Abstract
Free full text

Mature B cells class switched to IgD are autoreactive in healthy individuals
Abstract
Determination of the origin and fate of autoreactive B cells is critical to understanding and treating autoimmune diseases. We report that, despite being derived from healthy people, antibodies from B cells that have class switched to IgD via genetic recombination (and thus become class switched to Cδ [Cδ-CS] cells) are highly reactive to self antigens. Over half of the antibodies from Cδ-CS B cells bind autoantigens on human epithelioma cell line 2 (HEp-2) cells or antinuclear antigens, and a quarter bind double-stranded DNA; both groups of antibodies are frequently polyreactive. Intriguingly, some Cδ-CS B cells have accumulated basic residues in the antibody variable regions that mediate anti-DNA reactivity via somatic hypermutation and selection, while other Cδ-CS B cells are naturally autoreactive. Though the total percentage was appreciably less than for Cδ-CS cells, a surprising 31% of IgG memory cell antibodies were somewhat autoreactive, and as expected, about 24% of naive cell antibodies were autoreactive. We interpret these findings to indicate either that autoreactive B cells can be induced to class switch to IgD or that autoreactive B cells that use IgD as the B cell receptor are not effectively deleted. Determination of the mechanism by which the majority of Cδ-CS B cells are autoreactive may be important in understanding peripheral tolerance mechanisms and may provide insight into the enigmatic function of the IgD antibody.
Introduction
Autospecific B cells are normally controlled by clonal deletion (1, 2), receptor editing (3, 4), and clonal anergy (5, 6). Despite these mechanisms, B lymphocytes that express surface immunoglobulin that can bind to self antigens are fairly common in the mature human B cell repertoire (7, 8). Most knowledge about the mechanisms of B cell tolerance is derived from experiments with transgenic mice carrying pre-recombined autoantibodies; less is known about the fate of self-reactive B cells in humans. Because autoreactive B cells may be the precursors for the generation of pathological autoantibodies in various autoimmune diseases such as systemic lupus erythematosus (SLE) or rheumatoid arthritis, understanding their fate in healthy humans is critical. Evidence is presented herein that, in healthy people, B cells that are class switched to the IgD isotype are predominantly autoreactive.
In mature B cells that have not been antigen activated (naive cells), IgM and IgD antibodies are simultaneously encoded by the Cμ and Cδ exons via differential mRNA splicing of a single VDJ-Cμ-Cδ transcript. After activation, naive B cells can be induced to class switch. Immunoglobulin class switching specializes the function of antibodies through replacement of the IgM and IgD gene exons (Cμ and Cδ) with the IgG (Cγ), IgA (Cα), or IgE (Cε) exons by genetic recombination. However, a small proportion of B cells (1%–3%) actually class switch from Cμ to Cδ at the genetic level using cryptic switch regions between the Cμ and Cδ exons and resulting in an IgM–IgD+ phenotype. These are referred to as B cells class switched to Cδ (Cδ-CS) cells (9, 10) and appear to have resulted from an antigen-activated immune response because they can be isolated as GC cells, they are highly clonal (which suggests cell division), and the variable genes have extensive somatic mutations (11, 12). Cδ-CS B cells also predominantly express l-light chains and can differentiate into memory and plasma cells (13, 14). The predominant use of l-light chains suggests that most secreted IgD antibody in human sera (~1% of all antibodies) is from Cδ-CS B cells, as serum IgD is also predominantly l-light chain encoded (15). Cδ-CS B cells use a subset of immunoglobulin variable region genes long associated with autoreactivity (16) and may have been subjected to receptor editing during B cell development (12, 16, 17). These various observations suggest that Cδ-CS B cells are either generated by a mechanism of immune tolerance or they evade immune tolerance. Herein we set out to determine if indeed Cδ-CS B cells are autoreactive in healthy humans.
We found that human Cδ-CS B lymphocytes were autoreactive, as they encoded antibodies that often bind human epithelioma cell line 2 (HEp-2) cells, antinuclear antigens (ANAs), single-stranded DNA (ssDNA), double-stranded DNA (dsDNA), and they were frequently polyreactive. Consistent with a recent report from Tiller and colleagues, human IgG memory cells had low-intensity autoreactivity and were polyreactive at a surprisingly high frequency (8), although the frequency and intensity of autoantigen binding were significantly less than those of Cδ-CS B cells. Interestingly, anti-DNA reactivity was either naturally encoded by the unmutated variable genes or acquired during an immune response via somatic mutations. Thus, anti-DNA antibodies apparently resulting from an immune response might be able to arise in healthy people. These findings provide an important advance in understanding the tolerance mechanisms that control self-reactive B cells that may be disrupted in patients with autoimmune diseases.
Results
Determination of the autoantibody specificity of Cδ-CS B cells.
Cδ-CS B cells are a unique lineage found in tonsils and blood that are IgD+IgM– and can be isolated as GC (CD38+) or plasma (CD38++) cells from human tonsils (11, 14) (Figure (Figure1A)1A) or as memory cells (CD27+) from peripheral blood (13) (Figure (Figure1B).1B). In order to test the specificity of Cδ-CS B cells, recombinant monoclonal antibodies were produced from the variable region genes of isolated single cells. We used a modified strategy similar to that described in previous reports (7, 18), wherein single B cells were sorted by flow cytometry into 96-well plates and the variable genes amplified by multiplex, single-cell RT-PCR to identify and clone the immunoglobulin heavy-chain and light-chain genes from a random assortment of cells (the variable genes used are listed in Supplemental Table 1; supplemental material available online with this article; 10.1172/JCI27628DS1). These variable genes were then cloned into expression vectors and expressed with IgG constant regions in the 293A human cell line. A total of 100 antibodies were produced from Cδ-CS GC B cells (IgD+IgM–CD38+; Figure Figure1A)1A) isolated from 3 tonsil donors (by donor, n = 45, 34, and 21 antibodies) and compared with 78 antibodies from naive B cells from 3 donors (IgD+IgM+CD38–; by donor, n = 37, 27, and 14 antibodies) and 64 antibodies from IgG memory cells from 4 donors (IgD–IgM–CD27+; by donor, n = 25, 12, 7, and 20 antibodies). It is notable that the naive B cells as defined herein may have contained a minor population of B cells that were transitional cells but that still predominantly represented the naive repertoire of human B cells. It was important to avoid contaminating the naive B cells with IgM+D+ memory cells — because IgM memory cells from humans are infrequently autoreactive (19), contamination would cause us to underestimate the true frequency of autoantibodies detected. In addition to analyzing CD27– cells, to further avoid contaminating IgD+IgM+ memory cells, we only used antibodies from the naive cells that had no somatic mutations (i.e., that had less than the background frequency of 1 base exchange per variable gene). The identity of sorted Cδ-CS B cells was verified based on known characteristics of the variable gene repertoire including extensive somatic mutations (Figure (Figure1C)1C) (11) and abundant use of the JH6 gene segment (Figure (Figure1D)1D) (16). We were interested to know whether, as predicted by the findings of Klein and colleagues (13), variable genes from Cδ-CS peripheral blood memory cells are similar to Cδ-CS GC and plasma cells. Indeed, randomly cloned variable genes from Cδ-CS memory cells also had excessive somatic mutations and a preference for use of the autoimmune-associated JH6 gene segment (Figure (Figure1,1, C and D) (7, 16, 20). As described below, antibodies from Cδ-CS GC, naive, and IgG memory cells were tested for reactivity to HEp-2 cells by immunofluorescence and ANA binding by commercial immunosorbent assays and were screened by ELISA for reactivity to DNA or polyreactivity.
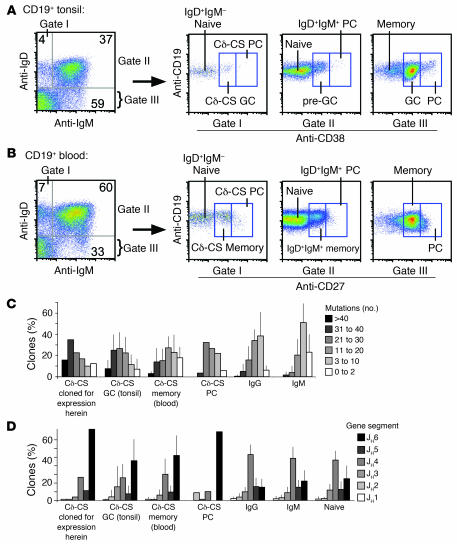
(A and B) Flow cytometry sorting gates used to isolate the various B cell types analyzed from blood or tonsils, including Cδ-CS GC cells (IgD+IgM–CD38+), naive B cells (IgD+IgM+ and CD38– tonsils or CD27– blood), and memory B cells (IgD–CD27+ from the “memory” box in B). PC, plasma cell. Analysis of somatic mutation frequency (C) and usage of the JH6 gene segment (D) demonstrate that all Cδ-CS B cell subsets and those expressed herein are similarly unique from IgG and IgM B cells. (C) Shown is the percentage of VH genes with the indicated number of mutations for each subset analyzed. (D) Use of various JH gene segments. Included are transcripts from 625 Cδ-CS GC cell variable genes from 11 donors (by donor, n = 44, 44, 57, 44, 62, 34, 225, 59, 16, 21, and 19), 78 Cδ-CS plasma cell variable genes from 1 donor, 124 Cδ-CS memory cell variable genes from 4 donors (by donor, n = 20, 20, 28, and 56), 620 IgG GC, memory, and plasma cell variable genes from 14 donors (by donor, n = 18, 28, 174, 40, 108, 37, 25, 21, 18, 22, 15, 24, 19, and 71), 681 IgM GC, memory, and plasma cell variable genes from 18 donors (by donor, n = 18, 91, 51, 158, 17, 10, 16, 48, 30, 19, 28, 11, 36, 29, 13, 22, 20, and 64), and 267 naive variable genes from 6 donors (by donor, n = 47, 30, 24, 15, 24, and 127).
Antibodies derived from Cδ-CS B cells bind self antigens.
The HEp-2 Slide assay is the classical clinical diagnostic test used to detect autoreactivity and ANA binding by sera antibodies as seen in autoimmune diseases such as SLE. This assay employs the use of cells from a human epithelioma cell line affixed to a microscope slide, and then detected by immunofluorescence after incubation with the sera (21). To determine if antibodies from Cδ-CS B cells bound to self antigens at a higher frequency than those from naive or memory B cells, antibodies from each population were tested for reactivity to antigens on the HEp-2 cell line. In addition, all antibodies were screened for binding to ANA using a commercial immunosorbent assay designed for detecting clinical ANA reactivity (QUANTA Lite ANA kit, INOVA Diagnostics Inc.). Antibodies that had detectable binding greater than that of a negative control sera in the HEp-2 immunofluorescence assay were scored as positive. For the ANA assay, an absorbance value was generated that allowed for a more quantitative assessment. Because the naive cells were the control population, we considered antibodies to be ANA positive if they produced absorbencies at the 25-μg/ml concentration that were greater than the mean ± SD of the absorbance of all naive cell antibodies. The mean ± SD absorbance of the naive antibodies was 0.4293 ± 0.2235 OD415, so positive tests had absorbencies of 0.6829 or greater. Previous reports have demonstrated that at concentrations of 25 to 50 μg/ml, about 70% of the antibodies from early immature B cells (prior to selection) bind HEp-2 cell antigens, whereas only 18% of antibodies from mature naive B cells have appreciable HEp-2 reactivity (7). We found a similar frequency of HEp-2 and ANA reactive antibodies from naive cells tested (16 of 70, or 23%; Figure Figure2A).2A). Surprisingly, but consistent with a recent report on human IgG antibody reactivity (8), 27% (17 of 64) of the antibodies from IgG memory B cells were HEp-2 or ANA reactive. In contrast, 60% (53 of 89) of the Cδ-CS–derived antibodies reacted with HEp-2 or ANA antigens. The increase in frequency of HEp-2 cell reactive antibodies from Cδ-CS cells compared with those from naive cells or IgG memory cells was highly significant both when all antibodies were compared as a whole (Figure (Figure2A;2A; χ2, P < 0.0001) and when compared by average frequency between donors (Figure (Figure2B;2B; Student’s t test, P < 0.05 versus naive or IgG antibodies).
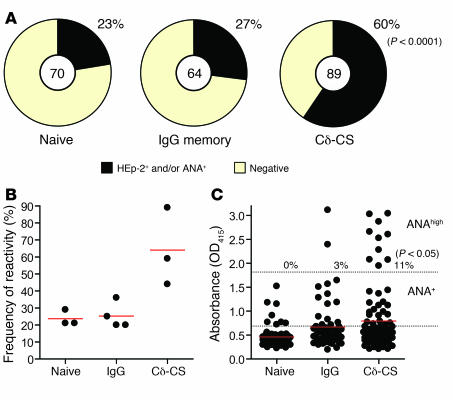
(A) Cδ-CS–derived antibodies are highly reactive to HEp-2 and ANA antigens compared with naive- and IgG-derived antibodies. Antibodies tested included 89 Cδ-CS antibodies (from 3 donors, n = 37, 18, and 34), 70 naive B cell antibodies (from 3 donors, n = 39, 14, and 17), and 64 antibodies from IgG memory cells (from 4 donors, n = 25, 5, 12, and 20). The frequency of HEp-2 reactive antibodies was determined by screening the antibodies by immunofluorescence using commercially prepared HEp-2 slides (Supplemental Figure 1), and the frequency of ANA reactive antibodies using commercial ANA immunosorbent assays (see Methods). Antibodies that bound HEp-2 slides more intensely than negative control serums provided by the manufacturer or that bound ANA more intensely than the mean ± SD of all naive cell antibodies were considered positive in the 2 assays. Results indicated that the incidence of autoreactivity was significantly higher in Cδ-CS–derived antibodies than in naive- or IgG-derived antibodies (χ2, P < 0.0001). (B) Frequency of HEp-2 and ANA reactivity by donor. Cδ-CS antibodies bound more often than IgG or naive cell–derived antibodies (Student’s t test, P < 0.05). (C) Although IgG cells were generally more reactive in the ANA assays than were naive cells, the reactivity was low intensity. In contrast, significantly more Cδ-CS antibodies bound to ANA with high intensity. Red lines indicate mean ANA binding absorbances. Dashed lines indicate the thresholds for positive scoring (lower dashed line indicates ANA+ that is the naive cell mean ± SD; upper dashed line indicates ANAhigh); the high absorbance level was achieved more commonly for Cδ-CS antibodies.
The Cδ-CS antibodies also bound to the ANA (Figure (Figure2C)2C) and to HEp-2 antigens (Supplemental Figure 1) with greater intensity than the naive or IgG memory cell antibodies. Eleven percent of the Cδ-CS antibodies tested bound ANA with particularly high intensity (ANAhigh) compared with 3% of the IgG and none of the naive antibodies (Figure (Figure3C;3C; χ2, P = 0.05). It is notable that approximately half of the IgG antibodies had low-level binding to the ANA antigens that was greater than that of the naive antibodies but not beyond a SD of the mean and so were scored as negative. This slight reactivity of IgG memory cells has recently been show to be due to accumulated somatic mutations (8) and caused an overall increased average absorbance. The Cδ-CS cells in turn had the greatest average ANA binding intensity (Figure (Figure2C;2C; 0.4593 ± 0.2235 OD415 for naive cells, 0.6691 ± 0.5092 OD415 for IgG, and 0.8004 ± 0.6906 OD415 for Cδ-CS antibodies). Finally, the HEp-2 autoantigens bound were variable and included cytoplasmic as well as classic ANA patterns (Supplemental Figure 1), thus the self reactivity is not targeted to a single autoantigen. In conclusion, antibodies from Cδ-CS B cells commonly bind various antigens on HEp-2 cells and in commercial ANA assays, demonstrating that they are commonly autoreactive.
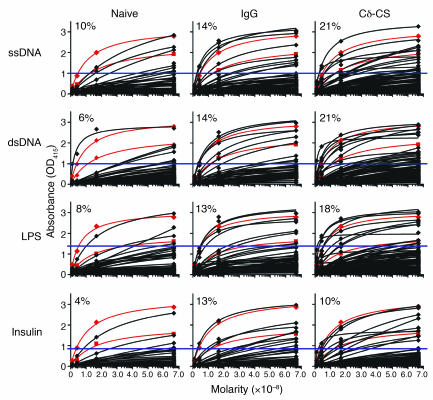
The degree of binding to antigen-coated microtiter plates (absorbance [OD415]) was measured at antibody concentrations of 6.67 × 10–8, 1.67 × 10–8, 4.17 × 10–9, and 1.04 × 10–9 M (which is 1 μg/ml and three 4-fold serial dilutions, x axis). All ELISA include 3H9 (red lines with diamonds) and H241 (red lines with squares) monoclonal antibodies with high and medium anti-DNA or polyreactivity, respectively. The assays were normalized based on the absorbencies of the 3H9 antibody. Percentages indicate the number of antibodies scored as positive. A total of 78 antibodies from naive B cells isolated from 3 donors (by donor, n = 37, 27, and 14 antibodies) were compared with 100 antibodies from Cδ-CS B cells from 3 donors (by donor, n = 45, 34, and 21 antibodies) and 64 antibodies from IgG memory cells from 4 donors (by donor, n = 25, 12, 7, and 20 antibodies). Blue lines represent the approximate positive thresholds determined by calculating the mean ± 2 SD of the naive antibodies (see Results).
Antibodies from human Cδ-CS B cells frequently bind to single-stranded and double-stranded DNA.
A hallmark of autoreactivity in SLE is the production of antibodies that react to DNA, particularly dsDNA. In order to further evaluate the potential autoreactivity of the Cδ-CS lineage compared with naive or IgG memory B cells, DNA binding was evaluated by ELISA. DNA binding was tested for the various antibodies at 1 μg/ml and 3 additional 4-fold serial dilutions. Saturation binding curves are presented in Figure Figure3.3. Anti-DNA binding affinity was determined by curve fitting. Antibodies with absorbencies calculated for the 1 μg/ml concentration that were 2 SD above the mean absorbance of 95% of the naive antibodies at that concentration were scored as anti-DNA positive (Figure (Figure3).3). For comparison using the same expression system, the variable genes encoding 2 well characterized antibodies that are commonly used to study anti-DNA reactivity were produced as chimeric mouse/human IgG-κ recombinant monoclonals (Figure (Figure3),3), including 3H9, used as a transgene to discover receptor editing (3), and H241 (22). Thus, these classic anti-DNA and polyreactive antibodies could be directly compared with identical detection reagents to the human antibodies tested herein. All ELISAs were normalized to the 3H9 control antibody included on each ELISA plate.
Antibodies reactive to ssDNA are more common in individuals with autoimmune conditions than in healthy individuals; however, they are not diagnostic of a pathological state. As indicated in Figure Figure3,3, 8 of 78 (10%) of the antibodies produced from naive B cells were reactive to ssDNA. This is consistent with a previous report by Wardemann et al. in which antibodies from 93 naive B cells were analyzed of which several bound ssDNA (7). A greater frequency (9 of 64, or 14%) of antibodies from IgG memory cells bound ssDNA. In contrast, 21% (21 of 100) of the Cδ-CS antibodies reacted to ssDNA (Figure (Figure3;3; χ2, P = 0.05 for Cδ-CS versus naive; P = not significant for Cδ-CS versus IgG memory).
More important than antibodies to ssDNA are antibodies to dsDNA, as they are more likely indicative of pathology (23, 24). Antibodies reactive to dsDNA were less frequent from naive (6%, or 5 of 78) and IgG memory B cells (14%, 9 of 64; Figure Figure3)3) than from Cδ-CS B cells. Antibodies from Cδ-CS cells were 2-fold more likely to bind dsDNA than antibodies from naive cells (21 of 100, or 21%; χ2, P = 0.006 for Cδ-CS versus naive). Again, because IgG antibodies tend to be somewhat polyreactive (8), although 50% increased, Cδ-CS antibodies did not bind dsDNA significantly more frequently. The variability between donors for all assays of autoreactivity is depicted in Figure Figure4A.4A. As noted previously by Tiller and colleagues, the IgG memory compartment has widely varying levels of anti-DNA reactivity (8). Antibodies that bound dsDNA were also tested for binding to the kinetoplasts of Crithidia luciliae, as this assay is more stringent and is considered the gold standard for detecting dsDNA reactivity in the clinical diagnosis of SLE. In general, there was good correspondence with our results from the ELISA, in that the antibodies with higher affinity for dsDNA by ELISA also bound Crithidia kinetoplasts (data not shown). Thus, similar to the results of the ELISA assays, one of the naive and several of the IgG anti-DNA antibodies bound Crithidia kinetoplasts with high intensity and another of the IgG antibodies had only slightly detectable binding. Also as predicted by the ELISA assays, 10 of the Cδ-CS antibodies bound Crithidia kinetoplasts and several others had detectable but low-intensity binding. From these experiments, we conclude that Cδ-CS B cells are more often reactive to ssDNA and dsDNA than are naive B cells, and Cδ-CS B cells tend to be more reactive than IgG antibodies.
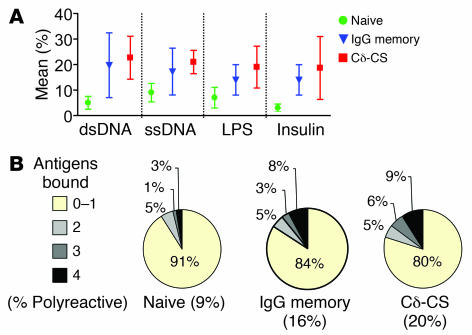
(A) Mean ± SEM for donors for the various assays performed. (B) The percentage of antibodies from each group (Cδ-CS B cells, naive B cells, or IgG memory B cells) that bound 0–1, 2, 3, or all 4 antigens tested (ssDNA, dsDNA, LPS, and insulin). Polyreactivity is defined as the binding of more than 1 antigen. The yellow portion of the chart indicates the percentage that bound up to 1 antigen and so were not polyreactive. Statistical significance (χ2, P = 0.04) was reached for Cδ-CS versus naive B cell antibodies.
Antibodies from Cδ-CS B cells are commonly polyreactive.
To determine whether Cδ-CS B cells tend to express polyreactive antibodies that interact with multiple self and non-self antigens, we also tested reactivity to recombinant human insulin and lipopolysaccharide from E. coli. As indicated in Figure Figure4B,4B, significantly more Cδ-CS cell– than naive cell–derived antibodies displayed polyreactivity, but not more than IgG cell–derived antibodies. Whereas 20% (20 of 100) of the Cδ-CS cell–derived antibodies bound at least 2 of the antigens by ELISA, significantly fewer antibodies from naive cells (9%, or 7 of 79; χ2, P = 0.039) interacted with 2 or more antigens. For IgG memory cells the incidence of polyreactivity was less than that of Cδ-CS (16% versus 20%, or 10 of 64 versus 20 of 100); however, significance was not reached. In conclusion, antibodies from B cells that had class switched to IgD or IgG were commonly polyreactive.
Altogether, considering the high frequency of HEp-2 and ANA reactivity and the frequency of anti-DNA and polyreactivity, we concluded that 56% Cδ-CS antibodies were autoreactive. Thus Cδ-CS cells were about 2-fold more frequently autoreactive than naive cell antibodies (24% autoreactive, χ2, P < 0.0001) and 1.8-fold more frequent than IgG cell–derived antibodies (31% autoreactive, χ2, P < 0.001). From this analysis, we concluded that Cδ-CS B cells are usually autoreactive.
IgD class switching occurs both in B cells with natural autoantibodies and for cells with autoreactivity generated by somatic hypermutations. It is possible that a class switch to IgD occurred only for autoreactivity introduced during immune responses due to the excessive somatic mutations characteristic of Cδ-CS B cells (Figure (Figure1C)1C) (11). A recent report from Tiller and colleagues found a surprisingly high frequency of IgG memory B cells that are polyreactive, and the reactivity was typically caused by accumulated somatic hypermutations (8). Alternatively, it is plausible that a class switch to IgD may have occurred prior to somatic mutation and independent of a normal GC reaction. Consistent with this conjecture, we have previously demonstrated that Cδ-CS B cells accumulate targeted somatic mutations that disrupt the natural autoreactivity of antibodies encoded by the VH4-34 gene segment (16). This finding suggested that the accumulation of excessive somatic hypermutations in the variable genes of Cδ-CS B cells may have resulted from selective pressure to alter amino acids causing natural autoreactivity as well as increasing affinity to foreign antigens. In order to gain insight into the origin of autoreactive B cells that are class switched to IgD, we characterized the role of somatic mutation in mediating the autoreactivity. The fact that 11 of 100 (11%) of the Cδ-CS antibodies expressed were from unmutated variable genes allowed us to determine if B cells that were class switched to IgD could have natural autoreactivity (not due to mutations). Note that the variable genes encoding these transcripts were otherwise similar to that of most Cδ-CS antibodies with preferential use of the JH6 gene segment, long complementarity determining regions 3 [CDR3s], and l-light chain usage (data not shown). As indicated in Figure Figure5A,5A, the frequencies of HEp-2 antigen autoreactivity, binding to dsDNA, and polyreactivity were similar in mutated and unmutated antibodies from the Cδ-CS B cells and were higher than the frequency of autoantibodies from naive cells and IgG memory cells. This analysis also provided verification that these Cδ-CS clones with unmutated variable-region genes were not contaminating naive cells. In addition, comparison of anti-DNA titers with the frequency of amino acid replacements indicated that there was no correlation between the affinity for dsDNA and the accumulation of somatic mutations, as titers even tended to be higher for the several clones without mutations (Figure (Figure5B).5B). From these observations, we concluded that cells class switched to IgD can express naturally (germline-encoded) autoreactive antibodies independent of somatic hypermutation.
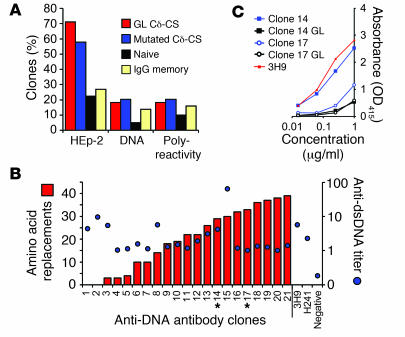
(A) Cδ-CS antibodies encoded by unmutated (germline) variable genes display levels of HEp-2 autoreactivity, DNA binding, and polyreactivity similar to those from somatically mutated variable genes. Shown are the percentages of antibodies from each subpopulation. (B) Binding curves of 1 μg/ml anti-DNA antibodies from Cδ-CS B cells to DNA were used to calculate absorbencies (blue dots). Comparison of these absorbencies with the frequency of amino acid replacements (red bars) showed that there is no correlation between the affinity for dsDNA and the accumulation of somatic mutations. The variable genes of clones 14 and 17 (asterisks) were reverted to their germline sequences to determine whether the somatic mutations might cause DNA binding. (C) DNA binding is lost when 2 anti-DNA Cδ-CS antibodies (clone 14, blue square; clone 17, blue circle) were expressed from variable genes reverted to the germline unmutated sequences (clone 14, black square; clone 17, black circle). Anti-DNA binding was evaluated by ELISA (absorbance [OD415]) relative to the control 3H9 monoclonal antibody with high affinity for DNA (red line). GL, germline.
Arginine is considered the most important residue for anti-DNA binding (25, 26). The antibodies with natural reactivity to DNA and a number of the other antibodies had arginines introduced to the CDR3 by VDJ recombination (Cδ-CS clones 1 and 2 were germline, also note that arginines were introduced in the CDRs of clones 4, 6, 8, 10, 11, 12, 14, and 21; Supplemental Figure 2). An important observation was that most of the Cδ-CS clones with anti-DNA reactivity also had arginines that were introduced because of codon alterations that resulted from somatic mutation (Supplemental Figure 2). In fact, 15 of 22 (68%) of these Cδ-CS dsDNA binding clones had as many as 7 arginines introduced by mutation into the heavy and/or light chains (clone 18 had the most arginines introduced; Supplemental Figure 2). This suggested that the autoreactivity we observed may have arisen as a result of somatic mutations introduced during immune responses. In order to explore this possibility, we generated and expressed the unmutated counterparts of the variable genes of 2 of the anti-DNA antibodies from Cδ-CS cells (clones 14 and 17; Figure Figure5B).5B). Neither of the unmutated variants bound dsDNA, demonstrating that the anti-DNA reactivity that we observed arose as a result of somatic hypermutation (Figure (Figure5C).5C). Thus, anti-DNA antibodies that eventually cause autoimmune disease may commonly arise as the result of antigen-specific immune responses in healthy people. Taken together, these analyses suggest that either Cδ-CS B cells can be either naturally autoreactive or that their autoreactivity can arise due to amino acid changes introduced by somatic hypermutation.
Discussion
Several possibilities exist for the high rates of autoreactivity found in Cδ-CS B cells. It is believed that class switching to IgD and the resulting Cδ-CS B cells arise during GC reactions because they typically express highly mutated variable genes, they are clonally expanded, and they can only be isolated as GC, memory, or plasma cells (11, 13, 14, 16). We were not able to discern whether B cells that are autoreactive are induced to class switch to IgD or whether IgD-expressing B cells that are autoreactive are preferentially selected. In either case, a number of hypothetical models can be envisioned. First, there may be a directed mechanism by which the cryptic Cδ switch region is targeted in activated human B cells that are self reactive. Such a mechanism may have evolved to rescue autoreactive B cells for some specialized or reduced role in immunity involving IgD. It is interesting to consider that the elusive function of IgD may be uniquely involved in self tolerance (reviewed in ref. 15). However, analyses of transgenic mice expressing IgD or IgM alone as autoantibodies found little difference between IgD and IgM in B cell tolerance or negative selection (27). In fact, a number of studies suggest that IgD augments IgM signaling to positively select B cells into the functional repertoire (reviewed in refs. 15 and 28). With these studies in mind, several other models can be envisioned in which IgD does not have a specific role in tolerance. One possibility is that autoreactive B cells may be inhibited from class switching to the downstream Ig isotypes (i.e., IgG, IgA, IgE), leaving only the cryptic Cδ switch region available for switch in cells that previously bound self antigen. This inhibition of class switch may occur as a manifestation of anergy. Both of the above models are interesting because they each suggest that tolerizing mechanisms can directly control immunoglobulin class switch.
An alternative hypothesis is that class switch to IgD is random (i.e., any cell can switch), and that selection of Cδ-CS B cells occurs after class switch, causing preferential survival of the autoreactive clones. For example, it has been suggested that, compared with IgM receptors alone, expression of IgD provides preferential survival (29) and recruitment of B cells to immune responses that might allow for rescue by chronic stimulation with self antigen (30). Another possible explanation is that, when stimulated by self antigens or without the accessory signals that are normally required, expression of IgD only on the B cell surface may not result in B cell death. Elucidation of the actual mechanism that causes Cδ-CS B cells to be autoreactive might provide important insights into the role of IgD as a B cell receptor and into peripheral mechanisms of immune tolerance.
IgG memory B cells commonly express low-affinity autoantibodies (8), mostly as a result of changes from accumulated somatic mutations. In our study, 31% of IgG antibodies had detectable autoreactivity. It is notable that recent analyses have found that antibodies from IgM+ memory B cells were rarely autoreactive, but when autoreactivity was found it was caused by accumulated somatic mutations (19). Similarly, most autoreactivity seen in IgG+ memory B cells from healthy people is caused by somatic mutations (8). It is known that IgG+ memory B cells have accumulated approximately 2-fold the frequency of point mutations as IgM memory cells (31) and correspondingly are more often autoreactive (8). Cδ-CS B cells in turn have accumulated the most point mutations, averaging 21 ± 7 base exchanges per VH gene compared with 14 ± 5 per gene for IgG and 8 ± 4 mutations per gene for IgM GC and memory cells (Figure (Figure1C).1C). Concurrently, Cδ-CS B cells are also the most often autoreactive, though unlike IgG and IgM memory cells, the 10% of Cδ-CS B cells that are not mutated are still autoreactive (Figure (Figure5A).5A). The mildly mutated IgM memory compartment may be more reminiscent of the naive B cell repertoire that is then further selected after antigen interaction, thus reducing the overall frequency of autoantibodies (19). In contrast, excessively activated B cells that accumulate more mutations and eventually class switch may be selected to avoid pathological autoreactivity, as evidenced by preferential differentiation to Cδ-CS B cells, but nevertheless, further divergence from germline does result in an increased frequency of IgG memory B cells that are autoreactive and polyreactive. Culton and colleagues recently reported that there is a selective checkpoint that removes autoreactive B cell clones during the transition to plasma cells (32). This checkpoint could suppress the ability of autoreactive IgG memory cells to become antibody secreting cells in healthy persons. One final conjecture is that there may be qualitative differences between autoreactive IgG and Cδ-CS antibodies, as suggested by the greater intensity of binding of Cδ-CS antibodies to ANA (Figure (Figure2C)2C) and HEp-2 antigens (Supplemental Figure 1). For example, the low-intensity potential autoreactivity of the IgG polyreactive antibodies might be absorbed or masked by nonspecific interaction with other molecules in serum and therefore not represent a significant threat to self but still be a threat to the antigen for which they were selected to bind with high affinity. In contrast, specificities that are selected in Cδ-CS B cells may be more blatantly autoreactive. A more in-depth analysis of IgG specificity compared with Cδ-CS specificity and affinity to autoantigens is required to clarify this possibility.
In conclusion, the findings herein demonstrate that Cδ-CS (IgD-only) B cells have a high rate of autoreactivity in healthy persons. Characterization of the mechanism that causes the majority of Cδ-CS B cells to be autoreactive may provide important advances in our understanding of peripheral mechanisms of immune tolerance and perhaps a better understanding of the elusive function of the IgD isotype.
Methods
B lymphocyte isolation and cytometry.
B lymphocytes were isolated from human tonsils or blood as previously described (11, 14, 31). All tissues and blood were isolated following established NIH guidelines with institutional review board approval from the Oklahoma Medical Research Foundation Institutional Review Board (IRB) (blood and tonsils) and The University of Oklahoma Health Sciences Center IRB (tonsils). Tonsils were obtained from The University of Oklahoma Children’s Hospital during routine tonsillectomy and blood was from the Oklahoma Blood Institute, Oklahoma City, Oklahoma, USA. Use of tonsil tissue and blood was exempt from written consent. All tonsil donors were healthy children with a history of inflamed tonsils (but without any current inflammation). Blood was from young adult volunteers. Briefly, individual cells were teased from the tonsils. B cell–enriched fractions were purified using RosetteSep reagents (StemCell Technologies Inc.) and a lymphoprep gradient (CellGro; Mediatech Inc.) with sheep rbc added. B cells were then enriched to near purity by magnetic bead depletion using the Miltenyi Biotec B Cell Isolation kit II. Naive B cells were isolated as IgD+IgM+CD38– (tonsil) or CD27– (blood) and Cδ-CS B cells were isolated as IgD+IgM–CD38+, and peripheral blood IgG memory cell transcripts were amplified by RT-PCR from sorted total memory cells (IgD–IgM–CD27+; Figure Figure1B)1B) using a Dako Cytomation MoFlo or a BD FACSAria flow cytometer. Plates of both Igκ+ and Igλ+ from naive and Cδ-CS cells were sorted from 2 of the donors. Because the Igκ and Igλ fractions were similar for all assays, the fractions were grouped for the analyses herein. As predicted, most Cδ-CS B cells were Igκ negative (Igλ+). Bulk cells of the 3 phenotypes were sorted and resorted into 96-well PCR plates to ensure purity of single cells (98%–99% purity detected on sort of the single cells). Antibodies used for flow cytometry were anti-IgM–conjugated to APCs (Southern Biotechnology Associates), anti-IgD–conjugated to PE (BD), biotinylated anti-CD38 followed by streptavidin–conjugated to tricolor (Invitrogen), anti-Igκ–conjugated to FITC (Invitrogen), and anti-CD27–conjugated to PE (Invitrogen).
Single-cell RT-PCR and PCR.
Single B cells were sorted into PCR plates containing 10 mM Tris-HCL with 40 units/μl of RNase inhibitor (Promega). The plates were immediately frozen on dry ice and stored at –80°C. Each cell was amplified in a 1-step RT-PCR reaction (Qiagen) using a cocktail of sense primers specific to the leader regions and antisense primers specific to the Cμ- (naive), Cδ- (Cδ-CS), or Cγ-constant regions for heavy chains and Cκ-constant or Cl-constant regions for the light chains. One microliter from each RT-PCR reaction was amplified in separate PCR reactions for the individual heavy- and light-chain gene families using nested primers. All primer sequences have been previously published (7, 18) except for the anti-Cδ primers (external, 5′-GTGTCTGCACCCTGATATGATGG-3′, nested, 5′-GGGAACACATCCGGAGCCTTG-3′) and the anti-Cγ primers (external, 5′-TCTTGTCCACCTTGGTGTTGCT-3′, nested, 5′-AGGTGCTCTTGGAGGAGGGT-3′). PCR products were sequenced (Applied Biosystems 3730 DNA Analyzer). Upon identification of the variable genes, sense primers unique to the particular variable genes and antisense primers binding the particular junctional genes were used to amplify 1 μl of the RT-PCR reaction to incorporate restriction sites at the ends of the variable genes for cloning and expression. Some of the variable gene sequences presented for comparison in Figure Figure1,1, C and D, were from historical data previously published by our laboratory (12, 16, 33, 34). To generate the reverted variable genes for the experiments in Figure Figure5,5, we used strand-overlap extension PCR of unmutated variable-heavy, variable-light, and J templates and overlapping primers encoding the CDR3 regions with gene-specific V and J primers.
Recombinant monoclonal antibody expression.
Following purification and digestion, the amplified cDNAs of the antibody variable genes from each single cell were cloned into expression vectors containing human IgG, Igκ, or Igλ constant regions as previously described (7). Maxi prep plasmids Plasmid Maxi Kit (Qiagen) containing the heavy- and light-chain Ig genes were cotransfected into the 293A cell line using calcium phosphate or Roche Applied Science FuGENE 6 transfection reagent as per the manufacturer’s suggested protocol. Transfected 293A cells were allowed to secrete antibodies in serum-free DMEM supplemented with 1% Nutridoma-SP (Roche) for 4 to 5 days. Antibodies were purified using immobilized protein A columns (Pierce). Proper antibody expression and purity were verified by polyacrylamide gel electrophoresis, and purified antibody concentrations were determined using the EZQ Protein Quantitation Kit (Invitrogen).
ELISA and HEp-2 analyses for autoreactivity.
To screen the expressed antibodies for DNA reactivity or polyreactivity, ELISA microtiter plates (Costar; Corning Inc.) were coated with 10 μg/ml calf thymus ssDNA or dsDNA (Invitrogen), LPS (Sigma-Aldrich), or recombinant human insulin (Fitzgerald). γ-chain–specific goat anti-human IgG–peroxidase conjugate (Jackson ImmunoResearch Laboratories Inc.) was used to detect binding of the recombinant antibodies, followed by development with horseradish peroxidase substrate (Bio-Rad). Absorbances were measured at OD415 on a microplate reader (Molecular Devices).
The antibodies were screened for HEp-2 reactivity by immunofluorescence using commercial HEp-2 slides as per the manufacturers suggested protocol (BION Enterprises Ltd.). HEp-2 slides were analyzed using a Zeiss Axioplan II fluorescent microscope. The antibodies were screened for ANA binding using the QUANTA Lite ANA kit (INOVA Diagnostics Inc.) and detected using goat anti-human IgG (γ-chain specific) peroxidase-conjugate (Jackson ImmunoResearch Laboratories Inc.) followed by development with horseradish peroxidase substrate (Bio-Rad). All HEp-2 and ANA reactivity was compared to positive control sera from a lupus patient and to nonreactive sera from a healthy blood donor. The QUANTA Lite ANA assays were normalized to an in house ANA+ monoclonal antibody (mAb: 050504P181L+B09).
Statistics.
Statistical analyses were performed using Microsoft Excel 2003 or GraphPad Prism 4 software. For the analyses in Figure Figure1,1, A and B, and Figure Figure2B,2B, 2-tailed Student’s t tests were performed. For the analyses in Figure Figure2,2, A and C, Figure Figure3,3, and Figure Figure4B,4B, χ2 tests were performed.
Acknowledgments
We thank Judith A. James for critically reading the manuscript and providing valuable suggestions. We thank Larry Wysocki for providing, and Martin Weigert and David Stollar for allowing us to use, the 3H9 and H241 anti-DNA positive control monoclones. Technical assistance was provided by Leni Abraham and Sheryl Christopherson from the Oklahoma Medical Research Foundation (OMRF) DNA Sequencing Facility, the staff of the OMRF Imaging Core Facility, and Jacob Bass and Diana Hamilton in the OMRF Flow Cytometry Core Facility. We also thank the University of Oklahoma Children’s Hospital and particularly Sarah Johnson and Jesus Medina for making tonsil specimens available. Lisa Ridgeway provided clerical assistance. This work was funded in part by NIH grants P20RR018758-01 and P20RR15577-02 to P.C. Wilson.
Footnotes
Nonstandard abbreviations used: ANA, antinuclear antigen; Cδ-CS, class switched to Cδ; CDR, complementarity determining region; dsDNA, double-stranded DNA; HEp-2, human epithelioma cell line 2; ssDNA, single-stranded DNA.
Conflict of interest: The authors have declared that no conflict of interest exists.
Citation for this article: J. Clin. Invest. 117:1558–1565 (2007). 10.1172/JCI27628
Kristi Koelsch and Nai-Ying Zheng contributed equally to this work.
References
Articles from The Journal of Clinical Investigation are provided here courtesy of American Society for Clinical Investigation
Full text links
Read article at publisher's site: https://doi.org/10.1172/jci27628
Read article for free, from open access legal sources, via Unpaywall:
http://www.jci.org/articles/view/27628/files/pdf
Citations & impact
Impact metrics
Article citations
Human antibody polyreactivity is governed primarily by the heavy-chain complementarity-determining regions.
Cell Rep, 43(10):114801, 10 Oct 2024
Cited by: 0 articles | PMID: 39392756 | PMCID: PMC11564698
Invasion of spontaneous germinal centers by naive B cells is rapid and persistent.
Sci Immunol, 9(93):eadi8150, 22 Mar 2024
Cited by: 1 article | PMID: 38517953 | PMCID: PMC11152582
IgD+IgM- B Cells in Common Variable Immunodeficiency.
Pathogens, 13(2):136, 01 Feb 2024
Cited by: 0 articles | PMID: 38392874 | PMCID: PMC10891963
B cell receptor repertoire abnormalities in autoimmune disease.
Front Immunol, 15:1326823, 31 Jan 2024
Cited by: 1 article | PMID: 38361948 | PMCID: PMC10867955
Review Free full text in Europe PMC
Biochemical and biophysical characterization of natural polyreactivity in antibodies.
Cell Rep, 42(10):113190, 05 Oct 2023
Cited by: 2 articles | PMID: 37804505 | PMCID: PMC10858392
Go to all (92) article citations
Other citations
Data
Data behind the article
This data has been text mined from the article, or deposited into data resources.
BioStudies: supplemental material and supporting data
Similar Articles
To arrive at the top five similar articles we use a word-weighted algorithm to compare words from the Title and Abstract of each citation.
IgD class switching: identification of a novel recombination site in neoplastic and normal B cells.
Eur J Immunol, 25(12):3504-3508, 01 Dec 1995
Cited by: 29 articles | PMID: 8566044
Human immunoglobulin selection associated with class switch and possible tolerogenic origins for C delta class-switched B cells.
J Clin Invest, 113(8):1188-1201, 01 Apr 2004
Cited by: 62 articles | PMID: 15085198 | PMCID: PMC385404
Accumulation of self-reactive naïve and memory B cell reveals sequential defects in B cell tolerance checkpoints in Sjögren's syndrome.
PLoS One, 9(12):e114575, 23 Dec 2014
Cited by: 23 articles | PMID: 25535746 | PMCID: PMC4275206
Class switch recombination in B lymphopoiesis: a potential pathway for B cell autoimmunity.
Autoimmun Rev, 3(6):464-469, 01 Aug 2004
Cited by: 5 articles | PMID: 15351312
Review
Funding
Funders who supported this work.
NCRR NIH HHS (4)
Grant ID: P20 RR015577
Grant ID: P20RR018758-01
Grant ID: P20 RR018758
Grant ID: P20RR15577-02