Abstract
Free full text

Functional Mineralocorticoid Receptors in Human Vascular Endothelial Cells Regulate ICAM-1 Expression and Promote Leukocyte Adhesion
Abstract
In clinical trials, aldosterone antagonists decrease cardiovascular mortality and ischemia by unknown mechanisms. The steroid hormone aldosterone acts by binding to the mineralocorticoid receptor (MR), a ligand-activated transcription factor. In humans, aldosterone causes MR-dependent endothelial cell (EC) dysfunction and in animal models, aldosterone increases vascular macrophage infiltration and atherosclerosis. MR antagonists inhibit these effects without changing blood pressure, suggesting a direct role for vascular MR in EC function and atherosclerosis. Whether human vascular EC express functional MR is not known. Here we show that human coronary artery and aortic EC express MR mRNA and protein and that EC MR mediates aldosterone-dependent gene transcription. Human EC also express the enzyme 11-beta hydroxysteroid dehydrogenase-2(11βHSD2) and inhibition of 11βHSD2 in aortic EC enhances gene transactivation by cortisol, supporting that EC 11βHSD2 is functional. Furthermore, aldosterone stimulates transcription of the proatherogenic leukocyte-EC adhesion molecule Intercellular Adhesion Molecule-1(ICAM1) gene and protein expression on human coronary artery EC, an effect inhibited by the MR antagonist spironolactone and by MR knock-down with siRNA. Cell adhesion assays demonstrate that aldosterone promotes leukocyte-EC adhesion, an effect that is inhibited by spironolactone and ICAM1 blocking antibody, supporting that aldosterone induction of EC ICAM1 surface expression via MR mediates leukocyte-EC adhesion. These data show that aldosterone activates endogenous EC MR and proatherogenic gene expression in clinically important human EC. These studies describe a novel mechanism by which aldosterone may influence ischemic cardiovascular events and support a new explanation for the decrease in ischemic events in patients treated with aldosterone antagonists.
INTRODUCTION
Ischemic cardiovascular disease is the leading cause of morbidity and mortality in the developed world. In clinical trials, aldosterone antagonists improve mortality and prevent ischemic events in cardiovascular patients1,2 by unknown mechanisms. The improvement in outcomes in these trials is greater than expected from the modest decreases in blood pressure observed, suggesting the potential for a direct beneficial effect of aldosterone antagonists on the vasculature. In humans, aldosterone infusion causes endothelial vasodilator dysfunction3 and in patients with chronic heart failure, the aldosterone antagonist spironolactone improves endothelial function4,5, supporting that aldosterone has negative effects on the vascular endothelium in humans.
Aldosterone is a steroid hormone that functions by binding to the intracellular mineralocorticoid receptor (MR), a ligand-activated transcription factor and member of the nuclear hormone receptor family6. Aldosterone antagonists inhibit binding of the hormone to MR. Nuclear hormone receptors bind to specific DNA sequences in promoters of hormone-responsive genes and recruit cofactors in a ligand-dependent manner, thereby modulating gene expression(reviewed in7). In vivo MR ligands include aldosterone and cortisol, which bind to human MR with equal affinity8. Aldosterone-responsive tissues express the cortisol-inactivating enzyme 11-beta-hydroxysteroid dehydrogenase type-2(11βHSD2), which locally converts cortisol to derivatives that have a low affinity for MR9. Deficiency or mutations in 11βHSD2 in humans results in the syndrome of apparent mineralocorticoid excess with hypertension and hypokalemia(reviewed in10). MR and 11βHSD2 are expressed in the kidney where they regulate renal sodium and potassium handling, thereby maintaining and in some situations contributing to elevated systemic blood pressure6. However, evidence has been accumulating for a direct role for aldosterone and MR in the cardiovascular system, independent of renal MR actions on blood pressure. We and others have demonstrated that MR and 11βHSD2 are expressed in the heart, large vessels, and vascular smooth muscle cells (VSMC; reviewed in11). We recently demonstrated the presence of functional MR in human VSMC capable of modulating endogenous gene expression12 and that aldosterone-stimulated MR in VSMC promotes vascular calcification in vitro13, a process that is associated with increased risk of cardiovascular events in humans.
Less is known about the function of MR in vascular endothelial cells (EC). In animal studies, immunohistochemistry with anti-MR antibody has demonstrated staining of the endothelial lining of rabbit atria, aorta, and pulmonary arteries14 and MR and 11βHSD2 mRNA have respectively been identified in bovine and rat aortic EC in culture15,16. In human samples, low levels of MR mRNA have been identified in pulmonary artery EC in culture17. EC treated with aldosterone demonstrate increased oxidative stress and cell volume15,18. These data suggest that vascular EC may respond directly to aldosterone. However, whether human vascular EC express transcriptionally functional MR and whether these receptors could participate in the vascular pathophysiology of atherosclerosis is not known.
Animal models suggest that aldosterone can promote vascular inflammation. In rat models of aldosterone-induced hypertension, aldosterone caused perivascular inflammation of the coronary arteries characterized by monocyte/macrophage infiltration, increased oxidative stress, and upregulation of inflammatory genes and adhesion molecules19–21. In mouse models of atherosclerosis, aldosterone increases atherosclerotic lesion size, macrophage infiltration, and inflammatory gene expression, effects that are inhibited by aldosterone antagonists22. However, these animal models have significant systemic hypertension as well, making unclear which tissue(s) contribute to the observed atherosclerotic effects of aldosterone.
In this study, we test the hypothesis that pro-atherosclerotic effects of aldosterone are mediated in part by induction of inflammatory gene expression via MR in human vascular EC. We demonstrate that primary human EC express functional MR and 11βHSD2 enzyme and show that MR activation by aldosterone promotes ICAM1 gene transcription, ICAM1 surface protein expression, and leukocyte-EC adhesion.
MATERIALS AND METHODS
Reagents and Cell Lines
Aldosterone, spironolactone, cortisol, TNFα, Actinomycin D, and glycerrhetinic acid were used as described12 with appropriate vehicle controls. See the online Methods Supplement for cell line and culture conditions.
Quantitative Reverse Transcription-Polymerase Chain Reaction (QRT-PCR), Immunoblotting, Immunoflourescence, Adenovirus Infection, Transfection, Luciferase Assay, Flow Cytometry, and RNA Interference for MR Knock-Down
See the online Methods supplement for detailed description of the culture conditions, hormone treatments, assay protocols, controls, and normalization. For flow cytometry, the cells were labelled with PE-conjugated mouse anti-human ICAM-1 mAb or IgG1 isotype control. Mean fluorescence index (MFI) was calculated by subtracting the isotype Ig control mean fluorescence from the ICAM-1-stained mean fluorescence. Data are expressed as percentage of MFI of vehicle treated cells at each treatment time.
Leukocyte Adhesion Assay
Monolayers of hCAEC were treated as for flow cytometry, incubated with flourescent U937 human monocytic cells, and adherent fluorescent cells were counted by a blinded investigator. For Figure 6C, after treatment with hormone for 18 hours, the hCAEC were incubated for 10 minutes with anti-human ICAM1 monoclonal Ab or control IgG prior to addition of the fluorescent leukocytes. Detailed methods are provided in the online Methods Supplement.
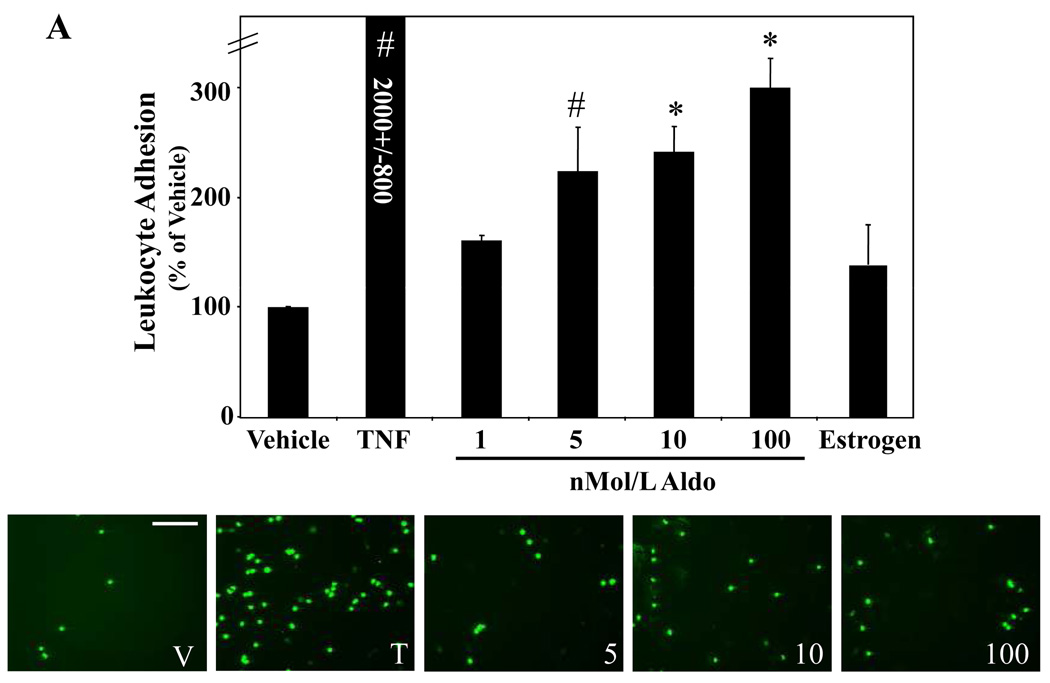
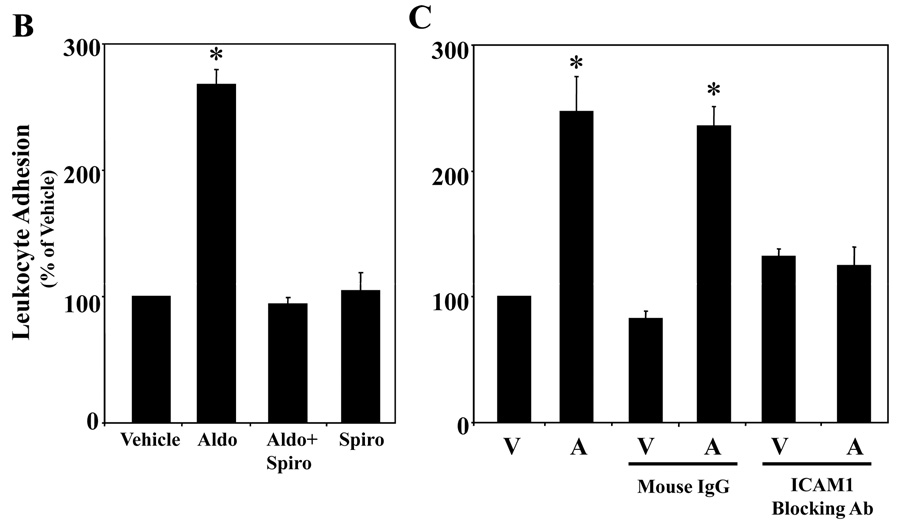
Fluorescently labeled leukocytes were incubated with hCAEC monolayers treated for 24 hours with vehicle, 5ng/ml TNF, 10nMol/L estrogen or the indicated concentrations of aldosterone and adherent leukocytes were quantified. The graphs display the number of adherent leukocytes per field expressed as the percent of vehicle treated cells. (A) Aldosterone Enhances EC-Leukocyte Adhesion. *p<0.001 vs vehicle, #p<0.05 versus vehicle. Representative fields for vehicle (V), TNFα (T) and aldosterone (5, 10, 100 nMol/L) treated EC are shown below (scale bar=100 microns). (B) Endogenous MR Mediates Aldosterone-Stimulated Leukocyte Adhesion to HCAEC. HCAEC monolayers treated for 24 hours with 100nMol/L aldosterone (Aldo) in the presence or absence of10µMol/L spironolactone (Spiro) as indicated. *p<0.001 vs Vehicle and Aldo+Spiro. (C) Aldosterone-Stimulated Leukocyte-EC Adhesion is Inhibited by ICAM1-Blocking Antibody. HCAEC were treated with vehicle (V) or aldosterone (A). Prior to addition of fluorescently labeled leukocytes, the EC were incubated with vehicle, mouse IgG, or ICAM1-blocking antibody. *p<0.001 vs vehicle, IgG, ICAM Ab, and Aldo+ICAM Ab.
Statistical Analysis
Quantitative RT-PCR, reporter assays, flow cytometry, and leukocyte adhesion assays were performed a minimum of three times. Values are reported as mean fold or percent change compared to vehicle control +/− SEM. Within-group differences were assessed with one-factor ANOVA. Post hoc comparisons were tested with the Student-Newman-Keuls test. For figure 4D, data was analyzed by one-factor ANOVA on Ranks and comparisons versus control were assessed with Dunn’s Method. P<0.05 was considered significant.
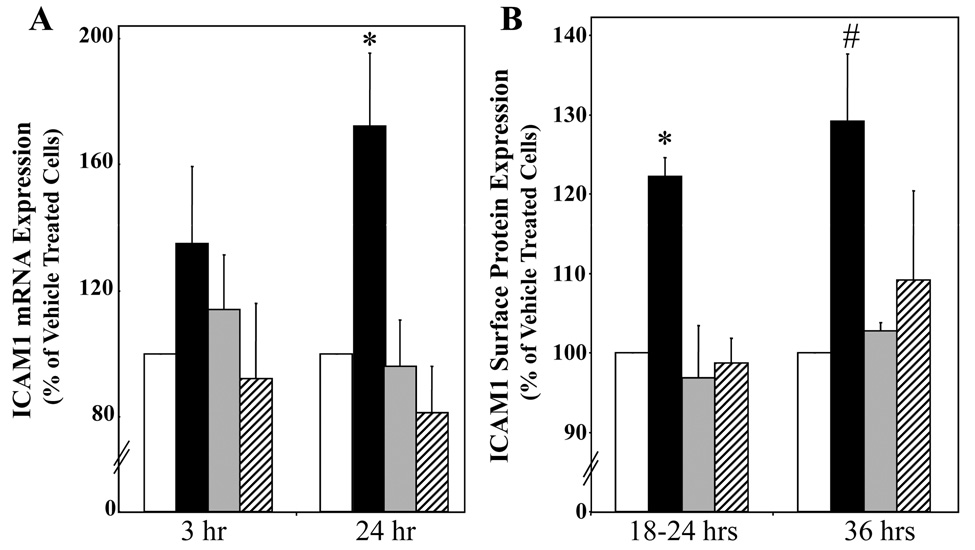
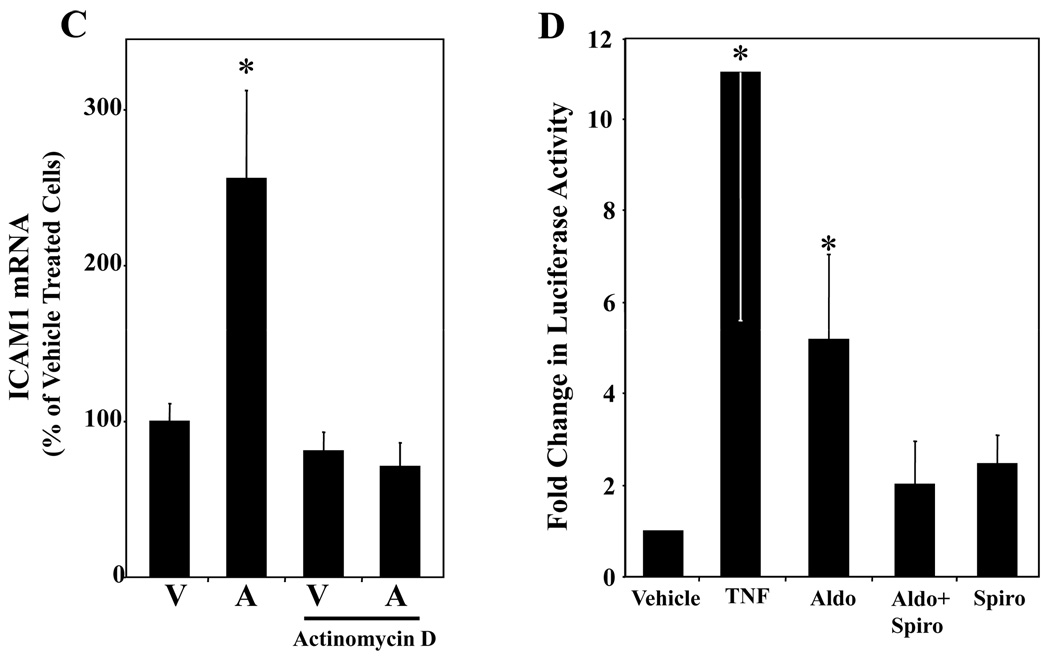
HCAEC were treated with vehicle (white), aldosterone (black), aldosterone+spironolactone (grey), or spironolactone (hatched) for the indicated times. (A) ICAM1 message is MR regulated. ICAM1 mRNA was measured by QRT-PCR and expressed as percent of vehicle treated cells at each timepoint. *p<0.05 vs 24hr vehicle and 24hr aldosterone + spironolactone. (B) ICAM1 cell surface protein expression is MR regulated. ICAM1 surface protein was measured by flow cytometry and expressed as percent of vehicle treated cells at each timepoint. *p<0.01 vs 18–24hr vehicle, 18–24hr aldosterone+spironolactone, and 18–24hr spironolactone, #p<0.05 verses 36hr vehicle. (C) Aldosterone stimulates ICAM1 gene transcription. HCAEC were treated for 24 hours with 10nMol/L aldosterone in the presence or absence of Actinomycin D (400nMol/L) and ICAM1 mRNA was measured by QRT-PCR. (D) ICAM1 Promoter Activity is MR Regulated. HCAEC were transfected with a luciferase reporter driven by the 3 kilobase ICAM1 promoter and treated for 18 hours with the indicated hormones or with TNFα(20ng/ml) as a control. Bars represent fold activation of normalized luciferase activity relative to vehicle-treated cells. *p<0.05 vs vehicle.
RESULTS
Human Vascular Endothelial Cells Express MR
The expression of MR in human vascular EC was studied first by QRT-PCR using RNA isolated from human EC (black bars) and compared to SMC (gray bars) derived for each cell type from the same individual or from the same vascular bed(Figure 1A). MR mRNA was detected in all human vascular EC examined and EC contained, on average, substantially more MR mRNA than VSMC(Figure 1). Coronary arterial EC also contained significantly less MR message than iliac and saphenous vein EC.
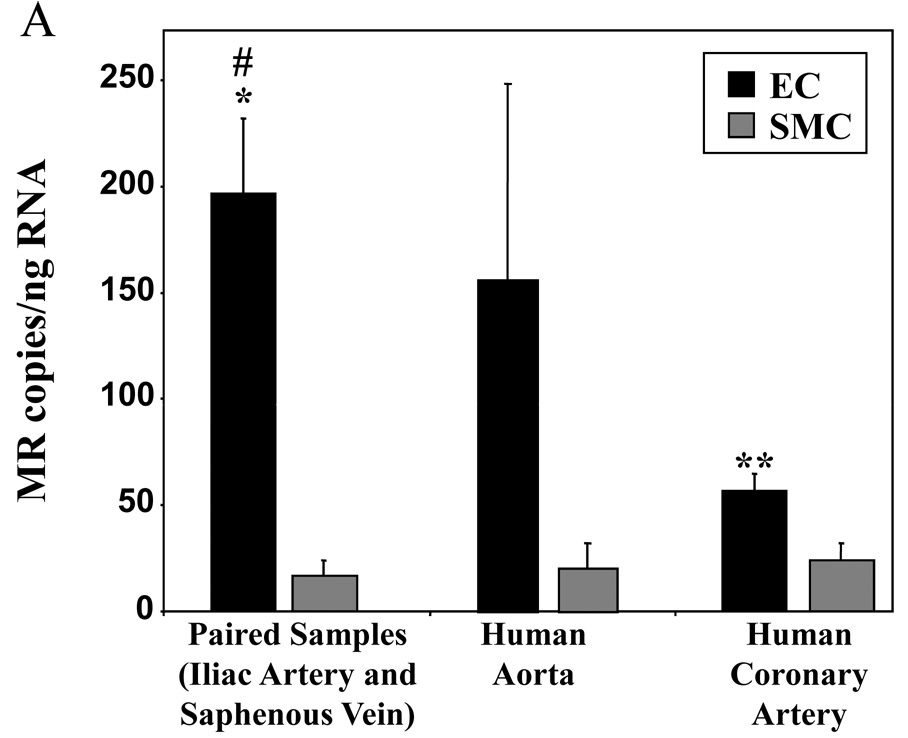
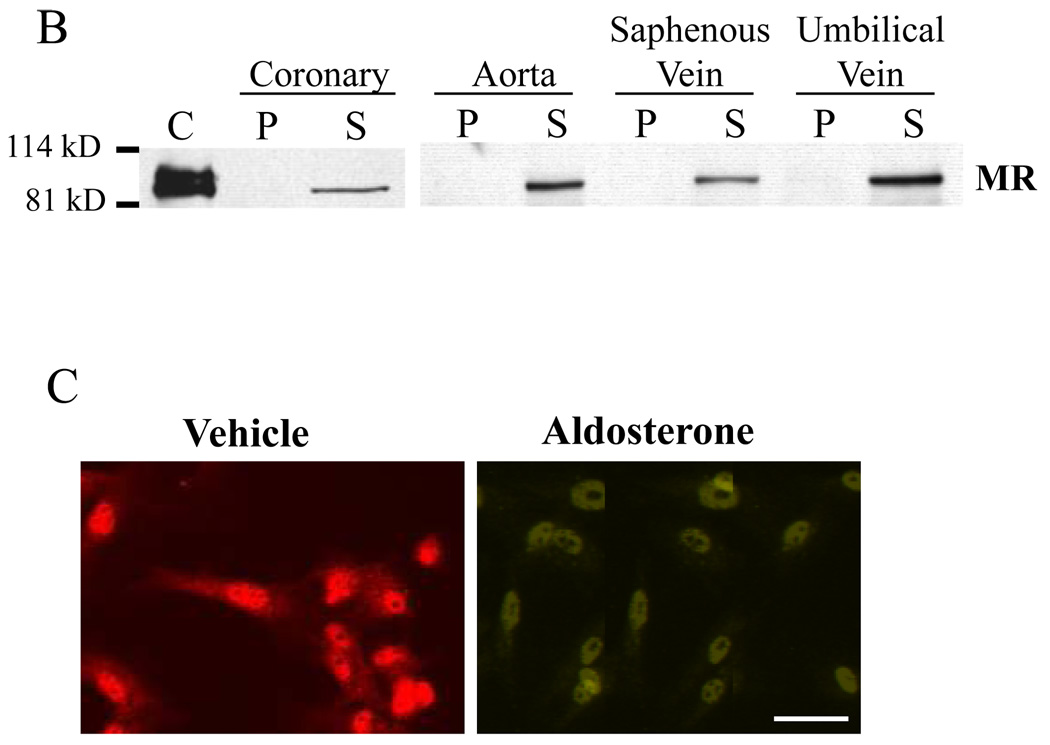
(A) QRT-PCR analysis of MR message expressed as mRNA copy number per nanogram (ng) of total RNA from human endothelial cells (EC) compared to smooth muscle cells (SMC) from the same individual (paired samples) or from the same vascular bed. *p<0.01 vs paired SMC, #p<0.01 vs coronary EC, **p<0.05 vs coronary SMC. (B) Representative immunoblot demonstrating MR protein in primary human coronary artery, aorta, saphenous vein, and umbilical vein EC. Cell lysates were separated into pellet(P) and supernatant(S) fractions along with control(C) lysate overexpressing MR and immunoblotted with anti-MR antibody. (C) Immunolocalization of MR protein in hCAEC treated with vehicle or 100nMol/L aldosterone for 18 hours as indicated (scale bar=10 microns).
The characteristic 107 kD MR protein8 also was detected in human coronary artery, aortic, saphenous vein, and umbilical vein endothelial cells(Figure 1B). MR protein was present predominantly in supernatant fractions from high salt lysates, as is characteristic for steroid hormone receptors7,12. The expression and cellular localization of MR protein in EC was also studied by immunofluorescence microscopy. In the absence of ligand, MR protein expression was detected in both the nucleus and, to a lesser extent, in the cytoplasm of human coronary artery EC(hCAEC, Figure 1C). Following exposure to aldosterone for 18 hours, MR was found almost exclusively in the nucleus(Figure 1C), as is characteristic of liganded steroid hormone receptors12,23. Pre-immune serum controls in these studies were consistently negative(data not shown). These data support that human vascular EC express MR mRNA and protein and that aldosterone-activated MR protein localizes to the nucleus of human coronary EC.
Human Vascular Endothelial Cells Express Functional Mineralocorticoid Receptor
To determine whether the endogenous EC MR is functionally capable of aldosterone-induced transcriptional transactivation, a sensitive adenoviral reporter of MR-mediated gene expression12 was infected into human aortic and coronary artery EC and MR-dependent gene expression was examined. Gene expression was activated in a dose-dependent manner beginning at 1nMol/L aldosterone in human aortic EC (hAEC) and 0.1nMol/L in hCAEC(Figure 2), consistent with both the Kd for aldosterone-MR binding8 and the physiologic concentration of aldosterone in vivo24. Aldosterone did not activate an estrogen response element-containing adenoviral reporter (data not shown) supporting that aldosterone-mediated transactivation in these studies was specific for the MR binding site. Spironolactone, the competitive inhibitor of MR hormone binding, inhibited aldosterone-mediated mineralocorticoid response element (MRE) activation in human EC, but had no effect on basal expression of the MR reporter(Figure 2). These data are consistent with studies in VSMC and in non-vascular cells showing spironolactone inhibition of MR activation at these concentrations8,12 and further support that the endogenous human aortic and coronary EC MR is a functional ligand-activated transcription factor.
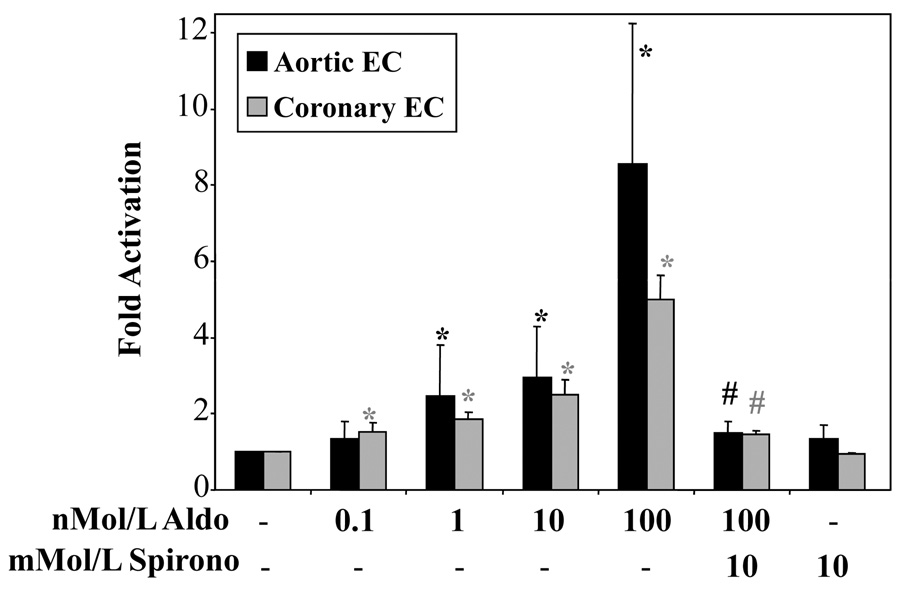
Human aortic (black) or coronary artery (grey) EC were infected with an MRE-luciferase reporter-containing adenovirus and treated with aldosterone and/or spironolactone for 18 hours as indicated. Bars represent fold-activation of luciferase activity relative to vehicle treated cells. *p<0.05 vs. MRE control; #p<0.05 verses 100nMol/L aldosterone.
Human Vascular Endothelial Cells Express Functional 11βHSD2
Aldosterone-responsive tissues express the enzyme 11βHSD2 to convert cortisol to cortisone, which is inactive on MR. Using overexpressed 11βHSD2 and human kidney 11βHSD2 as controls, immunoblotting studies confirmed that 11βHSD2 protein is expressed in human coronary artery, aorta, umbilical vein, and saphenous vein EC(Figure 3A). 11βHSD2 protein was concentrated in the pellet fractions(Figure 3A), consistent with studies in VSMC and non-vascular cells, in which 11βHSD2 enzyme is found localized to the membranous endoplasmic reticulum9,12,25. 11βHSD2 function in human aortic EC was next tested using the 11βHSD2 inhibitor glycyrrhetinic acid (GA) in the MRE-reporter assay. Doses of GA exceeding the Ki of GA for 11βHSD2 resulted in increased cortisol-stimulated MRE activation(Figure 3B) without affecting basal reporter activity, consistent with functional enzyme inactivation of cortisol in the absence of the inhibitor. Taken together, the data of Figure 1–Figure 3 demonstrate for the first time that human vascular EC contain both functional MR and 11βHSD2 and hence have the capacity to modulate gene expression in response to circulating aldosterone.
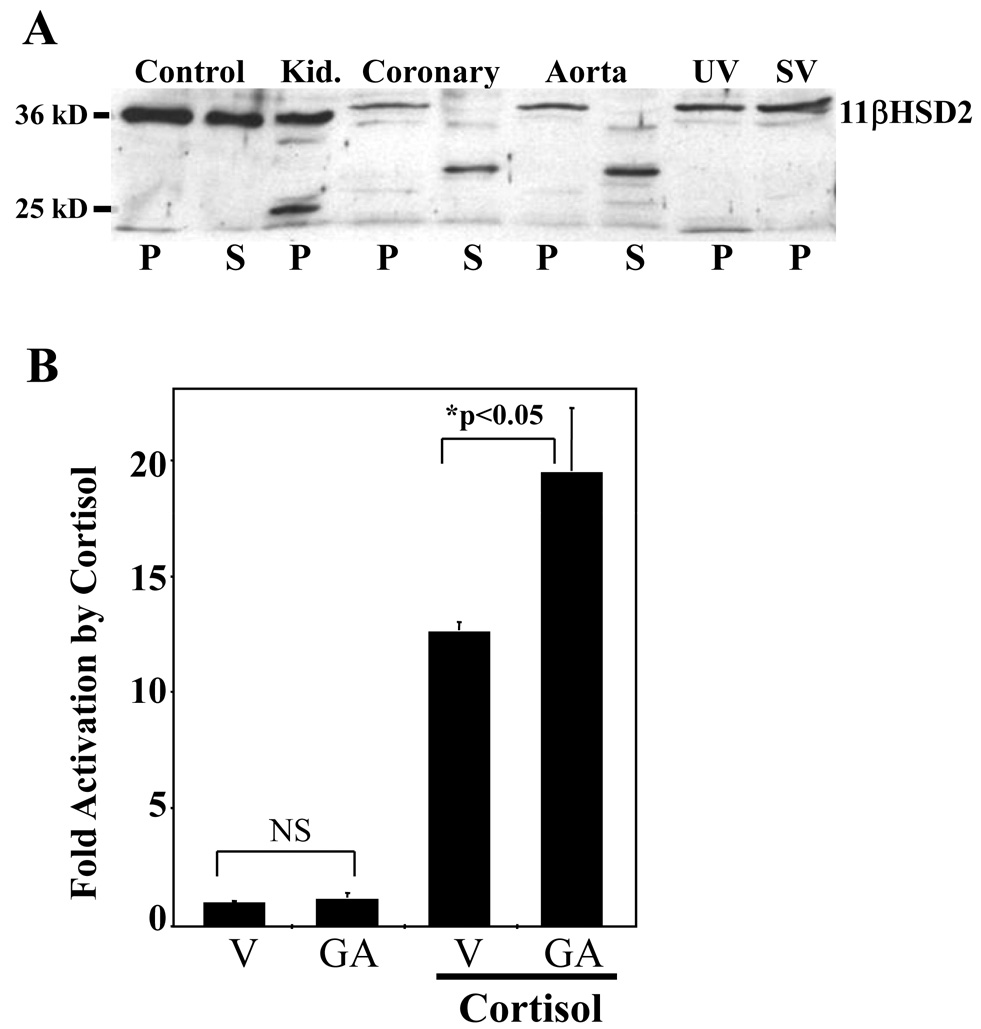
(A) Representative 11βHSD2 immunoblot demonstrating 11βHSD2 protein in primary human aorta, umbilical vein, and coronary artery EC. Cell lysates were separated into pellet (P) and supernatant (S) fractions along with cell lysates overexpressing 11βHSD2 (Control) as well as tissue lysates from human kidney(Kid.). (B) 11βHSD2 inhibition with Glycyrrhetinic acid (GA) enhances cortisol-mediated activation of MRE containing reporter. HAEC were infected with a MRE-luciferase-containing adenovirus and luciferase activity in response to vehicle or 20nMol/L cortisol was measured in the presence of vehicle (V) or 10µMol/L glycyrrhetinic acid (GA). *p<0.05 vs vehicle.
Endogenous MR-Regulated Genes in Human Coronary Artery EC
EC genes regulated by MR in hCAEC were explored next using a candidate gene approach. Animal studies support that aldosterone infusion stimulates expression of a set of specific genes involved in vascular oxidative stress and inflammation19–21,26–28. Based on these studies, we tested four candidate genes in EC for MR regulation: three NADPH oxidase (Nox) subunits; Nox126, Nox226,27, and Nox421, and the cell adhesion molecule ICAM119–21. Using QRT-PCR we tested RNA from hCAEC treated with 10nMol/L aldosterone in the presence or absence of the MR antagonist spironolactone (1µMol/L). ICAM1 and Nox4 gene expression were MR-regulated (Nox4: 120% increase after 3 hours (p<0.0001), inhibited by spironolactone (p<0.05), data not shown), while neither Nox1 nor Nox2 were regulated by MR. Aldosterone treatment for 3 hours increased ICAM1 mRNA (30%) compared to vehicle treated hCAECs (p=0.056 vs vehicle) and significantly increased ICAM1 mRNA (70–150%) after 24 hours(Figures 4A and 4C). ICAM1 has a known role in early atherosclerosis, where its expression on the surface of activated EC mediates leukocyte-EC adhesion. Next, hCAEC were treated with 10nMol/L aldosterone for 18 to 36 hours and surface ICAM1 protein was quantified by flow cytometry(Figure 4B). Aldosterone treatment increased surface ICAM1 protein 20% at 18–24 hours and 30% at 36 hours(Figure 4B). Stimulation for 12 hours with TNFα(5ng/ml), a known potent stimulator of EC inflammation, increased ICAM1 surface protein expression 405% (data not shown). The increase in ICAM1 mRNA and protein with aldosterone treatement was inhibited by the MR antagonist spironolactone and spironolactone alone had no effect on ICAM1 expression in these cells(Figures 4A and 4B). These data demonstrate that MR regulates endogenous ICAM1 message abundance and surface protein expression in hCAEC. Pretreatment of hCAEC with the transcriptional inhibitor actinomycin D completely inhibited aldosterone-stimulated ICAM1 mRNA induction(Figure 4C) demonstrating that the aldosterone effect is mediated by increasing ICAM1 gene transcription. In figure 4D, hCAEC were transfected with a luciferase reporter driven by the 3kb upstream ICAM1 promoter region that we confirm to be activated by TNFα. Aldosterone stimulated transcription from this element in an MR-dependent manner as demonstrated by inhibition by spironolactone. These data demonstrate that aldosterone binds to endogenous MR in human coronary EC thereby activating the ICAM1 upstream promoter and increasing ICAM1 gene transcription and surface protein expression.
MR Knock-Down in EC Inhibits Basal and Aldosterone-Activated ICAM1 Expression
Next, we used siRNA to knock down MR message in hCAEC and examined the effect on ICAM1 gene expression. QRT-PCR with receptor-specific primers confirmed that transfection of three unrelated MR-targeted siRNA oligos resulted in 90% knock-down of MR message 48 hours after transfection(Figure 5A) with no significant effect on expression of the highly homologous glucocorticoid receptor (GR, data not shown). MR knock-down abolished aldosterone-stimulated ICAM1 expression in hCAEC(Figure 5B) clearly implicating endogenous EC MR in the mechanism. In addition, MR knock-down resulted in 80% reduction in basal ICAM1 expression in these cells. These data are consistent with ligand-independent activation of MR by factors contained in charcoal stripped serum that tonically activate ICAM1 expression in human coronary EC.
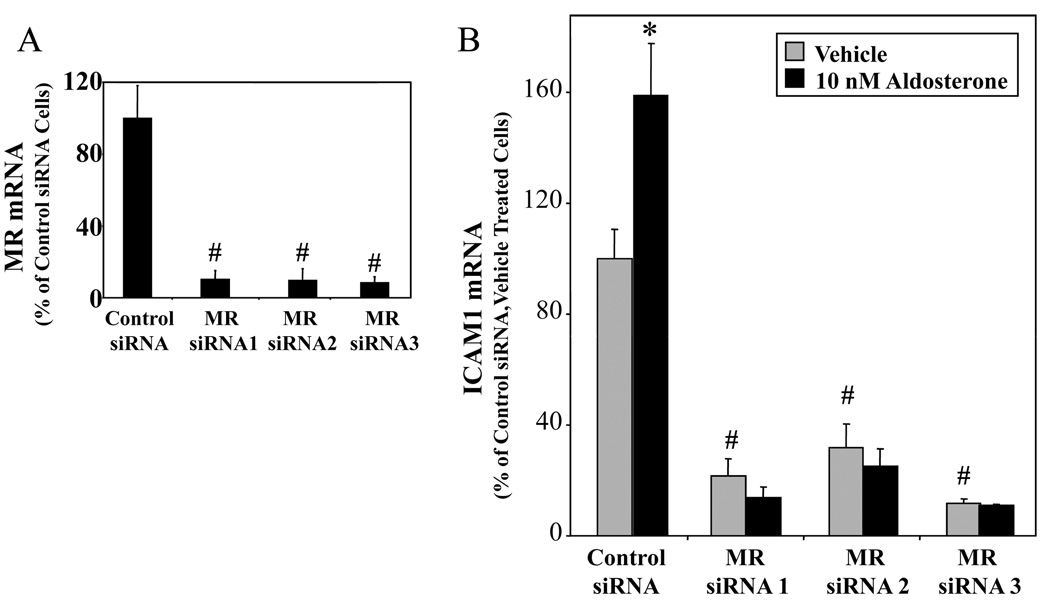
HCAEC were transfected with negative control RNAi or one of three unrelated MR-targeted siRNA oligos and treated with vehicle or 10nM aldosterone as indicated. (A) Confirmation of MR Knock-Down by QRT-PCR (expressed as percent of control oligo-transfected cells). (B) MR Knock-Down Inhibits Basal and Aldosterone-Stimulated ICAM1 Expression. ICAM1 message was quantified by QRT-PCR and expressed as percent of control oligo-transfected, vehicle-treated cells. *p<0.05 vs vehicle, # p<0.05 vs control oligo.
Effect of Aldosterone on Leukocyte Adhesion to Human Coronary Artery EC
We next investigated whether aldosterone promotes leukocyte adhesion to EC. Fluorescent labeled human monocytic cells (U937 cells) were incubated with monolayers of primary hCAEC pretreated for 24 hours with vehicle or increasing doses of aldosterone and adherent cells were quantified. Figure 6A demonstrates that at physiologically relevant concentrations, aldosterone significantly increases leukocyte-EC adhesion 2–3 fold compared to vehicle treated cells. TNFα, as a positive control, increased leukocyte adhesion 20 fold. Estrogen (17β-estradiol), a non-mineralocorticoid steroid hormone, had no effect on leukocyte adhesion suggesting that the effect is specific for aldosterone. To address the mechanism of aldosterone-stimulated leukocyte adhesion, we repeated the leukocyte adhesion assay in the presence of the MR antagonist spironolactone. Aldosterone-stimulated leukocyte adhesion to hCAEC was completely inhibited by spironolactone, while spironolactone alone had no effect(Figure 6B).
The leukocyte adhesion assay was repeated in the presence of anti-human ICAM1 antibodies capable of blocking ICAM1-mediated EC-leukocyte interactions. ICAM1 blocking antibody completely inhibited aldosterone-stimulated EC-leukocyte adhesion(Figure 6C). The same concentration of mouse IgG did not significantly alter aldosterone-mediated leukocyte adhesion supporting that this effect is specific to the ICAM1 antibody. The data in Figure 6 support that MR-mediated stimulation of ICAM1 surface protein expression in human coronary EC promotes EC-leukocyte adhesion.
DISCUSSION
The data presented here support a new mechanism for aldosterone-mediated vascular pathology. They show that human vascular EC express MR capable of sequence-specific, aldosterone-activated gene transcription at physiologic hormone levels. These endothelial cells also express the enzyme 11βHSD2 capable of inhibiting cortisol-dependent, MR-mediated gene transcription, supporting further that human vascular EC respond directly to aldosterone. Endothelial MR localizes to the nucleus following activation by aldosterone and can activate promoters containing mineralocorticoid response elements. EC MR promotes basal and aldosterone-activated ICAM1 transcription and aldosterone-activated MR enhances surface expression of ICAM1 protein and promotes leukocyte adhesion to human coronary EC. Based on these data we propose a model in which functional mineralocorticoid receptors in human vascular endothelial cells regulate ICAM1 expression and promote leukocyte adhesion(Figure 7), which provides a new mechanism for the vascular protective effects of MR antagonists. Although previous studies have demonstrated MR and 11βHSD2 expression in cardiovascular tissues in animals14,16 and in cultured human pulmonary artery EC17, our data demonstrate that functional MR also is expressed in human vascular EC from atherosclerosis-prone vascular beds and that EC MR is capable of directly modulating gene expression programs in response to aldosterone exposure. MR-mediated transcriptional changes may contribute to the long term effects of aldosterone on endothelial function that are especially relevant in patients with chronic vascular diseases like atherosclerosis and in the growing population of patients with chronically elevated aldosterone levels, including those with systolic and diastolic heart failure and low-renin hypertension.
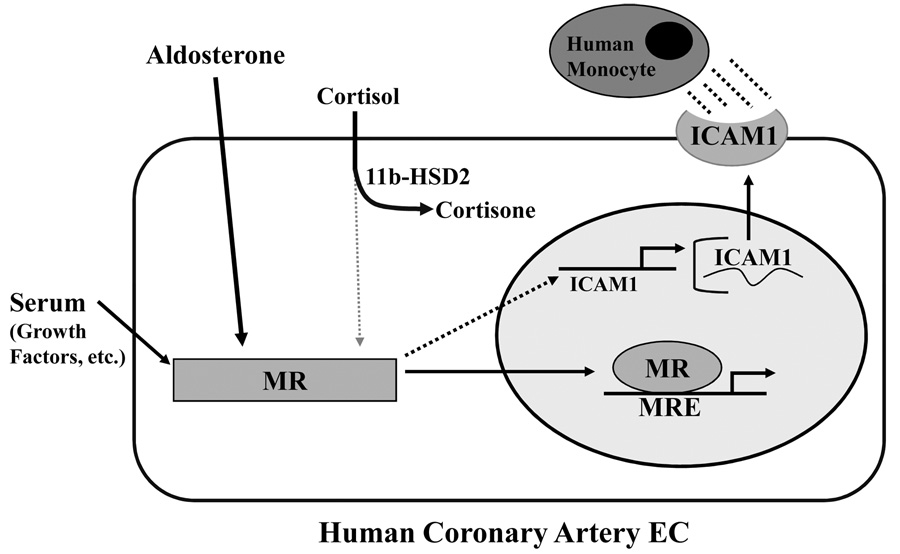
In Human coronary artery EC, mineralocorticoid receptor (MR) localizes to the nucleus and activates gene transcription from a MR response element (MRE) in response to aldosterone treatment. 11β hydroxysteroid dehydrogenase2 (11βHSD2) is expressed and inactivates cortisol in human EC. Ligand-independent activation of EC MR by serum promotes basal activation of Intercellular adhesion molecule 1 (ICAM1) expression and adosterone-activated MR stimulates ICAM1 promoter activity, mRNA transcription, and surface protein expression resulting in increased EC-monocyte adhesion, an early step in atherosclerosis.
Our data also revealed variation in the level of MR message in human coronary EC compared to the saphenous vein and iliac artery EC in the paired samples tested. Since the transcriptome of EC has been shown to vary depending on the vascular bed from which the cells originate(reviewed in 29), this may be explained by the different flow characteristics or circulating factors to which the EC in these vascular beds are exposed. Differences in MR expression may regulate variation in EC gene expression and physiology of the coronary vasculature. Alternatively, these differences may reflect biological variability in the samples studies due to factors like gender, age, race, or medical co-morbidities in the patients from which these cells were cultured. Further interpretation of differences in EC MR expression in different vascular beds will require validation in patient samples from larger cohorts.
Using a candidate gene approach, we identified Nox4 and ICAM1 as MR-regulated genes in human coronary artery EC. In animal models, aldosterone infusion increases cardiovascular ICAM1 expression19–21 however, the cause of increased ICAM expression in these in vivo models is not clear, since they also have significant systemic hypertension caused by activated kidney MR, which can result in secondary changes in vascular gene expression. In addition, the specific role of MR in the cardiomyocyte, VSMC, and EC, among others, in mediating ICAM1 gene regulation in these animal models is not clear. Our data demonstrate that MR in human EC directly regulates ICAM1 transcription independent of MR activation in the kidney or other vascular tissues, which provides a potentially important mechanism for human disease that merits further investigation. We also showed that human vascular EC express functional 11βHSD2, which may play an additional role in promoting EC-ICAM1 activation, since glucocorticoids, acting via the GR, have been shown to inhibit LPS induced ICAM1 expression and leukocyte adhesion to human umbilical vein EC30.
Though MR-activation by aldosterone stimulates ICAM1 promoter activity in human EC in our studies, in silico examination of the 3kb ICAM1 upstream promoter does not reveal an obvious MRE. There are few endogenous MR-regulated genes for which the MR-DNA binding site has been carefully studied and none in vascular EC. In the kidney, MR regulates the NaK-ATPase gene via an MRE that differs significantly from the canonical sequence31. Hence, the presence of a novel MRE in the ICAM1 promoter cannot be ruled out and remains to be tested. Alternatively, MR activation by aldosterone may regulate the expression or activity (by post-translational modification) of other transcription factors in EC that may subsequently regulate ICAM1 transcription. ICAM1 expression is tightly regulated, primarily at the level of transcription, by cell type-specific ICAM1 promoter activation mediated by multiple transcription factors binding sites including NFκB, Ets, AP1, SP1, and interferonγ responsive sites32,33. NFκB activity is increased in rats transgenic for human renin and angiotensin II (AngII) an effect that is inhibited by treatment with MR antagonists supporting that MR may activate NFκB in vivo34. In addition, aldosterone is known to increase vascular oxidative stress by a variety of mechanisms(reviewed in 35) including increased NADPH oxidase activity36 and decreased G6PD15. Reactive oxygen species have been implicated in regulating ICAM1 expression by many extracellular stimuli(reviewed in37) and we demonstrate that EC MR regulates expression of the NADPH oxidase Nox4. Thus the detailed molecular mechanisms of MR regulation of ICAM1 promoter activity are likely complex and merit further investigation.
In addition to inhibiting aldosterone-stimulated ICAM1 expression, knock-down of MR in human EC significantly decreased basal ICAM1 expression, consistent with tonic MR-mediated activation of ICAM1 expression in hCAEC. These experiments were performed in steroid-depleted serum and thus suggest that there may be some degree of basal, ligand-independent activation of MR by growth factors(Figure 7, a mechanism of activation of other steroid hormone receptors38) or AngII (which we have shown can activate MR-mediated gene transcription in human VSMC12), though this hypothesis needs to be tested further. Ligand-independent activation may be mediated by post-translational modifications such as phosphorylation, which occurs for several steroid hormone receptors, including MR, in other tissues38,39.
ICAM1 is a cell surface receptor that mediates the firm adhesion of leukocytes to EC required for transendothelial migration, an early step in atherogenesis(reviewed in 40–42). In animal models of aldosterone-induced hypertension and genetic predisposition to atherosclerosis, aldosterone promotes monocyte/macrophage accumulation in the vessel wall through unknown mechanisms20,22,43. Our data support the hypothesis that MR activation of ICAM1 expression in EC may in part mediate this effect(Figure 7) but ICAM1 activation by MR likely explains only part of the pro-inflammatory and atherogenic effects of aldosterone. Other cell adhesion molecules are important in leukocyte recruitment to atherosclerotic lesions40–42, particularly vascular cell adhesion molecule (VCAM1)44. The possibility that VCAM1 expression is also regulated by aldosterone (and that the ICAM1 blocking antibody used in Figure 6C can also inhibit VCAM1-mediated EC-leukocyte adhesion) remains to be investigated.
Animal models and clinical data support that vascular ICAM1 expression promotes atherosclerotic disease and ischemia and that aldosterone may play a role in ICAM1 regulation in humans. Genetic deficiency of ICAM1 decreases native artery and vein graft atherosclerosis in mouse atherosclerosis models45,46. In human endarterectomy specimens, EC ICAM1 expression is greater in plaques from symptomatic versus asymptomatic patients, suggesting a role for EC ICAM1 expression in plaque instability47. Serum levels of soluble ICAM1 (sICAM1) are elevated in patients at high risk for cardiovascular events and predict cardiovascular outcomes in apparently healthy subjects41. In patients with chronic, stable, congestive heart failure, serum aldosterone levels correlate with sICAM1 levels48. Treatment of cardiovascular patients with angiotensin converting enzyme inhibitors and angiotensin receptor blockers, drugs that decrease both aldosterone production and MR activation by AngII12, also decrease serum sICAM1 levels41. Thus, there is clinical data implicating ICAM1 in promoting atherosclerotic vascular disease, lending support to the possibility suggested by our data that MR regulation of ICAM1 expression in the coronary endothelium is worth exploring as a potential therapeutic target.
In summary, we have demonstrated that aldosterone activates endogenous MR and modulates gene expression of ICAM1 in human EC and promotes leukocyte adhesion to human coronary EC. This pathway may play a role in the pro-atherosclerotic effects of aldosterone and support a new explanation for the decrease in ischemic events in patients treated with aldosterone antagonists.
Acknowledgments
SOURCES OF FUNDING
This work was supported by NIH Grant HL074892 (I.Z.J.) and Italian Governmental Research Grants from the PRIN-MIUR (Progetti Ricerca Interesse Nazionale Ministero dell'Università e della Ricerca, 2005 (A.F.)) and from the Italian Ministery of Health (Ricerca Finalizzata, 2005, (G.R.)).
Footnotes
Publisher's Disclaimer: This is an un-copyedited author manuscript accepted for publication in Circulation Research, copyright The American Heart Association. This may not be duplicated or reproduced, other than for personal use or within the “Fair Use of Copyrighted Materials” (section 107, title 17, U.S. Code) without prior permission of the copyright owner, The American Heart Association. The final copyedited article, which is the version of record, can be found at <http://circres.ahajournals.org/>. The American Heart Association disclaims any responsibility or liability for errors or omissions in this version of the manuscript or in any version derived from it by the National Institutes of Health or other parties.
DISCLOSURE
None
Subject Codes: [128]ACE/Angiotension receptors; [96]Mechanism of atherosclerosis/growth factors; [143]Gene regulation; [95]Endothelium/vascular type/nitric oxide
References Cited
Full text links
Read article at publisher's site: https://doi.org/10.1161/circresaha.108.174235
Read article for free, from open access legal sources, via Unpaywall:
https://www.ahajournals.org/doi/pdf/10.1161/CIRCRESAHA.108.174235
Free to read at intl-circres.ahajournals.org
http://intl-circres.ahajournals.org/cgi/content/abstract/102/11/1359
Free after 12 months at intl-circres.ahajournals.org
http://intl-circres.ahajournals.org/cgi/content/full/102/11/1359
Free after 12 months at intl-circres.ahajournals.org
http://intl-circres.ahajournals.org/cgi/reprint/102/11/1359.pdf
Citations & impact
Impact metrics
Citations of article over time
Article citations
Mineralocorticoid receptor antagonists and heart failure with preserved ejection fraction: current understanding and future prospects.
Heart Fail Rev, 17 Oct 2024
Cited by: 0 articles | PMID: 39414721
Review
The mineralocorticoid receptor in diabetic kidney disease.
Am J Physiol Renal Physiol, 327(3):F519-F531, 18 Jul 2024
Cited by: 0 articles | PMID: 39024357
Review
The role of cardiac microenvironment in cardiovascular diseases: implications for therapy.
Hum Cell, 37(3):607-624, 18 Mar 2024
Cited by: 1 article | PMID: 38498133
Review
BAP31 Promotes Adhesion Between Endothelial Cells and Macrophages Through the NF-κB Signaling Pathway in Sepsis.
J Inflamm Res, 17:1267-1279, 26 Feb 2024
Cited by: 0 articles | PMID: 38434584 | PMCID: PMC10906674
First Evidence of Mineralocorticoid Receptor Gene and Protein Expression in Rat and Human Thyroid Tissues and Cell Cultures.
Int J Mol Sci, 25(2):754, 06 Jan 2024
Cited by: 1 article | PMID: 38255827 | PMCID: PMC10815259
Go to all (170) article citations
Data
Data behind the article
This data has been text mined from the article, or deposited into data resources.
BioStudies: supplemental material and supporting data
Similar Articles
To arrive at the top five similar articles we use a word-weighted algorithm to compare words from the Title and Abstract of each citation.
Angiotensin II and aldosterone regulate gene transcription via functional mineralocortocoid receptors in human coronary artery smooth muscle cells.
Circ Res, 96(6):643-650, 17 Feb 2005
Cited by: 230 articles | PMID: 15718497
Endothelial Mineralocorticoid Receptors Contribute to Vascular Inflammation in Atherosclerosis in a Sex-Specific Manner.
Arterioscler Thromb Vasc Biol, 39(8):1588-1601, 11 Jul 2019
Cited by: 36 articles | PMID: 31294624 | PMCID: PMC6656626
Myeloid Mineralocorticoid Receptor Transcriptionally Regulates P-Selectin Glycoprotein Ligand-1 and Promotes Monocyte Trafficking and Atherosclerosis.
Arterioscler Thromb Vasc Biol, 41(11):2740-2755, 07 Oct 2021
Cited by: 9 articles | PMID: 34615372 | PMCID: PMC8601161
Molecular mechanisms of mineralocorticoid receptor antagonism by eplerenone.
Mini Rev Med Chem, 5(8):709-718, 01 Aug 2005
Cited by: 18 articles | PMID: 16101407
Review
Funding
Funders who supported this work.
NHLBI NIH HHS (7)
Grant ID: K08 HL074892-04
Grant ID: HL074892
Grant ID: K08 HL074892
Grant ID: K08 HL074892-01A2
Grant ID: K08 HL074892-03
Grant ID: K08 HL074892-02
Grant ID: R01 HL095590