Abstract
Free full text

Blood-derived inflammatory dendritic cells in lymph nodes stimulate acute TH1 immune responses
Abstract
TH1-polarized immune responses, which confer protection against intracellular pathogens, are thought to be initiated by dendritic cells (DCs) that enter lymph nodes from peripheral tissues. We found following viral infection or immunization, inflammatory monocytes were recruited into lymph nodes directly from the blood to become CD11c+CD11bhiGr-1+ inflammatory DCs, which produced abundant interleukin 12(p70) and potently stimulated TH1 responses. This monocyte extravasation required CCR2 but not CCL2 or CCR7. Thus, inflammatory DC accumulation and TH1 responses were markedly reduced in Ccr2−/−mice, preserved in Ccl2−/− mice, and relatively increased in CCL19-CCL21-Ser-deficient plt mutant mice, in which all other lymph node DC types were reduced. We conclude that blood-derived inflammatory DCs play a major role in the development of TH1 immune responses.
Immune responses are initiated in lymph nodes when antigen-bearing dendritic cells (DCs) stimulate the activation and polarization of T cells1,2. In the classic view, T cell activation is stimulated primarily by migratory DC populations, such as Langerhans cells, that enter lymph nodes from the periphery via lymphatics, and most DC subtypes behave like Langerhans cells1,2. This view has changed in recent years with the findings that some lymph node DC subtypes arise from precursors recruited directly from the blood or only during inflammation and that, in many cases, migratory DC types do not directly activate T cells in vivo3–5. These findings have raised questions about which lymph node DC subtypes actually stimulate T cell activation and polarization after various immunogenic stimuli.
TH1-polarized T cells produce interferon-γ (IFN-γ) and provide protection against viruses and other intracellular pathogens. TH1 T cell polarization is stimulated most strongly by interleukin 12 (IL-12), which is produced by monocytes, macrophages, and certain DC subtypes upon activation6. Bioactive IL-12, IL-12(p70), is composed of two subunits: IL-12(p40), which is produced in fairly high amounts and is shared with IL-23, and IL-12(p35), whose production is more tightly regulated and represents the rate-limiting step in IL-12(p70) formation7. Among DC subtypes obtained from resting lymphoid organs, resident CD8+ DCs have the greatest TH1-polarizing activity8,9. However, this may not be true during infection or inflammation. During chronic cutaneous leishmania infection, peripheral ‘inflammatory’ DCs (derived from inflammatory Gr-1+ monocytes that enter peripheral tissues, become DCs, and migrate to lymph nodes via lymphatics) accumulate in large numbers and display robust TH1-polarizing activity10,11. Consistent with the idea that monocyte-derived DCs (moDCs) stimulate TH1 responses, these responses are markedly impaired in mice lacking the chemokine receptor that mediates inflammatory monocyte migration, CCR 2 http://www.signaling-gateway.org/molecule/query?afcsid=A000625 (refs. 12–14). This abnormality has been proposed to be due to a defect in the trafficking of monocytes to sites of immunization or infection13. However, this view is not consistent with the finding that mice lacking the major CCR2 ligand, CCL2 (also known as monocyte chemoattractant protein 1, MCP-1, http://www.signaling-gateway.org/molecule/query?afcsid=A001484), have normal or increased TH1 responses15,16. The cause of the divergent T cell responses in Ccr2−/− and Ccl2−/− mice has not been determined17.
Inflammatory DCs in lymph nodes can arise from moDCs recruited via lymphatics (peripheral moDCs) and from inflammatory monocytes that enter lymph nodes directly from the blood (blood-derived inflammatory DCs)10,11,18,19. Although inflammatory monocytes have been demonstrated to extravasate across high endothelial venules (HEVs) into lymph nodes and develop into DCs, the role that blood-derived inflammatory DCs may play in antigen-specific T cell responses has not been determined18. Here, we identify a population of CD11c+CD11bhiGr-1+ inflammatory DCs that was the major source of both IL-12(p70) production and T cell IFN-γ stimulatory activity in lymph nodes after immunization or viral infection. These inflammatory DCs arose from monocytes that entered inflamed lymph nodes directly from the blood and their accumulation in lymph nodes required CCR2, but not CCL2 or CCR7 http://www.signaling-gateway.org/molecule/query?afcsid=A000630. Inflammatory DCs were the only DC type to accumulate in the lymph nodes of mice with the paucity of lymph node T cell (plt) mutation, a deletion of the CCR7 ligand genes Ccl19 and Ccl21-Ser, which results in a loss of all CCL19 expression and CCL21 expression in secondary lymphoid organs20, leading to increased TH1 responses in these mice after immunization. Absence of inflammatory DC accumulation accounted for the markedly decreased TH1 responses seen in CCR2-deficient, but not CCL2-deficient, mice.
Results
Increased T cell responses in lymph nodes of plt mice
We identified lymph node moDCs in the course of examining immune response abnormalities in plt mice. Plt mice display a >95% reduction in the migration of naive T cells into lymph nodes and a 50–60% decrease in total lymph node DCs21,22. However, despite these defects, plt mice display increased antigen-specific T cell numbers and increased antigen-specific T cell proliferation for at least 16 months after immunization23,24. On both BALB/c (Fig. 1a) and C57BL/6 (Fig. 1b) genetic backgrounds, plt mice displayed delayed-type hypersensitivity (DTH) responses that are about 2-fold greater than seen in wild-type mice. This increased response was independent of whether Complete Freund’s adjuvant (CFA), monophosphoryl lipid A-trehalose dicorynomycolate (RIBI), or lipopolysaccharide (LPS) was used as the adjuvant for sensitization and of the number of sensitizing immunizations (Fig. 1c and data not shown). Plt mice also displayed contact hypersensitivity (CHS) responses that were markedly increased by day 7 and were maintained without reduction for at least eight weeks (Fig. 1d). After immunization with ovalbumin (OVA) in CFA, plt mice displayed a 6-fold increase in the proportion of CD4+ T cells that produced IFN-γ (Fig. 1e, f), indicating the development of markedly increased TH1 T cell responses. These increased T cell responses were not due to an intrinsic defect in T cells or DCs. After reciprocal bone marrow transplantations between wild-type and plt mice, the plt phenotype of increased DTH responses was seen in plt recipients, irrespective of the bone marrow donor genotype (Fig. 1g). In our hands, bone marrow transplantation typically leads to some reduction in DTH response relative to non-transplanted mice (data not shown). Like plt mice, CCR7-deficient mice display severe defects in the accumulation of T cells and DCs in draining lymph nodes25, but display increased T cell responses in contact hypersensitivity assays26. The cause of the paradoxically increased T cell responses seen in plt and CCR7-deficient mice has not been previously determined.
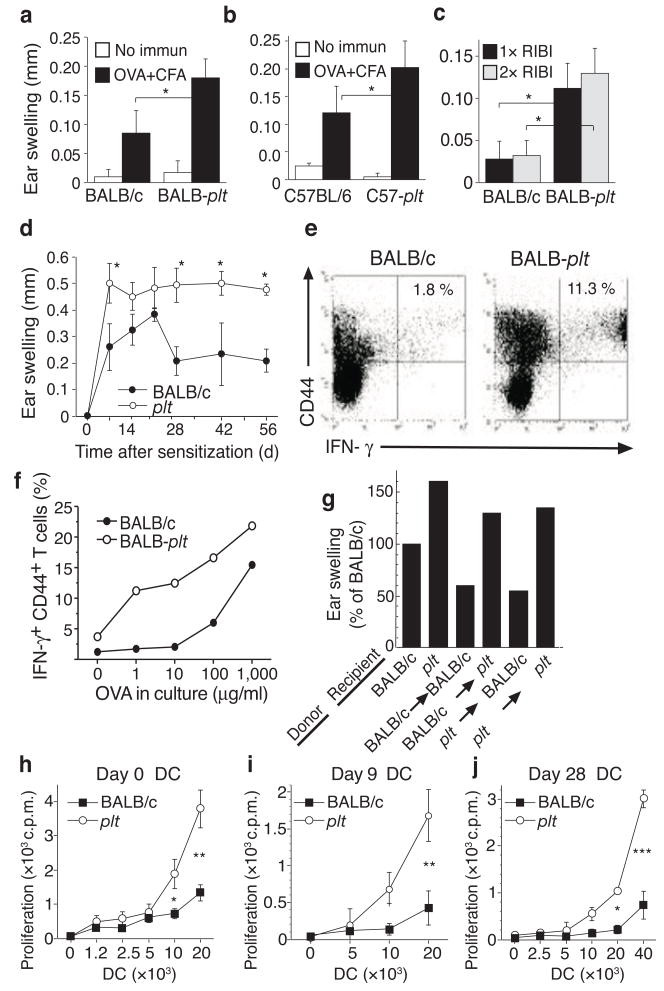
Increased immune responses in plt mice. (a–c) DTH responses in plt mice. WT and plt mice were sensitized with OVA/CFA (a,b) or OVA/RIBI x1 or x2 (c). Responses were elicited after 7 days and ear swelling measured at 24 h. (d) Time course of contact hypersensitivity (CHS) responses. BALB/c and plt mice were sensitized by oxazolone skin painting and ear swelling measured 24 h after elicitation. Data points represent mean ± SD for 5 mice (a–d). (e) IFN-γ intracellular staining of BALB/c and plt lymph node CD4+ T cells 9 days after immunization with OVA/CFA. Numbers indicate percentage of CD44+IFN-γ+ cells among CD4+ T cells. (f) Percentage of CD44+IFN-γ+ cells among CD4+ T cells after restimulation with increasing concentrations of OVA. (g) DTH responses, performed as above, after reciprocal bone marrow transplantation between BALB/c and BALB/c-plt mice. Bars represent mean ear swelling as a percentage of the responses in BALB/c mice. n = 5 mice/group, P<0.02 for BALB/c vs. plt recipients. (h) Allogeneic T cell proliferation induced by lymph node CD11c+ cells from untreated mice. (i,j) OVA-specific proliferation of a DO11.10 CD4 T cell line induced by total lymph node DCs 9 days (i) or 28 days (j) after immunization with OVA/CFA. Points represent mean ± SD for 3 mice (h j). * P < 0.05; ** P < 0.005; *** P < 0.0005 by Student’s t-test.
At baseline, T cells in plt lymph nodes are markedly reduced in number and are predominantly of memory phenotype, but display no obvious abnormalities in response to antigen stimulation21,23. The frequency of CD25+ T regulatory cells among total T cells in plt lymph nodes was normal at baseline and after immunization (data not shown). Thus, T cells in plt mice appear to be normal. In contrast, total CD11c+ DCs in the lymph nodes of naive plt mice displayed an increased capacity to stimulate the proliferation of allogeneic naive CD4+ T cells (Fig. 1h). Nine days after immunization, a time when T cell proliferative responses in plt mice are increased23, total lymph node DCs from plt mice, bearing only the antigen administered 9 days earlier, stimulated the robust proliferation of cells from an OVA-primed DO11.10 CD4+ T cell line, while DCs obtained from wild-type lymph nodes displayed no such ability (Fig. 1i). This increased ability to stimulate OVA-experienced T cells persisted for at least 28 days after immunization (Fig. 1j). These results suggest that the increased and prolonged T cell responses seen in plt mice are due, at least in part, to an increased and prolonged activity among lymph node DCs.
Alterations in DC subset composition in plt lymph nodes
To determine if the increased activity of total lymph node DCs in plt mice was due to alterations in the frequency of individual lymph node DC subtypes, we used the gating protocol shown in Fig. 2a to examine the frequency of six individual DC subtypes in plt popliteal lymph nodes at baseline and after footpad immunization. Five of these DC subtypes correspond to well-described lymph node DC populations27. These include CD11b−B220+ plasmacytoid DCs (pDCs); CD11chiCD40int cells (gate I), which include CD11b+CD8−DCs and CD11bintCD8+ (CD8+) DCs; CD11cintCD40hi dermal-derived DCs (gate II); and CD11chiCD40hi Langerhans cells (gate III). We also found a CD11cintCD11bhiCD40int DC population (gate IV) that displayed the phenotype described for moDCs11,28–30. These CD11cintCD40int cells were CD11bhi, CD80+, CD86+, major histocompatibility complex (MHC) class II+, CD4+, CD8−and expressed no CCR7 (Fig. 2a and Supplementary Fig. 1 online). Such cells were present in small numbers in resting wild-type lymph nodes but were difficult to discern among other cell types. The CD11cintCD40int cells were ablated in immunized CD11c-diphtheria toxin receptor transgenic mice after diphtheria toxin treatment, confirming their classification as DCs (data not shown).
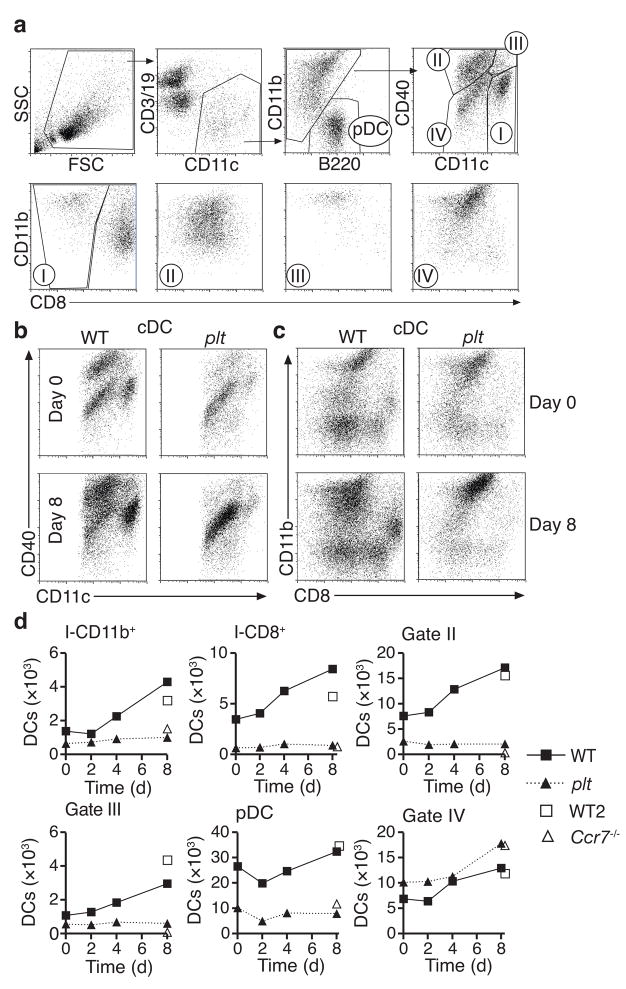
Quantification of individual lymph node DC subtypes in BALB/c and plt mice. (a) Gating protocol for identification of lymph node DC subsets. Subtypes indicated include CD11b− B220+ plasmacytoid DCs (pDC), CD11chiCD40int DCs (I), CD11cintCD40hi dermal DCs (II), CD11chiCD40hi Langerhans-derived DCs (III), and CD11cintCD40int inflammatory DCs (IV). DCs in gate I are further divided into CD11bhiCD8− and CD8+ DCs. (b) CD40 vs. CD11c, and (c) CD11b vs. CD8 flow profiles of BALB/c and plt lymph node CD11c+CD11b+B220− DCs (cDC) at baseline and 8 days after immunization with OVA/CFA. (d) Quantification of individual DC types in BALB/c, plt, and Ccr7−/− draining lymph nodes on the indicated days after footpad immunization with 20 μg OVA/CFA based on the gates shown in (a). Points represent the cell number per lymph node in samples pooled from 3 mice. Data is representative of 3 separate studies.
Resting lymph nodes in plt mice displayed a 45% decrease in total CD11c+ cells, but this decrease was not uniform among DC subtypes (Fig. 2b,c). Numbers of CD11cintCD40int DCs were normal in resting plt lymph nodes while the absolute number of all other DC subtypes was markedly (55%–81%) reduced (Fig. 2b,d). Following subcutaneous immunization with OVA in CFA, numbers of CD11cintCD40int DCs in plt lymph nodes increased normally, while all other DC subtypes displayed almost no change in number over the 8 days following immunization (Fig. 2d). By day 8, all DC subtypes in plt mice other than CD11cint CD11bhi CD40int DCs were reduced by at least 75% relative to wild-type mice (Fig. 2b,c), resulting in a markedly increased ratio of CD11cintCD11bhiCD40int DC to other DC subtypes (1:5 in wild-type vs. 2:1 in plt). Like plt mice, Ccr7−/− mice displayed normal numbers of CD11cintCD11bhiCD40int DCs eight days after immunization, but marked reductions in all other DC subtypes (Fig. 2d, open triangles). Ccr7−/− mice displayed a greater reduction in migratory DC types (gates II and III) than plt mice, consistent with their more complete loss of lymphatic CCR7 activity. These findings are consistent with prior studies31.
Because moDCs express the glycosyl phosphatidylinositol-anchored monocyte differentiation antigen Ly-6C for several days after their differentiation from inflammatory monocytes, they can be identified by staining with anti-Gr-1, which recognizes Ly-6C and Ly-6G30. We identified Gr-1+ DCs in lymph nodes as a subset of CD11cintCD11bhiCD40int (region IV) DCs. Gr-1 proved to be more specific than CD40 in identifying moDCs as a distinct population and was therefore used in all subsequent experiments. In wild-type mice, CD11bhiGr-1+ DC were rare in lymph nodes at baseline but accumulated after immunization (Fig. 3a). In plt mice, CD11bhiGr-1+ DCs were abundant in lymph nodes at baseline and were markedly increased as a proportion of total lymph node DCs after immunization (Fig. 3a). The absolute number CD11bhiGr-1+ DCs in lymph nodes was normal in plt mice at baseline and after immunization (Fig. 3b). CD11bhiGr-1+ DCs in plt lymph nodes were CD40int, CD80+, CD86+, and MHC class II+ (Supplementary Fig. 2 online). To ensure that the rapid accumulation of CD11bhiGr-1+ DCs in lymph nodes was not a specific response to CFA immunization, we examined another model of inflammation, acute viral infection. Five days after influenza virus infection, the frequency of CD11bhiGr-1+ DCs increased 7-fold in the mediastinal lymph nodes of wild-type mice (Fig. 3c). In plt mice, the frequency of CD11bhiGr-1+ DCs was increased at baseline and markedly increased after viral infection (Fig. 3c). The absolute number of CD11bhiGr-1+ DCs in plt mediastinal lymph nodes was similar to that seen in wild-type mice after viral infection (Fig. 3d).
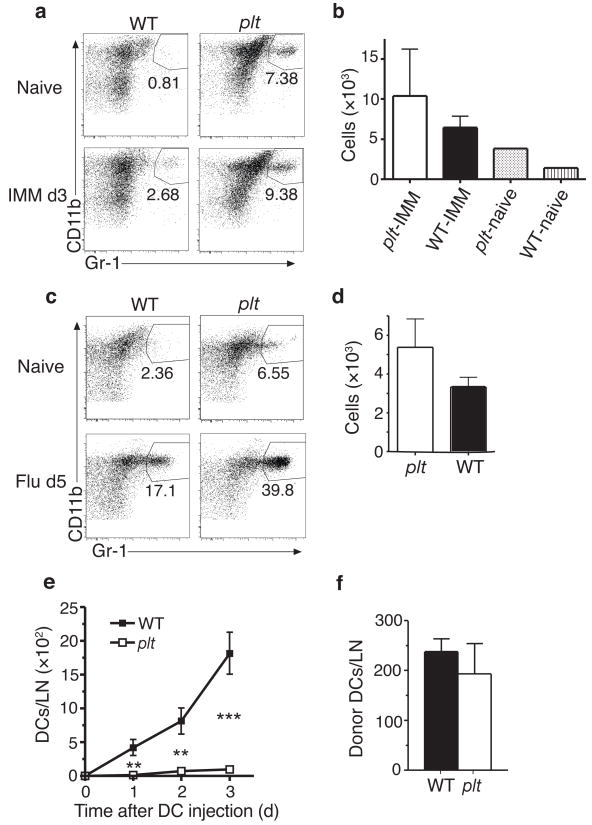
Accumulation of inflammatory DCs in the draining lymph nodes of plt and WT mice. (a,b) CD11bhiGr-1+ DCs in BALB/c and plt lymph nodes at baseline and 3 days after immunization with 100 μg OVA/CFA. (a) CD11b vs. Gr-1 flow profiles of CD11c+CD11b+B220−CD8− lymph node cells. (b) Mean number ± SD of CD11bhiGr-1+ DCs per 4 lymph nodes 3 days after immunization. n = 3 mice, P > 0.05. (c,d) CD11bhiGr-1+ DCs in BALB/c and plt mediastinal lymph nodes at baseline and 5 days after influenza infection. (c) CD11b vs. Gr-1 flow profiles as in (a). (d) Mean number ± SD of CD11bhiGr-1+ DCs per mediastinal lymph node. n = 3 mice, P > 0.05. (e) Time course of donor BM-derived DC accumulation in the popliteal lymph nodes of WT and plt mice. DCs were injected into footpads 3 days after injection of CFA. n = 3–4 mice. (f) Accumulation of donor moDCs (CD45.1+CD11c+CD11b+CD86+) in the draining lymph nodes of wild-type and plt mice 3 days after monocyte transfer. Monocytes were injected by tail vein 3 days after immunization of OVA/CFA. n = 3 mice, *P < 0.05; ** P < 0.005; *** P < 0.0005 by Student’s t-test.
Because DC migration through lymphatics requires CCR7 activity32, the preserved CD11bhiGr-1+ DC accumulation in plt and Ccr7−/− mice suggests that these cells enter lymph nodes directly from the blood as inflammatory monocytes. To determine whether DCs were capable of entering inflamed plt lymph nodes via lymphatics, we injected bone marrow-derived DCs into the footpads of wild-type and plt mice. Injected DCs accumulated in the draining popliteal lymph nodes of wild-type mice but not plt mice (Fig. 3e), a finding consistent with previous reports10. In contrast, intravenous injection of bone marrow monocytes resulted in a roughly equal accumulation of donor CD11c+CD11bhiCD86+ DCs in the lymph nodes of wild-type and plt mice after 3 days (Fig. 3f). These results suggest that the inflammatory DCs in plt lymph nodes are blood-derived and do not enter lymph nodes via lymphatics.
TH1 stimulatory activity of inflammatory DCs
The finding that CD11bhiGr-1+ DCs were increased in relative frequency in plt mice suggests that these cells may stimulate TH1 polarization. To test this notion, we purified CD11bhiGr-1+ and CD8+ DCs from the mediastinal lymph nodes of influenza-infected C57BL/6 mice and used these cells to stimulate OVA-specific OT2 T cells in vitro. CD8+ DCs were chosen for comparison because they have been previously described as the most potent TH1-stimulatory DC subtype8,9. CD11bhiGr-1+ DCs induced strikingly high amounts of T cell-generated IFN-γ relative to both CD8+ DCs purified from the same lymph nodes and total DCs purified from the spleens of naive mice (Fig. 4a). This difference in IFN-γ production was not due to differences in overall T cell proliferation (Fig. 4b) or T cell survival (data not shown). To determine why lymph node CD11bhiGr-1+ DCs had such potent IFN-γ stimulatory activity, we examined the production of IL-12 by lymph node DC subtypes. Individual mediastinal lymph node DC subtypes were purified by flow cytometry 4 days after influenza infection and stimulated in vitro with LPS and anti-CD40. Under these conditions, CD11bhiGr-1+ DCs, CD8+ DCs, and naive splenic DCs all produced significant amounts of the IL-12 subunit, p40 (Fig. 4c). However, only CD11bhiGr-1+ DCs produce detectable amounts of complete IL-12(p70) (Fig. 4d).
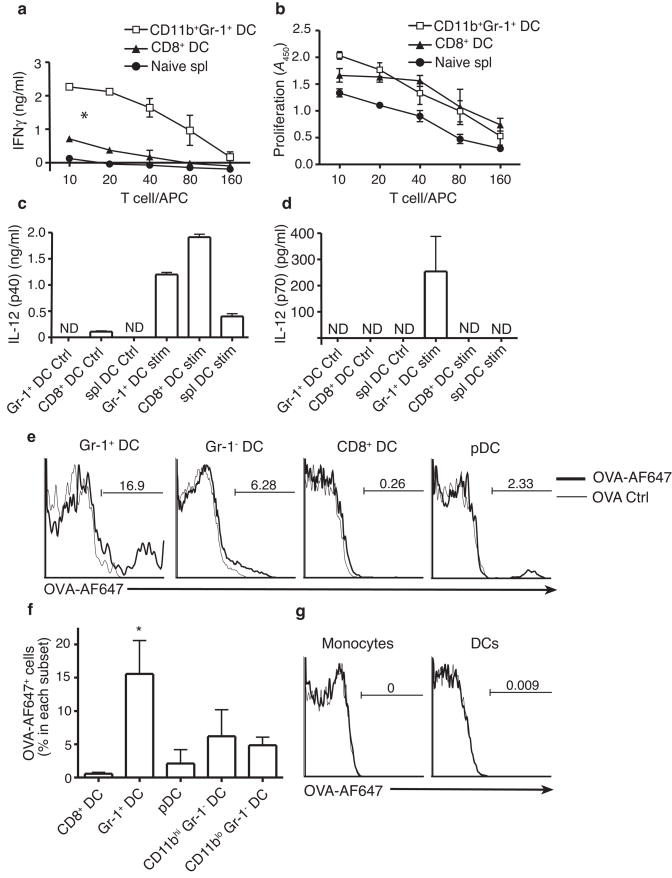
Activities of CD11bhiGr-1+ DCs. (a,b) CD8+ DCs and CD11bhiGr-1+ DCs, purified from the mediastinal lymph nodes of C57BL/6 mice 4 days after influenza infection, and total naive splenic DC were used to stimulate naive OT2 CD4+ T cells in the presence of OVA peptide. Significant differences in slopes of response curves were calculated by ANOVA. *p<0.01 for Gr-1+ DC vs. all other DC types. (a) IFN-γ production by stimulated OT2 cells as measured by ELISA. (b) Proliferation of stimulated OT2 cells as measured by BrdU incorporation in a colorimetric assay. (c,d) IL-12 production by DC subtypes. 2 × 104 CD8+, CD11bhiGr-1+, or splenic DCs were purified as above and cultured in media alone (Ctrl) or with LPS and αCD40 (Stim). After 24 h, IL-12p40 (c) and IL-12p70 (d) concentrations in the supernatants were measured by ELISA. (a–d) All data points indicate mean ± SD for 3 experiments, each using cells pooled from 5 to 7 mice. (e–g) Wild-type mice were immunized with OVA+CFA or OVA-AF647+CFA in the back. Brachial lymph nodes and blood were harvested 3 days later for flow analysis. n = 3 mice. (e) Flow histogram of OVA-AF647 for each lymph node DC subset. (f) Mean percentage of OVA-AF647+ cells in each DC subset. * P < 0.05 vs. all other DC types by ANOVA. (g) Histogram of OVA-AF647 accumulation in blood monocytes and DCs.
To determine the extent to which CD11bhiGr-1+ DC actually captured antigen in vivo, we immunized mice subcutaneously with OVA conjugated to Alexafluor-647 (AF-647) in CFA and examined the percentage of each DC subtype in lymph nodes that were AF-647+. Surprisingly, 3 days post immunization, the percentage of CD11bhiGr-1+ DCs that contained labeled antigen was much higher than any other lymph node DC subtype (Fig. 4e,f). In addition, CD11bhiGr-1+ DCs appeared to capture more antigen on a per cell basis than other lymph node DC types, based on the fluorescent intensity of AF-647+ cells (Fig. 4e). AF-647 staining was not detected on either monocytes or DCs in the blood of immunized mice (Fig. 4g), suggesting that antigen capture by CD11bhiGr-1+ DC occurred within the lymph node.
moDC accumulation requires CCR2 but not CCL2
Based on the above findings, we concluded that monocytes enter inflamed lymph nodes directly from the blood, that they give rise to inflammatory DCs with potent TH1 stimulatory activity, and that the entry of these cells into lymph node was CCR7-independent. Because inflammatory monocyte migration is typically CCR2-dependent, we recognized that, if correct, this model would provide an explanation for the reduced TH1 responses seen in Ccr2−/− mice. We therefore examined the accumulation of inflammatory DCs in the lymph nodes of CFA-immunized and influenza-infected mice. Consistent with our hypothesis, CD11bhiGr-1+ DCs were markedly reduced in the draining lymph nodes of immunized Ccr2−/− mice (Fig. 5a). CD11bhiGr-1+ DC accumulation was also markedly reduced in the mediastinal lymph nodes of Ccr2−/− mice during influenza infection (Fig. 5b,d). Importantly, when Ccl2−/− mice were examined in the same manner, they displayed no reduction in CD11bhiGr-1+ DC accumulation (Fig. 5c,d). This finding demonstrates that the accumulation of CD11bhiGr-1+ DCs in lymph nodes requires CCR2 but not CCL2. In contrast, the accumulation of DCs in footpads appears to be CCL2-dependent. The number of CD11c+CD11b+ DCs found in footpads 3 days after CFA injection was reduced to a similar extent in Ccr2−/− and Ccl2−/− mice (Fig. 5e).
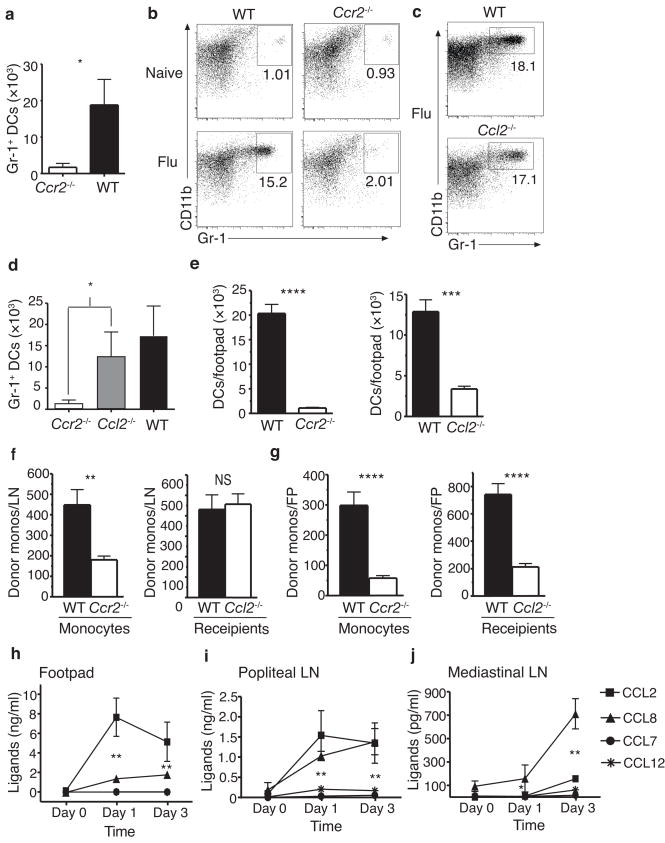
Chemokine dependence of inflammatory DC accumulation. (a) Number of CD11bhiGr-1+ DCs per 4 draining lymph nodes in Ccr2−/− and C57BL/6 (WT) mice 3 d after immunization with OVA/CFA. Significance determined by Student’s t-test, n = 3 mice. (b,c) Flow profiles of CD11c+CD11b+B220− CD8− mediastinal lymph node cells in WT and Ccr2−/− mice (b), or WT and Ccl2−/− mice (c) at baseline and 5 d after influenza infection. (d) Number of CD11bhiGr-1+ DCs per lymph node in mice described in (b,c). Significance determined by ANOVA using Bonferroni’s multiple comparison test. n = 6 mice. (e) Accumulation of CD11c+CD11b+ DCs in footpads of WT, Ccl2−/− and Ccr2−/− mice 3 d post OVA/CFA injection. n = 10. (f,g) Number of donor monocytes in inflamed draining lymph nodes (f) and footpads (g) 2 h after i.v. injection. n = 10. Left: mean ± SD of WT or Ccr2−/− CD11b+Ly6ChiCD45.2+ donor cells per WT recipient (CD45.1) lymph node or FP. Right: mean ± SD of WT CD11b+Ly6Chi donor cells per WT or Ccl2−/− recipient lymph node or FP. Significance determined by Student’s t-test. (h–j) Concentration of CCR2 ligands in footpads (e), and popliteal lymph nodes (f) after footpad injection of OVA/CFA, and in mediastinal lymph nodes (g) after influenza infection, as determined by ELISA. n = 3–4 mice. Significance determined by ANOVA for CCL2 vs. CCL8 (h,j) or both CCL2 and CCL8 vs. CCL7 and CCL12. *P < 0.05; ** P < 0.005; *** P < 0.0005; **** P < 0.00005
To confirm that the difference in inflammatory DC accumulation seen in Ccr2−/− and Ccl2−/− mice was due to a difference in inflammatory monocyte trafficking, we examined the accumulation of bone marrow monocytes in lymph nodes two hours after IV injection. To stimulate inflammation, mice were injected in the hind footpad with OVA plus CFA and, 72 hours later, injected by tail vein with labeled Gr-1+ bone marrow monocytes. When injected into wild-type mice, the migration of Ccr2−/− monocytes to the draining popliteal lymph nodes was significantly reduced relative to wild-type monocytes (Fig. 5f). In contrast, monocyte migration to lymph nodes in Ccl2−/− mice was not decreased relative to wild-type mice (Fig. 5f). In these studies, monocyte migration to the inflamed footpads was reduced to a similar extent in Ccr2−/− and Ccl2−/− mice (Fig. 5g).
To determine why monocyte extravasation into lymph nodes does not require CCL2, we examined the concentrations of various CCR2 ligand proteins in resting and inflamed lymph nodes and footpads. After CFA injection into footpads, CCL2 was abundantly produced in the footpad, with CCL8 (also known as MCP-2) seen at much lower concentrations and no CCL7 (MCP-3) or CCL12 (MCP-5) detected (Fig. 5h). At the same time, the draining popliteal lymph nodes contained high concentrations of both CCL2 and CCL8 (Fig. 5i). In contrast, CCL8 was the predominant CCR2 ligand expressed in mediastinal lymph nodes during influenza infection, with little CCL2 detected (Fig. 5j). These results provide a mechanistic basis for our finding that inflammatory monocyte extravasation was CCL2-dependent in inflamed footpads but CCL2-independent in inflamed lymph nodes and account for the divergence in inflammatory DC accumulation seen in the lymph nodes of Ccr2−/− and Ccl2−/− mice.
Reduced TH1 stimulatory DCs in Ccr2−/− lymph nodes
The finding that blood-derived inflammatory DCs accumulated in the lymph nodes of Ccl2−/− but not Ccr2−/− mice strongly suggests that these cells play an important role in stimulating TH1 immune responses. To directly compare TH1 T cell polarization in Ccr2−/− and Ccl2−/− mice, we examined lymph node T cells after subcutaneous immunization with OVA in CFA. Upon restimulation with OVA, IFN-γ production by T cells from Ccr2−/− mice was markedly reduced while T cells from Ccl2−/− mice displayed increased IFN-γ production (Fig. 6a). At the same time, T cells from Ccr2−/− and Ccl2−/− mice produced normal amounts of IL-2 (Fig. 6b). To confirm that the reduced T cell IFN-γ production seen in Ccr2−/− mice was due to a reduction in DC TH1-stimulatory activity, we examined this activity in wild-type and Ccr2−/− lymph node DC populations. To obtain sufficient cell numbers, DCs were purified from the mediastinal lymph nodes of influenza-infected mice. As above, CD11bhiGr-1+ DCs purified from wild-type lymph nodes stimulated robust T cell IFN-γ production (Fig. 6c). CD11bhiGr-1+ DCs were not present in the lymph nodes, spleens, or blood of infected Ccr2−/− mice and therefore could not be tested. CD11bhiGr-1− DCs purified from wild-type lymph nodes stimulated low amounts of IFN-γ, demonstrating a potency of about 25% that of Gr-1+ DCs. Among CD11bhiGr-1− DCs purified from Ccr2−/− lymph nodes, this activity was reduced, suggesting that a portion of inflammatory DCs may display a Gr-1− phenotype due to loss of Ly6-C expression30. CD8+ DCs from Ccr2−/− mice also stimulated production of low amounts of IFN-γ, with an activity similar to wild-type CD8+ DCs (compare Figs. 6c and and4a)4a) but much lower than CD11bhi Gr-1+ DCs. These differences in TH1-stimulating activity are not due to differences in the stimulation of T cell proliferation (Fig. 6d). We conclude that the reduction in TH1 responses seen in Ccr2−/− mice was due to an absence of TH1-stimulatory inflammatory DC within inflamed lymph nodes.
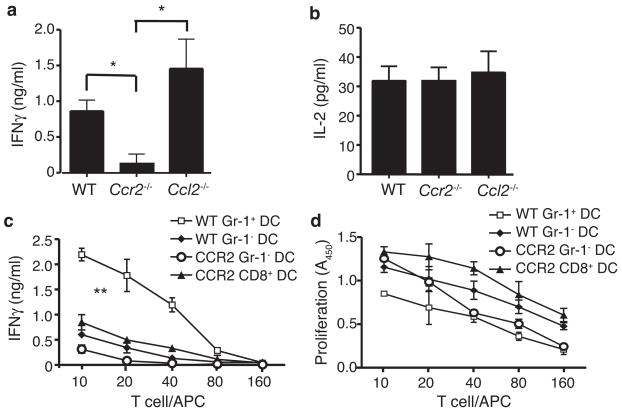
TH1 responses in the lymph nodes of Ccr2−/− and Ccl2−/− mice. (a–b) IFN-γ (a) and IL-2 (b) production by total lymph nodes cells isolated from WT, Ccr2−/− and Ccl2−/− mice 6 days after immunization with OVA/CFA. T cells were restimulated with OVA for 48 h and IFN-γ in the supernatant measured by ELISA. Data points represent mean ± SD, n = 3 mice. Significance determined by Student’s t-test. *p<0.05 (c,d) IFN-γ production by (c) and proliferation of (d) OT2 cells stimulated as in Fig. 4a with the indicated DC types. Data points represent mean ± SD for 3 experiments, each using cells pooled from 5–7 mice. Response curves were analyzed by linear regression, and P-value for slopes was determined by ANOVA. **p<0.0001 for WT Gr-1+ DC vs. all other DC types.
Discussion
Current evidence suggests that multiple DC subtypes cooperate to stimulate T cell responses. Resident lymph node DCs present antigen that drains to lymph nodes and stimulate the initial steps of T cell activation, including increased CD69 expression and retention within lymph nodes33,34. Migratory DCs transport high amounts of antigen to lymph nodes and stimulate the next steps of T cell activation, namely IL-2 production and proliferation33,34. Both these DC activities are required to stimulate robust T cell proliferation and the generation of effector responses33,34. As immune stimulation continues, monocytes recruited to peripheral sites develop into inflammatory DCs, which migrate to lymph nodes to provide a continuing stimulus for T cell proliferation or polarization11.
Here, we demonstrate that an additional lymph node DC type, blood-derived inflammatory DCs, plays a key role in the development of acute T cell responses, primarily by stimulating T cell polarization. Blood-derived inflammatory DCs appear in lymph nodes as CD11c+CD40intCD11bhiGr-1+ cells that accumulate early after a TH1-polarizing stimulus, capture antigen, produce large amounts of IL-12(p70), and stimulate CD4+ T cell IFN-γ production much more potently than other lymph node DC subtypes. Our findings indicate that CD11c+CD40intCD11bhiGr-1+ DCs arise from monocytes that enter lymph nodes directly from the blood. The accumulation of inflammatory DCs in lymph nodes requires CCR2, but not CCL2 or CCR7 and therefore provides an explanation for the defects in T cell polarization seen in Ccr2−/−, Ccl2−/− and plt mice. These defects have been recognized for some time, but have been difficult to reconcile with the classic view of T cell activation strictly by lymphatic-born DCs17.
Our findings strongly suggest that both CCL2 and CCL8 can stimulate monocyte extravasation into lymph nodes. Consistent with the relative concentrations of these chemokines, we find that monocyte extravasation is completely CCL2-independent in mediastinal lymph nodes and somewhat CCL2 dependent in popliteal lymph nodes (data not shown). A previous study demonstrated that monocyte extravasation into lymph nodes can be CCL2-dependent19. As reported in that paper, we find little CCL2 mRNA in inflamed lymph nodes (data not shown). Because most CCL2 protein in lymph nodes is produced remotely, lymph node CCL2 concentrations and the contribution of CCL2 to lymph node monocyte extravasation are likely to depend on the specific inflammatory stimulus, the time after stimulation, and the peripheral tissue being drained. Direct proof that CCL8 stimulates monocyte extravasation into lymph nodes will probably require an examination of mice that lack this chemokine.
We find that CCL2 is the predominant CCR2 ligand expressed in inflamed footpads and that inflammatory monocyte migration to footpads is CCL2-dependent. For this reason, Ccr2−/− and Ccl2−/− mice display similar defects in the accumulation of DCs in footpads and, presumably, similar alterations in lymph node DC populations derived from peripheral tissues. Thus, the presence of blood-derived inflammatory DCs appears to be the only substantial difference in lymph node DC populations between Ccr2−/− and Ccl2−/− mice. This difference is closely associated with the ability to develop TH1 responses, demonstrating that blood-derived inflammatory DCs provide a T cell polarizing activity that is sufficient for robust TH1 immune response. Blood-derived inflammatory DCs are likely to also be necessary for such responses under the conditions we examined, as no other lymph node DC subtype displays a comparable TH1-stimulatory activity. However, it is possible that both Ccr2−/− and Ccl2−/− mice lack a peripheral DC type that would be sufficient to stimulate TH1 polarization in the absence of blood-derived inflammatory DCs. T cell proliferative responses are normal in Ccr2−/− mice, demonstrating that blood-derived inflammatory DCs are not necessary for T cell proliferation35.
The immune response abnormalities seen in plt mice involve defects in both T cell polarization and the contraction phase of T cell response. Plt lymph nodes contain normal numbers of blood-derived inflammatory DCs, but are markedly deficient in all other, CCR7-dependent DC types. The increased TH1 responses seen in plt mice provide additional evidence that blood-derived inflammatory DCs are sufficient for TH1 polarization, but also suggest that this response is normally modulated by one or more CCR7-dependent DC types. Plt mice display normal T cell proliferative responses, suggesting that blood-derived inflammatory DCs can provide a stimulatory activity that is sufficient to drive T cell proliferation. This conclusion is supported by our finding that lymph node CD11b+Gr-1+ DCs stimulated T cell proliferation in vitro to an extent comparable to other lymph node DC types. Plt mice also display a marked prolongation of T cell responses, increased antigen-specific T cell numbers, and a significant decrease in the percentage of antigen-specific lymph node T cells that undergo apoptosis after immunization23,24. These findings suggest that a CCR7-dependent DC population stimulates the contraction phase of T cell responses, perhaps by inducing the apoptosis of activated T cells36.
Our findings suggest that inflammatory DCs capture antigen within lymph nodes, as we detected no antigen on circulating monocytes. This may occur via the capture of antigen in free form within lymph nodes or via the transfer from other DCs5,37. Based on the differing responses of plt and Ccr7−/− mice, we speculate that inflammatory DCs obtain antigen via the transfer from other DC types. Plt mice express low amounts of CCL21 on lymphatics and display some migration of Langerhans and dermal DCs to lymph nodes20,22. In contrast, Ccr7−/− mice lack all CCR7 activity and display almost no detectable migration of these cell types25. Because plt and Ccr7−/− mice would be expected to have normal amounts of free antigen in lymph nodes, any differences in antigen trafficking to lymph nodes are likely to be due to transport by migratory DCs. The activity of Ccr7−/− migratory DCs is not sufficient to support CD4+ T cell proliferation after OVA-CFA immunization34. Similarly, during influenza infection, antigen transport in Ccr7−/− mice is insufficient to support CD8+ T cell proliferation and results in only limited CD4+ T cell responses38. Consistent with our findings, those limited CD4+ T cell responses include a marked increase in T cell IFN-γ production38. The amount of antigen in lymph nodes required to stimulate T cell activation is very low but displays a sharp threshold that can be achieved with very few DCs39. It appears that this threshold is reached in plt mice, but in some cases, not Ccr7−/− mice. The increased T cell responses in plt mice appear to be due to their increased proportion of blood-derived inflammatory DCs, suggesting that differences in immune response between plt and Ccr7−/− mice are due to the delivery of antigen to these cells by migratory DC types.
Under the conditions we examined, blood-derived inflammatory DCs have a potent TH1-stimulatory activity, probably due to their robust production of IL-12(p70). CD11b+ Gr-1+ DCs obtained from IL-12(p35)-deficient mice fail to stimulate T cell IFN-γ production in vitro (data not shown). These cells may also stimulate TH2 T cell polarization. Inflammatory DCs stimulate TH2 responses after intraperitoneal injection of OVA in alum adjuvant40. Two publications41,42 and our own unpublished findings demonstrate that plt mice display increased TH2 responses when given a TH2-polarizing stimulus. At present, it is not clear whether increased TH2 responses in plt mice are due to an increased TH2 polarizing activity or to a decreased ability to modulate immune responses. TH2 responses are not decreased in Ccr2−/− mice, demonstrating that blood-derived inflammatory DCs are not necessary for TH2 polarization. Delineating the specific role that blood-derived inflammatory DCs play, relative to other DC types, in regulating immune responses will have important implications for efforts to enhance or inhibit these responses in clinical settings.
Methods
Mice
BALB/c, C3H/He, and C57BL/6 mice were purchased from Charles River Labs. CD11c-DTR, CD45.1, DO11.10, OT2, Ccr2−/− and Ccl2−/− mice were purchased from The Jackson Laboratory. DDD/1-plt mice were backcrossed to BALB/c mice 10 times and to C57BL/6 mice 11 times23, and maintained at Duke University Medical Center and Toho University School of Medicine. All mice were kept under specific pathogen-free conditions and used at 7 to 15 wk of age. Mice were immunized subcutaneously at two sites on the back with 100 μg chicken OVA (Sigma-Aldrich) in RIBI adjuvant (Sigma-Aldrich) or in a 1:1 emulsion of PBS and CFA (Difco or Sigma-Aldrich) or in the footpad with 10–20 μg OVA in CFA. For influenza infections, mice were anesthetized with ketamine-xylazine (100 mg/kg-10mg/kg) i.p., then infected with H1N1 influenza virus strain A/Puerto Rico/8/34 (PR8) (ATCC, Manassas, VA, VR-95) intranasally (30 μl of 5 × 105 TCID50/ml virus per mouse). Body weight of infected mice was monitored daily. All experiments were carried out according to institutional guidelines and approved by the Duke IACUC committee.
Antibodies
Purified, biotinylated or Alexa Fluor 488, Allophycocyanine (APC), FITC, Phycoerythrin (PE), PE-Cy5, PE-Cy5.5, APC-Cy7, APC-AF750, PE-Cy7 or PE-Texas Red-conjugated mAbs specific for the following mouse antigens were used; CD3 (145-2C11), CD4 (RM4-5), CD8α (53-6.7), CD11b (M1/70), CD11c (HL3), CD16/32 (2.4G2), CD19 (1D3), CD40 (3/23), CD44 (IM7), CD45R/B220 (RA3-6B2), CD49b (DX5), CD62L (MEL-14), CD80 (16-10A1), CD86 (GL1), I-Ab (25-9-17), I-Ad (AMS-32-1), I-A/I-E (2G9), Ly6C/G (Gr-1, RB6-8C5), Ly6G (1A8), Ly76 (TER119), DO11.10 TCR clonotype (KJ1-26), Sca-1 (E13-161.7), c-kit (2B8), CCR3 (83101), CCR7 (4B12) (purchased from BD Pharmingen, BioLegend, CALTAG or eBioscience). The following isotype control mAbs were used; mouse IgG1 (G155-178), rat IgG1 (R3-34), rat IgG2a (R35-95), rat IgG2b (A95-1), hamster IgG (G235-2356) (BD Pharmingen or eBioscience).
Flow cytometric analysis
Cells were stained with antibodies in Mg2+- and Ca2+-free Dulbecco’s PBS (Invitrogen) containing 3% FCS, 5% normal mouse serum, 5% normal rat serum, 5 μg/ml anti-CD16/32 mAbs and 10 mM EDTA for 30 min at 0°C. For cell sorting, HBSS was used instead of Dulbecco’s PBS. After washing, stained cells were analyzed on a FACS Calibur™ or FACS LSRII™ flow cytometer (Becton Dickinson) and CELLQuest™ or FACS Diva™ software, respectively. CD3+ T cells, CD19+ B cells, 7-aminoactinomycin D+ (7-AAD; BD Pharmingen) dead cells and FL3+ autofluorescent cells were excluded from data for lymph node DC analysis.
DC isolation and sorting
DCs were prepared as previously described with some modifications43. Lymph nodes or spleens were minced in Mg2+- and Ca2+-free HBSS (Invitrogen) containing 5% FCS and 10 mM HEPES pH 7.4 (GIBCO), then digested with 1 mg/ml of collagenase A (Roche) and 0.2 mg/ml of DNase I (Sigma-Aldrich) for 35 min at 37°C. EDTA (20 mM final concentration) was added for 5 min at 25°C. Single cell suspensions were prepared using a 70 μm-CellStrainer™ (Falcon) nylon mesh, layered over RPMI 1640 containing 10% FCS and 16% Metrizamide (Accurate Chemical and Scientific), or 17.2% Nycodenz (Accurate Chemical and Scientific), and centrifuged at 450g for 20 min at 25°C. Low-density cells at the interface were collected and washed with HBSS containing 5 % FCS, 10 mM HEPES pH 7.4 plus 10 mM EDTA.
To purify total CD11c+ DCs, low density cells from lymph nodes were incubated with biotinylated anti-CD11c and streptavidin-conjugated MACS beads (Miltenyi), then positively sorted on a magnetic activated cell sorter (MACS, Miltenyi) according to the manufacture’s instruction. Over 98% of the sorted cells were CD11c+. To purify DC subsets, cells were sorted as described previously43. Briefly, low density lymph node cells were stained with anti-CD3, CD8a, CD11b, CD11c, CD19, Ly6C/G mAbs for 30 min at 0°. After washing twice, individual DC subsets among CD11c+CD3−CD19− cells were sorted on a FACS Vantage™ flow cytometer (Becton Dickinson). Cell purity after sorting was 85~95%.
T cell culture and proliferation assays
Naive CD4+ T cells from DO11.10, OT2, or C3H/He mice were prepared as previously described43. Briefly, single cells suspensions of pooled lymph nodes and spleens were incubated with 1.25 μg/ml biotinylated anti-CD8, CD11b, CD11c, CD16/32, CD19, CD25, B220, CD49b, Ly6/G and TER119 for 30 min at 0°C, then streptavidin-conjugated MACS beads (Miltenyi) for 20 min at 0°C, negatively selected on a MACS LD column, and centrifuged on percoll (50% and 60%) (Invitrogen). Cells at the 50–60% percoll interface were collected as naive CD4+ T cells. >97% of the purified cells were CD4+, and >90% CD45RB+CD44int/lo. Cells were cultured in cRPMI-10 (RPMI-1640 (Invitrogen), 10% FCS (GIBCO), 20 mM HEPES pH 7.4, 2-mercaptoethanol (Sigma-Aldrich), 50 U/ml Penicillin, and 50 μg/ml Streptomycin) in U-bottom 96-well plates at 37°C in 5% CO2. To prepare the OVA-specific T cell line, 5 × 105 naive DO11.10 CD4+ T cells were cultured with 1 μM OVA323–339 peptide and 106 30 Gy-irradiated splenic low density cells in 2 ml cRPMI-10 supplemented 20 U/ml IL-2 (Shionogi) in 24-well plates. The stimulated CD4+ T cells were maintained in cRPMI-10 supplemented 10 U/ml IL-2 and restimulated with 1 μM OVA323–339 plus 106 spleen cells every 2–3 weeks. OVA-primed CD4+ T cells were rested for at least 6 days before use. For proliferation assays, 105 naive CD4+ T cells or 5 × 104 cells from the DO11.10 T cell line were co-cultured with freshly isolated and irradiated DCs in 200 μl cRPMI-10 in U-bottom 96-well plates for 3 to 6 days and T cell proliferation measured either by pulsing with 1.25μCi [3H]-thymidine (Amersham Bioscience) during the final 6 h of culture and measuring incorporation on a Matrix 96 direct beta counter (Packard Instruments) or by pulsing with 10 nM BrdU (Roche) in the final 3–6 h of culture, then measuring incorporation using a BrdU-ELISA kit (Roche) according to the manufacture’s instructions.
OVA re-stimulation of total lymph node cells
Total cells were isolated from the draining lymph nodes of immunized wild-type, plt, Ccr2−/−, or Ccl2−/− mice and cultured in the presence of 1 μg/ml OVA for 48 h. Cytokine production was measured by intracellular staining for IFN-γ and by analysis of supernatants for IL-2 and IFN-γ by ELISA. For ELISA assays, aliquots of T cell culture supernatants were collected before [3H]-thymidine or BrdU labeling and assayed by Opt-EIA (BD Bioscience) according to the manufacture’s instructions.
Delayed type hypersensitivity and contact hypersensitivity assays
Seven days after subcutaneous immunization with OVA-CFA or OVA-RIBI immunized and naive mice were injected intracutaneously with 10 μl PBS into right ear and 10 μl PBS with 25 μg OVA into left ear. Ear thickness was measured using a Peacock dial thickness gauge (Ozaki MFG) before and 24 h after elicitation. Ear swelling was calculated as the difference in thickness between 0 and 24 h. Reciprocal bone marrow transplants between BALB/c and BALB/c-plt mice were performed as previously described44. Briefly, 107 bone marrow cells were injected intravenously into 8.5 Gy gamma-irradiated recipients 30 days prior to immunization. For contact hypersensitivity assays, mice were sensitized with 50 μl 100 mg/ml oxazolone in vehicle (acetone:Olive oil, 4:1) on their shaved abdomens. 7 to 56 days later, their right ears were challenged with 10 μl of 10mg/ml oxazolone in vehicle and their left ears with vehicle alone. Ear thickness was measured before elicitation and at 24 h and ear swelling calculated as above.
DC migration assay
BALB/c BM-derived DCs were prepared by culturing bone marrow cells at 1 × 106/ml in cRPMI1640, 10% FBS, and 3.3 ng/ml GM-CFS (R&D), harvested at day 9, labeled with CFSE, then injected into footpads (8 × 105/pad) of anesthetized BALB/c or BALB/c-plt mice. Before or 1 – 3 days after DC injection, draining popliteal lymph nodes were harvested, prepared as above, and the number of CFSE+CD11c+autofluorescence− cells determined by flow cytometric analysis.
Monocyte purification, transfer, and migration
Bone marrow cells were collected in RPMI 10% FBS, prepared as single cell suspensions, and washed. Red blood cells were lysed using ACK buffer and the remaining cells incubated with 1.25 μg/ml biotinylated anti-CD3, CD4, CD8, CD11c, CD19, Sca-1, B220, c-kit, I-Ab, and TER119, and FITC-labeled anti-CCR3 and Ly6G for 30 min at 0°C followed by streptavidin-conjugated and anti-FITC MACS beads (Miltenyi) for 20 min at 0°C and negatively selected over a MACS LD or LS column. The resulting monocytes were labeled with CFSE and 3–6 × 106 injected intravenously into recipient mice 3 days after footpad injection of 10 μl OVA+CFA. Two hours later, the popliteal lymph nodes and footpads of recipient mice were harvested, digested with collagenase A and DNase I, processed for DC isolation as above using an 18% nycodenz gradient, and subjected to flow cytometric analysis. Donor monocytes were identified as CFSE+Ly6ChiCD11b+Ly6G− autofluorescence− cells.
OVA antigen uptake by DC and monocytes
C57BL/6 mice were immunized on the back with OVA+CFA (Sigma) (100 μg/site) or OVA-AF647 (Invitrogen) +CFA. After 3 days, draining lymph nodes and blood cells were examined for AF647 fluorescence by flow cytometry.
CCR2 ligands expression in footpad and lymph nodes
C57BL/6 mice were immunized in the footpads with OVA+CFA (10 μg/FP), infected with influenza virus as above, or left untreated (day 0). On days 1 and 3, mice were sacrificed, and their footpads, draining popliteal lymph nodes, or mediastinal lymph nodes harvested. Tissues were frozen and thawed once, and then homogenized by bead milling (Glen Mill) with vortexing in a FastPrep FP120 homogenizer (Thermo Savant). Supernatants were harvested by centrifugation and assayed for CCR2 ligands by ELISA. Quantikine® kits (R&D system) were used for measuring CCL2 and CCL12, Instant ELISA (Bender MedSystems) was used for CCL7. For CCL8 ELISAs, antibody pairs and recombinant proteins were purchased from R&D system and PeproTech, respectively.
Statistics
All numerical data were analyzed for significance by ANOVA or unpaired Student’s t-test using Prism software, as indicated in the figure legends.
Acknowledgments
We thank K. Nakano for excellent technical assistance and J. Whitesides, P. McDermott, and L. Olive for expert cell sorting. Supported by NIH grants AI047262 and HL085473 (MDG), the Japanese Ministry of Science and Technology (1579054, HN; 14021121, TK), the Duke Human Vaccine Institute (HN), the Japan Health Sciences Foundation (KH51052, TK), the Japan Society for the Promotion of Science (10670606, 12670621, TK) and the Japan Society for the promotion of Science for Young Scientists (MY), and in part by the intramural branch of the NIEHS, NIH.
References
Full text links
Read article at publisher's site: https://doi.org/10.1038/ni.1707
Read article for free, from open access legal sources, via Unpaywall:
https://europepmc.org/articles/pmc2668134?pdf=render
Citations & impact
Impact metrics
Citations of article over time
Alternative metrics
Article citations
γδ T cell-mediated activation of cDC1 orchestrates CD4+ Th1 cell priming in malaria.
Front Immunol, 15:1426316, 15 Aug 2024
Cited by: 0 articles | PMID: 39211036 | PMCID: PMC11357926
Tumor eradication by hetIL-15 locoregional therapy correlates with an induced intratumoral CD103intCD11b+ dendritic cell population.
Cell Rep, 42(5):112501, 12 May 2023
Cited by: 3 articles | PMID: 37178117 | PMCID: PMC10758290
Dendritic cell phenotype and function in a 3D co-culture model of patient-derived metastatic colorectal cancer organoids.
Front Immunol, 14:1105244, 25 Jan 2023
Cited by: 11 articles | PMID: 36761758 | PMCID: PMC9905679
Increased blood CD226- inflammatory monocytes with low antigen presenting potential correlate positively with severity of hemorrhagic fever with renal syndrome.
Ann Med, 55(2):2247000, 01 Jan 2023
Cited by: 0 articles | PMID: 37585670 | PMCID: PMC10435008
Dendritic Cells: The Long and Evolving Road towards Successful Targetability in Cancer.
Cells, 11(19):3028, 27 Sep 2022
Cited by: 6 articles | PMID: 36230990 | PMCID: PMC9563837
Review Free full text in Europe PMC
Go to all (231) article citations
Data
Data behind the article
This data has been text mined from the article, or deposited into data resources.
BioStudies: supplemental material and supporting data
Similar Articles
To arrive at the top five similar articles we use a word-weighted algorithm to compare words from the Title and Abstract of each citation.
CCR7 is critically important for migration of dendritic cells in intestinal lamina propria to mesenteric lymph nodes.
J Immunol, 176(2):803-810, 01 Jan 2006
Cited by: 263 articles | PMID: 16393963
Monocyte-derived dendritic cell recruitment and allergic T(H)2 responses after exposure to diesel particles are CCR2 dependent.
J Allergy Clin Immunol, 129(2):483-491, 08 Sep 2011
Cited by: 42 articles | PMID: 21906792
Clearance of influenza virus from the lung depends on migratory langerin+CD11b- but not plasmacytoid dendritic cells.
J Exp Med, 205(7):1621-1634, 01 Jul 2008
Cited by: 341 articles | PMID: 18591406 | PMCID: PMC2442640
In vivo manipulation of dendritic cell migration and activation to elicit antitumour immunity.
Novartis Found Symp, 256:241-54; discussion 254-69, 01 Jan 2004
Cited by: 22 articles | PMID: 15027495
Review
Funding
Funders who supported this work.
Intramural NIH HHS
NHLBI NIH HHS (3)
Grant ID: HL085473
Grant ID: R01 HL085473
Grant ID: R01 HL085473-01A2
NIAID NIH HHS (5)
Grant ID: R01 AI047262
Grant ID: R01 AI047262-01A2
Grant ID: R01 AI047262-02
Grant ID: R01 AI047262-03
Grant ID: AI047262