Abstract
Free full text

From the Cover
IKK1 and IKK2 cooperate to maintain bile duct integrity in the liver
Abstract
Inflammatory destruction of intrahepatic bile ducts is a common cause of vanishing bile duct syndrome and cholestasis, often progressing to biliary cirrhosis and liver failure. However, the molecular mechanisms underlying the pathogenesis of inflammatory biliary disease are poorly understood. Here, we show that the two IκB kinases, IKK1/IKKα and IKK2/IKKβ, display distinct collaborative and specific functions that are essential to protect the liver from cytokine toxicity and bile duct disease. Combined conditional ablation of IKK1 and IKK2, but not of each kinase alone, sensitized the liver to in vivo LPS challenge, uncovering a redundant function of the two IκB kinases in mediating canonical NF-κB signaling in hepatocytes and protecting the liver from TNF-induced failure. Unexpectedly, mice with combined ablation of IKK1 and IKK2 or IKK1 and NEMO spontaneously developed severe jaundice and fatal cholangitis characterized by inflammatory destruction of small portal bile ducts. This bile duct disease was caused by the combined impairment of canonical NF-κB signaling together with inhibition of IKK1-specific functions affecting the bile–blood barrier. These results reveal a novel function of the two IκB kinases in cooperatively regulating liver immune homeostasis and bile duct integrity and suggest that IKK signaling may be implicated in human biliary diseases.
Loss of intrahepatic bile ducts is a common cause of cholestasis, a serious condition that often progresses to biliary cirrhosis and liver failure (1–3). A number of pathogenic processes have been proposed to be involved in the pathogenesis of bile duct loss, including immunological, ischemic, and infectious etiologies (1–3). In particular, increased apoptosis of biliary epithelial cells in response to death receptor stimulation, immunological assaults, oxidative stress, toxins, and pathogens is believed to contribute to the development of bile duct disease (1–3). Impairment of bile duct regeneration after biliary epithelial cell apoptosis may be critical for establishing an irreversible loss of bile ducts and causing chronic disease (1–3).
The IKK/NF-κB signaling pathway regulates immune and inflammatory responses and plays a critical role in protecting cells from cytokine-induced death and oxidative damage (4–6). Thus, IKK/NF-κB signaling could be implicated in bile duct disease. However, despite numerous studies in vivo and in vitro, a role of NF-κB in the pathogenesis of biliary disease has not been recognized to date. Activation of NF-κB depends on the IκB kinase complex, consisting of two catalytic subunits, IKK1/IKKα and IKK2/IKKβ, and a regulatory subunit called NEMO/IKKγ (4, 5, 7). The two IκB kinases are structurally similar but functionally distinct. IKK2 has been suggested to mediate proinflammatory signal-induced “canonical” NF-κB activation, a function that also depends on NEMO/IKKγ (4–6). IKK1 is believed not to play a role in canonical NF-κB signaling but mediates NF-κB activation via the “alternative” pathway by controlling p100 processing and also displays nuclear functions independent of NF-κB (4–6).
Previous studies showed that NEMO-dependent NF-κB signaling in hepatocytes is essential to protect the liver from LPS/TNF-induced toxicity (8). Moreover, IKK/NF-κB signaling was reported to regulate liver cancer development (9, 10). Mice lacking NEMO specifically in liver parenchymal cells develop spontaneously chronic hepatitis characterized by increased apoptosis and elevated compensatory proliferation of hepatocytes, liver inflammation, and steatosis, ultimately resulting in the development of liver cancer (8). FADD-dependent death receptor signaling and oxidative stress were shown to be critical for the development of hepatitis in the NEMOLPC-KO mice. Here, we report that mice lacking both IKK1 and IKK2 specifically in liver parenchymal cells develop spontaneously a biliary disease characterized by inflammatory loss of intrahepatic bile ducts, resulting in cholestasis and poor survival. The development of biliary disease in this model depends on the inhibition of canonical NF-κB signaling in combination with the impairment of IKK1-specific functions regulating the formation of tight junctions in biliary epithelial cells. Thus, the two IκB kinases cooperate to maintain bile duct integrity in the liver.
Results and Discussion
IKK1 and IKK2 Share a Redundant Function in Mediating Canonical NF-κB Activation in Hepatocytes and Protecting the Liver from LPS-Induced Toxicity.
To investigate in vivo the function of IKK subunits in the liver, we generated mice lacking IKK1 (IKK1LPC-KO), IKK2 (IKK2LPC-KO), NEMO (NEMOLPC-KO), or both IKK1 and IKK2 (IKK1/2LPC-KO) in liver parenchymal cells by crossing mice carrying respective loxP-flanked alleles (11–13) with Alfp-cre transgenic mice, which mediate efficient Cre recombination in hepatocytes and intrahepatic biliary epithelial cells (14, 15). IKK1LPC-KO, IKK2LPC-KO, NEMOLPC-KO, and IKK1/2LPC-KO mice were born at the expected Mendelian ratio and showed efficient ablation of the respective proteins in the liver (Fig. 1a). We reported previously that NEMOLPC-KO mice suffered liver failure in response to LPS/TNF stimulation, demonstrating that NEMO-dependent NF-κB activation is essential to protect the liver from TNF-induced toxicity (8). Surprisingly, IKK2LPC-KO mice were resistant to similar LPS/TNF challenge (8), suggesting that an IKK2-independent mechanism mediates NF-κB activation and protects the liver from cytokine-induced toxicity. Although IKK1 is generally considered to be dispensable for proinflammatory signal-induced NF-κB activation (4, 5), we hypothesized that IKK1-mediated signaling protects hepatocytes lacking IKK2 from LPS/TNF challenge. To test the function of IKK1 in LPS-induced liver toxicity in vivo, we challenged IKK1LPC-KO and IKK1/2LPC-KO mice with LPS. IKK1LPC-KO mice did not show liver failure and substantial hepatocyte apoptosis upon LPS administration (Fig. 1 b–d). In contrast, mice lacking both IKK1 and IKK2 in liver parenchymal cells were sensitive to LPS challenge showing liver failure and increased hepatocyte apoptosis similarly to NEMOLPC-KO mice (Fig. 1 b–e). Furthermore, mice lacking p65/RelA specifically in liver parenchymal cells (p65LPC-KO) were also susceptible to LPS-induced liver failure, demonstrating that p65-dependent “canonical” NF-κB activity is essential to protect hepatocytes from TNF-induced apoptosis [supporting information (SI) Fig. S1]. Taken together, these findings demonstrate that the two IκB kinases share a redundant function in protecting the liver from LPS/TNF challenge and suggest that IKK1 can mediate canonical NF-κB signaling in hepatocytes in the absence of IKK2.
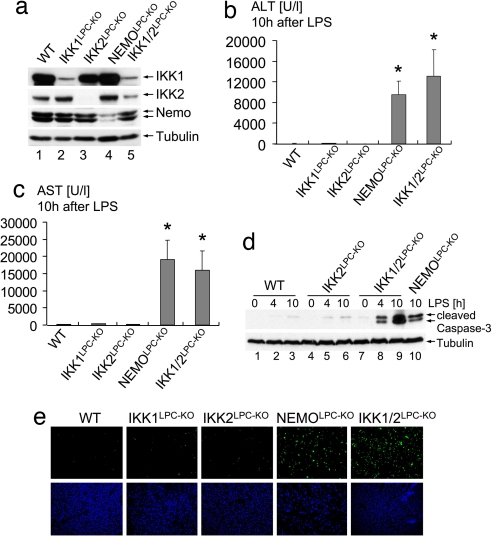
Redundant function of IKK1 and IKK2 in protecting the liver from LPS-induced toxicity. (a) Immunoblot analysis of IKK subunit expression on whole-liver protein lysates from mice with the indicated genotypes. Tubulin serves as loading control. (b and c) Liver damage 10 h after LPS administration assessed by analysis of serum ALT (b) and AST (c) levels. Error bars denote SEM (n = 3). *, P < 0.05. (d) Immunoblot analysis of caspase 3 cleavage on liver lysates prepared at the indicated time points after LPS stimulation. Tubulin serves as loading control. (e) TUNEL staining (green) on liver sections shows massive hepatocyte apoptosis in NEMOLPC-KO and IKK1/2LPC-KO mice 10 h after LPS stimulation. DAPI stains nuclei (blue). (Original magnification: ×200.)
To assess the function of IKK1 and IKK2 in inducing canonical NF-κB signaling in liver parenchymal cells, we analyzed TNF-induced NF-κB activation in primary hepatocytes lacking different IKK subunits. Electro-mobility-shift-assay (EMSA) analysis showed that IKK1- or IKK2-deficient hepatocytes displayed reduced NF-κB nuclear DNA binding activity upon TNF stimulation compared with WT cells (Fig. 2a). Immunoblot and immunohistochemical analyses confirmed that TNF-induced p65 nuclear translocation was impaired in hepatocytes lacking either IKK1 or IKK2 (Fig. 2b and Fig. S2a). Moreover, TNF-induced transcription of iNOS and A20, two classical NF-κB target genes, was decreased in IKK1- or IKK2-deficient hepatocytes compared with control cells but not completely abolished as in NEMO-deficient hepatocytes (Fig. S2 b and c). However, TNF-induced IκBα kinase activity was only mildly reduced in IKK1-deficient hepatocytes, whereas it was noticeably impaired in IKK2-deficient cells (Fig. 2c). Thus, lack of IKK1 in primary hepatocytes leads to a moderate inhibition of NF-κB activation albeit nearly intact IκBα degradation, suggesting that IKK1 controls NF-κB nuclear translocation and gene transactivation by a mechanism that seems to be independent of IκBα phosphorylation and degradation. In addition, WT and IKK2-deficient hepatocytes showed constitutive processing of p100 to p52, which was abrogated in IKK1-deficient hepatocytes (Fig. 2d). TNF-induced NF-κB DNA binding was abolished in primary hepatocytes lacking both IκB kinases (Fig. 2e), demonstrating that IKK1 and IKK2 show some degree of functional redundancy in mediating canonical NF-κB signaling in hepatocytes. These results are consistent with our in vivo experiments, which showed that LPS injection caused hepatocyte death and liver failure in IKK1/2LPC-KO or NEMOLPC-KO mice, whereas IKK1LPC-KO or IKK2LPC-KO mice were not sensitive to similar challenge. Therefore, the capacity of hepatocytes to activate NF-κB at levels above a certain threshold correlates with protection from LPS/TNF-induced liver damage. The role of NF-κB in protecting hepatocytes from death induced by circulating soluble TNF has been debated based on earlier findings showing that hepatocyte-specific knockout of IKK2 did not sensitize the liver to LPS/TNF challenge (16). Our results provide genetic evidence arguing for a different interpretation of these findings; namely, that IKK1 can compensate for the absence of IKK2 to induce NF-κB at levels that are adequate to protect the liver from LPS/TNF-mediated cytotoxicity. Our experiments demonstrating the important role of IKK1 in TNF-induced NF-κB activation in hepatocytes challenge the concept that IKK1 is dispensable for canonical NF-κB signaling (4, 5). The function of IKK1 in the classical NF-κB pathway does not seem to be restricted to hepatocytes. Earlier studies showed that IL-1- and TNF-induced NF-κB activation is impaired but not completely abolished in IKK2-deficient mouse embryonic fibroblasts (MEFs) (13, 17, 18). In contrast, MEFs lacking both IκB kinases show complete inhibition of IL-1- and TNF-induced NF-κB activation (19) similarly to NEMO knockout cells (13). Collectively, our results demonstrate that IKK1 contributes to canonical NF-κB signaling in hepatocytes and that NF-κB activation is essential to protect the liver from LPS/TNF cytotoxicity.
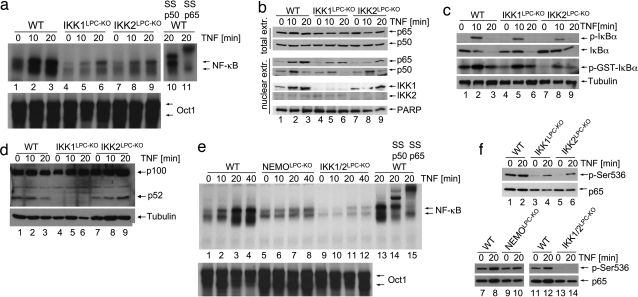
Redundant function of IKK1 and IKK2 in TNF-induced canonical NF-κB signaling. (a) Primary hepatocytes from mice with indicated genotypes were stimulated with TNF. EMSA was performed on nuclear extracts by using an NF-κB probe. In lanes 10 and 11, antibodies for p50 or p65 were added for supershift analysis. Equal loading was assessed by EMSA, using an Oct1 probe. (b) Immunoblot analysis on total (top two panels) and nuclear protein extracts (bottom five panels) from primary hepatocytes upon TNF stimulation. Anti-PARP immunoblot was used as loading control. (c) Immunoblot analysis of primary hepatocyte extracts with antibodies against phosphorylated IκBα (p-IκBα) or total IκBα (top two panels). IKK complexes were immunoprecipitated with anti-NEMO antibody, and kinase activity was measured by using recombinant GST-IκBα (1–54) as a substrate (third panel from top). Equal input into the immunoprecipitation reaction was verified by tubulin immunoblot of lysates (lowest panel). (d) Immunoblot analysis of TNF-stimulated primary hepatocyte extracts with an antibody that detects p100 and processed p52. Tubulin is used as loading control. (e) Nuclear extracts from TNF-stimulated primary hepatocytes were analyzed by EMSA with an NF-κB consensus probe. In lanes 14 and 15, antibodies for p50 or p65 were added for supershift analysis. Oct1 probe was used as loading control. (f) Immunoblot analysis of protein extracts from TNF-stimulated primary hepatocytes with antibodies against phosphorylated (Ser-536) or total p65.
Phosphorylation at serine 536 has been suggested to be essential for the full induction of p65 transcriptional transactivation potency (20). Constitutive p65 phosphorylation was observed in WT hepatocytes, which did not increase considerably upon TNF stimulation (Fig. 2f). p65 phosphorylation was impaired in resting hepatocytes lacking IKK1 or IKK2 but could be induced upon TNF stimulation. Both basal and TNF-inducible p65 phosphorylation were abolished in hepatocytes lacking both IKK1 and IKK2 (Fig. 2f), suggesting that both IκB kinases are capable of mediating phosphorylation of p65 at serine 536, although at present it remains unclear whether they directly act on p65 or whether they facilitate its phosphorylation by other kinases. p65 phosphorylation was not substantially affected in NEMO-deficient hepatocytes, indicating that it occurs independently from phosphorylation and degradation of IκBα.
Mice Lacking both IKK1 and IKK2 in Liver Parenchymal Cells Develop a Spontaneous Destructive Bile Duct Disease.
Surprisingly, IKK1/2LPC-KO mice exhibited severe growth retardation and wasting compared with WT littermates or mice lacking single IKK subunits in liver parenchymal cells (Fig. 3a). This phenotype was already apparent at weaning age and persisted to adulthood, resulting in poor survival with most IKK1/2LPC-KO mice dying within 7 months after birth. Similarly to NEMOLPC-KO mice (8), IKK1/2LPC-KO mice showed elevated levels of serum ALT (Fig. 3b), indicating underlying spontaneous liver damage. Unexpectedly, IKK1/2LPC-KO mice developed severe jaundice due to hyperbilirubinemia as shown by dramatically increased levels of bilirubin in the serum (Fig. 3c). Neither NEMOLPC-KO nor single IKK1LPC-KO and IKK2LPC-KO mice showed elevated levels of bilirubin in the serum (Fig. 3c). Further analysis showed that the bilirubin found in the serum of IKK1/2LPC-KO mice consisted mainly of direct, conjugated bilirubin (Fig. 3d) and that serum alcalic phosphatase was also strongly elevated (Fig. 3e). These findings suggested that a posthepatic, biliary system-related defect could be the reason for jaundice in IKK1/2LPC-KO mice. Subsequent histological analysis revealed a strong reduction of the number of intact small portal bile ducts together with signs of severe chronic cholestasis in the liver of IKK1/2LPC-KO mice (Fig. 3 f–j), whereas the larger, hilar bile ducts did not show histological alterations (Fig. 3 k and l). The remaining portal bile ducts in IKK1/2LPC-KO mice showed a damaged, flattened epithelium surrounded by inflammatory foci with infiltrates containing lymphocytes and ceroid macrophages (Fig. 3 m and n). To investigate whether the small bile duct defects are caused by a developmental phenotype, we examined livers from newborn mice. IKK1/2LPC-KO mice showed normal development of small bile ducts at birth (Fig. 3 o and p), suggesting that the observed biliary system phenotype is not caused by a developmental defect but is rather the result of a destructive bile duct disease developing postnatally.
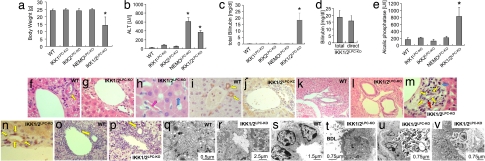
Mice with combined ablation of IKK1 and IKK2 in liver parenchymal cells develop spontaneous jaundice, severe cholestasis, and inflammatory destruction of small portal bile ducts. (a) Body weight analysis of 8-week-old male mice. Error bars denote SEM (n = 5). *, P < 0.05. (b–e) Analysis of serum levels of alanine aminotransferase (ALT) (b), total bilirubin (c), direct (conjugated) bilirubin (d), and alcalic phosphatase (e). Error bars denote SEM (n = 4). *, P < 0.05. (f–p) Representative liver sections stained with H&E (f–h, k–m, o, and p) or with an anti-cytokeratin 19 antibody (i, j, and n) that stains biliary epithelial cells. Portal bile ducts (indicated by yellow arrows) are present in control mice (f and i) but cannot be identified in most portal tracts in IKK1/2LPC-KO mice (g and j). Periportal hepatocytes in IKK1/2LPC-KO mice display increased positive anti-cytokeratin 19 staining as typical sign of cholestasis (j and n). (h) Tubular, “rosette”-like arrangement of hepatocytes around a dilated bile canaliculus (purple arrow) as typical histological feature of severe cholestasis (cholestatic liver cell rosettes). The blue arrow indicates a macrophage with yellow appearance (ceroid macrophage) as sign of phagocytosis of bilirubin metabolites. (k and l) Normal histological appearance of larger bile ducts in control (k) and IKK1/2LPC-KO (l) mice. (m and n) In a small number of portal tracts, remnants of small bile ducts with flattened, damaged epithelium can be identified (yellow arrows) that are often surrounded by an infiltrate containing lymphocytes (red arrow) and ceroid macrophages (blue arrow). (o and p) Representative H&E-stained liver sections from 2-day-old WT control mice (o) or IKK1/2LPC-KO mice (p) reveal normal formation of portal bile ducts at this age (yellow arrows). The portal tracts and sinusoids show extramedullary hematopoiesis, as normally seen in this stage of liver development. (Original magnification: h and n, ×400; f, g, i–m, o, and p, ×200.) (q–v) Electron microscopical analysis on livers from 8-week-old WT and IKK1/2LPC-KO mice. (q and r) Tubular arrangements of hepatocytes surrounding strongly dilated irregular lumen with disappearance of canalicular microvilli and thickened pericanalicular ectoplasma in IKK1/2LPC-KO mice (r) compared with normal canaliculus in control mice (q). Intercellular bile leakage in portal bile ducts in IKK1/2LPC-KO mice (t) showing flattened damaged epithelial cells surrounded by a thickened often doubled basement membrane compared with normal architecture in WT mice (s). In t, “BDL” indicates the bile duct lumen and “L” indicates an area of intercellular bile leakage. Biliary components are found in the lumen of cholestatic bile ducts (u) but also in the Disse space of the sinusoids (v) as signs of a disturbed junctional barrier in IKK1/2LPC-KO.
To further investigate the mechanisms underlying hyperbilirubinemia and reduction of portal bile ducts in IKK1/2LPC-KO mice, we performed ultrastructural analysis. Electron microscopy (EM) revealed tubular arrangements of hepatocytes and severe dilatation of bile canaliculi with disappearance of microvilli, formation of blebs, and thickening of pericanalicular ectoplasm, morphological findings consistent with severe cholestasis in IKK1/2LPC-KO mice (Fig. 3 q and r). Moreover, in the few remaining portal bile ducts, EM analysis revealed signs of disturbed intercellular connections with bile leakage between neighboring biliary epithelial cells, indicating disturbance of the biliary epithelial barrier (Fig. 3 s and t). Bile duct lumens filled with heterogeneous biliary components were observed (Fig. 3u), and, interestingly, these components were also found in the Disse space of sinusoids (Fig. 3v), suggesting that the blood–biliary barrier was disturbed in these livers. Impaired blood–bile barrier resulting in leakage of toxic bile components is likely to activate immune cells in the liver, leading to inflammatory destruction of portal bile ducts and, subsequently, severe cholangitis and cholestasis in IKK1/2LPC-KO mice. Collectively, these results show that combined ablation of IKK1 and IKK2 in hepatocytes and cholangiocytes results in destructive small bile duct disease, revealing a novel function of IKK1 and IKK2 to collaboratively maintain the integrity of the small bile duct system in the liver.
Combined Ablation of IKK1 and NEMO in Liver Parenchymal Cells Results in Bile Duct Disease Similarly to Double IKK1/IKK2 Deficiency.
To investigate whether IKK1 and IKK2 control biliary system homeostasis independently from NEMO, we generated mice with combined deletion of IKK1 and NEMO (IKK1/NEMOLPC-KO) or IKK2 and NEMO (IKK2/NEMOLPC-KO) in liver parenchymal cells (Fig. 4 a and b). Double deficient hepatocytes with combined ablation of IKK1/IKK2 or IKK2/NEMO showed similarly impaired NF-κB activation upon stimulation with TNF (Fig. 4c). In addition, p100 processing was inhibited in the liver of IKK1/NEMOLPC-KO mice (Fig. 4d), consistent with the specific function of IKK1 in inducing the alternative NF-κB pathway. Analysis of serum bilirubin revealed that IKK1/NEMOLPC-KO mice developed cholangitis similarly to IKK1/2LPC-KO mice, whereas IKK2/NEMOLPC-KO and also p65LPC-KO mice did not show this phenotype (Fig. 4e). Therefore, the development of cholangitis in this model is the result of inhibition of IKK1-specific signals in combination with impairment of canonical NF-κB signaling achieved by deletion of NEMO or of both IKK1 and IKK2. At this time, it remains unclear whether IKK1 mediates its bile-duct-specific functions through alternative NF-κB signaling or through an NF-κB-independent pathway. Because IKK1, but not IKK2, translocates to the nucleus in response to TNF stimulation in hepatocytes (Fig. 2b), inhibition of a nuclear function of IKK1 might also be implicated in the development of bile duct disease in these mice.
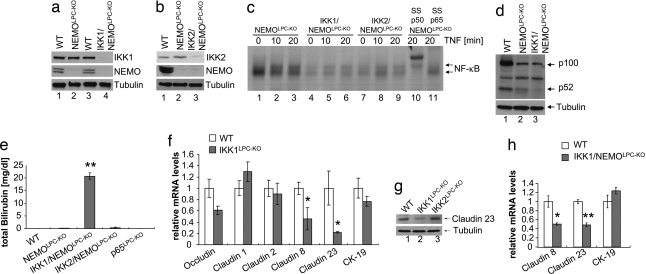
Combined inhibition of IKK1-specific functions regulating tight junction proteins in bile duct epithelium together with impairment of canonical NF-κB signaling causes bile duct disease. (a and b) Immunoblot analysis on primary hepatocyte protein extracts with indicated antibodies. (c) Primary hepatocytes were stimulated with TNF, and NF-κB DNA-binding activity was analyzed by EMSA. In lanes 10 and 11, antibodies against p50 or p65 were added for supershift analysis. This EMSA is overexposed to enhance the weak signals present in these IKK-deficient extracts. (d) Immunoblot analysis on primary hepatocyte protein extracts with an antibody recognizing full-length p100 and the cleaved form p52 (tubulin loading control). (e) Analysis of total serum bilirubin levels in mice with the indicated genotypes. Error bars denote SEM (n = 3). **, P < 0.01. (f) Relative mRNA expression of tight junction associated proteins was analyzed by quantitative RT-PCR in livers from 5-week-old mice. All values were normalized to ubiquitin expression. Cytokeratin 19 (CK-19) expression was measured to control for the presence of similar numbers of bile ducts in the samples used for analysis. Error bars denote SEM (n = 5). *, P < 0.05. (g) Claudin 23 expression was analyzed in livers from 8-week-old mice by immunoblot. (h) Relative mRNA expression of claudins 8 and 23 and of CK19 in livers from WT and IKK1/NEMOLPC-KO mice. All values were normalized to ubiquitin expression. Error bars denote SEM (n = 4). *, P < 0.05; **, P < 0.01.
IKK1 Regulates the Expression of Tight Junction Proteins in Biliary Epithelial Cells.
Our ultrastructural analysis indicated impairment of the blood–epithelial barrier in IKK double mutant mice. It has been shown previously that regulation of gap and tight junctions in liver cells is crucial for maintaining the blood–biliary barrier (21) and that tight-junction-related genes are regulated by inflammatory pathways in the liver (22). We showed previously that IKK1 is involved in maintaining the epidermal barrier by transcriptional regulation of tight junction proteins such as claudins (11). Moreover, human patients with NISCH-syndrome caused by mutations in the CLAUDIN1 gene show severe abnormalities in both the skin and the biliary system (23), suggesting that tight-junction-related defects may affect both the epidermal barrier and bile duct integrity. Therefore, we hypothesized that disturbance of IKK1-dependent tight junction protein expression could be involved in triggering bile duct disease in IKK1/2LPC-KO and IKK1/NEMOLPC-KO mice and analyzed how ablation of IKK1 affects the expression of various tight junction components in the liver. Indeed, expression of claudin 8 and claudin 23 was significantly down-regulated in the liver of IKK1LPC-KO mice compared with littermate controls, whereas expression of cytokeratin-19 (CK-19), a marker for biliary epithelial cells in the liver, was not affected, indicating the presence of similar amounts of biliary epithelial cells in the liver tissue analyzed (Fig. 4f). Down-regulation of claudin 23 in IKK1-deficient livers was confirmed at the protein level by immunoblot analysis (Fig. 4g). Expression of claudins 8 and 23 was also reduced in the liver of IKK1/NEMOLPC-KO mice compared with littermate controls (Fig. 4h). These findings indicate that impaired IKK1-dependent tight junction protein expression in biliary epithelial cells could contribute to the development of cholangitis in IKK1/IKK2LPC-KO and IKK1/NEMOLPC-KO mice. However, it is intriguing that single IKK1LPC-KO mice do not develop cholangitis despite impaired expression of claudins 8 and 23. Instead, lack of IKK1 leads to bile duct disease only when it is combined with complete inhibition of canonical NF-κB signaling in IKK1/2LPC-KO and IKK1/NEMOLPC-KO mice. Since inhibition of canonical NF-κB signaling in NEMOLPC-KO mice results in chronic liver disease characterized by increased hepatocyte apoptosis, liver inflammation and increased expression of TNF and other cytokines (8), it is likely that inflammation is an essential pathogenic component for the development of cholangitis in these mice. Indeed, infiltration of activated immune cells such as macrophages and lymphocytes in the liver has been suggested to be a priming event for bile duct injury and obstruction in humans (24). Furthermore, it has been shown that TNF disrupts the barrier function of cholangiocytes (25). In addition, inhibition of canonical NF-κB signaling caused increased liver damage upon bile duct ligation-induced cholestasis in mice, suggesting that NF-κB is crucial to protect liver cells from apoptosis during obstructive cholestasis (26). Taken together, these results suggest that the development of cholangitis in IKK1/NEMOLPC-KO and IKK1/IKK2LPC-KO mice is likely caused by an additive effect of liver inflammation and increased susceptibility of NF-κB-deficient liver parenchymal cells to cytokine-induced apoptosis, together with the impairment of an IKK1-specific function that regulates tight junction formation in cholangiocytes and the blood–biliary barrier.
Liver parenchymal cell restricted ablation of NEMO causes spontaneous chronic hepatitis characterized by increased apoptosis and compensatory proliferation of hepatocytes, liver inflammation, and steatosis, and ultimately results in the development of liver tumors resembling human hepatocellular carcinoma (HCC) with 100% penetrance at the age of 1 year (8). Because of the poor postnatal survival of IKK1/2LPC-KO mice, it has not been possible to obtain animals older than 7 months. Therefore, we could not assess whether these mice develop liver tumors similarly to NEMOLPC-KO mice at the age of 1 year. However, histological analysis of livers from IKK1/2LPC-KO mice at the age of 6 months did not show the early signs of tumor development, such as dysplasia, and severe anisokaryosis detected in NEMOLPC-KO mice of similar age. Furthermore, also mice with combined conditional ablation of IKK1 and NEMO in the liver did not show signs of HCC development at the age of 9 months (data not shown), suggesting that the additional ablation of IKK1 protects the NEMO-deficient liver from cancer development. Although at this stage it is not clear why the combined ablation of IKK1 and IKK2 or IKK1 and NEMO does not result in HCC development, it is likely that the additional ablation of IKK1 interferes with liver cancer development in this model. Although the mechanisms by which IKK1 might control liver tumorigenesis in NEMOLPC-KO mice remain elusive, recent studies implicated IKK1 and the alternative NF-κB pathway in oncogenesis (27–31). In the mammary gland, IKK1, p100/p52, and relB were shown to regulate cyclin D1 expression and epithelial cell proliferation, and mice lacking inducible IKK1 kinase activity showed delayed development of ErbB2-induced tumors, implicating alternative NF-κB signaling in mammary epithelial hyperplasia and oncogenesis (27, 29, 31). In addition, IKK1 was recently shown to antagonize p53-mediated gene transcription by mediating phosphorylation of CBP and switching its preference toward NF-κB-induced gene expression promoting cell growth (28). Moreover, p52 has been reported to promote cell growth by inducing the expression of cyclin D1 and repressing p53-mediated genes (30). The role of IKK1 and the alternative NF-κB pathway in suppressing p53-induced genes and inducing genes promoting cell proliferation might be relevant for HCC development in NEMOLPC-KO mice. Furthermore, lymphotoxin-β (LT-β) signaling was shown to be required for progenitor cell (oval cell)-dependent liver regeneration, suggesting that LT-β receptor-mediated activation of the alternative NF-κB pathway could be involved in the induction of oval cell proliferation (32). Because oval cells are thought to contribute to the development of liver tumors (33), IKK1-dependent activation of alternative NF-κB signaling downstream of LT-β receptor could also be involved in liver tumorigenesis by regulating liver progenitor cell proliferation.
In conclusion, our studies revealed two previously unrecognized functions of the two IκB kinases in the liver that are essential to protect this tissue from inflammation and disease. First, we demonstrate that IKK1 and IKK2 share a redundant function in mediating canonical NF-κB signaling in hepatocytes and protecting the liver from TNF toxicity. Second, we show that the combined ablation of IKK1-specific functions, most probably related to the regulation of tight junctions in biliary epithelial cells, and of canonical NF-κB signaling in the liver causes a destructive bile duct disease and severe cholangitis. The mechanisms regulating the development of cholangitis and cholestasis during chronic hepatitis and the genetic pathways modulating bile duct integrity in the liver remain poorly understood (2, 24). Our results point toward a potential role of IKK1 in human bile duct disease and substantiate genetic analysis in patients with cholangitis or biliary atresia for mutations in the CHUK/IKK1 gene. Such analysis might be especially promising in patients with overlap syndrome comprising features of autoimmune hepatitis and sclerosing cholangitis (34).
Materials and Methods
Mice.
The mouse strains used in this study are described in detail in SI Materials and Methods and Fig. S3. Animals received humane care, and all experiments were performed according to European, national, and institutional regulations.
Liver Injury Model.
Liver injury experiments were performed on male mice between 6 and 8 weeks of age. LPS (Sigma) was administered i.p. at a concentration of 25 μg/10 g body weight. Serum alanine aminotransferase (ALT), aspartate aminotransferase (AST), and alcalic phosphatase (AP) activities, and total and direct serum bilirubin levels were measured by standard clinical chemistry procedures.
Primary Hepatocyte Cultures.
Primary mouse hepatocytes were isolated by collagenase perfusion as described in ref. 8. In short, mouse livers were perfused with Krebs–Ringer buffer followed by perfusion of the liver with collagenase solution. Isolated hepatocytes were washed intensely with PBS. After determination of viability (>95%), 8 × 105 cells were plated on 6-cm dishes in Williams Medium E (Sigma–Aldrich) containing 10% FCS, penicillin/streptomycin, and insulin/transferrin/selenium supplement (Sigma). The medium was changed after 4 h. Before stimulation, the medium was changed to serum/supplement-free medium. Cells were stimulated with murine recombinant TNF (10 ng/ml).
Biochemical and Molecular Assays.
The protocols for the biochemical, molecular, and immunocytochemical assays used in this study are described in detail in SI Materials and Methods.
Statistics.
Results are expressed as the mean ± SEM or SD as indicated. Statistical significance between experimental groups was assessed by using an unpaired two-sample Student t test.
Acknowledgments.
We thank G. Schütz (German Cancer Research Center, Heidelberg, Germany) for the Alfp-cre transgenic mice; H. Buss and M. Kracht (Hannover Medical School, Hannover, Germany) for the GST-IκBα (1–54) plasmid; C. Libert [Flanders Institute for Biotechnology (VIB), Ghent, Belgium] for recombinant TNF; T. Tropartz, T. Gillis, and C. Uthoff-Hachenberg for technical assistance; and M. Lüdde and C. Netzer for valuable discussions. This work was supported by European Union Grants MUGEN LSHG-CT-2005-005203 and IMDEMI MRTN-CT-2004-005632 (to M.P.). T.L. was supported by a postdoctoral fellowship from the Schering Foundation, Berlin. J.H. was supported by a Ph.D. fellowship from the International Graduate School in Genetics and Functional Genomics at the University of Cologne.
Footnotes
The authors declare no conflict of interest.
This article is a PNAS Direct Submission. A.S.B. is a guest editor invited by the Editorial Board.
This article contains supporting information online at www.pnas.org/cgi/content/full/0800198105/DCSupplemental.
References
Articles from Proceedings of the National Academy of Sciences of the United States of America are provided here courtesy of National Academy of Sciences
Full text links
Read article at publisher's site: https://doi.org/10.1073/pnas.0800198105
Read article for free, from open access legal sources, via Unpaywall:
https://europepmc.org/articles/pmc2474544?pdf=render
Free after 6 months at www.pnas.org
http://www.pnas.org/cgi/content/abstract/105/28/9733
Free after 6 months at www.pnas.org
http://www.pnas.org/cgi/content/full/105/28/9733
Free after 6 months at www.pnas.org
http://www.pnas.org/cgi/reprint/105/28/9733.pdf
Citations & impact
Impact metrics
Citations of article over time
Smart citations by scite.ai
Explore citation contexts and check if this article has been
supported or disputed.
https://scite.ai/reports/10.1073/pnas.0800198105
Article citations
Implications of neonatal absence of innate immune mediated NFκB/AP1 signaling in the murine liver.
Pediatr Res, 95(7):1791-1802, 23 Feb 2024
Cited by: 1 article | PMID: 38396130
IKKε and TBK1 prevent RIPK1 dependent and independent inflammation.
Nat Commun, 15(1):130, 02 Jan 2024
Cited by: 2 articles | PMID: 38167258 | PMCID: PMC10761900
NEMO- and RelA-dependent NF-κB signaling promotes small cell lung cancer.
Cell Death Differ, 30(4):938-951, 18 Jan 2023
Cited by: 3 articles | PMID: 36653597 | PMCID: PMC10070460
A novel IRAK4/PIM1 inhibitor ameliorates rheumatoid arthritis and lymphoid malignancy by blocking the TLR/MYD88-mediated NF-κB pathway.
Acta Pharm Sin B, 13(3):1093-1109, 05 Dec 2022
Cited by: 10 articles | PMID: 36970199 | PMCID: PMC10031381
p62 Promotes Survival and Hepatocarcinogenesis in Mice with Liver-Specific NEMO Ablation.
Cancers (Basel), 14(10):2436, 15 May 2022
Cited by: 0 articles | PMID: 35626041 | PMCID: PMC9139637
Go to all (58) article citations
Data
Data behind the article
This data has been text mined from the article, or deposited into data resources.
BioStudies: supplemental material and supporting data
Similar Articles
To arrive at the top five similar articles we use a word-weighted algorithm to compare words from the Title and Abstract of each citation.
Zebrafish IkappaB kinase 1 negatively regulates NF-kappaB activity.
Curr Biol, 15(14):1291-1295, 01 Jul 2005
Cited by: 50 articles | PMID: 16051172
Human T-cell lymphotropic virus type 1 tax induction of biologically Active NF-kappaB requires IkappaB kinase-1-mediated phosphorylation of RelA/p65.
J Biol Chem, 279(18):18137-18145, 12 Feb 2004
Cited by: 78 articles | PMID: 14963024
NF-kappa B regulation by I kappa B kinase-2 in rheumatoid arthritis synoviocytes.
J Immunol, 166(4):2705-2711, 01 Feb 2001
Cited by: 87 articles | PMID: 11160335
Regulation and function of IKK and IKK-related kinases.
Sci STKE, 2006(357):re13, 17 Oct 2006
Cited by: 758 articles | PMID: 17047224
Review