Abstract
Free full text

Apoptosis and Autophagy: Decoding Calcium Signals that Mediate Life or Death
Abstract
Calcium is a versatile and dynamic 2nd messenger that is essential for the survival of all higher organisms. In cells that undergo activation or excitation, calcium is released from the endoplasmic/sarcoplasmic reticulum to activate calcium-dependent kinases and phosphatases, thereby regulating numerous cellular processes; for example, apoptosis and autophagy. In the case of apoptosis, endogenous ligands or pharmacological agents induce prolonged cytosolic calcium elevation, which in turn leads to cell death. In contrast, there is now evidence that calcium regulates autophagy by several mechanisms, and these may be important for maintaining cell survival. Here we summarize what is known about how calcium regulates these life and death decisions. We pay particular attention to pathways that have been described in lymphocytes and cardiomyocytes, as these systems provide optimal models for understanding calcium signaling in the context of normal cell physiology.
Apoptosis is a process of programmed cell death or suicide that occurs when cells have undergone irreversible stress or damage. It is required to maintain normal cell homeostasis or to eliminate a population of cells that may be harmful to the organism or unnecessary during organ development (Green 2003). For example, it is the primary mechanism by which potentially autoreactive T cells are eliminated from the immune system. There are two conventional apoptosis pathways: the extrinsic pathway, which is typically initiated by death receptors (e.g., Fas) on the plasma membrane and the intrinsic (mitochondrial) pathway, which involves permeabilization of the outer mitochondrial membrane followed by the release of cytochrome c. In this review, we primarily focus our attention on the intrinsic pathway due to the importance of intracellular calcium in the regulation of this process.
In brief, cytochrome c release stimulates apoptosis via its interaction with the protein Apaf-1, which in turn activates the initiator caspase-9 and the executioner caspase-3 (Green 2005). Caspases comprise a family of cysteine proteases that are essential for the classically observed cellular and biochemical characteristics of apoptosis, which include (but are not limited to) membrane blebbing, chromatin condensation, and DNA fragmentation. Another class of cysteine proteases, calpains, require calcium for their activation and are important mediators of apoptosis following ER stress. As discussed later in this review, calpains are reported to directly activate caspases, thus promoting apoptotic cell death independent of mitochondrial cytochrome c release. The following sections provide a more detailed explanation of the varied ways in which calcium signals induce cell death and are themselves regulated.
APOPTOSIS REGULATION BY ANTIGEN RECEPTORS: A MODEL FOR PROGRAMMED CELL DEATH
Much that is known about calcium signaling came from immunological studies using activated lymphocytes (Berridge 1997). Immature T cells are an ideal model for investigating apoptosis because they are programmed to die during development. This is evident by the fact that 95% of double positive (i.e., CD4/CD8) thymocytes undergo apoptosis as a consequence of negative selection (Starr et al. 2003). Apoptosis of thymocytes occurs when self antigen presented on thymic epithelial cells binds to T-cell receptors with strong avidity (Hogquist 2001). As depicted in Figure 1, ligation of the T-cell receptor activates a signaling pathway that results in autophosphorylation of Src family kinases Lck and Fyn, which are recruited to the plasma membrane to phosphorylate the zeta chain of the T-cell receptor (Latour and Veillette 2001; Mustelin and Tasken 2003; Palacios and Weiss 2004). Activation of these kinases facilitates the hydrolysis of phosphatidylinositol 4,5-bisphosphate by phospholipase Cγ, thereby generating diacylglycerol and inositol 1,4,5-trisphosphate (IP3) (Lewis 2001). IP3 mediates ER calcium release through the opening of IP3 receptors (IP3Rs), which in turn stimulates calcineurin-mediated activation of the nuclear factor for the activation of T cells (NFAT) (Gallo et al. 2006; Winslow and Crabtree 2005; Winslow et al. 2003). Cytosolic calcium release is also mediated by ryanodine receptors, which are calcium channels expressed in lymphocytes, cardiomyocytes, and neurons. It has been suggested that ryanodine receptors facilitate calcium flux in response to nicotinic acid adenine dinucleotide phosphate (NAADP) and cyclic adenosine dinucleotide phosphate ribose, both of which are produced during T-cell activation (Berg et al. 2000; Dammermann et al. 2009; Guse et al. 1999). However, unlike ADP ribose, the notion that NAADP is a direct activator of ryanodine receptors is not yet certain and may be context- or cell-type specific (Galione and Petersen 2005). Nevertheless, the generation of these 2nd messengers, along with IP3, may be required for robust calcium elevation in response to antigen receptor stimulation.
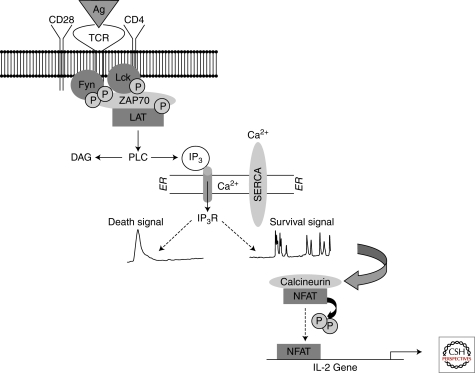
Calcium signaling mediated by T-cell activation. In a CD4 positive T cell, activation is induced by antigen binding to the T-cell receptor (TCR) and a co-stimulatory signal mediated by ligation of CD28. Src family kinases Fyn and Lck are activated by autophosphorylation and recruited to the plasma membrane to associate with CD3 (TCR) and CD4, respectively. This in turn leads to the phosphorylation of ZAP-70 and the adaptor protein LAT in order to activate phospholipase C and generate 2nd messengers IP3 and diacylglycerol (DAG). IP3 binds to the IP3R, resulting in ER calcium release. Calcium is released into the cytosol by way of a single calcium transient (left), which is associated with cell death by apoptosis. On the other hand, calcium oscillations activate calcineurin, which dephosphorylates NFAT, thereby sending it to the nucleus to activate transcription of IL-2. The Sarcoplasmic/Endoplasmic Reticulum Calcium ATPase (SERCA) is responsible for maintaining the appropriate concentration of luminal calcium by actively transporting calcium across the ER membrane.
In general, lymphocyte activation encodes distinct patterns of calcium signaling, which ultimately regulate cell proliferation, survival, and apoptosis (Berridge 1997; Lewis 2001) (Fig. 1). It has been proposed that strong agonist stimulation of T-cell receptors generates calcium transients that trigger apoptosis, whereas weak stimulation produces calcium oscillations that are needed for cell survival (Randriamampita and Trautmann 2003). The patterns of calcium elevation following strong and weak agonist stimulation are vastly different. For example, calcium transients can be detected in cells 1–2 minutes following activation of the T-cell receptor. They are generally synchronized and characterized by a broad peak that is high in amplitude. On the other hand, calcium oscillations consist of asynchronous and repetitious spikes that persist for as long as one hour following activation. Using fluorescence-activated cell sorting, we have shown that T cells with a high level of cytosolic calcium more readily undergo apoptosis compared to those that have lower levels (Zhong et al. 2006). Further, in those cells that undergo calcium oscillations in response to weak agonist stimulation, NFAT is rapidly de-phosphorylated, and this is associated with increased levels of IL-2 mRNA (Harr et al. 2009; Zhong et al. 2006). These observations are consistent with the strength of signal theory, which states that T cells undergo positive or negative selection according to the avidity of T-cell receptor activation (Hogquist 2001; Mariathasan et al. 1998). This provides one example in which the amplitude and frequency of calcium signals encodes information that regulates apoptosis or cell survival.
APOPTOSIS REGULATION BY HORMONES AND OTHER SIGNALING MOLECULES
Corticosteroids
Knowledge of calcium-dependent apoptosis evolved from studies examining the effects of glucocorticoids on immature T cells. Glucocorticoids, such as cortisol, are physiological immunomodulatory hormones that regulate immune cell development. Pharmacologically, synthetic glucocorticoids, such as prednisone and dexamethasone, are widely used to treat autoimmune disease and cancer (e.g., leukemia and lymphoma) because of their ability to suppress the immune system and selectively kill immature lymphocytes, respectively. Glucocorticoid hormones are secreted from thymic epithelial cells to antagonize self-antigen recognition in immature thymocytes (Ashwell et al. 2000). Thus, glucocorticoids negatively regulate T-cell activation by attenuating T-cell receptor signaling (Baus et al. 1996; Lowenberg et al. 2005; Van Laethem et al. 2001). Conversely, T-cell receptor signaling can also inhibit glucocorticoid-induced apoptosis, a concept known as mutual antagonism (Ashwell et al. 2000; Jamieson and Yamamoto 2000; Tolosa and Ashwell 1999).
While studying these inhibitory effects on T-cell activation, our laboratory discovered that short-term treatment with glucocorticoids modulates T-cell receptor-mediated calcium elevation by converting calcium transients to oscillatory signals (Harr et al. 2009). However, prolonged glucocorticoid treatment with pharmacological concentrations of prednisone or dexamethasone results in thymocyte apoptosis by a mechanism that is dependent, in part, on de novo transcription (Herold et al. 2006). Importantly, glucocorticoid-induced apoptosis in thymocytes is consistently associated with a sustained rise in cytosolic calcium (Bian et al. 1997; Cohen and Duke 1984; Kaiser and Edelman 1977; Lam et al. 1993; McConkey et al. 1989; Orrenius et al. 1991). This increase in cytosolic calcium is associated with the classically observed biochemical characteristics of apoptosis, including DNA fragmentation and endonuclease activity. While there is evidence that cytosolic calcium elevation contributes to the induction of apoptosis, this mechanism has not been firmly established. Marks and colleagues found that anti-sense mediated knockdown of IP3R1 protected cells from apoptosis induced by dexamethasone (Jayaraman and Marks 1997). However, we observed that dexamethasone-mediated up-regulation of IP3Rs did not contribute to cytosolic calcium elevation or apoptosis following glucocorticoid treatment (Davis et al. 2008). An alternative theory is that dexamethasone down-regulates the sarcoplasmic endoplasmic reticulum ATPase (SERCA) that pumps calcium into the ER (Chai et al. 2009), thereby decreasing ER luminal calcium and inducing apoptosis.
Angiotensins
Angiotensins are a second class of hormone that induce calcium-dependent apoptosis, specifically Angiotensin II (Cigola et al. 1997; Kajstura et al. 1997; Palomeque et al. 2009; Yamada et al. 1996). Angiotensins are oligomeric peptides released in response to steroid hormones, such as glucocorticoids and estrogen. They are powerful vasoconstrictors, and consequently, angiotensin receptors are targets for antihypertensive medications (Gradman 2009). In a cardiomyocyte, muscle contraction is stimulated by the opening of an L-type calcium channel that enables calcium release via ryanodine receptors (Fig. 2). Angiotensins bind to their receptors (AT1 and AT2) resulting in the generation of IP3 followed by transient calcium elevations (Mattiazzi 1997). While ryanodine receptors are more abundant than IP3Rs in cardiomyocytes, both calcium and IP3 are required for IP3R channel opening. While still not universally accepted, it is likely that calcium release via neighboring ryanodine receptors facilitates IP3R-opening, enabling both channels to function cooperatively in response to angiotensin ligands (Kockskamper et al. 2008).

Calcium signaling mediated by angiotensin II hormone in a cardiomyocyte. In a cardiomyocyte, calcium signaling is mediated by the influx of calcium through L-type calcium channels (LTCC). The Sarcoplasmic/Endoplasmic Reticulum Calcium ATPase (SERCA) is responsible for maintaining the appropriate concentration of luminal calcium by actively transporting calcium ion across the SR membrane, while ryanodine receptors and IP3Rs promote its release into the cytosol. Angiotensin, a peptide hormone, binds to the AT-1 receptor (AT-1R), a G-protein coupled receptor that activates phospholipase C (PLC) following GTP hydrolysis, thereby generating IP3 and diacylglycerol (DAG). It should be noted that ryanodine receptors are 50- to 100-fold more abundant than IP3Rs in cardiomyocytes. Therefore, calcium release via ryanodine receptors have shown to be much more robust compared to calcium responses that are mediated by IP3Rs (Kockskamper et al. 2008). Nevertheless, there is unequivocal evidence for the contribution of IP3Rs during SR-calcium release, which is likely due to synergy between the two calcium channels in mediating calcium-induced calcium release.
Much like glucocorticoids, treatment with higher concentrations of angiotensin II results in apoptosis that can be blocked by receptor antagonists (Andreka et al. 2004). As depicted in Figure 2, stimulation of cardiomyocytes with angiotensin II causes an acute release of cytosolic calcium, and several reports suggest that calcium elevation contributes to apoptosis (Kajstura et al. 1997). For instance, verapamil, an L-type calcium channel blocker, inhibits angiotensin-induced apoptosis (Goldenberg et al. 2001). Further, ectopic expression of angiotensin receptors (AT1 and AT2) results in apoptosis by a calcium-dependent mechanism (Aranguiz-Urroz et al. 2009).
Testosterone
Testosterone is a steroid hormone that has rapid effects on cardiomyocytes. In one study, it was shown that testosterone increased cytoplasmic calcium concentrations in 1–7 minutes by an IP3R-dependent fashion (Vicencio et al. 2006). A second study reported that testosterone signals activate the extracellular signal-regulated kinase (ERK), and this activation could be inhibited by 2-aminoethyldiphenyl borate and the phospholipase C inhibitor U-73122, suggesting that testosterone generates IP3 in cardiomyocytes (Altamirano et al. 2009). Similar rapid non-genomic effects of testosterone have been observed in T cells, where calcium influx was observed within seconds (Benten et al. 1997). However, it remains to be determined whether these calcium signals would eventually lead to apoptosis. Interestingly, in neuronal cells, low concentrations of testosterone result in calcium oscillations, whereas higher concentrations induce apoptosis, also by a mechanism that is IP3R dependent (Estrada et al. 2006). Thus, steroid hormones can have direct apoptotic effects on multiple cells types, as is the case for glucocorticoids and androgens, and perhaps indirectly via glucocorticoid regulation of angiotensin.
Nitric Oxide
Nitric oxide is an endogenous signaling molecule that regulates muscle contraction, oxygen consumption, and mitochondrial metabolism in the heart (Massion et al. 2003). It is produced by a family of calcium-dependent enzymes called nitric oxide synthases (NOS). NOS enzymes have been found to localize to the sarcoplasmic reticulum and can inhibit L-type channel and ryanodine-receptor activity (Barouch et al. 2002; Sears et al. 2003; Xu et al. 1999). Further, there is evidence that NOS enzymes can inhibit calcium channel activity by S-nitrosylation of thiol residues (Davidson and Duchen 2006; Razavi et al. 2005), which in turn decreases mitochondrial calcium uptake, thereby preventing cytochrome c release and mitochondrial metabolism (Brooks et al. 2000; Dedkova and Blatter 2005; Khan and Hare 2003). In addition, nitric oxide can also attenuate apoptosis by nitrosylation of caspases on cysteine residues (Dimmeler et al. 1997). The ability of nitric oxide to prevent apoptosis in a calcium-dependent manner may be important for understanding certain pathophysiologies such as ischemia or reperfusion injury.
APOPTOSIS REGULATION BY PHARMACOLOGICAL AGENTS
Although calcium-mediated apoptosis can occur by physiological signals, there are multiple cytotoxic agents that function to disrupt calcium homeostasis leading to apoptotic cell death. Among these are thapsigargin, staurosporine, and cisplatin.
Thapsigargin
Thapsigargin decreases the ER calcium pool by inhibiting SERCA pumps, which results in ER stress and apoptosis (Lam et al. 1993). Apoptosis induced by thapsigargin occurs by a mechanism that is dependent on the activation of caspase-12, a mammalian protease that localizes to the ER and is important for mediating apoptosis in response to ER stress (Szegezdi et al. 2003). This is exemplified by experiments performed in mice in which caspase-12 had been deleted (Nakagawa et al. 2000). In vitro studies have demonstrated that a calpain activates caspase-12 leading to the subsequent activation of caspase-9 (Morishima et al. 2002; Nakagawa and Yuan 2000; Rao et al. 2002). These data suggest the possibility that apoptosis induced by thapsigargin can occur independently of cytochrome c release and is thus directly induced by calcium via calpain activation.
Staurosporine
Staurosporine is a natural apoptosis-inducing alkaloid originally isolated from Streptomyces staurosporeus. It directly provokes calcium leak from the ER by activating caspase-3 mediated cleavage of IP3R1 (Hirota et al. 1999). Additionally, it was shown that cleavage of IP3R1 contributed, in part, to the induction of apoptosis by accelerating calcium leak (Assefa et al. 2004; Verbert et al. 2008). In these experiments, transfection of a mutant IP3R resistant to caspase-mediated cleavage partially inhibited apoptosis induction by staurosporine in B cells lacking wild type IP3Rs. A recent study by Mikoshiba and colleagues further identified IP3Rs as being important mediators of apoptosis induction by staurosporine. They determined that G protein-coupled receptor kinase interacting proteins (GITs) bind to IP3Rs to inhibit their function and suppress apoptosis in the presence of staurosporine (Zhang et al. 2009b). Finally, staurosporine also promotes the activation of a mitochondrial calpain that positively regulates apoptosis (Norberg et al. 2008), thus illustrating similarities with ER stress-driven pathways.
Cisplatin
A third example is cisplatin, a platinum-based chemotherapeutic agent used to treat several types of cancer. Cisplatin also causes an IP3R-dependent increase in cytosolic calcium and subsequent activation of a calpain prior to the induction of apoptosis (Mandic et al. 2003; Schrodl et al. 2009; Splettstoesser et al. 2007). Further, cisplatin treatment results in ER stress as suggested by increased expression of Grp78 and activation of caspase-12 (Mandic et al. 2003). Interestingly, IP3R1 contributes to cisplatin sensitivity in bladder cancer, as knocking down its expression in cell lines mediates resistance to apoptosis (Tsunoda et al. 2005). These results collectively indicate that cisplatin induces apoptosis, in part, by disrupting calcium homeostasis in a variety of cell types.
APOPTOSIS REGULATION BY ANTI-APOPTOTIC Bcl-2 FAMILY PROTEINS
In each of the previous examples in which apoptosis is regulated by calcium, cell death can be readily inhibited by anti-apoptotic proteins such as B cell leukemia/lymphoma-2 (Bcl-2) and other Bcl-2 family members. As its name implies, Bcl-2 was first identified because it was overexpressed in B cell follicular lymphoma (Tsujimoto et al. 1985). Membership in the Bcl-2 family is defined by the presence of Bcl-2 homology domains (BH domains) (Chipuk et al. 2010). Bcl-2 has four BH domains. BH1, BH2, and BH3 are located within the C-terminal half, where they participate in forming a hydrophobic groove that binds and thereby inhibits proapoptotic family members. The BH4 domain is located near the N-terminus and connected to the C-terminal half of Bcl-2 by an unstructured loop, facilitating intra- and intermolecular interactions. It is now known that Bcl-2 is overexpressed in a number of cancers because of its ability to inhibit cell death and promote survival of malignant cells (Cory and Adams 2002). In fact, Bcl-2 localizes not only to the outer mitochondrial membrane but also to the ER, where it regulates IP3-mediated calcium release. The observation that Bcl-2 regulates calcium release from the ER was initially made more than 15 years ago (Baffy et al. 1993; Lam et al. 1994) and the overall importance of Bcl-2 on the ER is exemplified in studies in which ER-targeted Bcl-2 inhibited apoptosis in response to agents that depolarize the mitochondrial membrane (Annis et al. 2001).
The Bcl-2-IP3 Receptor Interaction
Our laboratory was the first to show that Bcl-2 directly interacts with IP3Rs to inhibit IP3-dependent calcium flux (Fig. 3) (Chen et al. 2004). This interaction, as well as an interaction of the Bcl-2 homologue Bcl-xL with the IP3R, has subsequently been detected by a number of laboratories (Rong and Distelhorst 2007). Bcl-2 directly inhibits IP3R channel opening in vitro in lipid bilayer experiments and also inhibits IP3-induced calcium release in T cells (Chen et al. 2004; Zhong et al. 2006). We have now further elucidated the mechanism of the Bcl-2-IP3R interaction by demonstrating that the BH4 domain of Bcl-2 associates with the regulatory and coupling domain of IP3R1, specifically an 80 amino acid sequence within domain 3 (Rong et al. 2008). Further analysis indicated that the BH4 domain was both necessary and sufficient to inhibit IP3-mediated calcium signals and subsequently apoptosis in T cells (Rong et al. 2009). This is particularly interesting given that the BH4 domain of Bcl-2 and Bcl-xL seem to be involved in preventing apoptosis. Although our work has emphasized the interaction of Bcl-2 with the regulatory and coupling domain of the IP3R, there is also evidence that Bcl-2 and/or Bcl-xL may interact with the C-terminus of the IP3R, and through this interaction, enhance IP3-mediated calcium oscillations (White et al. 2005). In light of these discoveries, there is now increased interest in using small molecules to inhibit Bcl-2 and enhance proapoptotic calcium transients. One example of this was the use of a Bcl-2 inhibitor HA14-1 that induced cytochrome c release and apoptosis by a calcium-dependent mechanism in myeloid leukemia (An et al. 2004).
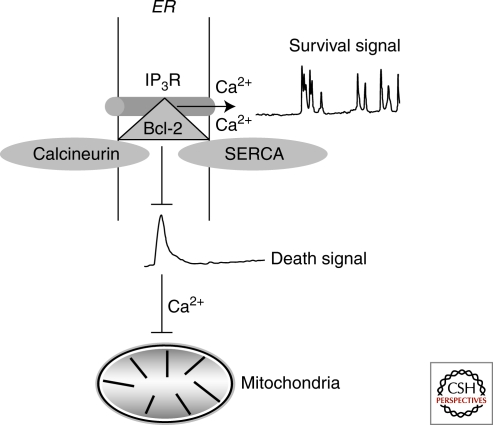
The Bcl-2-IP3R interaction inhibits ER-calcium release. Bcl-2 localizes to the ER where it binds IP3Rs to inhibit calcium transients. In T cells, calcium transients are activated in response to strong T-cell receptor ligation, which results in apoptosis that can be inhibited by Bcl-2. In contrast, calcium oscillations that are associated with cell survival are promoted by Bcl-2 and Bcl-xL. In addition, Bcl-2 regulates the level of ER luminal calcium by increasing membrane permeability or by interacting with the Sarcoplasmic/Endoplasmic Reticulum Calcium ATPase (SERCA). Bcl-2 also interacts with calcineurin, thereby forming a complex with both calcineurin and IP3Rs on the ER membrane.
Bcl-2 and IP3R Phosphorylation
Other proteins within the Bcl-2-IP3R complex may regulate ER calcium release by altering phosphorylation of IP3Rs. As shown in Figure 3, Bcl-2 binds the phosphatase calcineurin (Erin et al. 2003; Shibasaki et al. 1997), and calcineurin also interacts with IP3Rs (Cameron et al. 1995). Because IP3R channel activity is positively regulated by phosphorylation (DeSouza et al. 2002), it is reasonable to speculate that Bcl-2 may facilitate the dephosphorylation of IP3R by interacting with calcineurin. Although such a mechanism has not been definitively established, we have observed that IP3R phosphorylation is decreased in Bcl-2 overexpressing T cells (Chen et al. 2004). Furthermore, Bcl-2 has been reported to regulate IP3R phosphorylation in Bax/Bak double knockout cells (Oakes et al. 2005). Moreover, Xu et al. have implicated protein phosphatase 1 in the regulation of IP3R phosphorylation by Bcl-2 (Xu et al. 2007). Thus, much remains to be determined regarding the specific kinases and phosphatases that regulate IP3R channel opening, how these are regulated by Bcl-2-IP3R interaction, and how they contribute overall to the regulation of calcium signals by Bcl-2 and its relatives.
Bcl-2 and Mitochondrial Cross Talk
Another important function of Bcl-2 and Bcl-xL is to inhibit calcium-mediated cross talk between ER and mitochondria (Kruman and Mattson 1999; Pinton et al. 2008). Because both organelles are in close proximity, calcium is rapidly taken up by mitochondria via the calcium uniporter on the outer mitochondrial membrane (Hajnoczky et al. 2006; Hanson et al. 2004; Rizzuto et al. 2003; Szalai et al. 1999; Szlufcik et al. 2006). Bcl-2 and Bcl-xL inhibit calcium redistribution to the mitochondria, thereby limiting calcium uptake (Hanson et al. 2008; Pinton et al. 2008) (Fig. 3). In the context of apoptosis, it was shown that Bcl-2 inhibited mitochondrial calcium uptake following IL-3 and serum withdrawal in hematopoietic cells and fibroblasts, respectively (Baffy et al. 1993; Magnelli et al. 1994). Interestingly, Bcl-2 inhibited apoptosis in cardiomyocytes exposed to ceramide and staurosporine, which caused depolarization of the mitochondrial membrane and cytochrome c release (Pacher and Hajnoczky 2001). A more recent study suggests that Bcl-2 inhibits calcium release through L-type channels, thereby preventing mitochondrial calcium uptake (Diaz-Prieto et al. 2008). Finally, Mcl-1, an anti-apoptotic protein overexpressed in myeloid and lymphoid leukemia, also blocks calcium redistribution following exposure to staurosporine (Minagawa et al. 2005).
Bcl-2 Regulation of Luminal Calcium
In contrast to experiments reporting that Bcl-2 inhibits IP3R opening, there is also substantial evidence that Bcl-2 and Bcl-xL directly regulate the concentration of luminal calcium (Fig. 3). Initial studies showed that Bcl-2 increased membrane permeability, thereby resulting in calcium leak and decreased signaling in response to ATP (Foyouzi-Youssefi et al. 2000; Pinton et al. 2000). Another study documented that knocking down Bcl-2 prevented the loss of ER luminal calcium (Oakes et al. 2005). While these studies also cite the significance of the Bcl-2-IP3R interaction in regulating the calcium pool, others have found that Bcl-2 depletes luminal calcium by interacting with SERCA (Dremina et al. 2004; Dremina et al. 2006; Vanden Abeele et al. 2002). In spite of these differences in experimental findings, it cannot be refuted that Bcl-2 localizes to the ER to inhibit calcium signaling. Thus, mechanistic differences may be attributed to cell type in which the relative expression and localization of Bcl-2 family members are considerably distinct. Accordingly, the effect of luminal calcium is dependent upon the predominant IP3R isoform expressed, yet not necessary for Bcl-2 or Bcl-xL mediated effects on calcium signaling (Li et al. 2007).
Bcl-2 Regulation of Prosurvival Calcium Signals
Although Bcl-2 inhibits calcium transients that are associated with apoptosis, anti-apoptotic Bcl-2 family members can also enhance calcium oscillations that promote survival (Fig. 3). In T cells, Bcl-2 enhances calcium oscillations induced by weak T cell receptor stimulation and thus increases NFAT activation (Zhong et al. 2006). Similarly, Bcl-xL enhances prosurvival oscillations following weak ligand stimulation (Distelhorst and Zhong, unpubl). In DT40 B lymphocytes, Bcl-2, Bcl-xL, and Mcl-1 enhance calcium oscillations by sensitizing cells to lower concentrations of IP3 (Eckenrode et al. 2010; Li et al. 2007; White et al. 2005). This in turn leads to accelerated mitochondrial metabolism and cell survival. Additional studies in other cell types support this hypothesis by demonstrating that overexpression of Bcl-2 enhances calcium oscillations in epithelial cells following stimulation with ATP and also in neuronal cells to facilitate survival (Jiao et al. 2005; Palmer et al. 2004).
APOPTOSIS REGULATION BY PROAPOPTOTIC Bcl-2 FAMILY PROTEINS
In contrast to Bcl-2, proapoptotic Bcl-2 family proteins are missing a classic BH4 domain, although recent findings suggest the presence of consensus BH4 sequence in proapoptotic family members (Chipuk et al. 2010). Multidomain BH proteins (i.e., BH1-3) include Bax and Bak, both of which are essential for apoptosis driven by the mitochondrial pathway. Multiple studies using Bax/Bak knockout models have demonstrated that the loss of these proteins confers resistance to numerous apoptotic stimuli (Wei et al. 2001).
Calcium Regulation by Bax and Bak
Like Bcl-2 and Bcl-xL, Bax and Bak also localize to the ER where they regulate calcium homeostasis. In Bax/Bak knockout cells, ER luminal calcium is decreased compared to wild type cells, which compromises ER calcium release as well as mitochondrial uptake (Oakes et al. 2005; Scorrano et al. 2003). Consistent with these observations, the Bax Inhibitor-1 protein facilitates ER calcium leak, depleting the available calcium pool (Chae et al. 2004; Kim et al. 2008). Although the mechanism by which Bax and Bak decrease luminal calcium has not been determined, it is generally inferred that Bax/Bak ordinarily prevents Bcl-2-mediated ER calcium leak, and thus their deficiency promotes depletion of ER luminal calcium by Bcl-2. Moreover, Bax alone is required for calcium elevation in response to cytotoxic agents, such as staurosporine (Nutt et al. 2002a). For example, reconstitution of Bax in a prostate cancer cell line augmented cytosolic calcium elevation and restored mitochondrial uptake. The same group also reported that Bax/Bak overexpression induced calcium elevation followed by cytochrome c release and apoptosis (Nutt et al. 2002b). Interestingly, when Bak is targeted to the ER, it facilitates cytosolic calcium elevation and activates caspase-12; yet this does not occur when Bak is specifically targeted to mitochondria (Zong et al. 2003). In addition, ER-targeted Bak requires calcium in conjunction with ER stress for apoptosis to occur (Klee et al. 2009). This suggests that the localization of Bax and/or Bak may determine which effector pathway is induced. It is possible that Bax/Bak localization to the ER is favored when cells undergo apoptosis induced by ER stress.
Calcium Regulation by BH3-only Proteins
Other proapoptotic Bcl-2 family members have only the BH3 domain and therefore are designated as “BH3-only” proteins. These include Bim, Bad, Bik, BNIP3, PUMA, and NOXA (Cory and Adams 2002). Like their multi-domain counterparts, these proteins also localize to both ER and mitochondria. At the mitochondria, BH3-only proteins facilitate Bax/Bak oligomerization by two potential mechanisms. In brief, one model suggests they function to directly activate Bax and Bak, whereas another suggests they do so indirectly by sequestering anti-apoptotic Bcl-2 family members (Cheng et al. 2001; Willis et al. 2007). Although the mechanism remains controversial, it is now certain that BH3-only proteins are necessary for Bax/Bak activation and the induction of apoptosis.
While the role of these proteins at the mitochondria has been extensively studied, there are sufficient data to conclude that they also regulate apoptosis at the ER. For example, Bim translocates to the ER following ER stress and may be required for the activation of caspase-12 (Morishima et al. 2004). Thus, ER localization of Bim as well as Bak may be necessary for apoptosis in response to ER stress-inducing agents. Interestingly, dexamethasone induces Bim transcript and protein levels in T cells (Lu et al. 2006; Wang et al. 2003), a process that is associated with the elevation of cytosolic calcium and required for a robust apoptotic response. Engagement of T-cell receptors also stimulates de novo transcription of Bim by a calcium-dependent mechanism (Cante-Barrett et al. 2006). It has yet to be determined if this process contributes to apoptosis induced by T-cell receptor activation. Intriguingly, T cells deficient in Bim have impaired calcium release following their stimulation, and this is associated with increased binding of Bcl-2 to IP3Rs (Ludwinski et al. 2009). Thus, another role of Bim may be to enhance calcium elevation by sequestering Bcl-2 away from IP3Rs at the ER membrane.
Bik is a BH3-only protein that promotes ER calcium depletion in a Bax/Bak dependent manner (Mathai et al. 2005). Similarly, BNIP3 causes a leak of ER luminal calcium when selectively targeted to the ER (Zhang et al. 2009a). NOXA and PUMA are both p53 target genes that are up-regulated in response to genotoxic stress. A study by Shore and colleagues has shown that NOXA may function cooperatively with Bik to promote the activation of Bax and Bak (Germain et al. 2005). A recent study indicates that the mitochondrial targeting region of NOXA functions to increase mitochondrial permeability and release calcium. Interestingly, a peptide corresponding to this region was able to induce calcium-dependent cell death by necrosis (Seo et al. 2009). This demonstrates that BH3-only proteins are not only important for regulating calcium flux and homeostasis, but may also function to regulate other mechanisms of cell death. Collectively, the Bcl-2 family makes up a network of proteins and each contributes to the regulation of normal calcium homeostasis. It is clear that alterations in expression or localization of these proteins can have profound effects on cell viability by inducing apoptosis.
AUTOPHAGY
Autophagy is a process of self-eating whereby cellular organelles and proteins are phagocytosed in order to produce energy during metabolic stress (Levine and Klionsky 2004). It is an evolutionarily conserved physiological process that is thought to promote cell survival. Some cellular contexts in which autophagy may be induced include nutrient deprivation, hypoxia, ER stress, abnormal cell growth, and microbial infection (Mizushima et al. 2008). On the other hand, autophagy has also been shown to promote cell death under certain conditions and stimuli. In fact, autophagy is often referred to as type II programmed cell death (distinct from type I programmed cell death) because it does not require caspase activation or DNA fragmentation, which are classical characteristics of apoptosis (Levine and Yuan 2005). However, it is likely that both processes occur simultaneously, and thus, it is important to understand the signaling pathways that govern autophagy, especially when considering that many of the same mechanisms regulate apoptosis.
During autophagy, double membrane vesicles, or autophagosomes, fuse with the lysosome, leading to the degradation of cellular proteins (Mizushima 2007). An example of an autophagosome is illustrated by the electron micrograph in Figure 4; shown are autophagosomes from a WEHI 7.2 T cell stably expressing Bcl-2 and cultured in normal growth media containing dexamethasone. Glucocorticoids promote autophagy (Laane et al. 2009; Swerdlow et al. 2008), and this process is most evident when apoptosis is inhibited by Bcl-2 (Swerdlow et al. 2008).
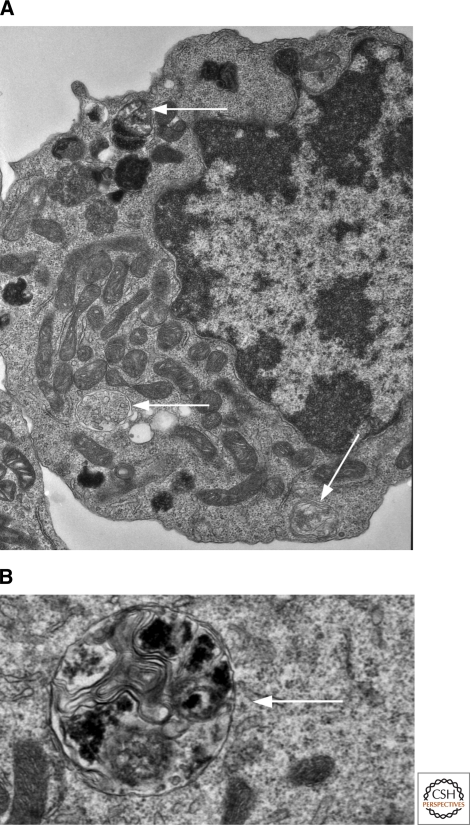
Electron micrograph of a malignant T cell undergoing autophagy. T cells that ectopically express Bcl-2 readily undergo autophagy in the presence of glucocorticoid hormones. Here, WEHI7.2 T cells stably expressing Bcl-2 (to inhibit apoptosis) were treated with 10−6 M dexamethasone for 72 hours and visualized by electron microscopy. (A) An electron micrograph of a single cell or (B) a region from within a cell. Examples of autophagosomes are shown next to the arrows.
Methods for the detection of autophagy are very well described by Klionsky and colleagues (Klionsky et al. 2008). Briefly, the most common method of analysis is the assessment of microtubule-associated protein-1 light chain-3 (LC3) by immunoblotting. LC3 is considered a marker for autophagy when it is proteolytically processed and conjugated to phosphatidylethanolamine (LC3II). GFP-tagged LC3 is commonly transfected into cells to detect LC3 aggregates or punctate GFP-LC3II, which is indicative of autophagosome formation. A second marker of autophagy is p62 degradation. The signaling adaptor p62, which is implicated in the activation of the transcription factor NFκB, is rapidly degraded by autolysosomes. Thus, p62 expression is inversely correlated with the conversion of LC3I to LC3II. Finally, as previously stated and shown in Figure 4, autophagosome formation can be readily visualized by electron microscopy, although this method is less amenable than others to quantitative interpretation.
Autophagy Induction by ER Stress Pathways
The link between calcium and autophagy was initially discovered by several groups reporting that autophagy could be provoked by ER stress (Bernales et al. 2006; Ogata et al. 2006; Yorimitsu et al. 2006). For example, both thapsigargin and tunicamycin stimulate autophagy (Ogata et al. 2006). ER stress affects Autophagy-Related Genes (atg), which are evolutionarily conserved and indispensable for autophagy in many cell systems. In yeast, the transcription factor Hac-1 (an ortholog of the ER-stress mammalian XBP-1) transactivates atg8 during the unfolded protein response (Bernales et al. 2006). Other studies have shown that mutations in ER stress-related proteins such as PERK or EIF2α can inhibit autophagy (Kouroku et al. 2007). Further, knockout of several atg genes prevents autophagy-mediated survival in the presence of tunicamycin (Ogata et al. 2006). While these data indisputably demonstrate that ER stress induces autophagy, a direct role for calcium had not been implicated at this point in time.
Autophagy Regulation by Calcium Signaling
Direct evidence that calcium signaling stimulates autophagy was first reported by Jaattela and colleagues (Hoyer-Hansen et al. 2007). They demonstrated that ER calcium mobilization induces autophagy when stimulated by agents such as vitamin D, ionomycin, and thapsigargin. Moreover, GFP-LC3 aggregates were inhibited with BAPTA-AM, suggesting that autophagosome formation was calcium dependent. They provided further evidence that autophagy occurred by the calcium-dependent activation of AMP activated protein kinase, which required upstream activation of the calcium/calmodulin kinase kinase β. AMPK is activated during nutrient deprivation to inhibit activity of the target of rapamycin (mTOR), a negative regulator of autophagy (Hoyer-Hansen and Jaattela 2007). Further evidence supporting a direct role for calcium in the induction of autophagy was the finding that calcium phosphate precipitates could induce autophagy when transfected into HEK293 cells (Gao et al. 2008). Importantly, autophagy mediated by calcium phosphate was also Beclin-dependent. Beclin is a newly discovered BH3-only protein that mediates autophagy by forming a complex between the class III PI3 kinase Vps34 and p150, which facilitates assembly of the autophagosome (Sinha and Levine 2008).
Autophagy in the Context of T-cell Activation
As previously described, significant contributions in the calcium field have been made by investigating signal transduction pathways in activated lymphocytes. Accordingly, autophagy may also be important for regulating lymphocyte activation. For example, T cells from atg5 knockout mice do not proliferate following ligation of the T-cell receptor, nor do they survive in the periphery (Pua et al. 2007), suggesting that autophagy is required for T-cell activation. Interestingly, it was shown that T-cell activation increases autophagy by NFkB-dependent transcription of Beclin-1 (Copetti et al. 2009a; Copetti et al. 2009b). In this study, the authors provide evidence that NFκB directly binds to the Beclin-1 promoter following activation of Jurkat T cells. Although a direct link has not been observed, it is possible that calcium-dependent activation of calcineurin stimulates this process, thus implicating a role for calcium in Beclin-1 transcription and autophagy.
In addition to producing IP3, T-cell activation also generates reactive oxygen species (Devadas et al. 2002; Hildeman et al. 2003). Thiol groups are found on IP3Rs and ryanodine receptors, and oxidation of both calcium channels favors their opening (Bootman et al. 1992; Bultynck et al. 2004; Joseph et al. 2006; Sun et al. 2001; Xia et al. 2000). Further, cyclic ADP ribose and NAADP govern redox reactions and are also endogenous ligands for ryanodine receptors and natural 2nd messengers produced by T-cell activation (Fliegert et al. 2007; Guse 2009). These studies have implicated a role for reactive oxygen species in regulating calcium signals. Not surprisingly, reactive oxygen species also contribute to the induction of autophagy. For example, hydrogen peroxide directly facilitates formation of the autophagosome by oxidizing Atg4 (Scherz-Shouval et al. 2007). Another study demonstrated that neurons undergo autophagy when mitochondrial fission is induced by nitric oxide (Barsoum et al. 2006). This observation is attractive in light of the fact that nitric oxide protects cardiomyocytes from apoptosis. Together, these data suggest the possibility that oxidative metabolites function as signaling molecules by activating calcium and triggering autophagy in lymphocytes, although more definitive data is necessary to support this important conclusion.
Autophagy Regulation by IP3Rs
There is now substantial evidence that autophagy is directly regulated by IP3 as well as by IP3Rs. For example, inhibition of inositol monophosphate by agents such as lithium and L-690,330 induced autophagy (Sarkar et al. 2005; Sarkar and Rubinsztein 2006). Kroemer and colleagues first showed that IP3Rs act as inhibitors of autophagy by demonstrating that knocking down IP3Rs or inhibiting their channel activity was sufficient to induce conversion of LC3I to LC3II. (Criollo et al. 2007). Moreover, Beclin-1 is in complex with IP3Rs along with Bcl-2 and perturbation of the Beclin-IP3R-Bcl-2 interaction with Xestospongin B or RNA interference is sufficient for autophagy to occur (Vicencio et al. 2009). Additionally, phosphorylation of Beclin promotes its dissociation from Bcl-xL in order to induce autophagy (Zalckvar et al. 2009). Interestingly, inhibition of IP3Rs or siRNA knockdown of Beclin-1 did not affect ER luminal calcium as measured in aequorin-expressing cells (Criollo et al. 2007; Vicencio et al. 2009). However, because Xestospongin significantly inhibited calcium responses following stimulation with histamine, it is possible that a lack of calcium release promotes autophagy without altering steady state levels under these conditions. Nevertheless, these findings indicate a role for calcium channels and signaling in the induction of autophagy.
CONCLUDING REMARKS
Calcium is a dynamic signal transducing ion that is absolutely required for life. Slight alterations in the frequency and/or amplitude of a calcium signal can lead to apoptosis or autophagy by numerous mechanisms. In addition, abnormal signaling not only alters calcium homeostasis in cells, but may contribute to several pathogenic states such as cancer, heart failure, diabetes, and Alzheimer’s disease (Berridge 2003; Berridge 2010; Huang et al. 2010; Luciani et al. 2009; Roderick and Cook 2008). It is therefore essential to understand the pathways by which calcium regulates life and death decisions, as they will not only provide insight into normal cell physiology, but may also facilitate the development of novel targets and treatments for chronic diseases.
ACKNOWLEDGMENTS
We thank Karen McColl for editing and proofreading this manuscript as well as Hisashi Fujioka of the electron microscopy core facility at Case Western Reserve University.
Footnotes
Editors: Martin D. Bootman, Michael J. Berridge, James W. Putney, and H. Llewelyn Roderick
Additional Perspectives on Calcium Signaling available at www.cshperspectives.org
REFERENCES
- Altamirano F, Oyarce C, Silva P, Toyos M, Wilson C, Lavandero S, Uhlen P, Estrada M 2009. Testosterone induces cardiomyocyte hypertrophy through mammalian target of rapamycin complex 1 pathway. J Endocrinol 202: 299–307 [Abstract] [Google Scholar]
- An J, Chen Y, Huang Z 2004. Critical upstream signals of cytochrome C release induced by a novel Bcl-2 inhibitor. J Biol Chem 279: 19133–19140 [Abstract] [Google Scholar]
- Andreka P, Nadhazi Z, Muzes G, Szantho G, Vandor L, Konya L, Turner MS, Tulassay Z, Bishopric NH 2004. Possible therapeutic targets in cardiac myocyte apoptosis. Curr Pharm Des 10: 2445–2461 [Abstract] [Google Scholar]
- Annis MG, Zamzami N, Zhu W, Penn LZ, Kroemer G, Leber B, Andrews DW 2001. Endoplasmic reticulum localized Bcl-2 prevents apoptosis when redistribution of cytochrome c is a late event. Oncogene 20: 1939–1952 [Abstract] [Google Scholar]
- Aranguiz-Urroz P, Soto D, Contreras A, Troncoso R, Chiong M, Montenegro J, Venegas D, Smolic C, Ayala P, Thomas WG, et al.2009. Differential participation of angiotensin II type 1 and 2 receptors in the regulation of cardiac cell death triggered by angiotensin II. Am J Hypertens 22: 569–576 [Abstract] [Google Scholar]
- Ashwell JD, Lu FW, Vacchio MS 2000. Glucocorticoids in T cell development and function. Annu Rev Immunol 18: 309–345 [Abstract] [Google Scholar]
- Assefa Z, Bultynck G, Szlufcik K, Nadif Kasri N, Vermassen E, Goris J, Missiaen L, Callewaert G, Parys JB, De Smedt H 2004. Caspase-3-induced truncation of type 1 inositol trisphosphate receptor accelerates apoptotic cell death and induces inositol trisphosphate-independent calcium release during apoptosis. J Biol Chem 279: 43227–43236 [Abstract] [Google Scholar]
- Baffy G, Miyashita T, Williamson JR, Reed JC 1993. Apoptosis induced by withdrawal of interleukin-3 (IL-3) from an IL-3-dependent hematopoietic cell line is associated with repartitioning of intracellular calcium and is blocked by enforced Bcl-2 oncoprotein production. J Biol Chem 268: 6511–6519 [Abstract] [Google Scholar]
- Barouch LA, Harrison RW, Skaf MW, Rosas GO, Cappola TP, Kobeissi ZA, Hobai IA, Lemmon CA, Burnett AL, O’Rourke B, et al.2002. Nitric oxide regulates the heart by spatial confinement of nitric oxide synthase isoforms. Nature 416: 337–339 [Abstract] [Google Scholar]
- Barsoum MJ, Yuan H, Gerencser AA, Liot G, Kushnareva Y, Graber S, Kovacs I, Lee WD, Waggoner J, Cui J, et al.2006. Nitric oxide-induced mitochondrial fission is regulated by dynamin-related GTPases in neurons. Embo J 25: 3900–3911 [Europe PMC free article] [Abstract] [Google Scholar]
- Baus E, Andris F, Dubois PM, Urbain J, Leo O 1996. Dexamethasone inhibits the early steps of antigen receptor signaling in activated T lymphocytes. J Immunol 156: 4555–4561 [Abstract] [Google Scholar]
- Benten WP, Lieberherr M, Sekeris CE, Wunderlich F 1997. Testosterone induces Ca2+ influx via non-genomic surface receptors in activated T cells. FEBS Lett 407: 211–214 [Abstract] [Google Scholar]
- Berg I, Potter BV, Mayr GW, Guse AH 2000. Nicotinic acid adenine dinucleotide phosphate (NAADP(+)) is an essential regulator of T-lymphocyte Ca2+-signaling. J Cell Biol 150: 581–588 [Europe PMC free article] [Abstract] [Google Scholar]
- Bernales S, McDonald KL, Walter P 2006. Autophagy counterbalances endoplasmic reticulum expansion during the unfolded protein response. PLoS Biol 4: e423. [Europe PMC free article] [Abstract] [Google Scholar]
- Berridge MJ 1997. Lymphocyte activation in health and disease. Crit Rev Immunol 17: 155–178 [Abstract] [Google Scholar]
- Berridge MJ 2003. Cardiac calcium signalling. Biochem Soc Trans 31: 930–933 [Abstract] [Google Scholar]
- Berridge MJ 2010. Calcium hypothesis of Alzheimer’s disease. Pflugers Arch 459: 441–449 [Abstract] [Google Scholar]
- Bian X, Hughes FM Jr, Huang Y, Cidlowski JA, Putney JW Jr 1997. Roles of cytoplasmic Ca2+ and intracellular Ca2+ stores in induction and suppression of apoptosis in S49 cells. Am J Physiol 272: C1241–1249 [Abstract] [Google Scholar]
- Bootman MD, Taylor CW, Berridge MJ 1992. The thiol reagent, thimerosal, evokes Ca2+ spikes in HeLa cells by sensitizing the inositol 1,4,5-trisphosphate receptor. J Biol Chem 267: 25113–25119 [Abstract] [Google Scholar]
- Brooks KJ, Hargreaves IP, Bates TE 2000. Nitric-oxide-induced inhibition of mitochondrial complexes following aglycaemic hypoxia in neonatal cortical rat brain slices. Dev Neurosci 22: 359–365 [Abstract] [Google Scholar]
- Bultynck G, Szlufcik K, Kasri NN, Assefa Z, Callewaert G, Missiaen L, Parys JB, De Smedt H 2004. Thimerosal stimulates Ca2+ flux through inositol 1,4,5-trisphosphate receptor type 1, but not type 3, via modulation of an isoform-specific Ca2+-dependent intramolecular interaction. Biochem J 381: 87–96 [Europe PMC free article] [Abstract] [Google Scholar]
- Cameron AM, Steiner JP, Roskams AJ, Ali SM, Ronnett GV, Snyder SH 1995. Calcineurin associated with the inositol 1,4,5-trisphosphate receptor-FKBP12 complex modulates Ca2+ flux. Cell 83: 463–472 [Abstract] [Google Scholar]
- Cante-Barrett K, Gallo EM, Winslow MM, Crabtree GR 2006. Thymocyte negative selection is mediated by protein kinase C- and Ca2+-dependent transcriptional induction of bim. J Immunol 176: 2299–2306 [Abstract] [Google Scholar]
- Chae HJ, Kim HR, Xu C, Bailly-Maitre B, Krajewska M, Krajewski S, Banares S, Cui J, Digicaylioglu M, Ke N, et al.2004. BI-1 regulates an apoptosis pathway linked to endoplasmic reticulum stress. Mol Cell 15: 355–366 [Abstract] [Google Scholar]
- Chai J, Xiong Q, Zhang P, Zheng R, Peng J, Jiang S 2009. Induction of Ca2+ signal mediated apoptosis and alteration of IP3R1 and SERCA1 expression levels by stress hormone in differentiating C2C12 myoblasts. Gen Comp Endocrinol 166: 241–249 [Abstract] [Google Scholar]
- Chen R, Valencia I, Zhong F, McColl KS, Roderick HL, Bootman MD, Berridge MJ, Conway SJ, Holmes AB, Mignery GA, et al.2004. Bcl-2 functionally interacts with inositol 1,4,5-trisphosphate receptors to regulate calcium release from the ER in response to inositol 1,4,5-trisphosphate. J Cell Biol 166: 193–203 [Europe PMC free article] [Abstract] [Google Scholar]
- Cheng EH, Wei MC, Weiler S, Flavell RA, Mak TW, Lindsten T, Korsmeyer SJ 2001. BCL-2, BCL-X(L) sequester BH3 domain-only molecules preventing BAX- and BAK-mediated mitochondrial apoptosis. Mol Cell 8: 705–711 [Abstract] [Google Scholar]
- Chipuk JE, Moldoveanu T, Llambi F, Parsons MJ, Green DR 2010. The BCL-2 family reunion. Mol Cell 37: 299–310 [Europe PMC free article] [Abstract] [Google Scholar]
- Cigola E, Kajstura J, Li B, Meggs LG, Anversa P 1997. Angiotensin II activates programmed myocyte cell death in vitro. Exp Cell Res 231: 363–371 [Abstract] [Google Scholar]
- Cohen JJ, Duke RC 1984. Glucocorticoid activation of a calcium-dependent endonuclease in thymocyte nuclei leads to cell death. J Immunol 132: 38–42 [Abstract] [Google Scholar]
- Copetti T, Bertoli C, Dalla E, Demarchi F, Schneider C 2009a. p65/RelA modulates BECN1 transcription and autophagy. Mol Cell Biol 29: 2594–2608 [Europe PMC free article] [Abstract] [Google Scholar]
- Copetti T, Demarchi F, Schneider C 2009b. p65/RelA binds and activates the beclin 1 promoter. Autophagy 5: 858–859 [Abstract] [Google Scholar]
- Cory S, Adams JM 2002. The Bcl2 family: regulators of the cellular life-or-death switch. Nat Rev Cancer 2: 647–656 [Abstract] [Google Scholar]
- Criollo A, Maiuri MC, Tasdemir E, Vitale I, Fiebig AA, Andrews D, Molgo J, Diaz J, Lavandero S, Harper F, et al.2007. Regulation of autophagy by the inositol trisphosphate receptor. Cell Death Differ 14: 1029–1039 [Abstract] [Google Scholar]
- Dammermann W, Zhang B, Nebel M, Cordiglieri C, Odoardi F, Kirchberger T, Kawakami N, Dowden J, Schmid F, Dornmair K, et al.2009. NAADP-mediated Ca2+ signaling via type 1 ryanodine receptor in T cells revealed by a synthetic NAADP antagonist. Proc Natl Acad Sci U S A 106: 10678–10683 [Europe PMC free article] [Abstract] [Google Scholar]
- Davidson SM, Duchen MR 2006. Calcium microdomains and oxidative stress. Cell Calcium 40: 561–574 [Abstract] [Google Scholar]
- Davis MC, McColl KS, Zhong F, Wang Z, Malone MH, Distelhorst CW 2008. Dexamethasone-induced inositol 1,4,5-trisphosphate receptor elevation in murine lymphoma cells is not required for dexamethasone-mediated calcium elevation and apoptosis. J Biol Chem 283: 10357–10365 [Europe PMC free article] [Abstract] [Google Scholar]
- Dedkova EN, Blatter LA 2005. Modulation of mitochondrial Ca2+ by nitric oxide in cultured bovine vascular endothelial cells. Am J Physiol Cell Physiol 289: C836–845 [Abstract] [Google Scholar]
- DeSouza N, Reiken S, Ondrias K, Yang YM, Matkovich S, Marks AR 2002. Protein kinase A and two phosphatases are components of the inositol 1,4,5-trisphosphate receptor macromolecular signaling complex. J Biol Chem 277: 39397–39400 [Abstract] [Google Scholar]
- Devadas S, Zaritskaya L, Rhee SG, Oberley L, Williams MS 2002. Discrete generation of superoxide and hydrogen peroxide by T cell receptor stimulation: selective regulation of mitogen-activated protein kinase activation and fas ligand expression. J Exp Med 195: 59–70 [Europe PMC free article] [Abstract] [Google Scholar]
- Diaz-Prieto N, Herrera-Peco I, de Diego AM, Ruiz-Nuno A, Gallego-Sandin S, Lopez MG, Garcia AG, Cano-Abad MF 2008. Bcl-2 mitigates Ca2+ entry and mitochondrial Ca2+ overload through downregulation of L-type Ca2+ channels in PC12 cells. Cell Calcium 44: 339–352 [Abstract] [Google Scholar]
- Dimmeler S, Haendeler J, Nehls M, Zeiher AM 1997. Suppression of apoptosis by nitric oxide via inhibition of interleukin-1beta-converting enzyme (ICE)-like and cysteine protease protein (CPP)-32-like proteases. J Exp Med 185: 601–607 [Europe PMC free article] [Abstract] [Google Scholar]
- Dremina ES, Sharov VS, Kumar K, Zaidi A, Michaelis EK, Schoneich C 2004. Anti-apoptotic protein Bcl-2 interacts with and destabilizes the sarcoplasmic/endoplasmic reticulum Ca2+-ATPase (SERCA). Biochem J 383: 361–370 [Europe PMC free article] [Abstract] [Google Scholar]
- Dremina ES, Sharov VS, Schoneich C 2006. Displacement of SERCA from SR lipid caveolae-related domains by Bcl-2: a possible mechanism for SERCA inactivation. Biochemistry 45: 175–184 [Abstract] [Google Scholar]
- Eckenrode EF, Yang J, Velmurugan GV, Foskett JK, White C 2010. Apoptosis protection by Mcl-1 and Bcl-2 modulation of inositol 1,4,5-trisphosphate receptor-dependent Ca2+ signaling. J Biol Chem 285: 13678–13684 [Europe PMC free article] [Abstract] [Google Scholar]
- Erin N, Bronson SK, Billingsley ML 2003. Calcium-dependent interaction of calcineurin with Bcl-2 in neuronal tissue. Neuroscience 117: 541–555 [Abstract] [Google Scholar]
- Estrada M, Varshney A, Ehrlich BE 2006. Elevated testosterone induces apoptosis in neuronal cells. J Biol Chem 281: 25492–25501 [Abstract] [Google Scholar]
- Fliegert R, Gasser A, Guse AH 2007. Regulation of calcium signalling by adenine-based second messengers. Biochem Soc Trans 35: 109–114 [Abstract] [Google Scholar]
- Foyouzi-Youssefi R, Arnaudeau S, Borner C, Kelley WL, Tschopp J, Lew DP, Demaurex N, Krause KH 2000. Bcl-2 decreases the free Ca2+ concentration within the endoplasmic reticulum. Proc Natl Acad Sci U S A 97: 5723–5728 [Europe PMC free article] [Abstract] [Google Scholar]
- Galione A, Petersen OH 2005. The NAADP receptor: new receptors or new regulation? Mol Interv 5: 73–79 [Abstract] [Google Scholar]
- Gallo EM, Cante-Barrett K, Crabtree GR 2006. Lymphocyte calcium signaling from membrane to nucleus. Nat Immunol 7: 25–32 [Abstract] [Google Scholar]
- Gao W, Ding WX, Stolz DB, Yin XM 2008. Induction of macroautophagy by exogenously introduced calcium. Autophagy 4: 754–761 [Europe PMC free article] [Abstract] [Google Scholar]
- Germain M, Mathai JP, McBride HM, Shore GC 2005. Endoplasmic reticulum BIK initiates DRP1-regulated remodelling of mitochondrial cristae during apoptosis. Embo J 24: 1546–1556 [Europe PMC free article] [Abstract] [Google Scholar]
- Goldenberg I, Grossman E, Jacobson KA, Shneyvays V, Shainberg A 2001. Angiotensin II-induced apoptosis in rat cardiomyocyte culture: a possible role of AT1 and AT2 receptors. J Hypertens 19: 1681–1689 [Abstract] [Google Scholar]
- Gradman AH 2009. Role of angiotensin II type 1 receptor antagonists in the treatment of hypertension in patients aged >or=65 years. Drugs Aging 26: 751–767 [Abstract] [Google Scholar]
- Green DR 2003. Overview: apoptotic signaling pathways in the immune system. Immunol Rev 193: 5–9 [Abstract] [Google Scholar]
- Green DR 2005. Apoptotic pathways: ten minutes to dead. Cell 121: 671–674 [Abstract] [Google Scholar]
- Guse AH 2009. Second messenger signaling: multiple receptors for NAADP. Curr Biol 19: R521–523 [Abstract] [Google Scholar]
- Guse AH, da Silva CP, Berg I, Skapenko AL, Weber K, Heyer P, Hohenegger M, Ashamu GA, Schulze-Koops H, Potter BV, et al.1999. Regulation of calcium signalling in T lymphocytes by the second messenger cyclic ADP-ribose. Nature 398: 70–73 [Abstract] [Google Scholar]
- Hajnoczky G, Csordas G, Das S, Garcia-Perez C, Saotome M, Sinha Roy S, Yi M 2006. Mitochondrial calcium signalling and cell death: approaches for assessing the role of mitochondrial Ca2+ uptake in apoptosis. Cell Calcium 40: 553–560 [Europe PMC free article] [Abstract] [Google Scholar]
- Hanson CJ, Bootman MD, Distelhorst CW, Wojcikiewicz RJ, Roderick HL 2008. Bcl-2 suppresses Ca2+ release through inositol 1,4,5-trisphosphate receptors and inhibits Ca2+ uptake by mitochondria without affecting ER calcium store content. Cell Calcium 44: 324–338 [Abstract] [Google Scholar]
- Hanson CJ, Bootman MD, Roderick HL 2004. Cell signalling: IP3 receptors channel calcium into cell death. Curr Biol 14: R933–935 [Abstract] [Google Scholar]
- Harr MW, Rong Y, Bootman MD, Roderick HL, Distelhorst CW 2009. Glucocorticoid-mediated inhibition of Lck modulates the pattern of T cell receptor-induced calcium signals by down-regulating inositol 1,4,5-trisphosphate receptors. J Biol Chem 284: 31860–31871 [Europe PMC free article] [Abstract] [Google Scholar]
- Herold MJ, McPherson KG, Reichardt HM 2006. Glucocorticoids in T cell apoptosis and function. Cell Mol Life Sci 63: 60–72 [Europe PMC free article] [Abstract] [Google Scholar]
- Hildeman DA, Mitchell T, Kappler J, Marrack P 2003. T cell apoptosis and reactive oxygen species. J Clin Invest 111: 575–581 [Europe PMC free article] [Abstract] [Google Scholar]
- Hirota J, Furuichi T, Mikoshiba K 1999. Inositol 1,4,5-trisphosphate receptor type 1 is a substrate for caspase-3 and is cleaved during apoptosis in a caspase-3-dependent manner. J Biol Chem 274: 34433–34437 [Abstract] [Google Scholar]
- Hogquist KA 2001. Signal strength in thymic selection and lineage commitment. Curr Opin Immunol 13: 225–231 [Abstract] [Google Scholar]
- Hoyer-Hansen M, Bastholm L, Szyniarowski P, Campanella M, Szabadkai G, Farkas T, Bianchi K, Fehrenbacher N, Elling F, Rizzuto R, et al.2007. Control of macroautophagy by calcium, calmodulin-dependent kinase kinase-beta, and Bcl-2. Mol Cell 25: 193–205 [Abstract] [Google Scholar]
- Hoyer-Hansen M, Jaattela M 2007. AMP-activated protein kinase: a universal regulator of autophagy? Autophagy 3: 381–383 [Abstract] [Google Scholar]
- Huang CJ, Gurlo T, Haataja L, Costes S, Daval M, Ryazantsev S, Wu X, Butler AE, Butler PC 2010. Calcium-activated calpain-2 is a mediator of beta cell dysfunction and apoptosis in type 2 diabetes. J Biol Chem 285: 339–348 [Europe PMC free article] [Abstract] [Google Scholar]
- Jamieson CA, Yamamoto KR 2000. Crosstalk pathway for inhibition of glucocorticoid-induced apoptosis by T cell receptor signaling. Proc Natl Acad Sci U S A 97: 7319–7324 [Europe PMC free article] [Abstract] [Google Scholar]
- Jayaraman T, Marks AR 1997. T cells deficient in inositol 1,4,5-trisphosphate receptor are resistant to apoptosis. Mol Cell Biol 17: 3005–3012 [Europe PMC free article] [Abstract] [Google Scholar]
- Jiao J, Huang X, Feit-Leithman RA, Neve RL, Snider W, Dartt DA, Chen DF 2005. Bcl-2 enhances Ca2+ signaling to support the intrinsic regenerative capacity of CNS axons. Embo J 24: 1068–1078 [Europe PMC free article] [Abstract] [Google Scholar]
- Joseph SK, Nakao SK, Sukumvanich S 2006. Reactivity of free thiol groups in type-I inositol trisphosphate receptors. Biochem J 393: 575–582 [Europe PMC free article] [Abstract] [Google Scholar]
- Kaiser N, Edelman IS 1977. Calcium dependence of glucocorticoid-induced lymphocytolysis. Proc Natl Acad Sci U S A 74: 638–642 [Europe PMC free article] [Abstract] [Google Scholar]
- Kajstura J, Cigola E, Malhotra A, Li P, Cheng W, Meggs LG, Anversa P 1997. Angiotensin II induces apoptosis of adult ventricular myocytes in vitro. J Mol Cell Cardiol 29: 859–870 [Abstract] [Google Scholar]
- Khan SA, Hare JM 2003. The role of nitric oxide in the physiological regulation of Ca2+ cycling. Curr Opin Drug Discov Devel 6: 658–666 [Abstract] [Google Scholar]
- Kim HR, Lee GH, Ha KC, Ahn T, Moon JY, Lee BJ, Cho SG, Kim S, Seo YR, Shin YJ, et al.2008. Bax Inhibitor-1 Is a pH-dependent regulator of Ca2+ channel activity in the endoplasmic reticulum. J Biol Chem 283: 15946–15955 [Europe PMC free article] [Abstract] [Google Scholar]
- Klee M, Pallauf K, Alcala S, Fleischer A, Pimentel-Muinos FX 2009. Mitochondrial apoptosis induced by BH3-only molecules in the exclusive presence of endoplasmic reticular Bak. Embo J 28: 1757–1768 [Europe PMC free article] [Abstract] [Google Scholar]
- Klionsky DJ, Abeliovich H, Agostinis P, Agrawal DK, Aliev G, Askew DS, Baba M, Baehrecke EH, Bahr BA, Ballabio A, et al.2008. Guidelines for the use and interpretation of assays for monitoring autophagy in higher eukaryotes. Autophagy 4: 151–175 [Europe PMC free article] [Abstract] [Google Scholar]
- Kockskamper J, Zima AV, Roderick HL, Pieske B, Blatter LA, Bootman MD 2008. Emerging roles of inositol 1,4,5-trisphosphate signaling in cardiac myocytes. J Mol Cell Cardiol 45: 128–147 [Europe PMC free article] [Abstract] [Google Scholar]
- Kouroku Y, Fujita E, Tanida I, Ueno T, Isoai A, Kumagai H, Ogawa S, Kaufman RJ, Kominami E, Momoi T 2007. ER stress (PERK/eIF2alpha phosphorylation) mediates the polyglutamine-induced LC3 conversion, an essential step for autophagy formation. Cell Death Differ 14: 230–239 [Abstract] [Google Scholar]
- Kruman II, Mattson MP 1999. Pivotal role of mitochondrial calcium uptake in neural cell apoptosis and necrosis. J Neurochem 72: 529–540 [Abstract] [Google Scholar]
- Laane E, Tamm KP, Buentke E, Ito K, Kharaziha P, Oscarsson J, Corcoran M, Bjorklund AC, Hultenby K, Lundin J, et al.2009. Cell death induced by dexamethasone in lymphoid leukemia is mediated through initiation of autophagy. Cell Death Differ 16: 1018–1029 [Abstract] [Google Scholar]
- Lam M, Dubyak G, Chen L, Nunez G, Miesfeld RL, Distelhorst CW 1994. Evidence that BCL-2 represses apoptosis by regulating endoplasmic reticulum-associated Ca2+ fluxes. Proc Natl Acad Sci U S A 91: 6569–6573 [Europe PMC free article] [Abstract] [Google Scholar]
- Lam M, Dubyak G, Distelhorst CW 1993. Effect of glucocorticosteroid treatment on intracellular calcium homeostasis in mouse lymphoma cells. Mol Endocrinol 7: 686–693 [Abstract] [Google Scholar]
- Latour S, Veillette A 2001. Proximal protein tyrosine kinases in immunoreceptor signaling. Curr Opin Immunol 13: 299–306 [Abstract] [Google Scholar]
- Levine B, Klionsky DJ 2004. Development by self-digestion: molecular mechanisms and biological functions of autophagy. Dev Cell 6: 463–477 [Abstract] [Google Scholar]
- Levine B, Yuan J 2005. Autophagy in cell death: an innocent convict? J Clin Invest 115: 2679–2688 [Europe PMC free article] [Abstract] [Google Scholar]
- Lewis RS 2001. Calcium signaling mechanisms in T lymphocytes. Annu Rev Immunol 19: 497–521 [Abstract] [Google Scholar]
- Li C, Wang X, Vais H, Thompson CB, Foskett JK, White C 2007. Apoptosis regulation by Bcl-x(L) modulation of mammalian inositol 1,4,5-trisphosphate receptor channel isoform gating. Proc Natl Acad Sci U S A 104: 12565–12570 [Europe PMC free article] [Abstract] [Google Scholar]
- Lowenberg M, Tuynman J, Bilderbeek J, Gaber T, Buttgereit F, van Deventer S, Peppelenbosch M, Hommes D 2005. Rapid immunosuppressive effects of glucocorticoids mediated through Lck and Fyn. Blood 106: 1703–1710 [Abstract] [Google Scholar]
- Lu J, Quearry B, Harada H 2006. p38-MAP kinase activation followed by BIM induction is essential for glucocorticoid-induced apoptosis in lymphoblastic leukemia cells. FEBS Lett 580: 3539–3544 [Abstract] [Google Scholar]
- Luciani DS, Gwiazda KS, Yang TL, Kalynyak TB, Bychkivska Y, Frey MH, Jeffrey KD, Sampaio AV, Underhill TM, Johnson JD 2009. Roles of IP3R and RyR Ca2+ channels in endoplasmic reticulum stress and beta-cell death. Diabetes 58: 422–432 [Europe PMC free article] [Abstract] [Google Scholar]
- Ludwinski MW, Sun J, Hilliard B, Gong S, Xue F, Carmody RJ, DeVirgiliis J, Chen YH 2009. Critical roles of Bim in T cell activation and T cell-mediated autoimmune inflammation in mice. J Clin Invest 119: 1706–1713 [Europe PMC free article] [Abstract] [Google Scholar]
- Magnelli L, Cinelli M, Turchetti A, Chiarugi VP 1994. Bcl-2 overexpression abolishes early calcium waving preceding apoptosis in NIH-3T3 murine fibroblasts. Biochem Biophys Res Commun 204: 84–90 [Abstract] [Google Scholar]
- Mandic A, Hansson J, Linder S, Shoshan MC 2003. Cisplatin induces endoplasmic reticulum stress and nucleus-independent apoptotic signaling. J Biol Chem 278: 9100–9106 [Abstract] [Google Scholar]
- Mariathasan S, Bachmann MF, Bouchard D, Ohteki T, Ohashi PS 1998. Degree of TCR internalization and Ca2+ flux correlates with thymocyte selection. J Immunol 161: 6030–6037 [Abstract] [Google Scholar]
- Massion PB, Feron O, Dessy C, Balligand JL 2003. Nitric oxide and cardiac function: ten years after, and continuing. Circ Res 93: 388–398 [Abstract] [Google Scholar]
- Mathai JP, Germain M, Shore GC 2005. BH3-only BIK regulates BAX,BAK-dependent release of Ca2+ from endoplasmic reticulum stores and mitochondrial apoptosis during stress-induced cell death. J Biol Chem 280: 23829–23836 [Abstract] [Google Scholar]
- Mattiazzi A 1997. Positive inotropic effect of angiotensin II. Increases in intracellular Ca2+ or changes in myofilament Ca2+ responsiveness? J Pharmacol Toxicol Methods 37: 205–214 [Abstract] [Google Scholar]
- McConkey DJ, Nicotera P, Hartzell P, Bellomo G, Wyllie AH, Orrenius S 1989. Glucocorticoids activate a suicide process in thymocytes through an elevation of cytosolic Ca2+ concentration. Arch Biochem Biophys 269: 365–370 [Abstract] [Google Scholar]
- Minagawa N, Kruglov EA, Dranoff JA, Robert ME, Gores GJ, Nathanson MH 2005. The anti-apoptotic protein Mcl-1 inhibits mitochondrial Ca2+ signals. J Biol Chem 280: 33637–33644 [Abstract] [Google Scholar]
- Mizushima N 2007. Autophagy: process and function. Genes Dev 21: 2861–2873 [Abstract] [Google Scholar]
- Mizushima N, Levine B, Cuervo AM, Klionsky DJ 2008. Autophagy fights disease through cellular self-digestion. Nature 451: 1069–1075 [Europe PMC free article] [Abstract] [Google Scholar]
- Morishima N, Nakanishi K, Takenouchi H, Shibata T, Yasuhiko Y 2002. An endoplasmic reticulum stress-specific caspase cascade in apoptosis. Cytochrome c-independent activation of caspase-9 by caspase-12. J Biol Chem 277: 34287–34294 [Abstract] [Google Scholar]
- Morishima N, Nakanishi K, Tsuchiya K, Shibata T, Seiwa E 2004. Translocation of Bim to the endoplasmic reticulum (ER) mediates ER stress signaling for activation of caspase-12 during ER stress-induced apoptosis. J Biol Chem 279: 50375–50381 [Abstract] [Google Scholar]
- Mustelin T, Tasken K 2003. Positive and negative regulation of T-cell activation through kinases and phosphatases. Biochem J 371: 15–27 [Europe PMC free article] [Abstract] [Google Scholar]
- Nakagawa T, Yuan J 2000. Cross-talk between two cysteine protease families. Activation of caspase-12 by calpain in apoptosis. J Cell Biol 150: 887–894 [Europe PMC free article] [Abstract] [Google Scholar]
- Nakagawa T, Zhu H, Morishima N, Li E, Xu J, Yankner BA, Yuan J 2000. Caspase-12 mediates endoplasmic-reticulum-specific apoptosis and cytotoxicity by amyloid-beta. Nature 403: 98–103 [Abstract] [Google Scholar]
- Norberg E, Gogvadze V, Ott M, Horn M, Uhlen P, Orrenius S, Zhivotovsky B 2008. An increase in intracellular Ca2+ is required for the activation of mitochondrial calpain to release AIF during cell death. Cell Death Differ 15: 1857–1864 [Abstract] [Google Scholar]
- Nutt LK, Chandra J, Pataer A, Fang B, Roth JA, Swisher SG, O’Neil RG, McConkey DJ 2002a. Bax-mediated Ca2+ mobilization promotes cytochrome c release during apoptosis. J Biol Chem 277: 20301–20308 [Abstract] [Google Scholar]
- Nutt LK, Pataer A, Pahler J, Fang B, Roth J, McConkey DJ, Swisher SG 2002b. Bax and Bak promote apoptosis by modulating endoplasmic reticular and mitochondrial Ca2+ stores. J Biol Chem 277: 9219–9225 [Abstract] [Google Scholar]
- Oakes SA, Scorrano L, Opferman JT, Bassik MC, Nishino M, Pozzan T, Korsmeyer SJ 2005. Proapoptotic BAX and BAK regulate the type 1 inositol trisphosphate receptor and calcium leak from the endoplasmic reticulum. Proc Natl Acad Sci U S A 102: 105–110 [Europe PMC free article] [Abstract] [Google Scholar]
- Ogata M, Hino S, Saito A, Morikawa K, Kondo S, Kanemoto S, Murakami T, Taniguchi M, Tanii I, Yoshinaga K, et al.2006. Autophagy is activated for cell survival after endoplasmic reticulum stress. Mol Cell Biol 26: 9220–9231 [Europe PMC free article] [Abstract] [Google Scholar]
- Orrenius S, McConkey DJ, Nicotera P 1991. Role of calcium in toxic and programmed cell death. Adv Exp Med Biol 283: 419–425 [Abstract] [Google Scholar]
- Pacher P, Hajnoczky G 2001. Propagation of the apoptotic signal by mitochondrial waves. Embo J 20: 4107–4121 [Europe PMC free article] [Abstract] [Google Scholar]
- Palacios EH, Weiss A 2004. Function of the Src-family kinases, Lck and Fyn, in T-cell development and activation. Oncogene 23: 7990–8000 [Abstract] [Google Scholar]
- Palmer AE, Jin C, Reed JC, Tsien RY 2004. Bcl-2-mediated alterations in endoplasmic reticulum Ca2+ analyzed with an improved genetically encoded fluorescent sensor. Proc Natl Acad Sci U S A 101: 17404–17409 [Europe PMC free article] [Abstract] [Google Scholar]
- Palomeque J, Delbridge L, Petroff MV 2009. Angiotensin II: a regulator of cardiomyocyte function and survival. Front Biosci 14: 5118–5133 [Abstract] [Google Scholar]
- Pinton P, Ferrari D, Magalhaes P, Schulze-Osthoff K, Di Virgilio F, Pozzan T, Rizzuto R 2000. Reduced loading of intracellular Ca2+ stores and downregulation of capacitative Ca2+ influx in Bcl-2-overexpressing cells. J Cell Biol 148: 857–862 [Europe PMC free article] [Abstract] [Google Scholar]
- Pinton P, Giorgi C, Siviero R, Zecchini E, Rizzuto R 2008. Calcium and apoptosis: ER-mitochondria Ca2+ transfer in the control of apoptosis. Oncogene 27: 6407–6418 [Europe PMC free article] [Abstract] [Google Scholar]
- Pua HH, Dzhagalov I, Chuck M, Mizushima N, He YW 2007. A critical role for the autophagy gene Atg5 in T cell survival and proliferation. J Exp Med 204: 25–31 [Europe PMC free article] [Abstract] [Google Scholar]
- Randriamampita C, Trautmann A 2003. Ca2+ signals and T lymphocytes “New mechanisms and functions in Ca2+ signaling”. Biol of the Cell 96: 69–78 [Abstract] [Google Scholar]
- Rao RV, Castro-Obregon S, Frankowski H, Schuler M, Stoka V, del Rio G, Bredesen DE, Ellerby HM 2002. Coupling endoplasmic reticulum stress to the cell death program. An Apaf-1-independent intrinsic pathway. J Biol Chem 277: 21836–21842 [Abstract] [Google Scholar]
- Razavi HM, Hamilton JA, Feng Q 2005. Modulation of apoptosis by nitric oxide: implications in myocardial ischemia and heart failure. Pharmacol Ther 106: 147–162 [Abstract] [Google Scholar]
- Rizzuto R, Pinton P, Ferrari D, Chami M, Szabadkai G, Magalhaes PJ, Di Virgilio F, Pozzan T 2003. Calcium and apoptosis: facts and hypotheses. Oncogene 22: 8619–8627 [Abstract] [Google Scholar]
- Roderick HL, Cook SJ 2008. Ca2+ signalling checkpoints in cancer: remodelling Ca2+ for cancer cell proliferation and survival. Nat Rev Cancer 8: 361–375 [Abstract] [Google Scholar]
- Rong Y, Distelhorst CW 2007. Bcl-2 Protein Family Members: Versatile Regulators of Calcium Signaling in Cell Survival and Apoptosis. Annu Rev Physiol 70: 73–91 [Abstract] [Google Scholar]
- Rong YP, Aromolaran AS, Bultynck G, Zhong F, Li X, McColl K, Matsuyama S, Herlitze S, Roderick HL, Bootman MD, et al.2008. Targeting Bcl-2-IP3 receptor interaction to reverse Bcl-2’s inhibition of apoptotic calcium signals. Mol Cell 31: 255–265 [Abstract] [Google Scholar]
- Rong YP, Bultynck G, Aromolaran AS, Zhong F, Parys JB, De Smedt H, Mignery GA, Roderick HL, Bootman MD, Distelhorst CW 2009. The BH4 domain of Bcl-2 inhibits ER calcium release and apoptosis by binding the regulatory and coupling domain of the IP3 receptor. Proc Natl Acad Sci U S A 106: 14397–14402 [Europe PMC free article] [Abstract] [Google Scholar]
- Sarkar S, Floto RA, Berger Z, Imarisio S, Cordenier A, Pasco M, Cook LJ, Rubinsztein DC 2005. Lithium induces autophagy by inhibiting inositol monophosphatase. J Cell Biol 170: 1101–1111 [Europe PMC free article] [Abstract] [Google Scholar]
- Sarkar S, Rubinsztein DC 2006. Inositol and IP3 levels regulate autophagy: biology and therapeutic speculations. Autophagy 2: 132–134 [Abstract] [Google Scholar]
- Scherz-Shouval R, Shvets E, Fass E, Shorer H, Gil L, Elazar Z 2007. Reactive oxygen species are essential for autophagy and specifically regulate the activity of Atg4. Embo J 26: 1749–1760 [Europe PMC free article] [Abstract] [Google Scholar]
- Schrodl K, Oelmez H, Edelmann M, Huber RM, Bergner A 2009. Altered Ca2+-homeostasis of cisplatin-treated and low level resistant non-small-cell and small-cell lung cancer cells. Cell Oncol 31: 301–315 [Abstract] [Google Scholar]
- Scorrano L, Oakes SA, Opferman JT, Cheng EH, Sorcinelli MD, Pozzan T, Korsmeyer SJ 2003. BAX and BAK regulation of endoplasmic reticulum Ca2+: a control point for apoptosis. Science 300: 135–139 [Abstract] [Google Scholar]
- Sears CE, Bryant SM, Ashley EA, Lygate CA, Rakovic S, Wallis HL, Neubauer S, Terrar DA, Casadei B 2003. Cardiac neuronal nitric oxide synthase isoform regulates myocardial contraction and calcium handling. Circ Res 92: e52–59 [Abstract] [Google Scholar]
- Seo YW, Woo HN, Piya S, Moon AR, Oh JW, Yun CW, Kim KK, Min JY, Jeong SY, Chung S, et al.2009. The cell death-inducing activity of the peptide containing Noxa mitochondrial-targeting domain is associated with calcium release. Cancer Res 69: 8356–8365 [Abstract] [Google Scholar]
- Shibasaki F, Kondo E, Akagi T, McKeon F 1997. Suppression of signalling through transcription factor NF-AT by interactions between calcineurin and Bcl-2. Nature 386: 728–731 [Abstract] [Google Scholar]
- Sinha S, Levine B 2008. The autophagy effector Beclin 1: a novel BH3-only protein. Oncogene 27: S137–148 [Europe PMC free article] [Abstract] [Google Scholar]
- Splettstoesser F, Florea AM, Busselberg D 2007. IP(3) receptor antagonist, 2-APB, attenuates cisplatin induced Ca2+-influx in HeLa-S3 cells and prevents activation of calpain and induction of apoptosis. Br J Pharmacol 151: 1176–1186 [Europe PMC free article] [Abstract] [Google Scholar]
- Starr TK, Jameson SC, Hogquist KA 2003. Positive and negative selection of T cells. Annu Rev Immunol 21: 139–176 [Abstract] [Google Scholar]
- Sun J, Xu L, Eu JP, Stamler JS, Meissner G 2001. Classes of thiols that influence the activity of the skeletal muscle calcium release channel. J Biol Chem 276: 15625–15630 [Abstract] [Google Scholar]
- Swerdlow S, McColl K, Rong Y, Lam M, Gupta A, Distelhorst CW 2008. Apoptosis inhibition by Bcl-2 gives way to autophagy in glucocorticoid-treated lymphocytes. Autophagy 4: 612–620 [Europe PMC free article] [Abstract] [Google Scholar]
- Szalai G, Krishnamurthy R, Hajnoczky G 1999. Apoptosis driven by IP(3)-linked mitochondrial calcium signals. Embo J 18: 6349–6361 [Europe PMC free article] [Abstract] [Google Scholar]
- Szegezdi E, Fitzgerald U, Samali A 2003. Caspase-12 and ER-stress-mediated apoptosis: the story so far. Ann N Y Acad Sci 1010: 186–194 [Abstract] [Google Scholar]
- Szlufcik K, Missiaen L, Parys JB, Callewaert G, De Smedt H 2006. Uncoupled IP3 receptor can function as a Ca2+-leak channel: cell biological and pathological consequences. Biol Cell 98: 1–14 [Abstract] [Google Scholar]
- Tolosa E, Ashwell JD 1999. Thymus-derived glucocorticoids and the regulation of antigen-specific T-cell development. Neuroimmunomodulation 6: 90–96 [Abstract] [Google Scholar]
- Tsujimoto Y, Cossman J, Jaffe E, Croce CM 1985. Involvement of the bcl-2 gene in human follicular lymphoma. Science 228: 1440–1443 [Abstract] [Google Scholar]
- Tsunoda T, Koga H, Yokomizo A, Tatsugami K, Eto M, Inokuchi J, Hirata A, Masuda K, Okumura K, Naito S 2005. Inositol 1,4,5-trisphosphate (IP3) receptor type1 (IP3R1) modulates the acquisition of cisplatin resistance in bladder cancer cell lines. Oncogene 24: 1396–1402 [Abstract] [Google Scholar]
- Van Laethem F, Baus E, Smyth LA, Andris F, Bex F, Urbain J, Kioussis D, Leo O 2001. Glucocorticoids attenuate T cell receptor signaling. J Exp Med 193: 803–814 [Europe PMC free article] [Abstract] [Google Scholar]
- Vanden Abeele F, Skryma R, Shuba Y, Van Coppenolle F, Slomianny C, Roudbaraki M, Mauroy B, Wuytack F, Prevarskaya N 2002. Bcl-2-dependent modulation of Ca2+ homeostasis and store-operated channels in prostate cancer cells. Cancer Cell 1: 169–179 [Abstract] [Google Scholar]
- Verbert L, Lee B, Kocks SL, Assefa Z, Parys JB, Missiaen L, Callewaert G, Fissore RA, De Smedt H, Bultynck G 2008. Caspase-3-truncated type 1 inositol 1,4,5-trisphosphate receptor enhances intracellular Ca2+ leak and disturbs Ca2+ signalling. Biol Cell 100: 39–49 [Europe PMC free article] [Abstract] [Google Scholar]
- Vicencio JM, Ibarra C, Estrada M, Chiong M, Soto D, Parra V, Diaz-Araya G, Jaimovich E, Lavandero S 2006. Testosterone induces an intracellular calcium increase by a nongenomic mechanism in cultured rat cardiac myocytes. Endocrinology 147: 1386–1395 [Abstract] [Google Scholar]
- Vicencio JM, Ortiz C, Criollo A, Jones AW, Kepp O, Galluzzi L, Joza N, Vitale I, Morselli E, Tailler M, et al.2009. The inositol 1,4,5-trisphosphate receptor regulates autophagy through its interaction with Beclin 1. Cell Death Differ 16: 1006–1017 [Abstract] [Google Scholar]
- Wang Z, Malone MH, He H, McColl KS, Distelhorst CW 2003. Microarray analysis uncovers the induction of the proapoptotic BH3-only protein Bim in multiple models of glucocorticoid-induced apoptosis. J Biol Chem 278: 23861–23867 [Abstract] [Google Scholar]
- Wei MC, Zong WX, Cheng EH, Lindsten T, Panoutsakopoulou V, Ross AJ, Roth KA, MacGregor GR, Thompson CB, Korsmeyer SJ 2001. Proapoptotic BAX and BAK: a requisite gateway to mitochondrial dysfunction and death. Science 292: 727–730 [Europe PMC free article] [Abstract] [Google Scholar]
- White C, Li C, Yang J, Petrenko NB, Madesh M, Thompson CB, Foskett JK 2005. The endoplasmic reticulum gateway to apoptosis by Bcl-X(L) modulation of the InsP3R. Nat Cell Biol 7: 1021–1028 [Europe PMC free article] [Abstract] [Google Scholar]
- Willis SN, Fletcher JI, Kaufmann T, van Delft MF, Chen L, Czabotar PE, Ierino H, Lee EF, Fairlie WD, Bouillet P, et al.2007. Apoptosis initiated when BH3 ligands engage multiple Bcl-2 homologs, not Bax or Bak. Science 315: 856–859 [Abstract] [Google Scholar]
- Winslow MM, Crabtree GR 2005. Immunology. Decoding calcium signaling. Science 307: 56–57 [Abstract] [Google Scholar]
- Winslow MM, Neilson JR, Crabtree GR 2003. Calcium signalling in lymphocytes. Curr Opin Immunol 15: 299–307 [Abstract] [Google Scholar]
- Xia R, Stangler T, Abramson JJ 2000. Skeletal muscle ryanodine receptor is a redox sensor with a well defined redox potential that is sensitive to channel modulators. J Biol Chem 275: 36556–36561 [Abstract] [Google Scholar]
- Xu KY, Huso DL, Dawson TM, Bredt DS, Becker LC 1999. Nitric oxide synthase in cardiac sarcoplasmic reticulum. Proc Natl Acad Sci U S A 96: 657–662 [Europe PMC free article] [Abstract] [Google Scholar]
- Xu L, Kong D, Zhu L, Zhu W, Andrews DW, Kuo TH 2007. Suppression of IP3-mediated calcium release and apoptosis by Bcl-2 involves the participation of protein phosphatase 1. Mol Cell Biochem 295: 153–165 [Abstract] [Google Scholar]
- Yamada T, Horiuchi M, Dzau VJ 1996. Angiotensin II type 2 receptor mediates programmed cell death. Proc Natl Acad Sci U S A 93: 156–160 [Europe PMC free article] [Abstract] [Google Scholar]
- Yorimitsu T, Nair U, Yang Z, Klionsky DJ 2006. Endoplasmic reticulum stress triggers autophagy. J Biol Chem 281: 30299–30304 [Europe PMC free article] [Abstract] [Google Scholar]
- Zalckvar E, Berissi H, Eisenstein M, Kimchi A 2009. Phosphorylation of Beclin 1 by DAP-kinase promotes autophagy by weakening its interactions with Bcl-2 and Bcl-XL. Autophagy 5: 720–722 [Abstract] [Google Scholar]
- Zhang L, Li L, Liu H, Borowitz JL, Isom GE 2009a. BNIP3 mediates cell death by different pathways following localization to endoplasmic reticulum and mitochondrion. Faseb J 23: 3405–3414 [Europe PMC free article] [Abstract] [Google Scholar]
- Zhang S, Hisatsune C, Matsu-Ura T, Mikoshiba K 2009b. G-protein-coupled receptor kinase-interacting proteins inhibit apoptosis by inositol 1,4,5-triphosphate receptor-mediated Ca2+ signal regulation. J Biol Chem 284: 29158–29169 [Europe PMC free article] [Abstract] [Google Scholar]
- Zhong F, Davis MC, McColl KS, Distelhorst CW 2006. Bcl-2 differentially regulates Ca2+ signals according to the strength of T cell receptor activation. J Cell Biol 172: 127–137 [Europe PMC free article] [Abstract] [Google Scholar]
- Zong WX, Li C, Hatzivassiliou G, Lindsten T, Yu QC, Yuan J, Thompson CB 2003. Bax and Bak can localize to the endoplasmic reticulum to initiate apoptosis. J Cell Biol 162: 59–69 [Europe PMC free article] [Abstract] [Google Scholar]
Articles from Cold Spring Harbor Perspectives in Biology are provided here courtesy of Cold Spring Harbor Laboratory Press
Full text links
Read article at publisher's site: https://doi.org/10.1101/cshperspect.a005579
Read article for free, from open access legal sources, via Unpaywall:
http://cshperspectives.cshlp.org/content/2/10/a005579.full.pdf
Citations & impact
Impact metrics
Citations of article over time
Article citations
Adaptation of STIM1 structure-function relationships for optogenetic control of calcium signaling.
J Biol Chem, 300(9):107636, 08 Aug 2024
Cited by: 0 articles | PMID: 39122007 | PMCID: PMC11402311
Review Free full text in Europe PMC
Mycobacterium tuberculosis strain with deletions in menT3 and menT4 is attenuated and confers protection in mice and guinea pigs.
Nat Commun, 15(1):5467, 27 Jun 2024
Cited by: 1 article | PMID: 38937463
Immunomodulatory effects of a cell processing device to ameliorate dysregulated hyperinflammatory disease states.
Sci Rep, 14(1):12747, 03 Jun 2024
Cited by: 0 articles | PMID: 38830924 | PMCID: PMC11148190
Optogenetically engineered calcium oscillations promote autophagy-mediated cell death via AMPK activation.
Open Biol, 14(4):240001, 24 Apr 2024
Cited by: 1 article | PMID: 38653331 | PMCID: PMC11057470
Blood-testis barrier: a review on regulators in maintaining cell junction integrity between Sertoli cells.
Cell Tissue Res, 396(2):157-175, 02 Apr 2024
Cited by: 3 articles | PMID: 38564020
Review
Go to all (115) article citations
Data
Similar Articles
To arrive at the top five similar articles we use a word-weighted algorithm to compare words from the Title and Abstract of each citation.
Various Aspects of Calcium Signaling in the Regulation of Apoptosis, Autophagy, Cell Proliferation, and Cancer.
Int J Mol Sci, 21(21):E8323, 06 Nov 2020
Cited by: 129 articles | PMID: 33171939 | PMCID: PMC7664196
Review Free full text in Europe PMC
The calcium-signaling toolkit: Updates needed.
Biochim Biophys Acta, 1863(6 pt b):1337-1343, 30 Nov 2015
Cited by: 14 articles | PMID: 26658643
Review
Quantitative assessment of cell fate decision between autophagy and apoptosis.
Sci Rep, 7(1):17605, 14 Dec 2017
Cited by: 28 articles | PMID: 29242632 | PMCID: PMC5730598
Bcl-2 protein family members: versatile regulators of calcium signaling in cell survival and apoptosis.
Annu Rev Physiol, 70:73-91, 01 Jan 2008
Cited by: 220 articles | PMID: 17680735
Review