Abstract
Free full text

Inhibition of Metastatic Outgrowth From Single Dormant Tumor Cells by Targeting the Cytoskeleton
Abstract
Metastatic breast cancer may emerge from latent tumor cells that remain dormant at disseminated sites for many years. Identifying mechanisms regulating the switch from dormancy to proliferative metastatic growth has been elusive due to the lack of experimental models of tumor cell dormancy. We characterized the in vitro growth characteristics of cells that exhibit either dormant (D2OR, MCF7, K7M2-AS.46) or proliferative (D2.A1, MDA-MB-231, K7M2) metastatic behavior in vivo. Although these cells proliferate readily in 2D culture, we demonstrate that when grown in 3D matrix, distinct growth properties of the cells were revealed that correlate to their dormant or proliferative behavior at metastatic sites in vivo. In 3D culture, cells with dormant behavior in vivo remained cell cycle arrested with elevated nuclear expression of p16 and p27. The transition from quiescence to proliferation of D2A1 cells was dependent upon fibronectin production and signaling through integrin β1, leading to cytoskeletal reorganization with f-actin stress fiber formation. We demonstrate that phosphorylation of myosin light chain by MLC kinase (MLCK) through integrin 1 is required for actin stress fiber formation and proliferative growth. Inhibition of integrin β1 or MLCK prevents transition from a quiescent to proliferative state in vitro. Iinhibition of MLCK significantly reduces metastatic outgrowth in vivo. These studies demonstrate that the switch from dormancy to metastatic growth may be regulated, in part, through epigenetic signaling from the microenvironment leading to changes in the cytoskeletal architecture of dormant cells. Targeting this process may provide therapeutic strategies for inhibition of the dormant-to-proliferative metastatic switch.
Introduction
Recurrence of breast cancer often follows a long latent period where metastases may become clinically apparent many years after removal of the primary tumor and adjuvant therapy. Recent evidence suggests that in many such cases, tumor cells have already seeded metastatic sites, even though the primary disease is diagnosed at an early stage (1–3). Approximately 30% and 74% of breast cancer patients diagnosed at the M0 or M1 TMN tumor stages, respectively, were found to have breast cancer cells in their bone marrow, although these cells appear to primarily exist as micrometastases that are not clinically manifest (1). Most of these micrometastatic cells do not express proliferation markers and, therefore, represent quiescent dormant tumors cells (QTCs), also referred to as cellular dormancy (1, 4–6). These cells are resistant to conventional therapies that target actively dividing cells (1, 7) which likely accounts for disease recurrence following apparent successful treatment of primary tumors. It has been proposed that cellular dormancy represents an early, quiescent phase of tumor cell dormancy that may progress to proliferative, micrometastases, whose size is limited by the lack of an angiogenic resonse, which may ultimately transition through an angiogenic switch and become clinically apparent metastases (6, 8).
Dissecting the mechanisms that either maintain prolonged cellular dormancy or activate dormant tumor cells to proliferate, and developing therapeutic approaches to eliminate dormant tumor cells, have been hampered by the lack of model systems that mimic the in vivo behavior of cellular dormancy and the emergence of clinical metastatic disease. Traditional 2-dimensional (2-D) cell culture techniques fail to recapitulate the in vivo dormant behavior of tumor cells. For instance, our previous work demonstrated that D2.0R mammary tumor cells exhibit dormant behavior at metastatic sites when injected into mice, but these cells readily proliferate when cultured in 2-D conditions (5), suggesting that the microenvironment may play an important role in tumor cell dormancy.
The tumor microenvironment has been increasingly recognized as a critical regulator of cancer progression (reviewed in (6, 9, 10)). The extracellular matrix (ECM), a key component of the microenvironment, is in immediate contact with the tumor cells and functions as a critical source for growth, survival, motility, and angiogenic factors that significantly affect tumor biology and progression. Additionally, cell adhesion to the ECM triggers intracellular signaling pathways that can regulate cell cycle progression, migration, and differentiation (11, 12) through integrins and other cell surface receptors. Thus, interactions between tumor cells and the ECM are critical modulators of the metastatic potential of tumor cells.
Culturing cells in 3D basement membrane cultures has been utilized in the past to study morphogenesis, differentiation, tumorigenesis, motility and invasion of cells through the basement membrane (12, 13). In this study, we characterize a novel 3-D in vitro system in which growth characteristics of several tumor cell lines in ECM correlate with the dormant or proliferative behavior of the tumor cells at a metastatic secondary site in vivo. Our results reveal that a stage of prolonged tumor cell quiescence, presumably preceding a later stage that is dependent upon angiogenesis for metastatic growth, exists due to cell cycle arrest. However, we demonstrate that the switch from quiescence to proliferative metastatic growth is strongly influenced by interactions with the ECM. Specifically we show that fibronectin signaling through Integrin β1 induces the switch from quiescence to proliferative growth. The transition is associated with dramatic reorganization of the cytoskeleton and activation of myosin light chain kinase (MLCK). Pharmacological and shRNA targeting of cytoskeletal reorganization via inhibition of MLCK inhibited metastatic growth of QTCs in vivo, suggesting that interference with cytoskeletal reorganization may be an important avenue for preventing recurrent disseminated disease.
Methods
3-D cell cultures
Mouse mammary cancer cells D2.0R, D2A1 (5, 14) and 4T1 (15) (obtained from Dr. Fred Miller), mouse osteosarcomas K7M2 and K7M2AS1.46 cells (16), human MDA-MB-231 (ATCC, Rockville, MD), MDA-MB-231 stably expressing GFP (17) (obtained from Dr. Danny Welch) and MCF-7 breast cancer cells (ATCC, Rockville, MD) were maintained in Dulbecco’s Modified Eagle’s Medium (DMEM) high glucose, 10% FBS and antibiotics (GIBCO BRL, Bethesda, MD). The cells were cultured in growth factor-reduced 3-D Cultrex® Basement Membrane Extract (Trevigen Inc., Gaithersburg, MD), as previously described (18) with slight modifications as described in the Supplementary Methods. Cell morphology was monitored by light microscopy, and time -lapse images were captured by a Zeiss LSM 510 confocal laser scanning microscope. ML-7 (Biomol International L.P Plymouth Meeting, PA) and W13 (Calbiochem, San Diego, CA) were used to inhibit MLCK and calmodulin, respectively. Antibody against Integrin 1 clone 9EG7 sodium azide free was used to neutralize Integrin β1 function (BD Biosciences, San Jose, CA) and control cultures were treated with the same concentration of nonspecific IgG.
shRNA silencing experiments
An shRNA expression vector targeting MLCK was generated as described in the Supplementary Methods. Scrambled shRNA was used as a negative control. shRNA was transfected to D2A1 cells (0.5x106 cells) using Amaxa Nucleofector technology (Amaxa, Inc., Gaithersburg, MD), trypsinized (Invitrogen Carlsbad, CA) after 48 h and cultured in 3-D for five days. In parallel, RNA was isolated from the transfected cells from 2-D culture at 120 h. The RNA was reverse-transcribed using RNase H reverse transcriptase (SuperScript II, Invitrogen, Carlsbad, CA). Semiquantitative PCR was repeated in triplicate using MLCKprimers: forward primer 5′CTCGGAACCTCTGCGTCAAAG-3′ and reverse primer -5′CAGTCTACCATGTCAACGCTA-3′.
Proliferation assay
96 well plates were coated with 50–100 l Cultrex®. Cells were resuspended in 100 l DMEM low glucose supplemented with 2% FBS and 2% Cultrex® or 2% FBS supplemeted with Cultrex®+Fibronectin, and grown on the coated plates. 1.5–2 X 103 cells/well (for D2A1, D2.0R, MDA231, MCF-7 and 4T1) and 2.0x103 cells/well for K7M2 and K7M2AS1. 46. The Cell Titer 96® AQueous One Solution cell proliferation assay kit (Promega; Madison, WI) was used to measure cell proliferation as described in the Supplementary Methods.
Immunostaining
Cells (1x104) were cultured in 3-D Cultrex® using 24 well plates coated with 300 μl Cultrex®. The cells were fixed with 10% buffered formalin. Paraffin sections were analyzed by hematoxylin and eosin (H&E), TUNEL staining, and Ki67 (Vector laboratories, Burlingame, CA) immunohistochemistry. Immunostaining for p27 (Medical and Biological Laboratories, Naka-ku Nagoya, Japan) (1:50) and p16 (Cat# Sc-1661, Santa Cruz Biotechnologies; Santa Cruz, CA) (1:50) was carried out with the DAKO ARK, peroxidase kit (DAKO, Glostrup, Denmark). Antigen retrieval was carried out for p27 staining using Citrate Buffer. Multiple sections from the 3-D Cultrex® blocks were analyzed. At least 100 cells were analyzed for immunoreactivity.
Animal studies
D2A1 and MCF cells were infected with the lentivirus pHR-GFP and D2.0R cells were infected with pSico-GFP (19). MCF-7, MDA-MB-231 and D2A1 stably expressing GFP were additionally labeled with Cell tracker™ Green CMFDA (5-chloromethylfluorescein diacetate) according to the manufacturer’s protocol (Molecular Probes, Eugene, Oregon). 4–6-week-old female BALB/c-nu/nu athymic mice were injected via tail vein with 1x 106 cells. In the case of MCF-7 cells the mice were implanted 24h post injection, with 17β Estradiol 0.36mg/pellet for 60 days. Cytoskeletal organization and immunostaining were examined in dormant and proliferating metastatic cells in vivo as described in the Supplementary Methods. For inhibition of myosin light chain kinase activity in D2A1 cells in vivo, mice were injected with D2A1 cells stably expressing GFP and labeled with Cell tracker™. Mice were anesthetized 24h post injection and a small horizontal incision was made in the interscapular area into which a 200 μl osmotic pump (Alzet, Cupertino, CA) with a 0.25 μl/hour release rate was implanted containing either ML-7 (22mM) in dimethyl sulfoxide (DMSO) (experimental group) or DMSO (control group). Mice were sacrificed after 1 week, lungs were removed, inflated with PBS and subjected to SCOM imaging immediately. All mice were treated in accordance with the guidelines of the Animal Care and Use of Laboratory Animals (NIH publication No. 86-23, 1985) under 7 an approval animal protocol.
In vivo/ex vivo imaging
Lungs removed from the mice, as described in the Supplementary Methods, were imaged by inverted fluorescent videomicroscopy (Leica DM IRB) at x 25 and 100 magnification. The whole lung was sequentially captured for each mouse at x100 magnification. Each captured frame was analyzed using OpenLab software to measure the surface area of the metastases that are larger than 10 pixels. The percentage of lesions <1000 pixels (single cells) vs. lesions>1000 pixels.
Immunofluorescence
Staining for f-actin, Integrin β1 and fibronectin in vitro was carried out by overnight incubation, as described in the Supplementary Methods. Frozen lung sections (8 μm) were fixed with 4% PFA for 10 min, washed with PBS (3x 5 min), and blocked with 5% BSA (Sigma, St. Louis, MO) for 15 min. Slides were then washed 3X with PBS (as above) and incubated with Alexa Texas Red®-X phalloidin (Molecular Probes, Eugene, Oregon) (1:20) for 1 h at 37°C, washed 3X with PBS, and mounted with VECTASHIELD mounting medium with DAPI. The slides were imaged using a Leica confocal microscope (Leica Microsystems AG, Wetzlar, Germany).
Statistical analyses
Student’s t -test was used for the proliferation assays and for the in vivo analysis. Statistical significance was defined as *P≤0.05, ** P≤0.001 and *** P≤0.0001.
Results
In vitro model for solitary tumor cell dormancy
To explore whether the ECM influences the dormant (non-proliferative) or proliferative behavior of metastatic cells, we initially studied the well characterized D2.0R, and related D2A1 mammary tumor cell model system for tumor cell dormancy (5, 7, 20). When injected into mice, D2.0R cells invade distant metastatic sites, remain as single quiescent cells for prolonged periods of time, but occasionally may emerge from a dormant state and proliferate into metastatic tumors. In contrast, D2A1 cells remain dormant for a relatively short period of time following injection and subsequently form numerous metastatic tumors quite rapidly compared to D2.0R cells. Despite the divergent behavior of these cells in vivo, both cell lines readily proliferate when cultured in 2-D on a plastic substrate (Figure 1A). When these cells are cultured in 3-D Cultrex® basement membrane extracted from a transplantable murine tumor, D2.OR cells do not proliferate but remain quiescent through the entire experimental 14 day culture period (Figure 1A and B), whereas the highly metastatic D2A1 cells only remain quiescent for four to six days after which they begin to proliferate (Figure 1A and B). During the initial quiescent phase of both cell lines in the 3-D culture, many cells remain solitary, whereas other non-proliferating cells aggregate and form multi-cellular spheroids (Supplementary Figure 1 and Supplementary videos 1&2). Thus, these initial studies suggested that when exposed to a 3-D environment in vitro, the distinct growth characteristics of these cells could be correlated to their dormant or proliferative behavior at the metastatic sites in vivo.
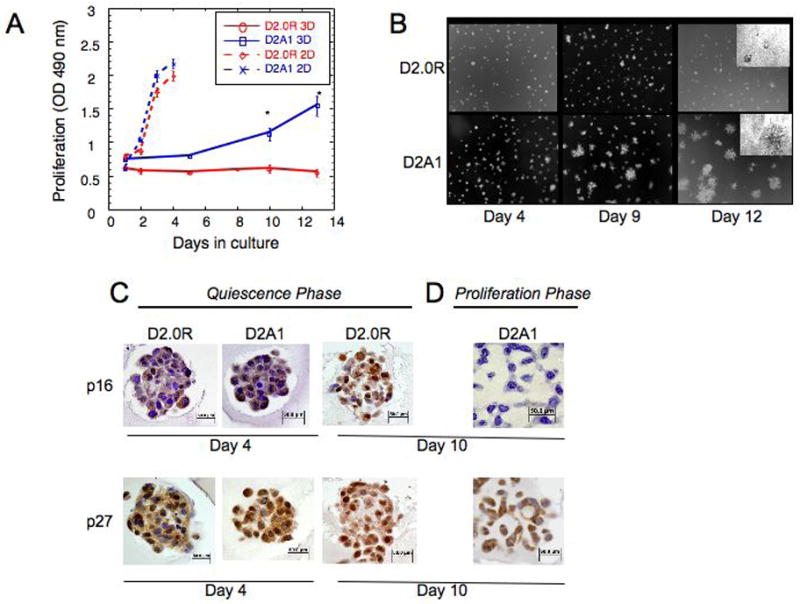
A) Proliferation of D2.0R and D2A1 in 2-D culture and in 3-D Cultrex® (as described in Methods) n=8 (mean ± SE). Representative result of three experiments (* p≤0.05). B) D2.0R and D2A1 cells were cultured in 3-D Cultrex® (as described in Methods). Images of the cells were acquired at day 4, day 9 and day 12, magnification x20. Images in the upper right corner, day 12, magnification x40. C–D) Quiescence in the 3D is associated with elevated p16 and p27. Immunohistochemical staining for p16 and p27. C) Quiescent phase: D2.0R cells, culture days 4 and 10; D2A1 cells, day 4. D) Proliferative phase of D2A1 cells, day 10. Note loss of p16 expression and reduction of p27 nuclear localization during D2A1 proliferative phase, but continued high expression in quiescent D2.OR cells. Magnification x40.
Solitary dormant tumor cells are cell cycle arrested
To determine the growth dynamics of D2.0R and D2A1 cells in 3-D culture, staining for the proliferation marker proliferation Ki67 and for apoptosis (TUNEL assay) was performed at day 4 of in vitro culture, when no differences in overall cell number were observed for both cell lines. Few D2.0R or D2A1 cells exhibited nuclear staining for Ki67 (5 and 7%, respectively), and TUNEL staining for apoptosis was negative in both cell lines (data not shown). D2.0R and D2A1 cells exhibited low levels of nuclear staining for p16 at culture day 4 (13% and 17%, respectively) (Figure 1C). However, in both cell lines, over 60% of the nuclei stained positively for p27 (Figure 1C). After 10 days in culture, only 25 % of D2A1 cells exhibited nuclear staining for p27 (Figure 1D). Similarly, p16 staining was absent in proliferating D2A1 cells at day 10 (Figure 1D), whereas, 50% of the nuclei of D2.0R cells stained positively for p16 and 77% of the nuclei stained positively for p27 at day 10 (Fig 1C). Thus, the lack of growth of these cell types in 3-D culture is not a consequence of a balance between apoptosis and proliferation, but rather due to cell cycle arrest.
3D culture as a predictive model for cellular dormancy
The growth characteristics of additional cell lines with known in vivo metastatic growth properties were assessed. The metastatic mammary cancer cell lines, human MDA-MB-231 and mouse 4T1 cells, and the human MCF-7 cell line, which rarely develops metastases when injected into nude mice (21), were investigated using the 3-D system. MDA-MB-231 cells remained quiescent for only two days in 3-D culture and subsequently began to proliferate at day 3, while 4T1 cells proliferated within one day in 3-D culture (Figure 2A,B). In contrast, MCF-7 cells remained quiescent for the 14 day culture period (Figure 2A,B). Similar to D2.0R cells, a high percentage (50%) of MCF-7 cells demonstrated nuclear staining for p27 at day 10 (data not shown).
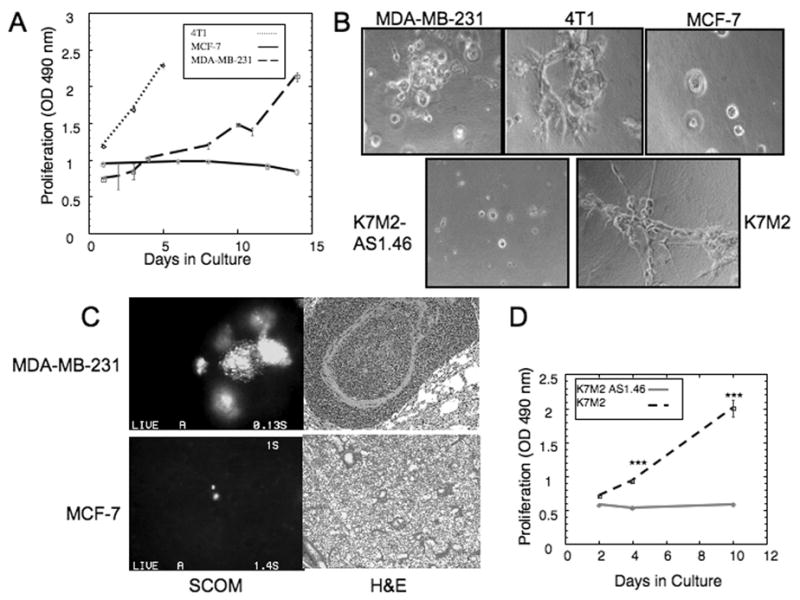
A) Proliferation of MDA-MB-231, 4T1, and MCF-7 cells in 3-D Cultrex® n=8 (mean ± SE). Representative result of three experiments. B) Morphology of MDA-MB-231, 4T1, MCF-7, K7M2 AS1.46 and K7M2 cells cultured in 3-D Cultrex®, magnification x40. C) Representative lungs from a mouse injected with GFP-expressing MDA-MB-231 (2 weeks post injection) or MCF-7 cells (9 weeks post injection). Left panels: SCOM images of MDA-MB-231 lung metastasis and dormant MCF-7 cell, magnification x100. Right panels: representative H&E staining of MDA-MB-231 lung metastasis and of lung with MCF-7 cells lacking proliferative metastases, magnification x20. D) Proliferation of K7M2 and K7M2 AS1.46 cells in 3-D Cultrex® n=8 (mean ± SE). Representative result of three experiments. (*** p≤0.001).
Importantly, we determined that MCF-7 cells exhibit dormant properties when disseminated to the lung as a metastatic site. GFP-expressing MCF-7 and MDA-MB-231 cells were injected via tail vein into nude mice (see Methods). Lungs were removed 2 weeks post-tail vein injection for GFP-expressing MDA-MB-231 cells (due to the rapid development of metastases) or 9 weeks post-injection for MCF-7-GFP expressing cells (which had not developed multicellular metastases by that time) an imaged using fluorescent single cell whole organ microscopy (SCOM) (16) and histologically. While MDA-MB-231 cells developed large metastases (Figure 2C), only scattered, individual MCF-7 cells were found in the lungs with no evidence of proliferating, multi-cellular metastases (Figure 2C). Isolated MCF-7 cells were similarly identified in mice 6 months after cell injection (data not shown). Thus, the growth characteristics of MDA-MB-231 and MCF-7 cells in 3-D culture correlated with their dormant or poor metastatic growth potential in vivo.
Similar results were obtained for the previously described mouse osteosarcoma cell lines K7M2 (highly metastatic) and K7M2AS1.46, a clonal derivative of K7M2 engineered to express reduced levels of ezrin. Unlike K7M2, which are highly metastatic, the K7M2AS1.46 seed metastatic sites when injected in vivo, but do not form pulmonary metastases (16). Although both cell lines proliferate similarly in 2-D culture (data not shown), when introduced into the 3-D culture system, K7M2AS1.46 cells remain quiescent through the 14 day experimental period whereas, K7M2 cells were quiescent for only 4 days and subsequently began to proliferate (Figure 2B,D).
Interaction with the ECM alters cytoskeletal architecture and influences the dormant to proliferative switch
The transition of D2A1 cells from a quiescent to proliferative state in 3-D culture (Figure 1B) was associated with dramatic changes in cell morphology. Therefore, we examined changes in cytoskeleton reorganization during the transition from quiescence to proliferation.
Both D2.0R and D2A1 cells formed filopodia during their quiescent phase in 3D culture (Supplementary Figure 2A and B). Cells were stained with phalloidin on days 1, 4, and 7 to observe the organization of f-actin fibers (Figure 3A). D2.0R cells displayed cortical f-actin staining at all time points (Figure 3A, arrowheads). In contrast, f-actin stress fibers began to appear in D2A1 cells on day 4 (prior to cell proliferation, as seen in Figure 1B, arrow), and were most prominent in proliferating cells on day 7 (Figure 3A, arrows). Increased phosphorylation of myosin light chain (MLC), which is required for actin stress fiber formation (22), was elevated in D2A1 cells at days 4 and 7, correlating with extensive actin stress fiber formation (Figure 3B arrows; Supplementary Figure 2C) and the transition from a quiescent to a proliferative state. Similarly, the metastatic 4T1, MDA-MB-231, and K7M2 cells, formed actin stress fibers in the 3-D in vitro system in association with proliferation (Figure 3C, arrows). Like D2.OR cells, non-metastatic MCF-7 cells and K7M2AS1.46 cells displayed only cortical f-actin staining (Figure 3C, arrowheads). Thus, all of the non-proliferating cells studied exhibited only cortical f-actin localization, whereas cells that were emerging from quiescence had extensive stress fiber formation.
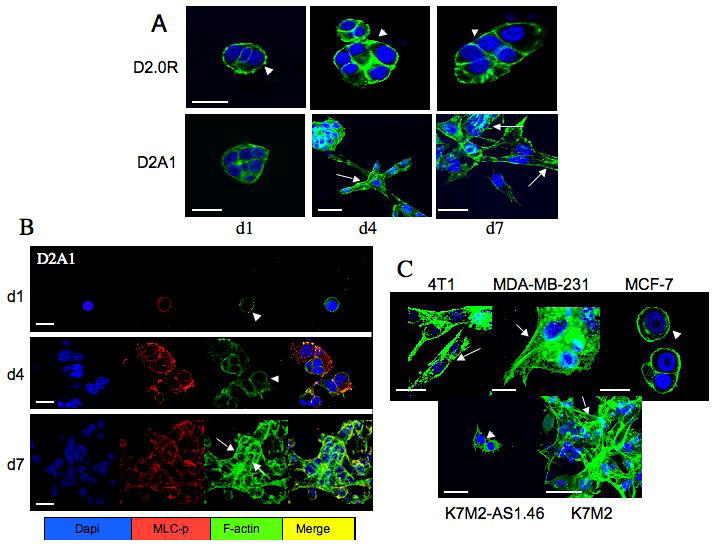
D2.0R, D2A1, MCF-7, MDA-MB-231, 4T1, K7M2 and K7M2 AS1.46 cells were cultured in 3-D Cultrex® on glass cover slips (as described in Methods). Cells were fixed and stained with DAPI (blue) for nuclear localization , phalloidin (green) for f-actin and with an antibody against the phosphorylated form of myosin light chain (red), as indicated at various time points; confocal microscopy, magnification x63. A) Proliferative growth is associated with actin stress fibers formation. Cortical f-actin staining was evident in D2.0R cells at day 1 (d1), day 4 (d4) and day 7 (d7) (arrowheads). D2A1 cells exhibited cortical f-actin at day 1(d1) (arrowhead), but actin stress fibers form at day 4 (d4) and day 7 (d7) (arrows). B) Actin stress fiber formation and proliferative growth is associated with MLC phosphorylation. D2A1 cells show localization of phosphorylated MLC with actin at day 1(d1), and with actin stress fibers by day 7 (d7). C) 4T1 and MDA-MB-231 (day 4) and K7M2 cells (day 6) displayed actin stress fibers (arrows), whereas non -proliferative MCF-7 (day 14) and K7M2-AS1.46 (day 10) displayed cortical f-actin staining (arrowheads). White bar equals 20 microns
Similar cytoskeletal reorganization was observed in vivo. Lungs removed from nude mice tail vein-injected via with GFP-expressing D2A1, D2.0R, MCF-7 or MDA-MB-231- cells (1x106 cells/mice) were imaged by SCOM (Figure 4A), and sections were then stained for f-actin (Figure 4B). Single dormant D2A1-GFP cells were apparent at 1 and 7 days post-injection (Figure 4A) and exhibited cortical staining for f-actin (Figure 4B, arrowheads). However, D2A1-GFP cells in growing metastatic lesions 3 weeks post-injection (Figure 4A) displayed cytoskeletal reorganization and formation of actin stress fibers (Figure 4B, arrows). Solitary dormant D2.0R-GFP cells (Fig 4A) with cortical f-actin staining (arrowheads) were detected 4 weeks post injection, while one out of five injected mice developed metastasis after 12 weeks (Fig 4A) that exhibited actin stress fiber formation (Fig 4B, arrows). Similarly, metastatic lesions from the MDA-MB-231 cell line (Figure 4A) formed actin stress fibers (Figure 4B, arrows), while solitary dormant MCF-7 cells (Figure 4A) displayed only cortical f-actin staining (Figure 4B, arrowheads). These results demonstrate that actin stress fiber formation is associated with proliferative metastatic growth in vivo.
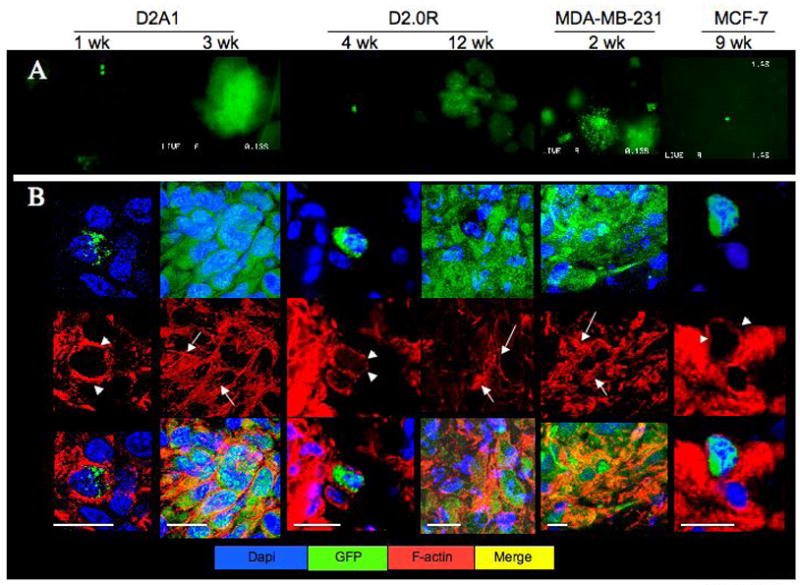
A) SCOM images (magnification x100) of lungs from mice injected with either D2A1-GFP (removed 1 or 3 weeks post-injection), D2.0R GFP (removed 4 or 12 weeks post-injection) MDA-MB-231-GFP (removed 2 weeks post-injection) or MCF-7-GFP cells (removed 9 weeks post-injection). Time points varied according to the growth properties of the cells. B) Frozen sections of lungs from mice injected with the above cells at the indicated time points stained for f-actin (red); confocal microscopy, white bar equals 20 microns. White arrowheads depict cortical actin staining; white arrows indicate f-actin stress fibers.
MLCK activation is required for actin stress fiber formation and the transition from dormancy to growth
To determine whether formation of actin stress fibers is required for the transition from a quiescence to proliferation, phosphorylation of MLC by MLCK required for f-actin stress fiber formation - was inhibited by 1) ML-7, a specific inhibitor of MLCK (23); 2) shRNA to target MLCK RNA; or 3) W13, a specific inhibitor of Ca2+ calmodulin that regulates MLCK activity (24). MLCK expression was significantly reduced by transfection of an MLCK shRNA expressing vector (see supplementary Figure 3A). MLCK activity in D2A1 cells was inhibited at day 5 with either ML-7, W13, or transfection of the MLCK shRNA targeting vector, leading to a reduction in phosphorylated MLC (localized mainly to the cytoplasmic membrane), disruption of actin stress fibers, and the rounding of cells (Figure 5A). Cell proliferation was reduced (p≤0.05) (Figure 5B) and p27 nuclear staining increased from 30% positive nuclei in the non-treated cells, to 50% positive nuclei in ML-7 treated cells (supplementary Fig 3B (arrow)). ML-7 also significantly inhibited proliferation (p≤0.05) in MDA-MB-231 and 4T1 cells (supplementary Figure 3C). These results demonstrate that MLCK activity and the formation of actin stress fibers are required for the transition of these metastatic cells from a quiescent to a proliferative state in vitro.

A) D2A1 cells were cultured in 3-D Cultrex on glass cover slips. Cells were untreated (control), or treated with ML-7 (5 μM), or with W13 (5μM) for 48 hr beginning on culture day 5, or treated with scrambled or MLCK shRNA as described in Methods, and stained for the phosphorylated form of myosin light chain (red), f- actin (green), and nuclei (blue). Merge of f-actin, and MLC-p staining (yellow). Light and confocal microscopy, magnification x40 and x63, respectively. White bar equals 20 microns B) Time course of D2A1 cell proliferation in 3-D Cultrex® in the absence or presence of ML-7 (5 μM), n=8 (mean ±SE). C) Inhibition of the metastatic outgrowth in lungs of mice treated with ML-7. Data presented as the percentage of single cells vs. proliferative metastatic lesions in mice that received either ML-7 (22mM) or vehicle (control group) (p≤0.0001 across all samples)(n=9). Single cells (<1000 pixel intensity) and clusters of cells (>1000 pixel intensity), as depicted in upper right panel.
The role of MLCK was further assessed in vivo. Nude mice were tail vein-injected with D2A1-GFP cells also labeled with Cell Tracker™ and treated systemically with either ML-7 or vehicle. In vivo targeted effects of ML-7 inhibition of MLC phosphorylation were demonstrated (see Supplementary Figure 3D). Lungs were removed, tumor cells imaged by SCOM and the areas of lung metastases were quantified by fluorescent signal. The vast majority (80%) of the metastatic lesions present in ML-7 treated mice persisted as single cells displaying cortical f-actin organization (data not shown), whereas, in the untreated control mice only 30% of the lesions were single cells and 70% of the metastatic lesions were clusters of cells. (Figure 5C, p≤0.001). Thus, inhibition of MLCK by ML-7 can significantly reduce the progression of solitary dormant tumor cells to proliferating metastatic lesions.
Fibronectin activation of MLCK through integrin β1 signaling activates the transition from quiesence to growth
Since fibronectin has been identified as one of several ECM genes in gene expression signatures related to breast cancer metastases (25, 26), we explored whether this ECM protein might play a role in the dormant to proliferative switch in the 3D system since Cultrex does not contain fibronectin.
D2A1 cells express very low levels of fibronectin by day 5 in 3D culture (data not shown) which significantly increases by day 7 (Fig 6A). Similarly lung lesions of D2A1-GFP cells express fibronectin (supplementary Figure 4A). In contrast, D2.0R cells do not express fibronectin throughout the 14 day culture period in matirx (Fig 6A). Importantly, both cell lines, as well as D2A1metastatic lesions express the fibronectin receptor-, integrin β1((Fig 6A); supplementary Fig 4A). However expression of integrin β1 in D2.0R cells is reduced over time in the 3D culture.
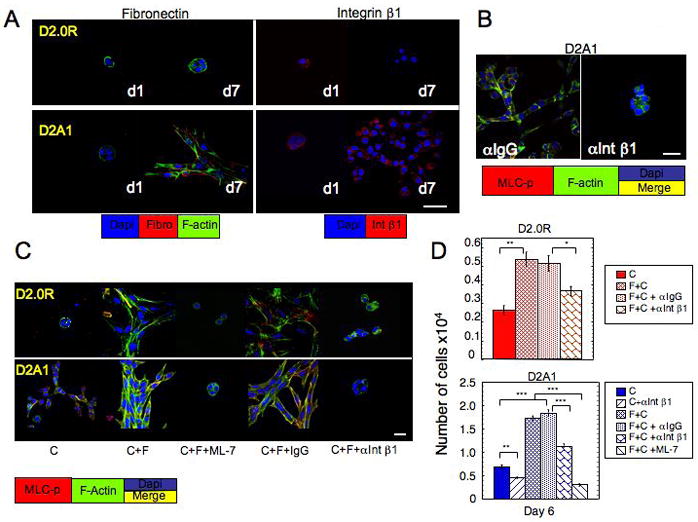
D2.0R and D2A1 cells were cultured in 3-D Cultrex® (designated as C) on glass cover slips (as described in Methods). A) Staining for fibronectin and Intβ1 in D2.0R and D2A1 cells day 1 and day 7. B, C) D2A1 and D2.0R cells stained for MLC-p (red) f-actin (green) and nuclei (blue). Merge of f-actin, and MLC-p staining (yellow). B) D2A1 cells treated with either control IgG or neutralizing antibody against Int 1 for 6 days. C) D2.0R or D2A1 cells cultured on 3-D Cultrex® mixed with fibronectin (designated as C+F) (750μg/ml) for 6 days in the presence of either nonspecific IgG (150μg/ml), antibody against Integrin β1 (150μg/ml) or ML-7 (5μM). Confocal microscopy, magnification x63, white bar equals 20 microns
In order to functionally test whether fibronectin, through integrin β1, could induce the dormant to proliferative switch requiring activation of MLC, we blocked integrin β1 function through the addition of 150μg/ml neutralizing antibody to integrin β1 (α Int β1) to the overlay media (see Methods). Inhibition of integrin β1 led to inhibition of MLC phosphorylation , loss of actin stress fiber formation and significant inhibition of D2A1 proliferation (Fig 6B,D; supplementary Fig 4B). Treatment with control IgG antibody had no effect. Supplementation of fibronectin to Cultrex (see Methods) induced spreading of D2A1 and D2.0R cells by day 3 (data not shown). By day 6 there was a greatly enhanced phosphorylation of MLC and actin stress fiber formation in both cell lines (Fig 6C; supplemental Fig 5). Addition of fibronectin to the matrix also significantly enhanced cell proliferation of D2A1 cells by day 6 and led to a modest proliferation D2.0R cells. However, fibronectin could not promote continuous proliferation of D2.0R cells by day 6 (Fig 6D). The effect of fibronectin on both cell lines was significantly inhibited by the neutralizing antibody to integrin β1. Addition of ML-7 (5 M) at day 3 for 72h inhibited the fibronectin effect on cell spreading and MLC phosphorylation in both cell lines, but had a significant inhibitory effect on proliferation only on D2A1 cells (Fig 6C–D; supplementary Fig 5). These results demonstrate that fibronectin signaling through integrin β1 activates phosphorylation of MLC by MLCK in D2A1 cells, leading to the transition from quiescence to growth.
Discussion
Mortality from breast cancer is primarily due to metastatic disease, which often appears years after successful treatment of the primary tumor, presumably due to the activation of latent, dormant metastatic tumor cells. Little is known about what maintains cellular dormancy and what evokes the emergence of proliferative metastatic growth from dormant tumor cells.
The focus of the present study has been to evaluate in vitro and in vivo models of solitary quiescent tumor cells that precede the dormant micrometastasic stage, and to demonstrate that growth of such cells can be highly influenced by the ECM. We report that quiescent or proliferative growth characteristics of tumors cells in a 3D in vitro culture system can be correlated with their in vivo proliferative metastatic growth potential. This system provides a basis for exploring mechanisms involving the ECM that regulate the maintenance of cellular dormancy or the switch to proliferative metastatic growth.
Although all cells examined in this study readily proliferate in 2D culture, an important finding of this work is the remarkably different growth characterisitics of the cells in 3D culture that correlates with their proliferative characteristics as disseminated metastases to the lung. D2.OR, MCF7 and K7M2-AS1.46 cells, which remain dormant in vivo, are quiescent in 3D culture, whereas highly metastatic D2A1, MDA-MB-231 and K7M2 cells readily proliferate in 3D culture after variable, but relatively short periods of quiescence. p16 and nuclear p27 were the predominant negative cell cycle regulators expressed in the quiescent cells, whereas their nuclear expression levels were significantly reduced in the cells that switched to a proliferative state.
There are several possibilities as to how the 3D environment may influence quiescence or proliferation, including the presence or absence of growth factors, cytokines, proteases, or alterations in the composition or structure of ECM proteins. Previous reports have demonstrated that contact with a component of the ECM inhibits metastatic melanoma cell proliferation (27, 28). Recent work has also demonstrated that changes in tensile properties of the ECM may be important regulators of malignant transformation of tumor cells (29).
Striking differences in cell morphologies were observed between the quiescent and proliferative cells in 3D leading us to explore further the cytoskeletal architecture of the cells in 3D since cell shape and cytoskeletal dynamics play critical roles in regulating cell cycle progression in anchorage dependent cells by influencing molecular signaling pathways (6, 29–31). Results from our study suggest that the cytoskeletal configuration within the cell is a major regulator of the transition from cellular dormancy to a metastatic proliferative state. Significant morphologic differences are observed between the dormant and metastatic cell lines when placed in 3-D culture. The metastatic cells form actin stress fibers during their transition from quiescence to proliferation, which indicates a strong adherence of the cells to the ECM (32). In contrast, the quiescent cells remain rounded, with cortical actin staining, formation of filopodia representing transient attachment to the matrix, and no evidence of actin stress fiber formation in vitro.
A similar relationship between cytoskeletal dynamics and proliferative activity is observed when the cells are analyzed using an in vivo experimental metastasis model system. D2A1 and MDA-MB-231 cells undergo cytoskeletal reorganization with actin stress fiber formation during their transition from cellular dormancy to proliferative metastases in the lung in vivo. Similarly, after a long latency, the occasional metastatic outgrows of D2.OR cells form actin stress fibers as well. MCF-7 cells do not form proliferative metastatic lesions in the lung, but remain as solitary dormant cells with cortical actin staining.
In order to functionally assess the role of stress fiber formation in the dormant to proliferative switch, we attempted to inhibit actin stress fiber formation by blocking two known pathways involved in stress fiber formation, Rho kinase (32) and myosin light chain kinase (MLCK). Although Rho signaling has been shown to be important in metastatic processes (33), inhibition of Rho did not inhibit stress fiber formation or the quiescent to proliferative switch of D2A1 cells in 3D culture. However, inhibition of MLCK (either with shRNA, the inhibitor ML-7 or indirectly with W13 through inhibition of the MLCK activator calmodulin (34)) prevented stress fiber formation and proliferative growth of D2A1, MDA-MB-231 and 4T1 cells in 3D culture. This was accompanied by increased nuclear p27. Inhibition of proliferative outgrowth with W13 to block calmodulin function suggests that altering calcium flux may also significantly influence cytoskeletal dynamics and the dormant to proliferative switch of QTCs.
In order to determine whether inhibition of MLCK could reduce metastatic outgrowth in vivo, ML-7 or vehicle was administered to mice whose lungs were seeded with D2A1 cells. A significant reduction in macrometastases and concomitant increase in single (dormant) cells was observed in mice receiving the MLCK inhibitor, ML-7. These findings are consistent with a recent report that identified a direct correlation between levels of MLCK expression and re-occurrence of non-small cell lung cancer (35). Previous studies have demonstrated that MLCK is involved in other key aspects of tumorigenesis, including the growth of primary tumors and tumor cell motility (36, 37). MLCK has also been localized in the cleavage furrow and may also play a role in this aspect of cell division and metastatic growth. This is the first demonstration that an inhibitor of MLCK and stress fiber formation can reduce metastases in vivo by apparently maintaining single cells in a quiescent state.
Given our demonstration that the ECM could greatly effect the proliferative characteristics of the metastatic cell lines we studied, and that ECM components have repeatedly been identified in metastatic signatures of various tumor types (25, 26), we sought to determine whether fibronectin, an ECM component often upregulated in metastases (38) and present in the premetastatic niche (39) , might be functionally involved in the transition of D2A1 cells from a quiescent to proliferative state. Fibronectin is not contained in the 3D matrix we used.
Our results demonstrated that proliferation of D2A1 cells was associated with fibronectin production whereas the fibronectin receptor, integrin β1, was continuosly present in 3D culture. D2.OR cells did not produce fibronectin in 3D culture and integrin β1 expression diminished over time. Addition of fibronectin to the culture media led to a modest proliferation of D2.OR cells and accelerated proliferation of D2A1 cells in 3D culture with MLC phosphorylation and actin stress fiber formation. Importantly, we demonstrate that the proliferative outgrowth induced by fibronectin is mediated through integrin β1 and MLC phosphorylation, as either neutralizing antibody to integrin β1 or ML-7 inhibited proliferation. These results demonstrate that at least one component of the ECM, fibronectin, can regulate the proliferative outbreak of metastatic cells. However fibronectin could not promote continuous proliferation of D2.0R cells. This may be a consequence of reduced integrin β1 expression in D2.0R cells or the failure of generating a self -sustained positive feedback loop that is needed to promote continuous growth (29, 40).
Additional factors are also likely necessary to initiate and sustain the dormant to proliferative switch. Future studies will address differences in the expression and activation of other integrins that interact with the ECM, which can lead to dramatic changes in the biological behavior of cells (29, 41–45). Additionally, interactions between the tumor cells and the ECM may induce proteolytic activities of metastatic cells that substantially alter the extracellular-environment. Thus, differences in protease activities between dormant and metastaic cells may exist and can be explored in future studies.
Dormant metastatic tumor cells often evade standard adjuvant chemotherapies, which target actively proliferating cells (7). In this regard, the cellular dormancy may share biologic features with cancer stem or progenitor cells. Our results suggest that targeting pathways affecting the cytoskeleton and, specifically, actin stress fiber formation, may provide an important means of inhibiting the switch from tumor cell dormancy to clinical metastatic disease. This approach, perhaps in combination with immunotherapeutic strategies, may reduce the incidence of tumor recurrence from disseminated, dormant tumor cells.
Acknowledgments
We would like to thank Drs. Arnulfo Mendoza, Sung-Hyeok Hong, and Alexander N. Shoushtari for excellent technical support, and Drs. Glenn Merlino, Stuart Yuspa, Kent Hunter, Judah Folkman, George Naumov and Primal de Lanerolle for useful discussions. This research was supported in part by the Intramural Research Program of the National Cancer Institute and National Dental Institute, NIH, and by the Canadian Institutes of Health Research grant #42511. A.F.C. is Canada Research Chair in Oncology.
References
Full text links
Read article at publisher's site: https://doi.org/10.1158/0008-5472.can-07-6849
Read article for free, from open access legal sources, via Unpaywall:
https://aacrjournals.org/cancerres/article-pdf/68/15/6241/2594629/6241.pdf
Free to read at cancerres.aacrjournals.org
http://cancerres.aacrjournals.org/cgi/content/abstract/68/15/6241
Free after 12 months at cancerres.aacrjournals.org
http://cancerres.aacrjournals.org/cgi/content/full/68/15/6241
Free after 12 months at cancerres.aacrjournals.org
http://cancerres.aacrjournals.org/cgi/reprint/68/15/6241.pdf
Citations & impact
Impact metrics
Article citations
Loss of myosin light chain kinase induces the cellular senescence associated secretory phenotype to promote breast epithelial cell migration.
Sci Rep, 14(1):25786, 28 Oct 2024
Cited by: 0 articles | PMID: 39468273 | PMCID: PMC11519378
Natural Killer Cell Regulation of Breast Cancer Stem Cells Mediates Metastatic Dormancy.
Cancer Res, 84(20):3337-3353, 01 Oct 2024
Cited by: 0 articles | PMID: 39106452 | PMCID: PMC11474167
Deciphering genetic and nongenetic factors underlying tumour dormancy: insights from multiomics analysis of two syngeneic MRD models of melanoma and leukemia.
Biol Res, 57(1):59, 03 Sep 2024
Cited by: 0 articles | PMID: 39223638 | PMCID: PMC11370043
Advancements in Understanding the Hide-and-Seek Strategy of Hibernating Breast Cancer Cells and Their Implications in Oncology from a Broader Perspective: A Comprehensive Overview.
Curr Issues Mol Biol, 46(8):8340-8367, 01 Aug 2024
Cited by: 0 articles | PMID: 39194709 | PMCID: PMC11352233
Review Free full text in Europe PMC
Tumor Dormancy and Reactivation: The Role of Heat Shock Proteins.
Cells, 13(13):1087, 23 Jun 2024
Cited by: 0 articles | PMID: 38994941 | PMCID: PMC11240553
Review Free full text in Europe PMC
Go to all (269) article citations
Similar Articles
To arrive at the top five similar articles we use a word-weighted algorithm to compare words from the Title and Abstract of each citation.
An in vitro system to study tumor dormancy and the switch to metastatic growth.
J Vis Exp, (54):2914, 11 Aug 2011
Cited by: 24 articles | PMID: 21860375 | PMCID: PMC3211127
Metastatic growth from dormant cells induced by a col-I-enriched fibrotic environment.
Cancer Res, 70(14):5706-5716, 22 Jun 2010
Cited by: 244 articles | PMID: 20570886 | PMCID: PMC3436125
Combined SFK/MEK inhibition prevents metastatic outgrowth of dormant tumor cells.
J Clin Invest, 124(1):156-168, 09 Dec 2013
Cited by: 90 articles | PMID: 24316974 | PMCID: PMC3871237
Regulation of Metastatic Tumor Dormancy and Emerging Opportunities for Therapeutic Intervention.
Int J Mol Sci, 23(22):13931, 11 Nov 2022
Cited by: 9 articles | PMID: 36430404 | PMCID: PMC9698240
Review Free full text in Europe PMC
Funding
Funders who supported this work.
Intramural NIH HHS (1)
Grant ID: Z01 BC005740-15