-mediated function of liver NK1.1Ag+ T cells in mice by interleukin-12 pretreatment.
Immunology, 113(1):35-43, 01 Sep 2004
Cited by: 20 articles | PMID: 15312134
Innate Valpha14(+) natural killer T cells mature dendritic cells, leading to strong adaptive immunity.
Immunol Rev, 220:183-198, 01 Dec 2007
Cited by: 111 articles | PMID: 17979847
Review