Abstract
Free full text

Reduced growth, abnormal kidney structure, and type 2 (AT2) angiotensin receptor-mediated blood pressure regulation in mice lacking both AT1A and AT1B receptors for angiotensin II
Abstract
The classically recognized functions of the renin–angiotensin system are mediated by type 1 (AT1) angiotensin receptors. Whereas man possesses a single AT1 receptor, there are two AT1 receptor isoforms in rodents (AT1A and AT1B) that are products of separate genes (Agtr1a and Agtr1b). We have generated mice lacking AT1B (Agtr1b −/−) and both AT1A and AT1B receptors (Agtr1a −/−Agtr1b −/−). Agtr1b −/− mice are healthy, without an abnormal phenotype. In contrast, Agtr1a −/−Agtr1b −/− mice have diminished growth, vascular thickening within the kidney, and atrophy of the inner renal medulla. This phenotype is virtually identical to that seen in angiotensinogen-deficient (Agt−/−) and angiotensin-converting enzyme-deficient (Ace −/−) mice that are unable to synthesize angiotensin II. Agtr1a −/−Agtr1b −/− mice have no systemic pressor response to infusions of angiotensin II, but they respond normally to another vasoconstrictor, epinephrine. Blood pressure is reduced substantially in the Agtr1a −/− Agtr1b −/− mice and following administration of an angiotensin converting enzyme inhibitor, their blood pressure increases paradoxically. We suggest that this is a result of interruption of AT2-receptor signaling. In summary, our studies suggest that both AT1 receptors promote somatic growth and maintenance of normal kidney structure. The absence of either of the AT1 receptor isoforms alone can be compensated in varying degrees by the other isoform. These studies reaffirm and extend the importance of AT1 receptors to mediate physiological functions of the renin–angiotensin system.
The renin–angiotensin system (RAS) regulates blood pressure and body fluid balance and plays a role in growth and development (1–3). The biological functions of the RAS and its major effector peptide, angiotensin II, are mediated by specific receptors. Two angiotensin receptor subtypes, type 1 angiotensin receptor (AT1) and AT2, can be distinguished pharmacologically. The classically recognized actions of the RAS are mediated by AT1 receptors (1, 2). Whereas man possesses a single AT1 receptor, rodents possess two AT1 receptor isoforms, designated AT1A and AT1B. These receptors are the products of distinct but highly homologous genes (Agtr1a and Agtr1b) located on separate chromosomes (3, 4). Expression of the AT1A receptor subtype predominates in nearly all tissues except the anterior pituitary gland and the adrenal cortex, where AT1B receptors are more highly expressed (5–9). Because pharmacological AT1 receptor antagonists block both AT1A and AT1B receptors, it has been difficult to separate their distinct functions (2).
Experiments using gene targeting have provided insight into the roles of RAS genes in regulating blood pressure, body fluid homeostasis, and development. For example, mice that are unable to generate angiotensin II because of targeted mutations in the angiotensinogen (Agt −/−) or angiotensin-converting enzyme (Ace −/−) genes have virtually identical phenotypes characterized by reduced survival, low blood pressure, and abnormal kidney structure. In the kidney, these animals develop thickening of arterial walls, focal areas of renal cortical inflammation, and hypoplasia of the inner medulla, which is associated with a defect in urinary concentrating function (10–13). The reduced survival and kidney abnormalities of Agt −/− mice can be rescued by human renin and angiotensinogen transgenes (14).
Thus, the absence of angiotensin II results in a severe phenotype. Two major features of this phenotype, reduced survival and abnormal kidney morphology, are not recapitulated by targeted deletions of the individual angiotensin receptors, AT1A, AT1B, or AT2 (15–20). Furthermore, the relative roles of each of these receptors in the regulation of blood pressure and circulatory homeostasis are not clear. To define the contribution of the AT1 receptor subtypes to these phenotypes, we have generated mice lacking AT1B receptors (Agtr1b −/−) and mice lacking both AT1A and AT1B receptors (Agtr1a −/−Agtr1b −/−). These animals have reduced growth, abnormal kidney structure, and reduced blood pressure. In the mice that completely lack AT1 receptors, we find evidence for regulation of blood pressure by AT2 receptors.
MATERIALS AND METHODS
Generation of AT1B Receptor-Deficient Mice.
To clone the Agtr1b gene, we used a probe that we had previously used to clone the AT1A receptor (15). This probe was used to screen a genomic library constructed with genomic DNA isolated from the mouse embryonic stem (ES) cell line E14tg2a that had been partially digested with the restriction endonuclease MboI. The phage clone containing the Agtr1b gene was identified by mapping using known sequences from the AT1B receptor cDNA (3). Hybridizing phage was purified and a restriction map of the genomic DNA fragment was prepared, as depicted in Fig. Fig.11A. To construct the targeting vector shown in Fig. Fig.11B, a 10.5-kb HindIII fragment containing all of the protein coding sequences was subcloned into pBluescript KS (Stratagene). A 0.4-kb MscI/EagI fragment containing coding sequences was replaced by a neomycin resistance cassette (neo) from pMC1neopolA. The thymidine kinase (TK) gene from pMC1TK was inserted downstream of the 3′ homology arm. ES cells were grown, transformed, and screened by using standard methodologies (21). Colonies in which the plasmid had integrated by homologous recombination were identified by PCR using the primer pair depicted in Fig. Fig.11C. The sequences for these primers are: TTGGGGACAAGGGTATCATAGCCA and TGGCGGACCGCTATCAGGAC.
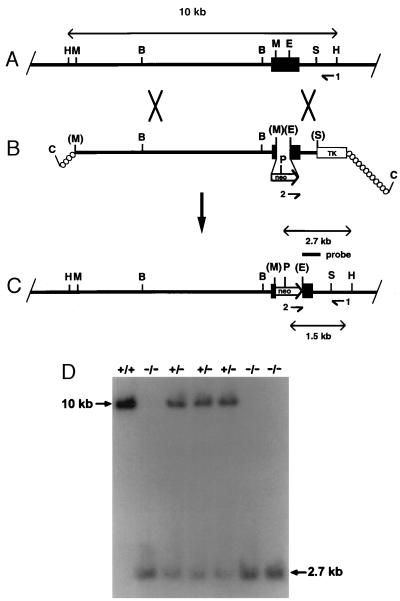
Targeted disruption of the Agtr1b gene. (A) The endogenous Agtr1b gene locus. The intronless coding region is depicted by the black box. (B) Targeting construct. neo, neomycin resistance cassette; TK, thymidine kinase. (C) Structure of the disrupted gene. The PCR primers (indicated by arrowheads and numbers), the lengths of diagnostic restriction fragments, and the probe used for Southern analysis are shown. H, HindIII; M, Msc1; E, EagI; S, Spe1; P, PstI; C, ClaI B, BamHI. Enzyme sites in brackets are destroyed during ligation. (D) Southern blot of offspring of an Agtr1b +/− × Agtr1b +/− cross. A 10.2-kb PstI/HindIII fragment indicates the wild-type allele, and a 2.7-kb fragment identifies the targeted locus.
Targeted ES cells were introduced into C57BL/6 blastocysts. The resulting male chimeras were mated with C57BL/6 or 129/SvEv females to identify germ-line competent chimeras that were capable of transferring the ES cell genome to their offspring. The targeted Agtr1b allele was detected by Southern blot analysis of genomic DNA isolated from tail biopsies as shown in Fig. Fig.11D. Offspring carrying the mutant allele were intercrossed to obtain animals that were homozygous for the targeted mutation (Agtr1b −/−).
Production of Double Homozygous Agtr1a −/−Agtr1b −/− Mice.
Mice with targeted mutations of the AT1A receptor gene (Agtr1a) have been produced in our laboratories as described previously (15). Several breeding strategies were used to produce double homozygous Agtr1a −/−Agtr1b −/− mice. First, Agtr1a−/− and Agtr1b −/− mice were crossed to produce double heterozygous Agtr1a +/−Agtr1b +/− animals that were then intercrossed. To increase the likelihood of obtaining double homozygotes, we also intercrossed Agtr1a −/−Agtr1b +/− or Agtr1a +/−Agtr1b −/− mice. Finally, once double homozygous mice were obtained, they were crossed with Agtr1a −/−Agtr1b +/− or Agtr1a +/−Agtr1b −/− mice.
RNA Isolation and Reverse Transcription—PCR (RT-PCR) Analysis of AT1B Expression.
Adrenal glands were harvested from Agtr1b +/+ and Agtr1b −/− mice and RNA was extracted from the tissues by using a commercially available reagent (Tri-reagent; Sigma). Total RNA was reverse transcribed to cDNA by using poly-dT (22). A fragment of the AT1B receptor cDNA was than amplified by PCR by using a sense primer derived from a portion from the Agtr1b gene that was deleted in the targeted gene (5′-ATACCGCTATGGAATACCAG-3′) and an antisense primer from the 3′-region of the Agtr1b gene (5′-TTGATAACCCTGCATGCGACC-3′). The diagnostic 700-bp fragment was identified on ethidium-stained gels. The presence and identity of the fragment were then confirmed by Southern blotting with a probe from the Agtr1b gene that was internal to the primer sequences.
Analysis of Growth and Development and Kidney Histology.
To determine the effects of the combined Agtr1a/Agtr1b gene disruptions on growth and development, we measured body weights along with wet weights of kidneys and heart in 4-month-old male wild-type and Agtr1a −/−Agtr1b −/− mice. The major organs from these animals were fixed in formalin, sectioned, and stained with hematoxylin and eosin. The histomorphology of these organs was then examined by light microscopy.
Assessment of Urinary Concentrating Capacity.
To examine the relative importance of the individual AT1 receptors in urinary concentration, we measured urine osmolality in groups of wild-type (n = 9), Agtr1a +/+Agtr1b −/− (n = 4), Agtr1a −/−Agtr1b +/+ (n = 14), and Agtr1a −/−Agtr1b −/− (n = 5) mice. Urine osmolalities were measured while mice had free access to drinking water and following 12 hours of complete water deprivation. Urine was collected after bladder massage and urine osmolality was measured immediately with a vapor pressure osmometer (Wescor, Logan, UT).
Vasoconstrictor Effects of Angiotensin II.
In preparation for studies to assess the vascular effects of angiotensin II, the angiotensin converting enzyme (ACE) inhibitor enalapril was administered to groups of Agtr1a +/+Agtr1b +/+ (n = 4), Agtr1a +/+Agtr1b −/− (n = 4) and Agtr1a −/−Agtr1b −/− (n = 4) mice to inhibit endogenous production of angiotensin II (23). Thirty mg/kg per day of enalapril was administered in the drinking water for 5 days. On the day of study, animals were anesthetized with isoflurane and a flexible plastic catheter (0.020ID; Braintree Scientific) was placed in the carotid artery to monitor arterial pressure. A second catheter was placed in the jugular vein to infuse angiotensin II. Intraarterial blood pressure was recorded continuously through the carotid catheter with a pressure transducer and windaq acquisition and playback software (Dataq Instruments, Akron, OH).
Beginning with an equilibration period of 5 min, blood pressure was recorded continuously for the duration of the experiment at 50 recordings per sec. Data collected from each animal were compressed to five recordings per sec, and the mean arterial blood pressure (MAP) recordings from animals in each experimental group were integrated and averaged. Immediately after the equilibration period, mice received a bolus injection of 0.9% sodium chloride solution (NS) in a volume equal to 0.1% of their body weight. This is the volume used for each of the injections throughout the remainder of the experiment. At 10-min intervals thereafter, increasing doses of 0.1, 1.0, and 10 μg/kg of angiotensin II (Peninsula Laboratories) were injected intravenously. Ten minutes after the 10 μg/kg dose of angiotensin II, 10 μg/kg of epinephrine was administered intravenously and MAP was monitored for an additional 5 min.
Systolic Blood Pressure Measurements in Conscious Mice.
Systolic blood pressures were measured in groups of Agtr1a+/+Agtr1b+/+ (n = 8), Agtr1a +/+Agtr1b −/− (n = 10), and Agtr1a −/−Agtr1b −/− (n = 13) mice by using a computerized tail-cuff system (Visitech Systems, Cary, NC) as previously described (24). Before the study was initiated, mice were adapted to the apparatus for at least 5 days. Systolic blood pressures were then measured for 10 days.
To examine the effect of reducing angiotensin II levels on blood pressure, these same groups of mice were treated with enalapril in drinking water at a dose of 30 mg/kg per day for 10 days, during which their systolic blood pressures were measured.
Statistical Analysis.
Data are presented as means ± SEM. Statistical significance between groups was assessed by an unpaired t test or by analysis of variance (Bonferroni/Dunn). Significance of comparisons within groups was determined by using a paired t test.
RESULTS AND DISCUSSION
Agtr1b Gene Targeting.
The gene encoding the AT1B receptor was disrupted in the E14Tg2a ES cell line by homologous recombination with the targeting plasmid shown in Fig. Fig.1.1. Targeted ES cell lines identified by PCR and confirmed by Southern blot analysis were introduced into blastocysts to generate chimeric mice. Male chimeras were crossed with female C57BL/6 mice and Agtr1b +/− offspring carrying the mutant allele were identified by Southern blot analysis as shown in Fig. Fig.11D.
Mice homozygous for the targeted mutation (Agtr1b −/−) were generated by intercrossing Agtr1b +/− animals. Among the progeny of these crosses, 25% were Agtr1b+/+, 54% were Agtr1b +/−, and 21% were Agtr1b −/−, corresponding to the proportions predicted by Mendelian inheritance. This shows that the absence of the AT1B receptors does not significantly affect intrauterine development or perinatal survival. Furthermore, the Agtr1b −/− animals could not be distinguished from wild-type littermates by simple observation. Histological examination of their major organ systems, including heart, kidney, and adrenal glands, did not reveal any pathological changes. Our findings confirm a previous report suggesting that the absence of AT1B receptors is not associated with an abnormal phenotype (20).
AT1B Receptor mRNA Expression in Mutant Mice.
To verify that the targeted mutation introduced into the Agtr1b locus resulted in inactivation of the gene, we examined expression of AT1B receptor mRNA in adrenal glands by RT-PCR. As shown in Fig. Fig.2,2, AT1B receptor mRNA could not be detected in Agtr1b −/− mice, confirming the efficacy of the gene targeting strategy.
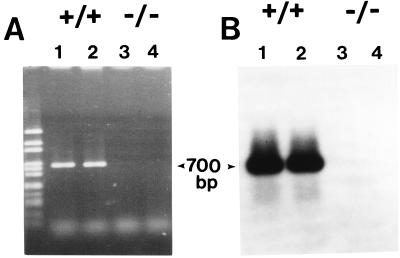
Assessment of AT1B mRNA expression by RT-PCR. Total RNA was isolated from adrenal glands of two wild-type and two Agtr1b −/− mice and each specimen was subjected to RT-PCR to amplify AT1B receptor mRNA. In tissues from the wild-type mice (lanes 1 and 2), a PCR product of the expected size (700 bp) was detected on an ethidium bromide stained gel (A) and was confirmed to be AT1B receptor mRNA by hybridization (B). This band was not detected in RNA from the Agtr1b −/− mice (lanes 3 and 4).
Production of Mice Lacking Both AT1A and AT1B Receptors.
Because the Agtr1a and Agtr1b genes are located on different chromosomes (4), the mutations could be combined through simple breeding. Agtr1a −/−mice were crossed with Agtr1b −/− mice to produce progeny that were heterozygous for both the Agtr1a and Agtr1b disruptions. These Agtr1a +/−Agtr1b +/− animals were then intercrossed to produce mice that were double homozygotes for the null Agtr1a and Agtr1b alleles. To increase the frequency of generating Agtr1a −/−Agtr1b −/− animals, we also crossed mice that were heterozygous for one disruption and homozygous for the other (e.g., Agtr1a +/−Agtr1b −/− × Agtr1a −/− Agtr1b +/−). Among 343 consecutive 21-day-old weanlings from these different crosses, there were significantly fewer Agtr1a −/−Agtr1b −/− pups than predicted by simple Mendelian inheritance (29 observed vs. 50 expected; P = 0.024 by χ2 analysis). Thus, in these mixed breedings, the complete absence of AT1 receptors is detrimental to survival, with an apparent perinatal mortality rate of approximately 40%. By comparison, the perinatal mortality rate of angiotensinogen-deficient (Agt −/−) weanlings in our colony is approximately 60% (13 observed vs. 37 expected; P = 0.0016 by χ2 analysis).
Once double homozygous Agtr1a −/−Agtr1b −/− mice were obtained, some of these animals were crossed with Agtr1a −/−Agtr1b +/− or Agtr1a +/−Agtr1b −/− mice to further increase the frequency of producing Agtr1a −/Ågtr1b −/− animals. When the genotypes of pups from these litters were analyzed, the observed frequency of Agtr1a −/−Agtr1b −/− weanlings was virtually identical to predicted (22 observed vs. 23 expected; P = 0.9 by χ2. Thus, the survival disadvantage associated with the absence of AT1 receptors can be overcome by selective breeding. This observation suggests that this trait can be modified significantly by background genes that are present in the C57BL/6 × 129 mixed breedings. In mice lacking only AT1A receptors, we have identified similar modifying effects of background genes (25).
Both male and female Agtr1a −/−Agtr1b −/− mice are fertile. The frequency of productive matings, gestation, delivery, and care of litters was not different between Agtr1a −/−Agtr1b −/− and wild-type females. Male Agtr1a −/−Agtr1b −/− mice produced litters of normal size. Our group (12) and others (10) have previously identified fertility problems in male ACE-deficient mice, but not in male angiotensinogen-deficient mice (11). In view of our current results, the defective fertility observed in ACE-deficient mice is not because of a lack of AT1-receptor signaling and may be caused by the loss of unique functions of testicular ACE probably unrelated to angiotensin II production. For example, other potential ACE substrates (bradykinin, enkephalins, and substance P) can alter functions of sperm (26, 27).
Growth Is Impaired in Mice with Combined AT1A/AT1B Receptor Deficiency.
In neonatal rats, AT1 receptor antagonists impair somatic and renal growth (28). However, in a previous study, we found that mice lacking only the AT1A receptor grow and develop normally (29). To determine whether the combined absence of AT1A and AT1B receptors adversely affects growth, we compared body, heart, and kidney weights between 4-month-old male wild-type and double homozygous Agtr1a −/−Agtr1b −/− mice. The surviving Agtr1a −/−Agtr1b −/− mice appeared healthy and could not be distinguished from wild-type littermates based on their external appearance. However, their body weights were significantly lower than wild-type mice (25.7 ± 1.4 vs. 29.6 ± 0.6g; P = 0.05). In addition, both kidney weights (0.25 ± 0.02 vs. 0.32 ± 0.02 g; P = 0.03) and heart weights (0.09 ± 0.01 vs. 0.13 ± 0.01 g; P = 0.02) were also significantly reduced in the AT1-deficient mice compared with wild-type controls. Because the ratios of heart weight to body weight (0.37 ± 0.02% vs. 0.45 ± 0.04%; P = 0.1) and kidney weight to body weight (0.96 ± 0.06% vs. 1.08 ± 0.06%; P = 0.2) are similar in Agtr1a −/−Agtr1b −/− and wild-type control mice, the combined absence of AT1A and AT1B receptors appears to cause a generalized impairment of growth.
Abnormalities in Kidney Structure in Double Homozygous Agtr1a −/−Agtr1b −/− Mice.
Agt −/− or Ace −/− mice that are unable to synthesize angiotensin II develop structural abnormalities in the kidney. These include thickening of arterial walls, focal areas of interstitial fibrosis and tubular atrophy, and atrophy of the renal papilla (10, 11, 13). The absence of any single known angiotensin II receptor, AT1A, AT1B, or AT2, does not completely recapitulate this phenotype (15–20). To determine whether the pathogenesis of these renal phenotypes requires the simultaneous absence of signaling through both AT1A and AT1B receptors, we examined renal histomorphology in Agtr1a −/−Agtr1b −/− mice and compared them to wild-type controls. As depicted in Fig. Fig.33B, Agtr1a −/−Agtr1b −/− mice develop atrophy of the inner medulla that is easily detected by light microscopy. These mice also develop severe thickening of arterial walls within the kidney (Fig. (Fig.33D) that resembles the vascular abnormalities seen in Agt −/− and Ace −/− mice (10–13). We also found focal areas of cortical interstitial inflammation, fibrosis, and tubular atrophy in the Agtr1a −/−Agtr1b −/− mice (Fig. (Fig.33F), similar to lesions that we observed in Agt −/−mice (11). These findings are in general agreement with those of Tsuchida et al., who recently reported that combined disruption of both AT1 receptor genes produces a severe kidney phenotype (30). There were no abnormalities noted in the histology of hearts and adrenal glands of Agtr1a −/−Agtr1b −/− mice. In particular, there was no evidence of arterial or arteriolar thickening at these sites.
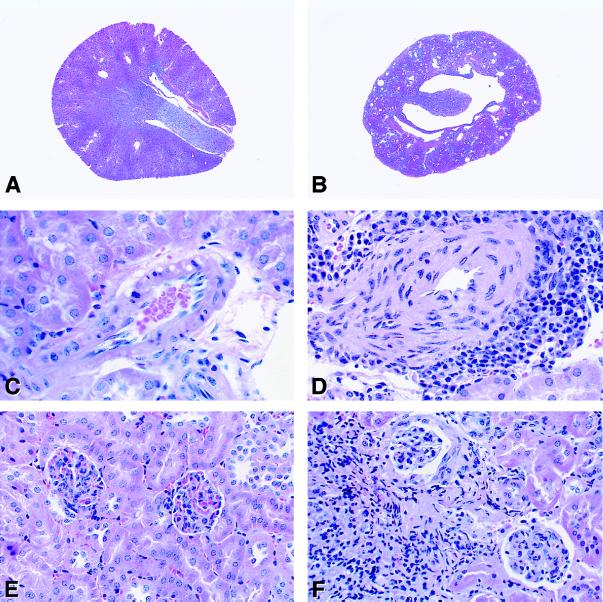
Kidney histomorphology of wild-type and Agtr1a −/−Agtr1b −/− mice. (A and B): Axial sections from wild-type (A) and Agtr1a−/−Agtr1b −/− mice (B). (×5.) There is marked atrophy of the papilla in the AT1-deficient kidney compared with the control. (C and D): Cross sections of representative small arteries in kidneys from wild-type (C) and Agtr1a −/−Agtr1b −/− (D) mice. The media of the vessels from the mutant animals was markedly thickened and many of the vessels were surrounded by inflammatory cells as shown. (×150.) (E and F): Sections of renal cortex from wild-type (E) and Agtr1a−/−Agtr1b −/− (F) mice. In the AT1-deficient mice there were focal areas with tubular dropout and inflammatory cell infiltration. (×100.)
These data suggest that both AT1 receptor isoforms contribute to the regulation of vascular structures in the kidney and to the maintenance of the renal papilla. Furthermore, as with the growth responses, the absence of a single AT1 receptor isoform can be compensated by the other. However, the AT1A receptors have a greater capacity for compensation than the AT1B receptors, perhaps reflecting their relative levels of expression in kidney (15, 31). Accordingly, renal morphology is completely normal in the Agtr1b −/− mice that possess the full complement of AT1A receptors. In contrast, subtle abnormalities can be detected in kidneys of AT1A-deficient mice. By using magnetic resonance microscopy, modest reductions in the size of the renal papilla are revealed in Agtr1a −/− mice that were not detected by routine pathological evaluation [Oliverio et al., unpublished work]. In addition, minimal but significant thickening of renal interlobular arteries has been detected in Agtr1a −/− mice by using a careful morphometric analysis (18). Nonetheless, the difference between the modest abnormalities seen in Agtr1a −/− mice and the florid pathology seen in double homozygous Agtr1a −/−Agtr1b −/− mice must be due to compensation by the AT1B receptor. This level of compensation seems remarkable because our previous studies have shown that AT1B receptor binding is virtually undetectable in kidneys of Agtr1a −/− mice (15). Alternatively, it has been hypothesized that atrophy of the papilla may not depend directly on expression of AT1 receptors in the kidney, but instead is related to loss of AT1 receptor functions in the urinary collecting system (32).
AT1 Receptors and Urinary Concentration.
Among its many biological actions, the RAS regulates water metabolism, in part through diverse effects on urinary concentrating mechanisms (33, 34). Whereas a range of abnormalities in water homeostasis has been described in mice lacking angiotensin II or AT1A receptors, impairment of urinary concentrating capacity is a feature common to all of these mutants (10, 35–37). To clarify the role of AT1A and AT1B receptors in these processes, we measured urinary concentrating capacities of wild-type, Agtr1a −/−, Agtr1b −/−, and Agtr1a −/−Agtr1b −/− mice. As shown in Fig. Fig.4,4, after 12 hours of water deprivation, urine osmolalities in Agtr1b −/− mice increased to the same extent as in wild-type controls. In contrast, Agtr1a −/− mice have a defect in urinary concentration that is clearly apparent after 12 hours of water deprivation. Furthermore, the combined absence of both AT1A and AT1B receptors causes a urinary concentrating defect that is even more severe. Their urine osmolality remains virtually unchanged after 12 hours of water deprivation. Whereas this severe defect in the Agtr1a −/−Agtr1b −/− mice is not unexpected given their inner medullary atrophy (see Fig. Fig.33B), the incremental differences in maximal urine osmolality between the three mutant groups further illustrate the relative abilities of the AT1A and AT1B receptor isoforms to compensate for one another.
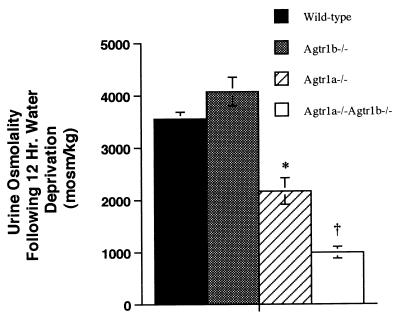
Urine osmolality following 12 hours of water deprivation. The experimental groups are represented as follows: open bar is Agtr1a−/−Agtr1b −/− group, gray bar is theAgtr1b− −/− group, hatched bar is the Agtr1a− −/−group, and the black bar is the wild-type group. (P < 0.0003 vs. wild-type or Agtr1b −/−; †P < 0.0001 vs. wild-type or Agtr1b −/−, P = 0.004 vs. Agtr1a −/−.
Vasoconstrictor Actions of Angiotensin II.
The vasoconstrictor actions of angiotensin II are mediated by AT1 receptors (2). We have previously shown that the AT1A receptor mediates a substantial portion of the pressor effects of angiotensin II based on the marked attenuation of these responses in Agtr1a −/− mice (15). However, when endogenous angiotensin II production is suppressed, a modest pressor and renal vasoconstrictor effect of angiotensin II can be detected in AT1A-deficient mice. This response is blocked by AT1 receptor antagonists, but not by sympatholytic agents, suggesting that it is a direct effect of AT1B receptors (23, 38).
To directly test this hypothesis and to clarify the role of other angiotensin receptors in the vasoconstrictor actions of angiotensin II, we examined pressor responses to angiotensin II in wild-type, Agtr1b −/− and Agtr1a −/−Agtr1b −/− mice. As shown in Fig. Fig.5,5, angiotensin II caused similar dose-proportional increases in mean arterial pressure in the wild-type and Agtr1b −/− mice. In contrast, angiotensin II had no discernable effect on MAP in the double homozygous Agtr1a −/−Agtr1b −/− mice. To determine whether responses to other pressor agents might also be impaired in these animals, each group received an infusion of epinephrine after the angiotensin II infusions were completed. As shown in Fig. Fig.5,5, epinephrine caused vasoconstriction with significant and equivalent increases in MAP in all of the groups, including the Agtr1a −/−Agtr1b −/− mice. These studies verify the importance of AT1 receptors in the vasoconstrictor effects of angiotensin II. In addition, they confirm the conclusions from our previous study that the vasoconstrictor actions of angiotensin II in Agtr1a −/− mice are mediated by AT1B receptors (23). Finally, the absence of any hemodynamic response to angiotensin II in Agtr1a −/−Agtr1b −/− mice suggests that there are no other angiotensin II receptors with significant vasoactive functions.
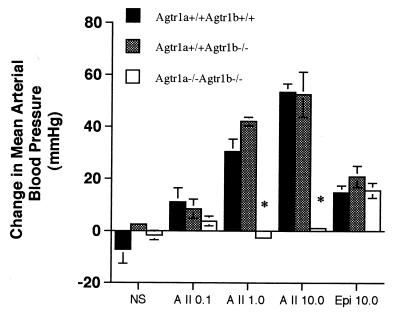
Hemodynamic effects of angiotensin II in enalapril-pretreated, anesthetized wild-type, Agtr1b −/−, and Agtr1a −/− Agtr1b −/− mice. The changes in MAP at 120 seconds after injection of vehicle, 0.1, 1.0, and 10 μg/kg of angiotensin II, or 10 μg/kg epinephrine are shown. The experimental groups are represented as follows: open bars are the Agtr1a −/−Agtr1b −/− group, gray bars are the Agtr1b −/− group, and black bars are the wild-type group (P < 0.0003 vs. wild-type or Agtr1b−/− groups).
Systolic Blood Pressure Regulation in Mice Lacking AT1A and AT1B Receptors.
Systolic blood pressures in Agtr1b −/− and wild-type mice were similar (114 ± 3 vs. 123 ± 3 mmHg (1 mmHg = 133 Pa). In contrast, blood pressure was significantly reduced in mice lacking both AT1A and AT1B receptors (87 ± 3 mmHg; P < 0.0001 vs. wild-type or Agtr1b −/−), as shown in Fig. Fig.6.6. This magnitude of blood pressure reduction is similar to levels that we have observed previously in Agtr1a −/− (15) and Agt −/− mice (11).
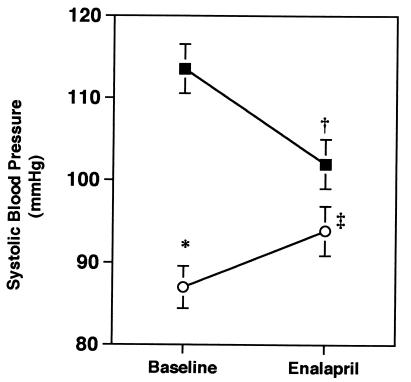
The effect of chronic ACE inhibition on systolic blood pressure in conscious mice. Systolic blood pressures were measured daily for 10 days (Baseline) followed by an additional 10-day period while mice were treated with enalapril 30 mg/kg per day in drinking water (Enalapril). Data are mean ± SEM. The black squares represent the wild-type group and the open circles represent the Agtr1a −/−Agtr1b −/− group. (P < 0.0001 vs. wild-type at baseline; †P < 0.002 vs. baseline for wild-type; ‡P < 0.03 vs. baseline for Agtr1a −/−Agtr1b −/−).
To determine the contribution of endogenous angiotensin II to the maintenance of blood pressure in these animals, each group was subsequently treated with the ACE inhibitor enalapril for 2 weeks, during which time systolic blood pressure was monitored. As shown in Fig. Fig.6,6, the ACE inhibitor significantly lowered blood pressures in the wild-type animals. In contrast, ACE inhibition had a paradoxical effect on blood pressure in the mice lacking both AT1A and AT1B receptors. In these mice, administration of the ACE inhibitor caused a significant rise in blood pressure from 87 ± 3 to 94 ± 3 mmHg (P = 0.03). Following ACE inhibition, systolic blood pressures in the Agtr1a −/−Agtr1b −/− mice were not significantly different from the wild-type mice on enalapril. We speculate that the paradoxical effect of ACE inhibition to increase blood pressure in Agtr1a −/−Agtr1b −/− mice represents inhibition of AT2 receptor signaling. This would be consistent with studies suggesting that AT2 receptors oppose the actions of AT1 receptors on blood pressure (16, 17) and other cellular functions (39). Because angiotensin II infusions do not alter blood pressure in Agtr1a −/−Agtr1b −/− mice (see Fig. Fig.4),4), AT2 receptors may regulate blood pressure by modulating renal sodium excretion rather than by affecting vascular tone.
Acknowledgments
The authors thank Norma B. Turner for secretarial and administrative help and Kamie Snow for expert technical assistance. We also thank Drs. William Arendshorst and Paul Klotman for their critical review of the manuscript. These studies were supported by the National Institutes of Health (HL58554, HL49277, DK38108, and GM20069) and the Research Service of the Department of Veterans Affairs.
ABBREVIATIONS
RAS | renin–angiotensin system |
AT1 | type 1 angiotensin receptor |
ES | embryonic stem |
ACE | angiotensin converting enzyme |
MAP | mean arterial blood pressure |
RT-PCR | reverse transcription—PCR |
References
Articles from Proceedings of the National Academy of Sciences of the United States of America are provided here courtesy of National Academy of Sciences
Full text links
Read article at publisher's site: https://doi.org/10.1073/pnas.95.26.15496
Read article for free, from open access legal sources, via Unpaywall:
https://europepmc.org/articles/pmc28071?pdf=render
Citations & impact
Impact metrics
Citations of article over time
Alternative metrics
Smart citations by scite.ai
Explore citation contexts and check if this article has been
supported or disputed.
https://scite.ai/reports/10.1073/pnas.95.26.15496
Article citations
RAAS-deficient organoids indicate delayed angiogenesis as a possible cause for autosomal recessive renal tubular dysgenesis.
Nat Commun, 14(1):8159, 09 Dec 2023
Cited by: 3 articles | PMID: 38071212 | PMCID: PMC10710424
Appraising the Preclinical Evidence of the Role of the Renin-Angiotensin-Aldosterone System in Antenatal Programming of Maternal and Offspring Cardiovascular Health Across the Life Course: Moving the Field Forward: A Scientific Statement From the American Heart Association.
Hypertension, 80(5):e75-e89, 23 Mar 2023
Cited by: 8 articles | PMID: 36951054 | PMCID: PMC10242542
Review Free full text in Europe PMC
Is the anti-aging effect of ACE2 due to its role in the renin-angiotensin system?-Findings from a comparison of the aging phenotypes of ACE2-deficient, Tsukuba hypertensive, and Mas-deficient mice.
Hypertens Res, 46(5):1210-1220, 14 Feb 2023
Cited by: 3 articles | PMID: 36788301 | PMCID: PMC9925940
Review Free full text in Europe PMC
Angiotensin II Type 1A Receptor Expressed in Smooth Muscle Cells is Required for Hypertensive Vascular Remodeling in Mice Infused With Angiotensin II.
Hypertension, 80(3):668-677, 11 Jan 2023
Cited by: 10 articles | PMID: 36628961 | PMCID: PMC9931681
Angiotensin II type 1a receptor deficiency alleviates muscle atrophy after denervation.
Sci Rep, 13(1):519, 10 Jan 2023
Cited by: 1 article | PMID: 36627369 | PMCID: PMC9832142
Go to all (210) article citations
Data
Similar Articles
To arrive at the top five similar articles we use a word-weighted algorithm to compare words from the Title and Abstract of each citation.
Murine double nullizygotes of the angiotensin type 1A and 1B receptor genes duplicate severe abnormal phenotypes of angiotensinogen nullizygotes.
J Clin Invest, 101(4):755-760, 01 Feb 1998
Cited by: 203 articles | PMID: 9466969 | PMCID: PMC508622
Angiotensin II responses in AT1A receptor-deficient mice: a role for AT1B receptors in blood pressure regulation.
Am J Physiol, 272(4 pt 2):F515-20, 01 Apr 1997
Cited by: 93 articles | PMID: 9140053
Targeting deletion of angiotensin type 1B receptor gene in the mouse.
Am J Physiol, 272(3 pt 2):F299-304, 01 Mar 1997
Cited by: 81 articles | PMID: 9087671
A genetic approach for studying the physiology of the type 1A (AT1A) angiotensin receptor.
Semin Nephrol, 17(5):404-411, 01 Sep 1997
Cited by: 7 articles | PMID: 9316208
Review
Funding
Funders who supported this work.
NHLBI NIH HHS (3)
Grant ID: HL49277
Grant ID: HL58554
Grant ID: R01 HL049277
NIDDK NIH HHS (2)
Grant ID: P01 DK038108
Grant ID: DK38108
NIGMS NIH HHS (2)
Grant ID: R01 GM020069
Grant ID: F31 GM020069