Abstract
Free full text

Macrophage Receptors for Influenza A Virus: Role of the Macrophage Galactose-Type Lectin and Mannose Receptor in Viral Entry
Abstract
Although sialic acid has long been recognized as the primary receptor determinant for attachment of influenza virus to host cells, the specific receptor molecules that mediate viral entry are not known for any cell type. For the infection of murine macrophages by influenza virus, our earlier study indicated involvement of a C-type lectin, the macrophage mannose receptor (MMR), in this process. Here, we have used direct binding techniques to confirm and characterize the interaction of influenza virus with the MMR and to seek additional macrophage surface molecules that may have potential as receptors for viral entry. We identified the macrophage galactose-type lectin (MGL) as a second macrophage membrane C-type lectin that binds influenza virus and is known to be endocytic. Binding of influenza virus to MMR and MGL occurred independently of sialic acid through Ca2+-dependent recognition of viral glycans by the carbohydrate recognition domains of the two lectins; influenza virus also bound to the sialic acid on the MMR. Multivalent ligands of the MMR and MGL inhibited influenza virus infection of macrophages in a manner that correlated with expression of these receptors on different macrophage populations. Influenza virus strain A/PR/8/34, which is poorly glycosylated and infects macrophages poorly, was not recognized by the C-type lectin activity of either the MMR or the MGL. We conclude that lectin-mediated interactions of influenza virus with the MMR or the MGL are required for the endocytic uptake of the virus into macrophages, and these lectins can thus be considered secondary or coreceptors with sialic acid for infection of this cell type.
Infection of host cells by influenza virus is initiated by attachment of virus to sialic acid residues on the host cell surface through the receptor-binding site at the distal tip of the viral hemagglutinin (HA) (43). After attachment, the virus is internalized by endocytosis, and acidification of the endosome triggers a conformational change in viral HA that results in fusion of the viral envelope and host cell membrane (34). At the cell surface, sialic acid residues are commonly found at the termini of oligosaccharide chains that are attached in O or N linkage to cell surface proteins; they are also an essential component of acidic glycosphingolipids (gangliosides) that are present in all mammalian cell membranes. Although the abundance of sialic acid on mammalian cells provides influenza virus with multiple potential receptors, virus attachment does not always lead to virus entry (5, 8, 46). Furthermore, sialic acid-independent infection of Madin-Darby canine kidney (MDCK) cells by influenza virus has been reported (35). The specific host cell molecules that serve as functional receptors (or coreceptors) for the infectious entry of influenza virus have yet to be defined.
We have studied the infectious entry of influenza virus into macrophages (M), which represents an early event in recognition of the virus by the innate immune system (23, 44). After intranasal infection of mice, influenza virus replicates productively in cells of the respiratory epithelium. M
are also infected and viral proteins are produced, but replication is abortive and no live progeny are released (32); infection of M
is thus a dead-end for the virus leading to a reduction in viral load. In addition, influenza virus infection of M
stimulates production and release of proinflammatory cytokines and alpha/beta interferon (28), which may assist in further limiting viral replication and spread within the respiratory tract. Depletion of airway M
from mice prior to intranasal influenza virus infection leads to increased virus titers in the lung, attesting to the important role of M
in early host defense against the virus (38, 44).
We observed in a previous study (30) that influenza A virus strains differed in their ability to infect murine M, strains carrying a more highly glycosylated hemagglutinin (HA) molecule being more efficient at infecting M
than less glycosylated strains, although binding of viruses to the M
cell surface was equivalent. Our investigation of this phenomenon indicated involvement of the M
mannose receptor MMR (CD206), a C-type lectin, in infectious viral entry (29, 30). The involvement of other receptors was not excluded, and our subsequent observation that influenza virus can infect the RAW 264.7 M
cell line, which does not express the MMR, indeed points to the existence of other routes of infectious entry of the virus into M
.
In the present study we used direct binding methods to confirm and characterize the interaction of influenza virus with the MMR and to seek additional M surface molecules that may have potential as receptors for viral entry. We identify the M
galactose-type lectin (MGL) as a second M
membrane C-type lectin that binds influenza virus and investigate its involvement in the infectious process.
MATERIALS AND METHODS
Viruses.
The influenza A viruses used were A/Guangdong/25/93 (H3N2) (Guangdong/93), A/HKx31 (H3N2), and the Mt. Sinai strain of A/PR/8/34 (H1N1) (PR8). HKx31 is a high growth reassortant of PR8 with A/Aichi/2/68 (H3N2) that bears the H3N2 surface glycoproteins (2). Viruses were grown in the allantoic cavity of 10-day embryonated hen's eggs by standard procedures and infectivity titers were determined by plaque assay on MDCK cells (1). For binding studies, viruses were concentrated from allantoic fluid by precipitation with 8% (wt/vol) polyethylene glycol 6000 (BDH Chemicals) and purified by rate zonal sedimentation on 25 to 70% (wt/vol) sucrose gradients. Purified Guangdong/93 virus was obtained from Michael Hocart, Influenza Process Development, CSL, Ltd., Melbourne, Australia. For the virus precipitation assay, Guangdong/93 virus was fixed for 4 min with 0.2% glutaraldehyde at 37°C. The glutaraldehyde was quenched with 0.5 vol of 2 M glycine, the fixed virus was dialyzed extensively with Tris-buffered saline (TBS; 0.05 M Tris-HCl, 0.15 M NaCl [pH 7.2]), and aggregates were removed by centrifugation (10,000 × g, 5 min).
Cells.
The murine M cell lines J774E (a mutant line of J774 M
selected for enhanced expression of MR [11]) and RAW 264.7 were maintained in α-minimal essential medium (Gibco) supplemented with 2 mM glutamine, 2 mM pyruvate, 30 μg of gentamicin per ml, and 10% fetal calf serum (FCS) (αMEM10), with 60 mM thioguanine added to media for J774E M
s. MDCK cells and LA-4 cells, a murine airway epithelial cell line, were passaged in RPMI 1640 supplemented with 2 mM glutamine, 2 mM pyruvate, 30 μg of gentamicin per ml, and 10% FCS (RF10). Resident alveolar M
from C57BL/6 mice were obtained and cultured as previously described (30).
Preparation of M
cell lysates.
M grown to semiconfluence in 80-cm2 tissue culture flasks (1 × 106 to 3 × 106 cells per flask) were rinsed twice with ice-cold phosphate-buffered saline (PBS) and harvested with cell scrapers. The cells were washed three times by centrifugation, resuspended in 0.5 ml of TBS on ice, and then mixed with an equal volume of 2× lysis buffer (final concentration; 50 mM Tris-HCl [pH 7.5], 150 mM NaCl, 0.5% Triton X-100, 1 mM CaCl2, 1 mM MgCl2) and protease inhibitor cocktail (EDTA-free complete inhibitor from Boehringer Mannheim). After 1 h on ice, the lysate was clarified by centrifugation for 3 min at 10,000 g. Final protein concentrations of the lysates ranged from 1-3 mg ml−1.
Sialidase treatment.
M cell lysates (100 μl) were treated with 1 μl (5 mU) of neuraminidase from Vibrio cholerae type III (Sigma) at 37°C for 4 to 6 h. The reaction was terminated by adding an equal volume of 2× nonreducing sodium dodecyl sulfate (SDS) sample buffer (final concentration = 2% SDS, 10% glycerol, 0.005% bromophenol blue, 62.5 mM Tris-HCl [pH 6.9]).
Cell surface labeling.
M were grown to semiconfluence in 80-cm2 tissue culture flasks, rinsed three times with ice-cold PBS containing 1 mM CaCl2 and 1 mM MgCl2 (PBS-Ca2+-Mg2+), and surface labeled by incubation for 30 min on ice with 0.5 mg ml−1 EZ-Link Sulfo-NHS-biotin (Pierce) in PBS-Ca2+-Mg2+; 5 ml per flask. Buffer was removed, and a second 5 ml of biotin solution was added for 30 min. Unreacted biotin was quenched with PBS-Ca2+-Mg2+ containing 0.1 M glycine (10 ml per flask), and cell lysates were prepared as described above.
Primary antibodies.
Monoclonal antibodies (MAb) specific for the HA of HKx31 and PR8 influenza viruses were generated within the Department of Microbiology and Immunology, University of Melbourne. Hyperimmune serum to Guangdong/93 virus was prepared in BALB/c mice by two intraperitoneal inoculations, 3 weeks apart, of virus in allantoic fluid. Polyclonal antiserum to MMR was generously provided by Philip D. Stahl, Washington University School of Medicine, St. Louis, MO, and biotin-labeled MAb MCA-2235B (anti-murine MMR) was purchased from Serotec, Oxford, United Kingdom. MAbs LOM-8.7 and LOM-14, specific for mouse MGL, were produced in the Laboratory of Cancer Biology and Molecular Immunology, The University of Tokyo, Tokyo, Japan (19). MAb MA-040 specific for the nucleoprotein (NP) of influenza A virus was provided by the WHO Collaborating Centre for Reference and Research on Influenza, Melbourne, Australia.
Virus overlay protein-binding assay (VOPBA).
M proteins were separated by SDS-PAGE under nonreducing conditions. Proteins were not boiled prior to electrophoresis with the exception of the MMR immunoprecipitates. After electrotransfer of M
proteins to polyvinylidene difluoride (PVDF) membranes, the blots were incubated for 16 h at 4°C on a rocking platform in binding buffer (BB; 1% bovine serum albumin, 154 mM NaCl, 0.05% Tween 20, 1 mM CaCl2, 1 mM NiCl2) to block nonspecific interactions and allow renaturation of blotted proteins (15). For virus binding and subsequent steps, BB containing an additional 5 mM Ca2+ (BB-Ca2+) was used as diluent and for washes, and all steps were conducted at 4°C to inhibit viral neuraminidase activity. Blots were incubated for 6 to 16 h with purified influenza virus (2 to 10 μg ml−1), rinsed twice, and washed three times, each time for 10 min.
To detect influenza virus bound to the PVDF membrane, antibody specific for the virus strain was added to the blot for 2 to 3 h, followed by multiple washes and incubation with horseradish peroxidase-conjugated rabbit anti-mouse immunoglobulin (Dako). After washing, the membranes were moved to room temperature, and a peroxidase substrate was immediately added. To study the effect of EDTA or monosaccharides on virus binding, these were included during the virus binding step and in the washes immediately prior to and subsequent to incubation of virus on the blot. Blots were developed by standard methods using chemiluminescence or diaminobenzidine and scanned, and the images were viewed with Adobe Photoshop software.
Immunoblots and detection of biotinylated proteins on blots.
Nonfat milk powder in PBS containing 0.1% Tween 20 was used for blocking, washing, and the dilution of antibodies. MMR was detected with polyclonal anti-MMR IgG, and MGL was detected with monoclonal anti-MGL IgG (LOM-8.7), followed by horseradish peroxidase-conjugated secondary antibodies. Biotinylated proteins were detected by incubating blots with peroxidase-conjugated streptavidin (Silenus). Bound antibody was detected by chemiluminescence.
Immunoprecipitation and affinity chromatography.
To immunoprecipitate the MMR, J774E cell lysate was first precleared by incubation with protein G-Sepharose (Amersham Pharmacia Biotech) for 2 h at 4°C, followed by removal of the Sepharose beads by centrifugation. Rabbit anti-MMR IgG (~3 μg of IgG per 250 μg of cellular protein) and additional protein G-Sepharose were added to the lysate, and the mixture was incubated on a rotating platform at 4°C for 16 h. Immune complexes bound by protein G-Sepharose were collected by centrifugation, washed extensively with lysis buffer, boiled in 50 μl of nonreducing SDS-sample buffer at 95°C for 10 min, and analyzed by VOPBA.
To isolate MGL by affinity chromatography, asialofetuin (ASF; Sigma) was coupled to CNBr-Sepharose 4B (Amersham Pharmacia Biotech) according to the manufacturer's instructions using 5 mg of ASF per ml of packed beads. J774E M lysate was treated with 5 mM EDTA to release endogenous ligands from MGL before addition to a 1/3 volume of ASF-Sepharose and recalcification with 20 mM CaCl2. After incubation, the Sepharose was collected, washed, and suspended in 0.3 M d-galactose in lysis buffer to elute J774E proteins.
Viral precipitation of M
proteins.
M lysate was prepared from biotin surface-labeled J774E cells and 1 ml of lysate (1 to 2 mg of total protein) was diluted to 5 ml with chilled lysis buffer. The suspension was clarified by ultracentrifugation at 100,000 × g for 45 min at 4°C (Beckman Optima series, Beckman Coulter) using a swinging-bucket rotor (SW55 Ti). To 1-ml aliquots of clarified lysate, 100 μg of glutaraldehyde-fixed influenza virus (Guangdong/93) was added, and the suspension was adjusted to contain 10 mM CaCl2 or 5 mM EDTA (pH 7). Tubes containing virus only or clarified lysate only in the presence of 10 mM CaCl2 served as negative controls. After incubation at 4°C for 2 h on a rotating platform to allow interaction of virus with cellular molecules, the contents were diluted to 5 ml with lysis buffer (with or without supplemental CaCl2 or EDTA, as used during the incubation period) and centrifuged at 100,000 × g for 45 min at 4°C to pellet the virus and any bound cellular proteins. The precipitated virus complexes were washed three times with lysis buffer by sequential resuspension and ultracentrifugation and then heated at 95°C for 5 min in 50 μl of nonreducing sample buffer and subjected to SDS-PAGE.
Detection of cell surface MMR and MGL by flow cytometry.
Aliquots of 106 cells were incubated on ice for 20 min with supernatant from hybridoma 2.4G2 to block Fc receptors and then stained for 30 min on ice with biotin-labeled MAbs to either MMR (MCA-2235B) or MGL (LOM-14) or with appropriate biotin-labeled isotype control MAbs in TBS containing 5 mM EDTA and supplemented with 10% normal mouse serum. After a washing step, bound MAbs were detected with streptavidin conjugated to allophycocyanin, and the cells were analyzed on a FACSCalibur flow cytometer (BD Biosciences) collecting data on 20,000 cells. The data were analyzed by using the FlowJo program (Treestar).
Infection of M
and epithelial cells by influenza virus.
Eight-well chamber slides (Lab-Tek, Nunc) were seeded with M or epithelial cells at 2 × 105 cells/ml in 250 μl, followed by incubation overnight. The resulting monolayers were washed and incubated for 1 h at 37°C with 106 PFU of influenza virus in 300 μl of serum-free medium. Unadsorbed virus was removed, and the cells were incubated for a further 6 to 8 h. The cells were then washed, fixed in acetone, and stained with a MAb specific for the nucleoprotein of type A influenza viruses, followed by fluorescein isothiocyanate-conjugated sheep anti-mouse immunoglobulin, as previously described (30). Fluorescent and total cell numbers were determined in four fields (>200 cells in total), and the percentage of fluorescent cells was determined. To test the effect of mannan or ASF on viral infection, cells were preincubated for 30 min at 37°C in 200 μl of serum-free medium containing either compound at 1.5 times its final concentration, before the addition of 106 PFU of virus in 100 μl. Mannan or ASF were included in all washing and culture steps of the experiments.
Statistical analysis.
When comparing three or more sets of values, the data were analyzed by one-way analysis of variance (ANOVA; nonparametric), followed by post-hoc analysis using Tukey's multiple comparison test.
RESULTS
Interaction of influenza virus with the MMR.
Our previous studies had implicated the MMR, a C-type lectin, as a major endocytic receptor in the infectious entry of influenza virus into murine M (30). In seeking to confirm a direct interaction between virus and MMR, we carried out a VOPBA in which lysate from J774E M
was resolved by SDS-PAGE and probed with influenza A virus HKx31 in the presence of Ca2+. Detection of bound virus revealed its interaction with numerous proteins, with particularly intense binding to a molecule that migrated with an apparent molecular mass of 175 kDa (Fig. (Fig.1A).1A). The mobility of this protein correlated with that of MMR, as evidenced by comparing duplicate blots that had been probed with virus and with polyclonal anti-MMR IgG (Fig. (Fig.1B).1B). The distinct 175-kDa virus-binding band was not observed in VOPBA of lysate from the RAW 264.7 M
cell line, which does not express the MMR (12). Immunoprecipitation of MMR from the J774E lysate led to marked depletion of the 175-kDa virus-binding protein, and virus bound strongly to MMR recovered from the immunoprecipitate (Fig. (Fig.1C1C).
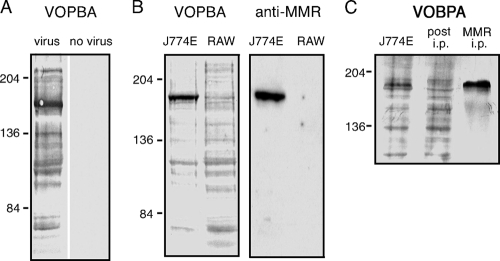
Use of VOPBA to determine direct interaction between influenza virus and the MMR. (A) Whole-cell lysate from the murine M-like cell line J774E was electrophoresed by SDS-7% PAGE under nonreducing conditions, transferred to PVDF membrane, and probed with influenza virus strain HKx31. Virus was omitted from the diluent as a control for the specificity of virus binding. (B) Lysates from J774E and RAW 264.7 (RAW) cells were analyzed by VOPBA with HKx31 virus as described above. A duplicate blot was stained with polyclonal antibody raised against the MMR. (C) MMR was immunoprecipitated from J774E lysate (MMR i.p.) and resolved by SDS-7% PAGE alongside total J774E lysate precleared with protein G-Sepharose (J774E), and the lysate postimmunoprecipitation (post i.p.). A VOPBA with virus strain HKx31 was performed. The approximate molecular mass positions are indicated in kilodaltons.
Sialic acid represents the primary receptor for influenza virus, and the MMR is known to be sialylated (20). To determine whether virus binding to MMR was dependent on sialic acid, J774E lysate was treated with V. cholerae neuraminidase (sialidase) prior to separation by SDS-PAGE and VOPBA. As expected, virus binding to the majority of M proteins was abolished after sialidase treatment (Fig. (Fig.2A,2A, HKx31 virus; Fig. Fig.2B,2B, Guangdong/93 virus). In contrast, desialylation of the MMR, which was accompanied by a slight increase in its mobility on SDS-PAGE (Fig. (Fig.2C),2C), did not eliminate virus binding (Fig. 2A and B). Binding of virus to the desialylated MMR was abrogated in the presence of EDTA (Fig. (Fig.2B,2B, lane 4), a finding consistent with Ca2+-dependent recognition of viral carbohydrate by the C-type lectin domains of the MMR. Interestingly, EDTA reduced but did not eliminate binding of virus to the untreated MMR (Fig. (Fig.2B,2B, lane 3). This suggests that the interaction of virus with native MMR occurs in two ways, through binding of the viral HA to sialic acid on MMR, as well as by recognition of oligosaccharide on the viral glycoproteins by the C-type lectin activity of the MMR. The strong binding of virus to immunoprecipitated MMR observed in Fig. Fig.1C1C likely reflects binding to sialic acid on MMR, since boiling of the immunoprecipitate prior to VOPBA would be expected to destroy lectin activity of the MMR.
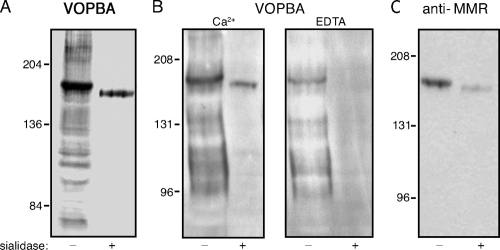
Mode of interaction of influenza virus with the MMR. J774E M proteins were sialidase-treated (+) or not treated (−) prior to electrophoresis on SDS-7% PAGE and electrotransfer. Blots were probed with influenza virus in a VOPBA (A and B) or immunoblotted with anti-MMR IgG (C). The viruses used in the VOPBA were HKx31 (A) and Guangdong/93 (B), and the buffer used was BB-Ca 2+ buffer as described in Materials and Methods, except for the lanes marked “EDTA,” in which the virus-binding step was carried out in BB buffer lacking CaCl2 and supplemented with 5 mM EDTA. The approximate molecular mass positions are indicated in kilodaltons.
Influenza virus binds to MGL.
To investigate virus-binding proteins of lower molecular mass, a higher concentration of acrylamide (10%) was used for SDS-PAGE and subsequent VOPBA of J774E lysate. This revealed Guandong/93 virus binding to a 44-kDa protein band and, in desialylated lysate, to a protein species of slightly faster mobility (Fig. (Fig.3A).3A). The possible involvement of another lectin was considered, a candidate being the M galactose-type lectin (MGL, known previously as galactose/N-acetylgalactosamine-specific C-type lectin), which is an integral membrane glycoprotein with a reported molecular mass of 42 kDa (17, 19). In a duplicate blot probed with antibody specific to MGL, the mobilities of these virus-binding bands in native and desialylated lysates were indeed shown to correspond to those of MGL (Fig. (Fig.3A3A).
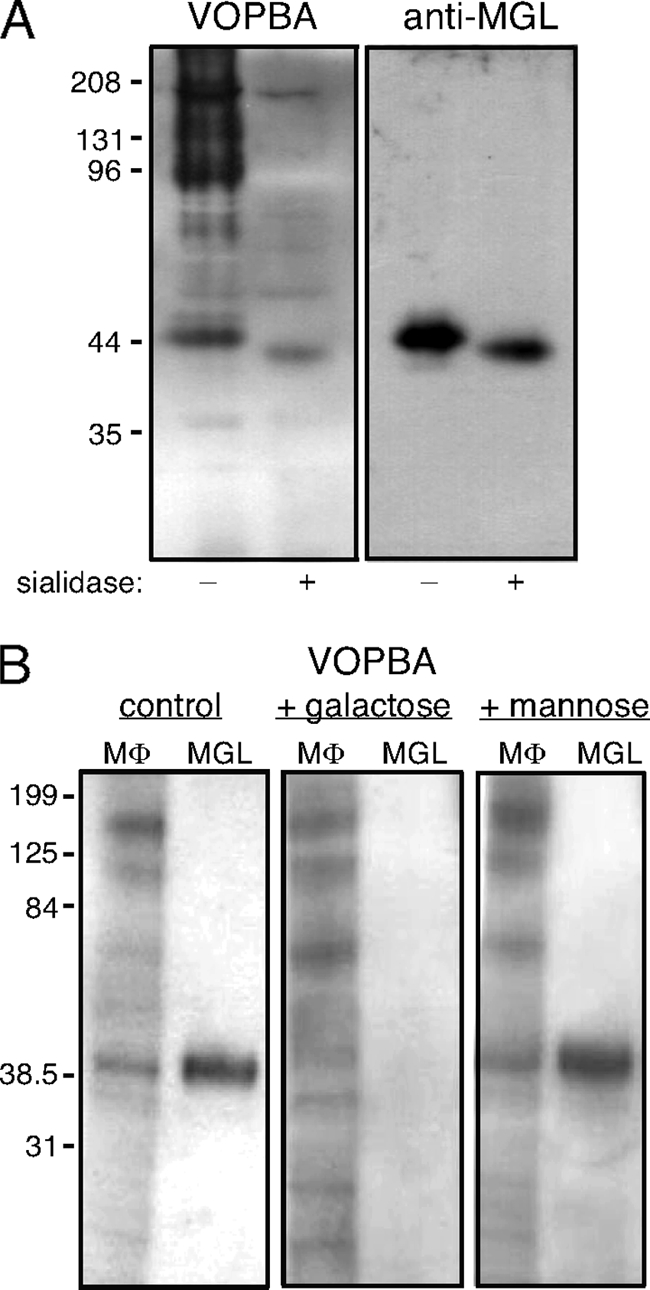
Influenza virus binds to MGL. (A) Comparison of M proteins recognized by influenza virus and by anti-MGL antibody. J774E M
lysate that had been sialidase-treated (+) or not treated (−) was resolved in duplicate by SDS-10% PAGE, and blots were probed with virus (Guangdong/93) in a VOPBA or immunoblotted with MAb LOM-8.7 to detect MGL. (B) MGL was isolated from J774E M
by affinity chromatography on ASF (MGL) and resolved by SDS-10% PAGE alongside J774E cell lysate (M
). The binding of Guangdong/93 virus was examined in VOPBA using virus binding buffer NiBB-Ca2+ (control) or the same buffer supplemented with 0.1 M galactose or 0.1 M mannose. The approximate molecular mass positions are indicated in kilodaltons.
Like the hepatic asialoglycoprotein receptor, MGL functions as an endocytic receptor for unsialylated glycoproteins (17, 26). To isolate MGL, J774E M cell lysate was subjected to affinity chromatography using immobilized ASF and elution with galactose as described in Materials and Methods. Subsequent SDS-PAGE and immunoblotting with antibody to MGL confirmed the recovery in the eluate of MGL as a 44-kDa protein (data not shown).
In VOBPA, binding of influenza virus to MGL was abrogated in the presence of 0.1 M d-galactose but not d-mannose, as shown in Fig. Fig.3B3B for both MGL in the whole J774E lysate and isolated MGL. Binding of virus to MGL thus appears to be mediated solely through the galactose-specific C-type lectin activity of MGL, there being no evidence for additional binding through sialic acid as had been shown to occur for the interaction of influenza virus with MMR.
Analysis of cell surface proteins precipitated by influenza virus from M
lysates.
Being endocytic receptors, only a fraction of MMR and MGL molecules are expressed at the cell surface at any one time, the remainder being located intracellularly (22, 45). Since the VOPBA blots described above were conducted with whole-cell lysates, some of the observed binding of virus would have been to lectin molecules derived from inside the cell. To confirm that influenza virus could bind to cell surface-expressed MMR and MGL, J774E cells were surface labeled with biotin prior to lysis, the lysate was mixed with influenza virus and subjected to ultracentrifugation, and the precipitate was analyzed for biotinylated molecules that had coprecipitated with the virus. In order to stabilize the virus in the presence of detergent in the M lysate, glutaraldehyde-fixed virus was used for this experiment. Among the biotinylated proteins precipitated by Guangdong/93 virus were two major species, of masses ~175 and ~44 kDa, whose precipitation was abrogated in the presence of EDTA (Fig. (Fig.4).4). By immunoblotting with specific antibodies, these proteins were confirmed to be MMR and MGL, respectively (data not shown).

Coprecipitation of M cell surface proteins with influenza virus after ultracentrifugation. J774E cells were surface labeled with biotin prior to cell lysis. Influenza virus (Guangdong/93) was incubated with the lysate in the presence of 10 mM Ca2+ or 5 mM EDTA and then precipitated by ultracentrifugation and washed. Final pellets were boiled and then resolved by SDS-12% PAGE and blotted with streptavidin. Samples of biotinylated lysate only (M
) and virus only (virus) were subjected to the same treatment and served as controls. The approximate molecular mass is indicated in kilodaltons.
Infection of M
by influenza virus is blocked by ligands of MGL and MMR.
We had shown previously that infection of M by influenza virus was blocked at a postbinding step by yeast mannan, a ligand of MMR, suggesting a role for MMR in viral entry (30). The RAW 264.7 M
cell line lacks MMR expression and yet is readily infected by influenza virus. To investigate a possible role for MGL in viral entry, we examined the effect of ASF, a multivalent ligand of MGL, together with mannan, on influenza virus infection of J774E M
(MMR+, MGL+); RAW 264.7 M
(MMR−, MGL+); and LA-4, a mouse respiratory epithelial cell line that lacks expression of either receptor (Fig. (Fig.5A5A).

Expression of MMR and MGL by M correlated with ability of mannan and/or ASF to inhibit infection by influenza virus. (A) Expression of MMR and MGL on J774E M
, RAW 264.7 M
(RAW) or LA-4 epithelial cells. Cells were stained with biotin-labeled MAbs to MMR or MGL (shaded histograms) or with appropriate biotin-labeled isotype control MAbs (open histograms). A minimum of 20,000 cells were collected for analysis. (B) Monolayers of J774E M
, RAW 264.7 M
or LA-4 epithelial cells were incubated for 30 min at 37°C in serum-free medium alone or supplemented with increasing concentrations of either mannan or ASF, as indicated, prior to the addition of 106 PFU of Guangdong/93. Mannan or ASF were included during subsequent culture of the cells. Cells were fixed 6 to 8 h postinfection and stained for the expression of influenza virus NP, and the percentage of virus-infected cells was determined via immunofluorescence. The data represent the mean percent infection ± 1 standard deviation of three independent experiments. *, P < 0.05; **, P < 0.01 (percent infected cells in the presence of inhibitor that were significantly different from the percent infected cells recorded in the absence of either inhibitor as determined by one-way ANOVA).
Cells were incubated with Guangdong/93 influenza virus in chamber slides in the absence or presence of various concentrations of ASF or mannan, and infection was monitored by subsequent detection of viral NP expression by immunofluorescence microscopy. As shown in Fig. Fig.5B,5B, ASF was found to inhibit infection of both J774E M and RAW 264.7 M
in a dose-dependent manner, whereas mannan inhibited infection of J774E M
but not of RAW 264.7 M
. Neither ASF nor mannan had any significant effect on infection of LA-4 epithelial cells. Inhibition of infection by each carbohydrate ligand thus correlated directly with expression of its cognate receptor. These findings are consistent with MMR and MGL both being able to mediate viral entry into M
.
Interaction of PR8 influenza virus with M
lectins.
The Mt. Sinai strain of A/PR8/34 influenza virus infects murine M very poorly (30). This phenomenon is illustrated in Fig. Fig.6A,6A, which compares the Guangdong/93, HKx31, and PR8 viruses for their ability to infect different M
populations and the epithelial cell lines LA-4 and MDCK cells. HKx31 (H3N2) is a reassortant of PR8 (H1N1) with A/Aichi/68 (H3N2) virus and differs from PR8 only in its surface glycoproteins (2). The HA of PR8 (Mt. Sinai) is poorly glycosylated, bearing glycans on the fibrous stalk of the HA molecule but none on the globular head (6). In contrast, HKx31 carries two (39), and Guangdong carries four (14) potential glycosylation sites on the head of the HA molecule, in addition to the stalk glycans. If MMR and MGL function as receptors for viral entry into M
, the poor infectivity of PR8 for M
may reflect a poor interaction with these C-type lectins.
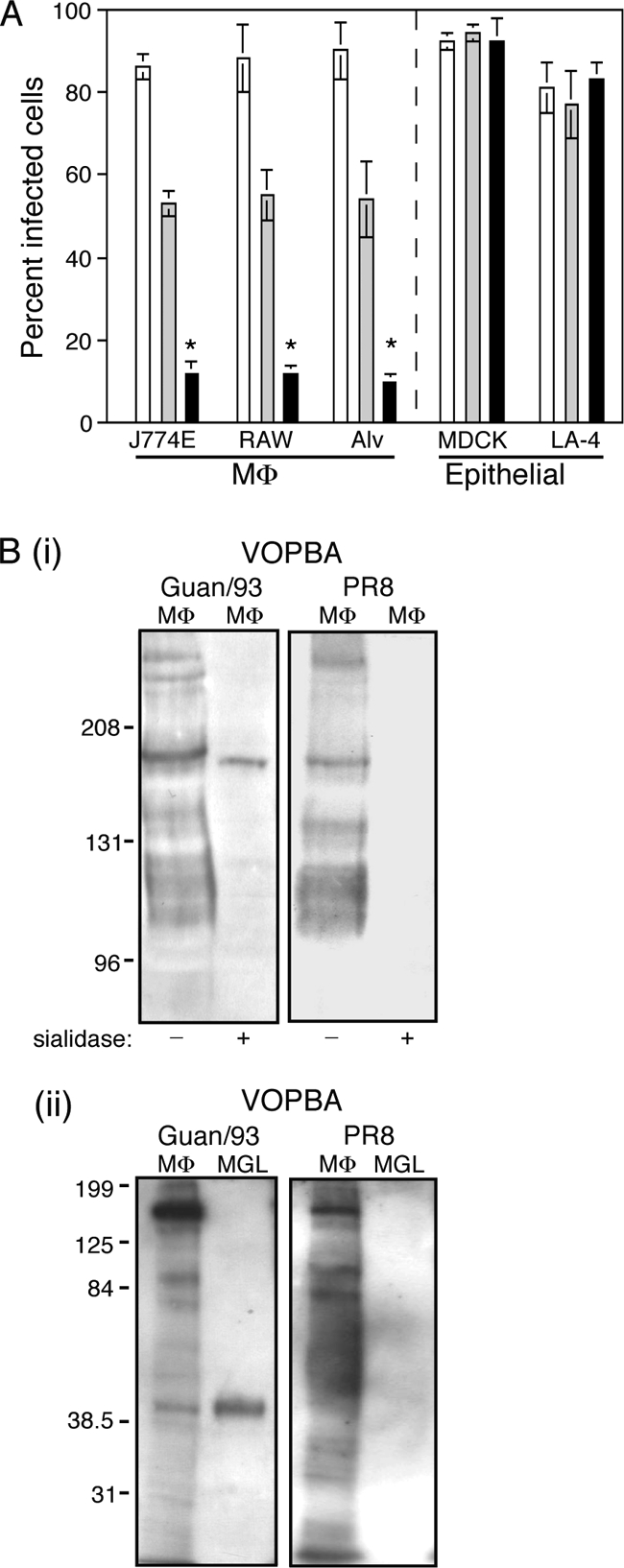
The poor ability of strain PR8 to infect M correlates with weak interactions with MMR and MGL. (A) Susceptibility of M
and epithelial cell lines to infection by Guangdong/93 (□), HKx31 (
), and PR8 (
) influenza viruses. Alv, resident alveolar M
. Monolayers of cells were infected in chamber slides with 106 PFU of influenza virus as described in Materials and Methods. The percentage of infected cells was determined in five independent fields per chamber by fluorescence microscopy. The data are expressed as mean percent infected cells ± 1 standard deviation and are representative of at least two independent experiments. *, P < 0.01 (exposure of cell monolayers to PR8 resulted in a significantly lower percentage of infected cells compared to cell monolayers exposed to either Gundaong/93 or HKx31 [one-way ANOVA]). (B) Comparison of M
proteins bound by PR8 and Guangdong/93 strains of influenza virus in VOPBA. (i) Samples of J774E lysate (M
) that had been sialidase treated (+) or not treated (−) were resolved by SDS-7% PAGE prior to blotting and application of virus. (ii) J774E lysate and isolated MGL, obtained by ASF-Sepharose affinity chromatography of lysate, were resolved by SDS-10% PAGE prior to blotting and application of virus.
The binding of PR8 to MMR and MGL was examined in VOPBA, and the results are shown alongside the binding pattern obtained for Guandong/93 in the same experiment (Fig. 6Bi and ii). (Note that the blot shown for Guangdong/93 in Fig. 6Bi is the same as that shown in Fig. Fig.2B,2B, left panels, PR8 binding having been tested in parallel with Guangdong/93 binding as part of the larger experiment depicted in Fig. 2B and C). Like Guangdong/93 virus, PR8 virus bound to numerous proteins from the untreated J774E lysate, including to a 175-kDa protein corresponding in size to the MMR (Fig. 6Bi); unlike Guangdong/93, however, binding of PR8 to the desialylated form of MMR was undetectable. This indicated that while PR8 may recognize sialic acid on MMR, the lectin domains of the MMR do not bind the carbohydrate on PR8 virus.
In virus blots to examine binding to MGL in J774E lysate and to isolated MGL (Fig. 6Bii), Guangdong/93 bound to MGL, as shown previously; in contrast, no binding of PR8 to MGL was detected at all. Together, these results support the hypothesis that the inefficiency of PR8 virus infection of M is related to poor recognition by the C-type lectins MMR and MGL, a finding consistent with an important role for these lectins as routes of entry for influenza virus into M
.
DISCUSSION
Although sialic acid has long been recognized as the primary receptor for attachment of influenza virus to the cell surface, the particular receptor or coreceptor molecules involved in mediating viral entry have not yet been elucidated for any cell type. The present study has used direct binding methods to investigate the cellular receptors involved in infection of M by influenza virus and extends our previous study that implicated the MMR in this process (30). As shown here by the VOPBA technique, virus bound to sialic acid on numerous M
glycoproteins. Binding of influenza virus Guangdong/93 to the MMR was confirmed, and binding to a second M
surface C-type lectin, MGL, was demonstrated, using VOPBA and virus-precipitation techniques. Binding to these latter receptors occurred independently of sialic acid, through Ca2+-dependent recognition of viral glycans by the carbohydrate recognition domains of the two lectins, although, as discussed further below, binding of virus to sialic acid on MMR also occurred. Both MMR and MGL are known to function as endocytic receptors (20, 26). Mannan and ASF, multivalent ligands of MMR and MGL, respectively, were found to block infection of M
by Guangdong/93 in a manner that correlated with expression of these receptors on different M
populations, while having no effect on infection of epithelial cells which lack these receptors. The PR8 (Mt. Sinai) strain of influenza virus, which is poorly glycosylated and infects M
very poorly, failed to show detectable binding in VOPBA to the lectin domains of either MGL or MMR. Together, these findings point to the importance of lectin-mediated interactions of virus with MMR and/or MGL in the infection process.
The mode of binding of Guangdong/93 virus to MMR was examined in VOPBA using conditions of desialylation and/or Ca2+ depletion with EDTA. The results indicated binding occurred in two ways: (i) by recognition of viral carbohydrate by the C-type lectin domains of MMR and (ii) by binding of the virus to sialic acid on MMR. An additional mode of ligand recognition by MMR has been reported, namely, Ca2+-independent recognition of sulfated oligosaccharides by the cysteine-rich domain of the molecule (13, 21). Although sulfation of HA has been described (9, 16), sulfated oligosaccharides have been associated with the stalk rather than the globular head of HA molecule (41, 42) and may be not be accessible for MMR binding. We obtained no evidence for this mode of binding to influenza virus, given that desialylation of MMR and removal of Ca2+ abrogated all binding of Guangdong/93.
In contrast to the dual mode of binding of influenza virus to MMR, interaction of MGL appeared to be mediated by the C-type lectin activity of MGL alone, since the binding of Guangdong/93 was fully abrogated in the presence of 0.1 M galactose (Fig. (Fig.3B)3B) and PR8 virus showed no binding at all to MGL in VOPBA (Fig. 6Bii). Although MGL is sialylated, as evidenced by its enhanced mobility after sialidase treatment (Fig. (Fig.3A),3A), the type of sialic acid, or the nature of its linkage to adjacent sugars, is apparently not recognized by influenza virus.
Sialidase treatment of M greatly reduces susceptibility to influenza virus infection (30). Thus, although binding of Guangdong/93 virus to desialylated MMR and MGL was clearly demonstrated in VOPBA (Fig. 2A and B and Fig. Fig.3A),3A), such interactions at the M
cell surface are not sufficient to mediate efficient infection. Efficient infection requires the contribution of both sialic acid and the lectin activity of MMR and/or MGL. A similar phenomenon has been reported previously where the enhanced entry of H5N1 pseudotype viral particles into stable cell lines expressing the C-type lectin DC-SIGN was abolished if cells were treated with bacterial sialidase prior to exposure to virus (40).
Our results support a model for infection of M by influenza virus in which MMR and MGL act as coreceptors with sialic acid. After attachment of virus to multiple sialylated molecules (glycoproteins and glycolipids) at the cell surface, virus binds through its glycans to the lectin domains of MGL or MMR. These endocytic receptors subsequently mediate the uptake of virus into endosomes, along with adjacent sialylated molecules to which the virus remains bound, the HA-sialic acid interaction being required for efficient fusion of viral and endosomal membranes at acid pH in the late endosome (10). The dual nature of binding of MMR to influenza virus, through both sialic acid and C-type lectin interactions, may enhance the efficiency of this route of entry. Indeed, through mediating both binding and entry functions, the MMR may conceivably behave as a functional receptor in its own right. Interestingly, VOPBA of PR8 virus on M
lysate appeared to indicate binding to the sialic acid on MMR (Fig. 6Bi). If so, such binding is clearly not sufficient to mediate efficient infection by PR8 virus, suggesting engagement of the lectin domains of MMR is essential for efficient entry of the virus to occur.
In RAW 264.7 M, which express MGL but not MMR, infection by Guangdong/93 virus was blocked by ASF but not by mannan, a finding consistent with viral entry being mediated by MGL in this cell type (Fig. (Fig.5).5). In J774E M
, which express both MMR and MGL, strong blocking of infection (>80%) could be achieved by either ligand alone. This suggests that when both MMR and MGL are present, they may act cooperatively to facilitate viral entry and that in blocking experiments, engagement of one type of receptor with its multivalent ligand affects the expression or functioning of the other receptor. Such a situation could occur if MMR and MGL are physically associated or clustered in the M
membrane and the binding of mannan to MMR, or ASF to MGL, sterically hinders access of viral glycans to the other receptor or results in its downmodulation by coendocytosis within the same coated pit. Experiments to monitor the surface expression of MMR and MGL on J774E M
in the absence or presence of cognate and noncognate ligand may help to resolve this issue.
The binding of influenza viruses to the lectin domains of MMR and MGL will reflect the type, number and accessibility of glycans carried on the virus. Glycosylation of influenza virus glycoproteins is N linked, and glycans may be high mannose, complex, or hybrid in type (3, 18). Complex glycans of influenza virus bear terminal galactose due to removal of sialic acid by the viral neuraminidase (4) and thus represent potential ligands for galactose-binding lectins such as MGL. High-mannose glycans represent a likely ligand for MMR, although glycans with exposed fucose or N-acetylglucosamine residues also represent potential targets (20, 33). There is considerable variation among influenza A viruses in the level of glycosylation of the HA molecule, particularly on its globular head. HKx31 and Guangdong/93 viruses carry two and four glycosylation sites, respectively, on the head of HA (14, 39). In contrast, PR8 virus carries no glycans on the head of its HA molecule (6) although, like all influenza A viruses, it carries glycosylation on the stalk region of HA at sites which are highly conserved between subtypes (24, 25, 31). The failure of PR8 virus to bind in VOPBA to the lectin domains of either MGL or MMR suggests that the stalk glycans on HA of intact virions are not readily accessible to binding by either receptor, and it is the glycans on the head of HA that are important in the interaction.
Two other recent studies have attempted to dissect the steps involved in binding and entry of influenza virus into cells. Stray et al. (35) found that influenza virus was able to infect sialidase-treated MDCK cells, albeit at lower efficiency, indicating the existence of sialic acid-independent functional receptors for influenza virus on this cell type. Chu and Whittaker (8) reported that influenza viruses could bind to but not infect Lec1 cells, a mutant line of CHO cells. Lec1 cells have a mutation in the N-acetylglucosaminyltransferase 1 gene (GnT1) that results in deficient terminal N-linked glycosylation, although gangliosides are still produced and O-linked glycosylation should not be deficient. Influenza virus displayed efficient binding to these cells, but infection was arrested at the level of internalization, and the virus was not endocytosed. These studies support a model of influenza virus infection in which the receptors for viral entry are distinct from primary attachment receptors.
The functional receptors for influenza virus appear to differ in different cell types. In the present study, receptors for influenza virus entry into murine M were identified as C-type lectins, the MMR and MGL, which are not expressed in MDCK cells or in LA-4 respiratory epithelial cells. Although the specific virus receptors on these latter cells have not yet been identified, other mannose- or galactose-specific lectins would not appear to be involved since mannan and ASF had no effect on infection of these cell types. Likewise, the functional receptors on MDCK and LA-4 cells would appear to be absent from murine M
since PR8 influenza virus, which is markedly inefficient at infecting M
, is able to infect MDCK and LA-4 cells with high efficiency. PR8 is a highly mouse-adapted virus and its ability to evade both MMR and MGL receptors and subsequent uptake and destruction by mouse M
, could be one factor contributing to its virulence for mice. Whereas mouse M
do not support productive replication of influenza virus (32), studies suggest that at least some human macrophage populations do (7, 27). Thus, the precise role of airway M
in host defense against influenza virus may differ in mice compared to humans.
Recently, mice have been shown to express two closely related MGL proteins, MGL1 and MGL2, with distinct carbohydrate specificities (37). MGL1 shows specificity for LewisX trisaccharides, whereas MGL2, like human MGL, preferentially binds to terminal N-acetylgalactosamine residues (36, 37). In the studies presented here we used MAbs LOM-8.7 (specific for MGL1) and LOM-14 (cross-reactive with MGL1 and MGL2) to detect expression of murine MGL. Subsequent studies have shown that J774 and RAW 264.7 M cell lines express MGL1, but not MGL2, at the cell surface (K. Denda-Nagai and T. Irimura, unpublished results). Our data pointing to MGL as a receptor for influenza virus entry into murine M
can thus be interpreted as function of MGL1. Whether MGL2 can also function in this way requires further study.
Acknowledgments
This study was supported by project grants 970283 and 509230 from The National Health and Medical Research Council (NH&MRC) of Australia. P.C.R. is a NH&MRC R. D. Wright Research Fellow. The Melbourne WHO Collaborating Centre for Reference and Research on Influenza is supported by the Australian Government Department of Health and Ageing.
REFERENCES
Articles from Journal of Virology are provided here courtesy of American Society for Microbiology (ASM)
Full text links
Read article at publisher's site: https://doi.org/10.1128/jvi.02148-09
Read article for free, from open access legal sources, via Unpaywall:
https://jvi.asm.org/content/jvi/84/8/3730.full.pdf
Free after 4 months at jvi.asm.org
http://jvi.asm.org/cgi/content/full/84/8/3730
Free to read at jvi.asm.org
http://jvi.asm.org/cgi/content/abstract/84/8/3730
Free after 4 months at jvi.asm.org
http://jvi.asm.org/cgi/reprint/84/8/3730
Citations & impact
Impact metrics
Citations of article over time
Alternative metrics
Article citations
Mannose Ligands for Mannose Receptor Targeting.
Int J Mol Sci, 25(3):1370, 23 Jan 2024
Cited by: 5 articles | PMID: 38338648 | PMCID: PMC10855088
Review Free full text in Europe PMC
The Alpha-1 Subunit of the Na+/K+-ATPase (ATP1A1) Is a Host Factor Involved in the Attachment of Porcine Epidemic Diarrhea Virus.
Int J Mol Sci, 24(4):4000, 16 Feb 2023
Cited by: 1 article | PMID: 36835408 | PMCID: PMC9966514
Role of a 49 kDa Trypanosoma cruzi Mucin-Associated Surface Protein (MASP49) during the Infection Process and Identification of a Mammalian Cell Surface Receptor.
Pathogens, 12(1):105, 07 Jan 2023
Cited by: 1 article | PMID: 36678452 | PMCID: PMC9865002
Influenza A Virus Exacerbates Group A Streptococcus Infection and Thwarts Anti-Bacterial Inflammatory Responses in Murine Macrophages.
Pathogens, 11(11):1320, 10 Nov 2022
Cited by: 3 articles | PMID: 36365071 | PMCID: PMC9699311
Glycosylation in SARS-CoV-2 variants: A path to infection and recovery.
Biochem Pharmacol, 206:115335, 31 Oct 2022
Cited by: 8 articles | PMID: 36328134 | PMCID: PMC9621623
Review Free full text in Europe PMC
Go to all (81) article citations
Data
Similar Articles
To arrive at the top five similar articles we use a word-weighted algorithm to compare words from the Title and Abstract of each citation.
N-linked glycosylation facilitates sialic acid-independent attachment and entry of influenza A viruses into cells expressing DC-SIGN or L-SIGN.
J Virol, 85(6):2990-3000, 29 Dec 2010
Cited by: 84 articles | PMID: 21191006 | PMCID: PMC3067946
Mannose receptor and macrophage galactose-type lectin are involved in Bordetella pertussis mast cell interaction.
J Leukoc Biol, 94(3):439-448, 21 Jun 2013
Cited by: 16 articles | PMID: 23794711
The macrophage galactose-type lectin can function as an attachment and entry receptor for influenza virus.
J Virol, 88(3):1659-1672, 20 Nov 2013
Cited by: 30 articles | PMID: 24257596 | PMCID: PMC3911607
SARS-CoV-2 Evolutionary Adaptation toward Host Entry and Recognition of Receptor O-Acetyl Sialylation in Virus-Host Interaction.
Int J Mol Sci, 21(12):E4549, 26 Jun 2020
Cited by: 53 articles | PMID: 32604730 | PMCID: PMC7352545
Review Free full text in Europe PMC