Abstract
Free full text

Cyclic adenosine monophosphate is a key component of regulatory T cell–mediated suppression
Associated Data
Abstract
Naturally occurring regulatory T cells (T reg cells) are a thymus-derived subset of T cells, which are crucial for the maintenance of peripheral tolerance by controlling potentially autoreactive T cells. However, the underlying molecular mechanisms of this strictly cell contact–dependent process are still elusive. Here we show that naturally occurring T reg cells harbor high levels of cyclic adenosine monophosphate (cAMP). This second messenger is known to be a potent inhibitor of proliferation and interleukin 2 synthesis in T cells. Upon coactivation with naturally occurring T reg cells the cAMP content of responder T cells is also strongly increased. Furthermore, we demonstrate that naturally occurring T reg cells and conventional T cells communicate via cell contact–dependent gap junction formation. The suppressive activity of naturally occurring T reg cells is abolished by a cAMP antagonist as well as by a gap junction inhibitor, which blocks the cell contact–dependent transfer of cAMP to responder T cells. Accordingly, our results suggest that cAMP is crucial for naturally occurring T reg cell–mediated suppression and traverses membranes via gap junctions. Hence, naturally occurring T reg cells unexpectedly may control the immune regulatory network by a well-known mechanism based on the intercellular transport of cAMP via gap junctions.
T cells with suppressive properties were described for the first time more than 30 yr ago and experienced an overwhelming renaissance in the last 10 yr (1, 2). Although different kinds of induced regulatory T cells (T reg cells) were described, it is common sense that naturally occurring T reg cells are of capital importance for preventing autoimmunity (3). Basic research and preclinical studies revealed that they do not only play a crucial role in the maintenance of peripheral tolerance but also in the control of allergic, autoimmune, and infectious diseases and anti-tumor responses as well (3). Today it is widely accepted that naturally occurring T reg cells exert their regulatory capacity via cell contact–dependent, cytokine–independent mechanisms. For instance, the use of T cells, defective in TGF-β signaling, or the application of neutralizing antibodies to TGF-β and IL-10 had no effect on suppressor function in vitro (4). In addition, the use of TGF-β–deficient naturally occurring T reg cells in a T cell transfer colitis model or the adoptive transfer of naturally occurring T reg cells from IL-10–deficient mice in an asthma model doubtlessly revealed that these immune suppressive cytokines are not directly involved in naturally occurring T reg cell–mediated suppression in vivo (5, 6).
The inhibition of IL-2 gene expression in the responder T cells is a characteristic feature of naturally occurring T reg cell–mediated suppression (7). However, the underlying molecular mechanisms of this process still remain to be discovered (3). The first description of a physiological role of cAMP in 1957 gave rise to a brilliant concept of cAMP being a second messenger that mediates the effects of a variety of different biologically active agents (8, 9). Among miscellaneous functions, cAMP is known to be a potent inhibitor of T cell growth, differentiation, and proliferation (10). However, the mechanisms by which cAMP exerts this suppressive function are largely undetermined. The first evidence that cAMP-dependent signal transduction directly attenuates the production of IL-2 in CD4+ T cells was recently further corroborated by the finding of a novel cAMP-inducible transcriptional repressor termed inducible cAMP early repressor (ICER; references 11, 12). Collectively, a multitude of studies using cAMP-elevating agents such as prostaglandin E2, cholera toxin, and forskolin clearly demonstrated that cAMP is a potent inhibitor of IL-2 production and subsequent proliferation of CD4+ T cells.
In this study we show that naturally occurring T reg cells mediate their suppressive capacity by transferring cAMP into responder CD4+ T cells via gap junctions (GJs). This analysis reveals for the first time an unexpected role for a classical second messenger in combination with an ubiquitous system of intercellular communication in naturally occurring T reg cell–mediated suppression.
RESULTS AND DISCUSSION
Comparative analyses of naturally occurring T reg cells and conventional CD4+ T cells (CD4+ T cells) using gene array technology and quantitative RT-PCR revealed that the expression of the cAMP-cleaving enzyme phosphodiesterase 3B (PDE3B) was strongly reduced in naturally occurring T reg cells (unpublished data). This finding was in agreement with recently published data (13) and led us to suggest that naturally occurring T reg cells harbor high concentrations of cAMP that profoundly suppress the production of IL-2 in naturally occurring T reg cells, which is a characteristic feature of this T cell population (3).
Assessment of cAMP detected high concentrations of cAMP in naturally occurring T reg cells, whereas in CD4+ T cells this second messenger was scarce (Fig. 1 A, t = 0). This difference further increased after activation of both T cell types (Fig. 1 A, t = 4–16 h). In contrast, 16 h upon co-culture with naturally occurring T reg cells, CD4+ T cells showed a substantial increase of cAMP (Fig. 1 A, triangle). The usage of MHC-disparate T cell populations and the additional staining of CD4+ T cells with CFSE allowed us to exclude a contamination of the FACSorted CD4+ T cells with co-cultured naturally occurring T reg cells (Fig. S1, available at http://www.jem.org/cgi/content/full/jem.20062129/DC1). Thus, these data suggested that high concentrations of endogenous cAMP are responsible for the anergic phenotype of naturally occurring T reg cells. Furthermore, the vigorous rise of cAMP in the suppressed CD4+ T cells implied that the suppressive potency of naturally occurring T reg cells is also mediated by cAMP.
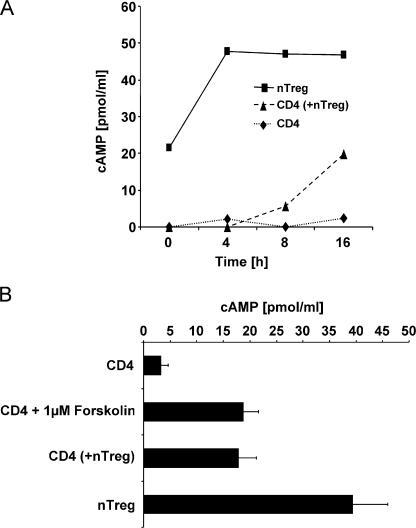
Naturally occurring T reg cell–mediated suppression is accompanied by a strong increase of cAMP in the suppressed CD4+ T cells. (A) CFSE-labeled CD4+ T cells (CD4; ♦) and naturally occurring T reg cells () were stimulated alone or in co-culture with anti-CD3 mAb and A20 cells as accessory cells for 4, 8, and 16 h. At the indicated time points, the CD4+ T cells from the co-culture were reisolated using FACS-based cell sorting (CD4 (+nTreg);
) to a purity >99%. The cytosolic cAMP concentration was assessed using a cAMP-specific ELISA. One representative result of two independent experiments is shown. (B) CD4+ T cells and naturally occurring T reg cells were activated as described in A. Alternatively, CD4+ T cells were stimulated in the presence of 1 μM forskolin. After 20 h of stimulation, cytosolic cAMP concentrations were measured. Shown are the means ± SD of three independent experiments each performed in triplicates.
Similar to the suppressive effects of naturally occurring T reg cells, dibutyryl-cAMP or the “cAMP-elevating agent” forskolin are also potent inhibitors of IL-2 expression and T cell proliferation (references 10, 14–18; Fig. S2, A–C, available at http://www.jem.org/cgi/content/full/jem.20062129/DC1). Furthermore, addition of IL-2 compensated for the antiproliferative potency of forskolin and naturally occurring T reg cells for CD4+ T cells (reference 19; Fig. S2 D). Besides the compensatory effect of exogenous IL-2, co-stimulation via CD28 was shown to abrogate naturally occurring T reg cell–mediated suppression (7). Interestingly, co-stimulation via CD28 was recently found to potentiate T cell responses by down-regulating cAMP levels via recruitment of PDE4 to lipid rafts (20). Thus, these observations suggested that naturally occurring T reg cells and cAMP trigger comparable if not identical mechanisms in suppressed CD4+ T cells. Therefore, the influence of naturally occurring T reg cells and forskolin on cAMP levels and proliferation of CD4+ T cells was further analyzed. Fig. 1 B demonstrates that forskolin (1 μM) as well as the addition of naturally occurring T reg cells (1:1) increased the concentration of cAMP in CD4+ T cells to identical levels. Moreover, a simultaneous assessment of the proliferation revealed that forskolin and co-cultured naturally occurring T reg cells exhibited the same antiproliferative potency on CD4+ T cells (Fig. S2 C).
To further clarify the function of cAMP in naturally occurring T reg cell–mediated suppression, cAMP activity was blocked in CD4+ T cells by a cAMP antagonist, termed Rp-cAMPS (21). Notably, after blockade of cAMP, IL-2 mRNA expression of CD4+ T cells was not substantially altered (unpublished data). However, upon co-culture with naturally occurring T reg cells, the strong inhibitory activity of these cells on IL-2 production of co-cultured CD4+ T cells was neutralized in a concentration-dependent manner. A considerable effect could be observed at a ratio of 1:1 (Fig. 2 A) and inhibition was almost completely abrogated when the number of naturally occurring T reg cells was halved (1:0.5, Fig. 2 B). In addition to the expression of IL-2, the suppressed proliferation of co-cultured CD4+ T cells can also be restored by Rp-cAMPS (Fig. S3, available at http://www.jem.org/cgi/content/full/jem.20062129/DC1). Hence, these findings indicate that cAMP is essentially involved in naturally occurring T reg cell–mediated suppression. Regarding the underlying molecular mechanisms, it has been suggested that the transcriptional repressor ICER attenuates IL-2 expression by binding in competition to the transcription factor CREB (cAMP response element binding protein) to the IL-2 promoter (10). Interestingly, we found that the expression of ICER in CD4+ T cells was enhanced upon co-culture with naturally occurring T reg cells (Fig. S4, calcein-high), indicating that this transcriptional repressor is involved in naturally occurring T reg cell–mediated suppression most likely via cAMP (22).
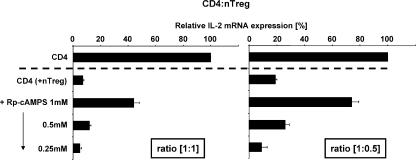
cAMP is a key component of naturally occurring T reg cell–mediated suppression. CD4+ T cells were preincubated for 30 min either with the specific cAMP antagonist Rp-cAMPS or its solvent as described in Materials and methods. Upon preincubation, cells were washed and activated separately (CD4) or in co-culture with naturally occurring T reg cells (CD4 +nTreg) at the indicated ratios. After 20 h of stimulation quantitative RT-PCR for the expression of IL-2 mRNA was performed and data were normalized according to the expression of EF1α mRNA and expressed as a percentage of IL-2 mRNA by setting the relative IL-2 mRNA expression of the CD4 single culture to 100%. One representative assay performed in triplicates ± SD is shown.
Although different surface molecules like CTLA-4 and membrane-bound TGF-β were described to be involved, no satisfying explanation for the mechanisms of naturally occurring T reg cell–mediated suppression has been put forward so far (4). Therefore, the question arose whether and how cAMP can be intercellularly transferred from naturally occurring T reg cells to other T cells. With regard to this, GJs have been shown to transport cAMP very efficiently (23). GJs are build from connexins, a well-known family of membrane proteins that could play an as yet unexpected role in naturally occurring T reg cell–mediated suppression. Hence, accumulation of cAMP in suppressed CD4+ T cells implied that it is directly transmitted from naturally occurring T reg cells to suppressed CD4+ T cells via GJ intercellular communication (GJIC). Both naturally occurring T reg cells as well as CD4+ T cells express several members of the connexin family that will allow GJIC (Table S1, available at http://www.jem.org/cgi/content/full/jem.20062129/DC1).
To pursue this model, we used the permeant dye calcein that spreads from donor to recipient cells only via GJ (24). RAG-2–deficient, CD90.2-positive OT-II mice that contain CD4+ T cells, which express a transgenic T cell receptor recognizing OVA323-339 but lacking naturally occurring T reg cells, were immunized with OVA323-339 in CFA in the left hind footpad. 6 h later, preactivated congenic calcein-loaded naturally occurring T reg cells expressing CD90.1 and the same transgenic T cell receptor (OVA323-339) were adoptively transferred. After 24 h, draining and non-draining LNs were prepared and the host CD4+ T cell population was analyzed by FACS staining. CD4+ T cells isolated from the draining LN of immunized mice that were not treated with calcein-loaded naturally occurring T reg cells served as control (Fig. 3 A, left). A substantial part of the CD4+, CD90.1-negative T cells from the draining LNs of immunized mice that received calcein-loaded naturally occurring T reg cells exhibited a strong calcein staining (Fig. 3 A, middle, green). The transferred naturally occurring T reg cells can be identified in this blot as CD90.1-positive cells with high calcein staining (Fig. 3 A, middle, black). In contrast, CD4+, CD90.1-negative T cells from the nondraining LNs prepared from the same mouse did not exhibit any calcein staining, although comparable numbers of transferred naturally occurring T reg cells could also be detected in these LNs (Fig. 3 A, right, black population in top right).
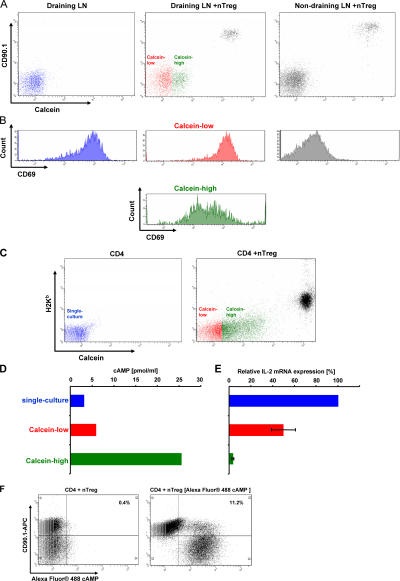
Naturally occurring T reg cells and conventional CD4+ T cells communicate via GJ in vivo and in vitro. (A) OT-II, CD90.2-positive, RAG-2–deficient mice were immunized subcutaneously with OVA323-339 in the left hind footpad. 6 h later vehicle or calcein-loaded naturally occurring T reg cells isolated from the spleens of CD90.1-positive congenic OT-II mice and preactivated for 48 h in vitro were injected i.v. 24 h after immunization, the draining and the nondraining LNs were dissected and LN cells were stained for the expression of CD4, CD69, and CD90.1. The intracellular calcein-level (A) and the expression of CD69 (B) were analyzed by gating on propidium iodide–negative and CD4+ LN cells. (C) CD4+ T cells from BALB/c mice (H2-Kd) were stimulated in vitro in the absence (single-culture; blue) or presence of calcein-loaded naturally occurring T reg cells isolated from the spleens of C57BL/6 (H2-Kb). After 20 h, cells were stained with an anti–H2-Kb mAb and GJIC was assessed by analyzing calcein transfer (red, calcein-low; green, calcein-high). (D and E) Simultaneously, CD4+ T cells from the single culture and H2-Kb-negative, calcein-high (green) or calcein-low (red) CD4+ T cells were reisolated from the co-cultures using FACS-based cell sorting and analyzed for their cytosolic cAMP concentration and the expression of IL-2 mRNA. (C–E) One representative result of three independent experiments. (F) Conventional CD90.1-positive CD4+ T cells were stimulated in the presence of CD90.2-positive naturally occurring T reg cells or CD90.2-positive naturally occurring T reg cells that had been transfected with Alexa Fluor 488 cAMP. After 20 h, cells were stained for expression of CD90.1 to discriminate CD4+ T cells and T reg cells and analyzed for the diffusion of Alexa Fluor 488 cAMP to CD90.1-positive CD4+ T cells.
Obviously, only activated CD4+ T cells are qualified to interact with naturally occurring T reg cells by GJIC, suggesting that additional activation-induced surface molecules are required to enable GJIC. According to this, FACS analyses of the early activation marker CD69 revealed that almost all CD4+ T cells from the draining LNs of the control mice were activated in the absence of naturally occurring T reg cells (Fig. 3 B, left). In mice that received naturally occurring T reg cells, the CD4+ host T cells that did not contain calcein also exhibited a high expression of CD69 (Fig. 3 B, middle). In contrast, the expression of CD69 was strongly reduced in the host CD4+ T cells, which received calcein from naturally occurring T reg cells, indicating that cell contact is also in vivo required for naturally occurring T reg cell–mediated inhibition by GJIC (Fig. 3 B, middle, green). CD4+ T cells from the nondraining LNs did not express CD69 (Fig. 3 B, right). Hence, these findings demonstrate that only the activation of CD4+ T cells that had received calcein upon contact with the transferred naturally occurring T reg cells was substantially suppressed. Therefore, these data indicate that in vivo a close cell contact and GJIC are necessary for naturally occurring T reg cell–mediated suppressive activities, whereas soluble mediators like IL-10 or TGF-β do not seem to be directly involved. This assumption is corroborated by publications showing that IL-10– or TGF-β–deficient, naturally occurring T reg cells were functionally not compromised in vitro and in vivo (4–6). Because calcein does not influence the activation of CD4+ T cells (IL-2 production and proliferation; unpublished data) these findings support our model that naturally occurring T reg cell–mediated suppression is mediated by cAMP transferred via GJIC.
To analyze this assumption in more detail, CD4+ T cells and calcein-loaded naturally occurring T reg cells were coactivated in vitro. Upon co-culture, CD4+ T cells that received high amounts of calcein from naturally occurring T reg cells (Fig. 3 C, green) and CD4+ T cells that contained no or low amounts of calcein (Fig. 3 C, red) were FACSorted and the cAMP content of both populations was compared. Fig. 3 D illustrates that calcein-high CD4+ T cells harbor high concentrations of cAMP (green bar), whereas calcein-low CD4+ T cells harbor only low concentrations of cAMP (red bar) slightly higher than CD4+ T cells that had been activated in the absence of naturally occurring T reg cells (blue bar). The assessment of IL-2 expression revealed that calcein-high CD4+ T cells that harbor high amounts of cAMP express very low levels of IL-2 (Fig. 3 E, green bar), whereas calcein-low CD4+ T cells that harbor low amounts of cAMP express comparatively high levels of IL-2 (Fig. 3 E, red bar). This oppositional behavior of cAMP content and IL-2 expression clearly indicates that an elevated level of cAMP leads to the inhibition of IL-2 expression. CD4+ T cells activated in the absence of naturally occurring T reg cells expressed even more IL-2 (Fig. 3 E, blue bar) compared with calcein-low CD4+ T cells from the co-culture. This finding can be explained by a floating transition between the calcein-high and -low fraction of co-cultured CD4+ T cells resulting in a reduced IL-2 expression corresponding to a slightly increased cAMP content of the calcein-low CD4+ T cell population. To rule out an additional endogenous production of cAMP in suppressed CD4+ T cells by adenylate cyclases (ADCYs), experiments using ADCY inhibitors were conducted. Unfortunately, all commercially available ADCY inhibitors were toxic for freshly isolated mouse T cells preventing us from using such reagents to solve this question. Therefore, a contributing activation of endogenous ADCYs in suppressed CD4+ T cells cannot be completely excluded. However, because it was published that GJs are permeable for Alexa dyes (25), we used Alexa Fluor 488 cAMP to demonstrate a direct transfer of cAMP via GJIC (Fig. 3 F). The transfer rate was comparatively low, obviously because Alexa Fluor 488 cAMP is considerably larger than cAMP, which strongly implies that cAMP alone can much better trespass the cell membrane. Experiments with calcein-stained and nonstained CD4+ T cells also revealed a GJIC among CD4+ T cells (unpublished data). Because CD4+ T cells contain only minimal concentrations of cAMP the observed GJIC did not lead to any suppression. In addition, it should be noted that separation of naturally occurring T reg cells and CD4+ T cells by transwell cultures not only abrogated naturally occurring T reg cell–mediated suppression but also completely prevented the transfer of calcein (unpublished data). This underscores the cell contact– dependent mechanism of calcein and cAMP transfer via GJ between both cell types.
To further corroborate the participation of GJ in cAMP transport, GJIC should be disrupted. PCR analyses revealed that naturally occurring T reg cells and CD4+ T cells expressed connexin 31.1 (Cx31.1), Cx32, Cx43, Cx45, and Cx46 (Table S1). These connexins can form homo- or heteromeric hexameric hemichannels (connexons), which align in neighboring cells and build up homo- and heterotypic GJ during cell contact (26). This multitude of different GJ required a general inhibitor of GJIC. Unfortunately, substances like 18β-glycyrrhetinic acid were highly toxic for T cells (unpublished data) or were shown to have other disadvantages like nonspecific side effects and a large size, which can limit their access, especially to the intercellular region of the GJ (27). In contrast, synthetic peptides that mimic connexins have been described to interfere with GJIC. GAP27 was especially described to be a potential GJ inhibitory peptide (27). This peptide is identical in sequence to a portion of an extracellular loop of Cx43 and interferes with both GJ formation and stability. The addition of GAP27 to co-cultures reduced the transfer of calcein to ~50%, whereas a scrambled control peptide (SC-GAP27) did not have any effect (Fig. 4 A). Simultaneously, the suppression of IL-2 mRNA expression in CD4+ T cells upon contact with naturally occurring T reg cells was substantially abrogated by the addition of GAP27, whereas SC-GAP27 had no effect (Fig. 4 B). It was not possible to completely block the transfer of calcein, mostly because GAP27 could not inhibit all potential GJ variants with the same efficiency (27).
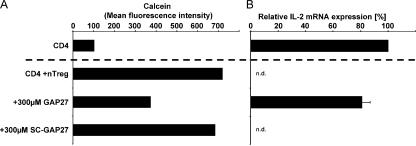
Blocking GJIC simultaneously reduces the influx of calcein and the inhibition of IL-2 expression in CD4+ T cells upon contact with naturally occurring T reg cells. (A) CD4+ T cells from BALB/c mice (H2-Kd) were activated separately (CD4) or in co-culture with calcein-loaded naturally occurring T reg cells (CD4 +nTreg) from C57BL/6 mice (H2-Kb). As depicted, the cells were stimulated in the presence or absence of the GJ mimetic peptide GAP27 or a scrambled control peptide SC-GAP27, consisting of the same amino acids in a different order. 20 h upon stimulation, the calcein transfer was assessed by gating on CD4+ T cells (H2-Kb negative, propidium iodide negative). (B) In parallel, the CD4+ T cells were reisolated from the co-culture using FACS-based cell sorting to a purity >99%. Expression of IL-2 mRNA was assessed in triplicates by quantitative RT-PCR and data were normalized according to the expression of EF1α mRNA. Shown are the means ± SD of three independent experiments. N.d., not detectable.
In conclusion, these data indicate that naturally occurring T reg cells mediate their suppressive capacity by transferring cAMP into responder cells via GJIC. Our analyses uncovered a new and exciting function for a classical second messenger in combination with an ubiquitous system of intercellular communication. The in vivo relevance of these findings can be deduced from preclinical studies demonstrating that cAMP-elevating agents like vasoactive intestinal peptide or PDE inhibitors like pentoxifylline can alleviate autoimmune diseases via the increase of cAMP in self-reactive effector T cells (28, 29). Thus, the mechanism of action of such agents could be at least partially explained by their influence on naturally occurring T reg cell–mediated suppression.
MATERIALS AND METHODS
Mice.
Mice of strains BALB/c and C57BL/6 were obtained from Charles River Laboratories and bred in our own animal facility. OT-II mice, transgenic for the OVA323–339-specific TCR-αβ (30), and RAG2-deficient mice (RAG−/−) were obtained from Charles River Laboratories and bred in our own animal facility. OT-II RAG−/− mice were obtained by intercrossing these lines accordingly. Males and females were used at 6–8 wk of age. All mice used for this study were bred and housed in a specific pathogen-free colony at the animal facility of Johannes Gutenberg University using institutionally approved protocols (permission was obtained from the Landesuntersuchungsamt Koblenz).
Cytokines, antibodies, and reagents.
Hybridoma cells producing anti-CD4 mAb GK1.5 were obtained from American Type Culture Collection. PE-labeled rat anti-CD25 (7D4), PE-labeled anti-H2-Kb mAb (AF6-88.5), PE-Cy7–labeled hamster anti-CD69 (H1.2F3), and all corresponding isotypes were purchased from BD Biosciences. IL-2–specific ELISA was performed using anti–mIL-2 (JES6-1A12) and biotinylated anti–mIL-2 (JES6-5H4; both from BD Biosciences). In addition, the following mAbs were used: anti-CD3 mAb 145-2C11 and anti-CD28 mAb 37.51. If required, mAbs were affinity purified using protein G–Sepharose (GE Healthcare) and coupled with FITC or biotin. Mouse recombinant IL-2 was affinity purified using an anti–IL-2 mAb bound to sepharose. Mitomycin C and forskolin were purchased from Sigma-Aldrich. 5-(and-6)-carboxyfluorescein diacetate, succinimidyl ester (5(6)-CFDASE; CFSE) and calcein AM were purchased from Invitrogen. CFDASE was dissolved in DMSO and calcein AM was dissolved in 100% ethanol. The connexin mimetic peptide GAP27 (SRPTEKTIFII) and a control-peptide consistent with the same amino acids but with a randomly shuffled sequence were synthesized and HPLC purified by Peptide Specialty Laboratories to a purity >98%. Peptides were dissolved in DMSO and added to cell cultures. Adenosine 3′,5′-cyclic monophosphorothioate, Rp-isomer, triethylammonium salt (Rp-cAMPS) was purchased from Calbiochem and dissolved in aqua ad injectabilia. Alexa Fluor 488 cAMP was purchased from Invitrogen.
Cytokine assays.
Cytokines were assayed by specific two-site ELISA with reference standard curves using known amounts of the respective cytokine. For the detection of cytokines, we used the following combinations of mAbs: IL-2 JES6-1A12 and biotinylated JES6-5H4.
Preparation of T cell populations.
Conventional CD4+CD25− T cells (CD4+ T cells; GK1.5-FITC) and naturally occurring CD4+CD25+ T reg cells (7D4-PE) were isolated as described previously (31). Preactivation of naturally occurring T reg cells was performed using a combination of plate-bound anti-CD3 mAb (145-2C11; 3 μg/ml) and anti-CD28 mAb (37.51; 10 μg/ml). After 48 h the cells were harvested and used as preactivated naturally occurring T reg cells.
FACS-based cell sort.
CD4+ T cells were stained with CFDASE as described previously (31). After co-culture, CFSE-labeled CD4+ T cells were washed in PBS containing 4% FCS and isolated using a cell sorter (FACSvantage SE and CellQuest Pro; BD Biosciences), with exclusion of dead cells by propidium iodide incorporation. The purity of the sorted cells was typically >99% (see Fig. S2).
T cell stimulation and proliferation assays.
The T cell stimulation and proliferation assays were performed as described previously (31).
Polyclonal T cell activation.
CD4+ T cells (106/ml) from BALB/c mice were activated in the presence of mitomycin C–treated (60 μg/ml/107 cells, 30 min) A20 B tumor cells (105/ml) and soluble anti-CD3 mAb (3 μg/ml) in the absence or presence of naturally occurring T reg cells (106/ml) from BALB/c mice or C57BL/6 mice. To elevate the intracellular cAMP concentration, forskolin was added as indicated.
Transfection of naturally occurring T reg cells.
Naturally occurring T reg cells (2 × 106 naturally occurring T reg cells/cuvette) were transfected with 240 μM Alexa Fluor 488 cAMP according to the instructions of the manufacturer (AMAXA). Cells were allowed to recover in medium for 2 h. 5 × 105 CD90.2 naturally occurring T reg cells were co-cultured with 5 × 105 CD90.1 CD4+ T cells in a 96-well round-bottom plate and stimulated for 20 h as described previously (31). Cells were washed with PBS and immunostaining was performed using anti–CD90.1-APC antibody and analyzed on a FACS Canto (BD Biosciences).
Blocking GJIC with the connexin mimetic peptide GAP27.
CD4+ T cells from BALB/c mice were stained with CFSE, activated in single or co-culture with naturally occurring T reg cells from C57BL/6 mice in the presence or absence of 300 μM GAP27 or SC-GAP27, respectively. 20 h upon stimulation, the CD4+ T cells were reisolated using FACS-based cell sorting and lysed in Trizol (Invitrogen) to isolate total RNA.
Rp-cAMPS.
To evaluate the role of cAMP in naturally occurring T reg cell–mediated suppression, CD4+ T cells were preincubated with varying amounts of Rp-cAMPS for 30 min, washed three times, and stimulated solely or in co-culture with naturally occurring T reg cells (1:1 and 1:0.5). After 20 h of stimulation total RNA was prepared and quantitative RT-PCR for the detection of IL-2 mRNA was performed.
cAMP ELISA.
To assess cytosolic cAMP concentrations, T cells were washed three times in ice-cold PBS, lysed in 0.1 N HCl (107/ml) and a cAMP-specific ELISA (Correlate EIA Direct Cyclic AMP Enzyme Immunoassay kit; Assay Design) was performed.
Calcein AM staining and GJIC detection in vitro.
Naturally occurring T reg cells (107/ml) were incubated with 1 μM calcein AM in IMDM at 37°C in 5% CO2 for 30 min. The cells were then washed three times with IMDM and stimulated as described above in a 48-well plate in co-culture (2 × 106 naturally occurring T reg cells + 2 × 106 conventional CD4+ T cells; ratio 1:1) for 20 h. Calcein transfer was detected on a FACS Canto.
Assessment of GJIC in vivo.
OT-II, RAG-2–deficient, CD90.2-positive mice were immunized by injecting 50 μg OVA323-339 in CFA subcutaneously into the left hind footpad. 6 h later, 8 × 106 polyclonally preactivated, calcein-loaded, naturally occurring T reg cells from OT-II, CD90.1-positive mice were injected i.v. into the OVA323-339-immunized OT-II, RAG-2–deficient, CD90.2-positive mice. 24 h upon immunization the draining and the nondraining popliteal LNs were removed and LN cells were stained for the expression of CD4, CD90.1, and CD69. By gating on propidium iodide–negative CD4+ LN cells calcein transfer, the expression of CD90.1 (naturally occurring T reg cells) and the early activation marker CD69 were analyzed using a FACS Canto.
mRNA detection.
RNA was isolated using Trizol and cDNA was synthesized with RevertAid M-MuLV reverse transcriptase following the recommendations of the supplier (MBI Fermentas). Quantitative RT-PCRs were performed using the following oligonucleotides:
IL-2 forward, CCT GAG CAG GAT GGA GAA TTA CAG G; IL-2 reverse, GCA CTC AAA TGT GTT GTC AGA GCC C; elongation factor-1 α (EF1α) forward, GAT TAC AGG GAC ATC TCA GGC TG; EF1α reverse, TAT CTC TTC TGG CTG TAG GGT GG; Cx26 forward, CAA CGC TGC ACG CTC CTC CC; Cx26 reverse, GCC AGG CTG GAG CGT GTT GC; Cx30 forward, TGC AGT GAC TCT TGA GCT GG; Cx30 reverse, CAT GTC TGT AGT AGG CCA CG; Cx30.3 forward, GAC CCA AGA AGG AAG GAA AG; Cx30.3 reverse, CTC ATC ATA GCA GAC CTT GG; Cx31 forward, TCG TTC CTC CTC TGC TGT GG; Cx31 reverse, TCG GCA GCC ACC ACA TAC AC; Cx31.1 forward, CCA AGC CCT CCG AGA AAA AC; Cx31.1 reverse, TCC GAG CCC AGA AAG ATG AG; Cx32 forward, GAA GAG GTA AAG AGA CAC AAG G; Cx32 reverse, ACG TTG AGG ATA ATG CAG ATG; Cx37 forward, AGA GAG GCC CTG GAA ACA TG; Cx37 reverse, CAT AGC AGA CGT TGG TGC AG; Cx40 forward, AGA GCC TGA AGA AGC CAA C; Cx40 reverse, AAC AGG ACA GTG AGC CAG AC; Cx43 forward, TAC CAC GCC ACC ACT GGC; Cx43 reverse, AAT CTC CAG GTC ATC AGG; Cx45 forward, AAA GAG CAG AGC CAA CCA AA; Cx45 reverse, GTC CCA AAC CCT AAG TGA AGC; Cx46 forward, GGA AAG GCC ACA GGG TTT CCT GG; Cx46 reverse, GGG TCC AGG AGG ACC AAC GG; Cx50 forward, AAG ACA GCA CCA GTT TCT CC; Cx50 reverse, GAC AGT GGA GTG CTC ATT CA; ICER forward, ATG GCT GTA ACT GGA GAT GAA; ICER reverse, AGC AAA TGT CTT TCA AAC TTT CAA.
Oligonucleotides were chosen to span at least one intron at the level of genomic DNA. Quantitative RT-PCR analyses to quantify the expression of IL-2, the indicated connexins, and EF1α mRNAs were performed in triplicates on an iCycler (Bio-Rad Laboratories) using the IQ SYBR Green Supermix (Bio-Rad Laboratories). After normalization of the data according to the expression of EF1α mRNA, the relative expression levels of IL-2 and connexin mRNAs were calculated.
Online supplemental material.
Fig. S1 shows the purity of FACSorted CD4+ T cells upon co-culture with naturally occurring T reg cells. Fig. S2 shows that naturally occurring T reg cells and the cAMP-elevating agent forskolin comparatively affect IL-2 gene expression and IL-2 production of CD4+ T cells. Fig. S3 shows the abrogation of naturally occurring T reg cell–mediated suppression of proliferation by the addition of the cAMP antagonist Rp-cAMPS. Fig. S4 shows the elevation of ICER mRNA expression in CD4+ T cells upon co-culture with naturally occurring T reg cells. Table S1 summarizes the connexin genes expressed by CD4+ T cells and naturally occurring T reg cells. The online supplemental material is available at http://www.jem.org/cgi/content/full/jem.20062129/DC1.
Acknowledgments
We would like to thank S. Gerecht, A. Hobel, and I. Volkmer for technical help, Dr. Christoph Becker for generating TGF-β–induced T reg cells, and Drs. Cathy Srokowski, Karen Lingnau, and Erwin Ruede for critically reading the manuscript.
This work was supported by SFB 548: A6, A8, and A10 grants and the Immunology Cluster of Excellence “Immunointervention” of the Johannes Gutenberg University.
The authors have no conflicting financial interests.
References
Articles from The Journal of Experimental Medicine are provided here courtesy of The Rockefeller University Press
Full text links
Read article at publisher's site: https://doi.org/10.1084/jem.20062129
Read article for free, from open access legal sources, via Unpaywall:
https://rupress.org/jem/article-pdf/204/6/1303/1130061/jem_20062129.pdf
Citations & impact
Impact metrics
Citations of article over time
Alternative metrics

Discover the attention surrounding your research
https://www.altmetric.com/details/102019558
Article citations
A Novel Subset of Regulatory T Cells Induced by B Cells Alleviate the Severity of Immunological Diseases.
Clin Rev Allergy Immunol, 28 Oct 2024
Cited by: 0 articles | PMID: 39465485
Review
Differential proteins from EVs identification based on tandem mass tags analysis and effect of Treg-derived EVs on T-lymphocytes in COPD patients.
Respir Res, 25(1):349, 28 Sep 2024
Cited by: 0 articles | PMID: 39342213 | PMCID: PMC11439212
Influencing factors and solution strategies of chimeric antigen receptor T-cell therapy (CAR-T) cell immunotherapy.
Oncol Res, 32(9):1479-1516, 23 Aug 2024
Cited by: 0 articles | PMID: 39220130 | PMCID: PMC11361912
Review Free full text in Europe PMC
Application of PARP inhibitors combined with immune checkpoint inhibitors in ovarian cancer.
J Transl Med, 22(1):778, 21 Aug 2024
Cited by: 0 articles | PMID: 39169400 | PMCID: PMC11337781
Review Free full text in Europe PMC
A liver immune rheostat regulates CD8 T cell immunity in chronic HBV infection.
Nature, 631(8022):867-875, 10 Jul 2024
Cited by: 3 articles | PMID: 38987588 | PMCID: PMC11269190
Go to all (367) article citations
Data
Data behind the article
This data has been text mined from the article, or deposited into data resources.
BioStudies: supplemental material and supporting data
Similar Articles
To arrive at the top five similar articles we use a word-weighted algorithm to compare words from the Title and Abstract of each citation.
Inhibition of cAMP degradation improves regulatory T cell-mediated suppression.
J Immunol, 182(7):4017-4024, 01 Apr 2009
Cited by: 63 articles | PMID: 19299699
Notch3 and pTalpha/pre-TCR sustain the in vivo function of naturally occurring regulatory T cells.
Int Immunol, 21(6):727-743, 21 May 2009
Cited by: 20 articles | PMID: 19461123
Functional waning of naturally occurring CD4+ regulatory T-cells contributes to the onset of autoimmune diabetes.
Diabetes, 57(1):113-123, 10 Oct 2007
Cited by: 108 articles | PMID: 17928397
Cyclic AMP underpins suppression by regulatory T cells.
Eur J Immunol, 42(6):1375-1384, 01 Jun 2012
Cited by: 53 articles | PMID: 22678893
Review