Abstract
Objective
Cathepsin K (CatK) is one of the most potent mammalian elastases. We have previously shown increased expression of CatK in human abdominal aortic aneurysm (AAA) lesions. Whether this protease participates directly in AAA formation, however, remains unknown.Methods and results
Mouse experimental AAA was induced with aortic perfusion of a porcine pancreatic elastase. Using this experimental model, we demonstrated that absence of CatK prevented AAA formation in mice 14 days postperfusion. CatK deficiency significantly reduced lesion CD4(+) T-cell content, total lesion and medial cell proliferation and apoptosis, medial smooth muscle cell (SMC) loss, elastinolytic CatL and CatS expression, and elastin fragmentation, but it did not affect AAA lesion Mac-3(+) macrophage accumulation or CD31(+) microvessel numbers. In vitro studies revealed that CatK contributed importantly to CD4(+) T-cell proliferation, SMC apoptosis, and other cysteinyl cathepsin and matrix metalloproteinase expression and activities in SMCs and endothelial cells but played negligible roles in microvessel growth and monocyte migration. AAA lesions from CatK-deficient mice showed reduced elastinolytic cathepsin activities compared with those from wild-type control mice.Conclusions
This study demonstrates that CatK plays an essential role in AAA formation by promoting T-cell proliferation, vascular SMC apoptosis, and elastin degradation and by affecting vascular cell protease expression and activities.Free full text

Cathepsin K Deficiency Reduces Elastase Perfusion-Induced Abdominal Aortic Aneurysms in Mice
Abstract
Objectives
Cathepsin K (CatK) is one of the most potent mammalian elastases. We have previously shown increased expression of CatK in human abdominal aortic aneurysm (AAA) lesions. Whether this protease participates directly in AAA formation, however, remains unknown.
Methods and Results
Mouse experimental AAA was induced with aortic perfusion of a porcine pancreatic elastase. Using this experimental model, we demonstrated that absence of CatK prevented AAA formation in mice 14 days post-perfusion. CatK deficiency significantly reduced lesion CD4+ T-cellcontent, total lesion and medial cell proliferation and apoptosis, medial smooth-muscle cell (SMC) loss, elastinolytic CatL and CatS expression, and elastin fragmentation, but did not affect AAA lesion Mac-3+ macrophage accumulation or CD31+ microvessel numbers. In vitro studies revealed that CatK contributed importantly to CD4+ T-cell proliferation, SMC apoptosis, and other cysteinyl cathepsin and matrix metalloproteinase expression and activities in SMC and endothelial cells, but played negligible roles in microvessel growth and monocyte migration. AAA lesions from CatK-deficient mice showed reduced elastinolytic cathepsin activities compared with those from wild-type control mice.
Conclusion
This study demonstrates that CatK plays an essential role in AAA formation by promoting T-cell proliferation, vascular SMC apoptosis, and elastin degradation, and by affecting vascular cell protease expression and activities.
INTRODUCTION
The pathogenesis of human AAA involves extensive vascular wall matrix protein remodeling, which requires the participation of proteases.1–3 Cysteine protease cathepsins K, L, and S are among the most potent mammalian elastases.4 We showed previously that human atherosclerosis and AAA lesions contained high levels of these proteases.5 In contrast, their endogenous inhibitor cystatin C was deficient in these lesions.6 Absence of these proteases protected mice from diet-induced atherosclerosis, whereas cystatin C-deficient mice had enlarged aortic diameters,7–10 suggesting essential roles of cysteine proteases in aortic wall remodeling. Since we first showed increased expression of cathepsins S, L, and K in human AAA lesions,6 other studies have found similar expression patterns. Aortic tissue extract cathepsin activities were significantly higher in AAA patients than in those with aortic occlusion diseases, but cystatin C levels were regulated inversely.11 Aortic tissues from patients with growing AAA and ruptured AAA contained significantly higher cathepsin mRNA and protein levels than did control aortas,12 suggesting the involvement of cathepsins in AAA pathogenesis, but a direct role of this class of proteases has never been proven. In this study, we used cathepsin K (CatK)-deficient mice and aorta elastase perfusion-induced experimental AAA to test whether this elastase contributes directly to AAA formation.
MATERIALS AND METHODS
Mouse AAA model and lesion characterization
Male 10-week-old CatK-deficient mice (Ctsk−/−, C57BL/6/129S background)13 and male wild-type (Ctsk+/+) littermate controls underwent aortic perfusion with 0.411 U/ml type I porcine pancreatic elastase (E1250, Sigma, St. Louis, MO) to produce experimental AAA, as previously described.14 We used 9~10 mice per experimental group and harvested the aortic tissues at 7 or 14 days post-perfusion. Mouse aortic diameters were measured using a surgical microscope (Zeiss Stemi. SV11) equipped with a micrometer eyepiece (14 mm/0.1, SG02.T0218c, Motic Instruments, Inc., Vancouver), which allowed us to read aortic diameters at any time during the surgical procedure or during tissue harvesting.14,15 The micrometer eyepiece was calibrated under each magnification. Pre-perfusion, immediate post-perfusion (5 minutes after perfusion restoration), 7-day post-perfusion, or 14-day post-perfusion aortic diameters were measured to a resolution of 0.1 mm, while mice were under anesthesia and physiological blood pressure. Two independent investigators measured aortic diameters, with no significant inter-observer or intra-observer variability. Aortic diameter expansion ≥100% of that before perfusion defined AAA.14 Each mouse aorta was isolated for frozen section preparation (a total of 20 sections per aorta were prepared for immunohistology analysis) and tissue protein extraction in a pH 5.5 buffer containing 1% Triton X-100, 40 mM sodium acetate, and 1 mM EDTA.5 Frozen sections were used for immunostaining for macrophages (Mac-3), smooth-muscle cells (SMC) (α-actin), T cells (CD4), endothelial cells (EC) (CD31), elastin (Verhoeff–van Gieson), apoptotic cells (TUNEL) and proliferating cells (Ki67), as described previously.7,15,16 Medial elastin fragmentation and SMC contents were graded as described previously, except that SMC content was presented as grades for medial SMC loss.15 T cells, apoptotic cells, proliferating cells, and microvessels in the adventitia were counted and compared blindly by two independent observers to ensure reproducibility. Adventitial macrophage-positive areas were measured with the use of computer-assisted image analysis software (Image-Pro Plus; Media Cybernetics, Bethesda, MD).
Cysteine protease active site labeling and gelatin gel zymogram
SMC and EC were lysed into a pH 5.5 buffer. Five micrograms of protein from each sample were incubated with 12 mmol/L dithiothreitol and 1 µL of biotin-conjugated JPM in 30 µL of a pH 5.5 buffer, for 1 hour at 37°C. Protein samples were then separated on a 12% SDS-PAGE, followed by immunoblot detection with horseradish peroxidase-conjugated avidin (1:10,000, Sigma).3 Matrix metalloproteinase (MMP) activity was detected with a gelatin gel zymogram, essentially the same as reported previously.17 Equal protein loading (20 µg protein/lane) was confirmed with immunoblot analysis with goat anti-mouse β-actin polyclonal antibodies (1:2000, Santa Cruz Biotechnologies, Santa Cruz, CA).
Real-time polymerase chain reaction (RT-PCR)
RT-PCR determined protease mRNA levels in SMC and EC. Mouse aortic SMC and EC were prepared from Ctsk+/+ mice and Ctsk−/− mice, as reported previously.7,18 Aortic SMC purity was examined with the expression of α-smooth muscle actin and calponin.7 Total cellular RNA was extracted from SMC and EC using TRIzol reagent (Invitrogen, Carlsbad, CA) and treated with RNase-free DNase (Ambion, Austin, TX) to remove genomic DNA contaminants. Equal amounts of RNA were reverse transcribed, and quantitative PCR was assessed in a single-color RT-RCR detection system (Stratagene, La Jolla, CA). The level of each protease transcript was normalized to that of the β-actin transcript.
SMC apoptosis
SMC apoptosis was performed using primary cultured Ctsk+/+ and Ctsk−/− mouse aortic SMC on an 8-well chamber slide. Confluent SMC were stimulated to apoptosis overnight with 60 µM pyrrolidine dithiocarbamate (PDTC).19 Apoptotic cells were detected with In Situ Cell Death Detection Kit according to the manufacturer’s instructions (Roche Diagnostics Co., Indianapolis, IN).
Aortic ring assay
An aortic ring assay was used to test the role of CatK in angiogenesis. In brief, a 96-well plate was coated with 50 µl of Matrigel (BD Biosciences, San Diego, CA). Mouse aortic rings of 1 mm in length from Ctsk+/+ and Ctsk−/− mice were laid on top of the Matrigel and covered with 100 µl of Matrigel. After solidification, 150 µl of RPMI (10% FBS) was added to each well. After 7–10 days of culture, the aortas were photographed, and the endothelial outgrowth was analyzed using Image-Pro Plus software and presented as square millimeters. Basic fibroblast growth factor (bFGF) (10 ng/mL, PeproTech, Inc., Rocky Hill, NJ) was used as a positive control.
In situ elastin zymography
AAA lesion elastinolytic activity was determined in 8-µm frozen sections using elastin conjugated with quenched fluorescein (DQ elastin; Invitrogen) as a substrate, which requires cleavage by elastinolytic enzymes to become fluorescent. In brief, DQ elastin (1 mg/ml in H2O) was mixed 1:10 with 1% low-melting agarose (Sigma). This mixture (20 µl) was added on top of each section, coverslipped, and gelled at 4°C. Following incubation at 37°C (48 hours), fluorescence was examined under fluorescent microscopy. Cysteine protease activity was determined using an EDTA-containing pH 5.5 buffer5 with or without a generic cathepsin inhibitor E64d (20 µM). Zymographic images were acquired using identical shutter conditions. The percentage of fluorescence intensity of each cross section, excluding the media area due to medial elastin filament autofluorescence, was measured using computer-assisted image quantification (Image-Pro Plus software). Medial cathepsin elastase activity was calculated by subtracting the percentages of fluorescence intensity in media regions of E64d-treated sections from those of untreated sections.
Cell proliferation assay
CD4+ T cells were purified from splenocytes by depleting MHC-class II–positive cells and CD8+ T cells using anti-mouse I-Ab and CD8 monoclonal antibodies (BD Biosciences), followed by complement depletion, as described previously.20 Monocytes were isolated from peripheral blood by Percoll (Sigma) gradient centrifugation. T-cell and monocyte proliferation were assessed with the Cell Titer 96AQ Assay kit, according to the manufacturer’s instructions (Promega, Madison, WI). Equal numbers of T cells and monocytes from Ctsk+/+ mice and Ctsk−/− mice were plated on 96-well plates at serial dilutions in 100 µL of 10% FBS RPMI 1640 per well, and cultured for 2 days at 37°C. Then, 20 µL of a mixture of tetrazolium compound and phenazine methosulfate was added, and the absorbance was determined at 492 nm. Monocyte proliferation was determined under spontaneous condition, whereas CD4+ T-cell proliferation was measured in the presence of anti-CD3 monoclonal antibodies (1 µg/ml, Pharmingen, San Diego, CA). Serial dilutions of known numbers of T cells and monocytes were used to generate standard curves.
Transmigration assay
T-cell and monocyte transmigration assay was performed on a type-I collagen (100 ng/25 µl/well in a pH7.0 HEPES buffer) pre-coated 96-well Chemotaxis plate, according to the manufacturer’s instructions (Neuro Probe, Inc., Gaithersburg, MD). SDF1α (0, 10, 100, 1000 ng/ml, PeproTech) in a plain RPMI containing 1% bovine serum albumin (BSA, Sigma) was used as chemokine. Although SDF1α is an important chemokine for stem cells or progenitor cells, it has been widely used in inflammatory cell in vitro migration assays.21–23 Briefly, T cells and monocytes (25,000 cells in 25 µl 1% BSA RPMI) were seeded on the top of the filter, and 30 µl of SDF1α was added to the bottom chamber. After incubation (3 hours for monocytes; 1.5 hours for T cells), the remaining filter top cells were removed and the bottom cells were collected (1500 rpm, 5 minutes), fixed with 1% paraformaldehyde, and counted by FACS analysis.
Blood cell and splenocyte FACS analysis
Blood cells and splenocytes from Ctsk+/+ mice and Ctsk−/− mice, and those treated with angiotensin-II (Ang-II), were analyzed for contents of Ly6G+, CD11b+, CD4+, and CD8+ cells. To treat Ctsk+/+ and Ctsk−/− mice with Ang-II, mice received Alzet osmotic minipumps (Model 2004, Alzet, Durect Corporation, Cupertino, CA) filled with Ang-II (Sigma, 1000 ng/kg/min). Blood samples were collected after 28 days. Total blood cells were isolated by cardiac puncture, and splenocytes were prepared as described previously.20 Blood cells and splenocytes were stained with rat anti-mouse Ly6G-FITC (RB6-8C5, eBioscience, CA), rat anti-mouse CD11b-PE (M1/70, eBioscience, San Diego, CA), rat anti-mouse CD4-PE (GK1.5, Biolegend, San Diego, CA), and rat anti-mouse CD8-Alexa 610 (5H10, Invitrogen). Ly6G+, CD11b+, CD4+, and CD8+ cell percentages were determined by FACS analysis (Cytomics FC500, Bechman Coulter, Brea, CA).
Statistical analysis
Due to relatively small sample sizes and data distribution abnormality, we selected the non-parametric Mann-Whitney U test for non-paired data sets and the Wilcoxon signed-rank test for paired data sets to examine statistical significance throughout this study. P<0.05 was considered statistically significant.
RESULTS
Reduced AAA in cathepsin K-deficient mice
Both Ctsk−/− mice and Ctsk+/+ mice underwent aortic elastase perfusion to induce AAA. In mice used for the 7-day time point, there were no significant differences in aortic diameters between Ctsk+/+ mice and Ctsk−/− mice before and immediately after elastase perfusion. At 7 days post-perfusion, Ctsk+/+ mice and Ctsk−/− mice also showed no significant differences in aortic diameters, and none of these mice developed AAA, as defined by a 100% increase in aortic diameter (Figure 1A).14 In Ctsk−/− mice used for the 14-day time point, both pre-perfusion (0.55±0.01 mm vs. 0.48±0.01 mm, P<0.001) and immediate post-perfusion (0.87±0.03 mm vs. 0.79±0.02 mm, P=0.02) aortic diameters were larger than those from Ctsk+/+ mice, although we cannot explain this observation. At 14 days post-perfusion, however, aortic diameters from Ctsk−/− mice were not significantly different from those immediately post-perfusion (P=0.562), but were significantly smaller that those from Ctsk+/+ mice (P=0.001) (Figure 1B). At this time point, all Ctsk+/+ mice (10 out of 10) developed AAA, but none of the Ctsk−/− mice did.
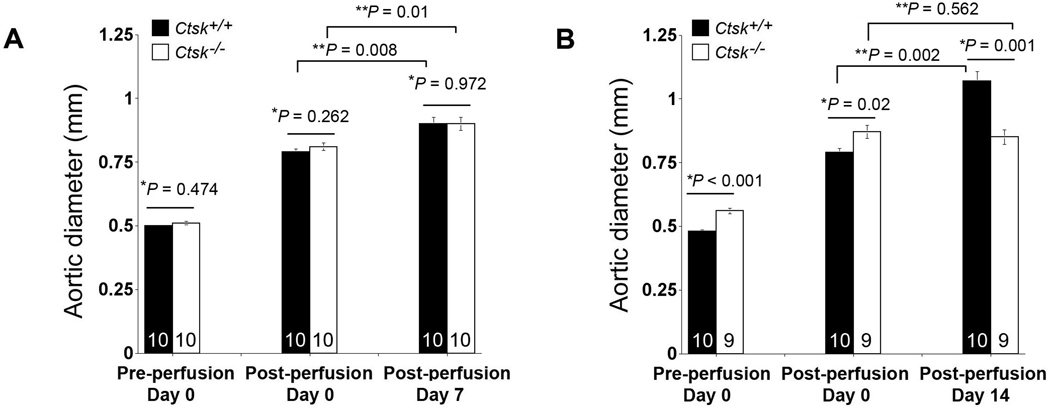
Reduced AAA formation in Ctsk−/− mice. Aortic diameters in both Ctsk+/+ mice and Ctsk−/− mice were measured pre-perfusion, immediately post-perfusion, and at 7 days and 14 days post-perfusion. The number of mice per group is indicated in each bar. Data are mean ± SE. P<0.05 is considered statistically significant; *non-paired Mann-Whitney U test, **paired Wilcoxon signed-rank test.
Inflammatory cells in AAA lesions
Inflammatory cell infiltration is an important event following AAA initiation in humans and animals.24 Although we detected no significant differences in lesion macrophage contents between Ctsk+/+ and Ctsk−/− mice at either time point (Figure 2A), Ctsk+/+ mouse AAA lesions contained significantly higher numbers of CD4+ T cells than did Ctsk−/− AAA lesions at 14 days post-perfusion (Figure 2B). The reduced numbers of CD4+ T cells in Ctsk−/− mouse lesions suggest that CatK activity contributed to T-cell migration or proliferation. To test these possibilities, we performed Boyden chamber cell transmigration assay using SDF1α as a chemoattractant. When either monocytes or CD4+ T cells were used, we did not detect significant differences in their transmigration through collagen-coated transwells with or without CatK (data not shown). In contrast, CD4+ T cells (Figure 2C), but not monocytes (data not shown), proliferated differently between the two genotypes. CD4+ T cells from Ctsk+/+ mice proliferated much faster than those from Ctsk−/− mice. These data may explain the unchanged macrophage content but reduced CD4+ T-cell content in Ctsk−/− mouse AAA lesions (Figure 2A/B). In AAA lesions, Ki67+ proliferating cells were significantly reduced in Ctsk−/− mice, compared with those in Ctsk+/+ mice at 14 days post-perfusion (Figure 2D). Anti-CD4 and anti-Ki67 monoclonal antibody co-immunostaining examined proliferating CD4+ T cells in AAA lesions. Among all Ki67+ proliferating cells, the percentages of CD4+ T cells were significantly lower in Ctsk−/− mice than in Ctsk+/+ mice (Figure 2E), suggesting a role for CatL in T-cell proliferation in vivo.

CatK activities on T-cell proliferation. Absence of CatK did not affect AAA lesion Mac-3+ macrophage-positive area (A), but reduced CD4+ T-cell numbers (B) in the adventitia at 14 days post-perfusion. In vitro cell proliferation assay showed that CatK deficiency impaired CD4+ T-cell proliferation (C). In AAA lesion adventitia, numbers of Ki67-positive cells (D) and percentage of CD4+ T-cells among Ki67-positive cells (E, arrows indicate Ki67+CD4+ T cells) were also reduced in Ctsk−/− mice at 14 days post-perfusion. The number of mice per group is indicated in each bar. All data are mean ± SE. P<0.05 is considered statistically significant; Mann-Whitney U test. Representative images are shown to the right in panels B, D, and E.
CatK function in vascular cell apoptosis
SMC apoptosis determines tunica media thinning and characterizes both human and animal AAA lesions,25–27 whereas infiltrating leukocytes release apoptotic stimuli to promote vascular cell apoptosis.26,28 The absence of CatK reduced AAA lesion apoptotic cells significantly. TUNEL-positive cells were reduced in whole AAA lesions (Figure 3A) and in the media (Figure 3B, mainly SMC) from Ctsk−/− mice, compared with those in Ctsk+/+ mice at 14 days post-perfusion. In correlation with this observation, medial SMC loss in AAA lesions from Ctsk−/− mice also was impaired significantly at this time point (Figure 3C), although both lesion cell apoptosis and medial SMC loss were not significantly different between the phenotypes at the 7-day time point (Figure 3A/C). To examine the contribution of CatK to SMC apoptosis, we induced SMC apoptosis with PDTC. When SMC from Ctsk+/+ mice underwent apoptosis in the presence of PDTC, those from Ctsk−/− mice were protected (Figure 3D). Although the mechanisms behind these observations merit further investigation, these results suggest an important role of CatK in SMC apoptosis.

CatK activities on lesion cell apoptosis. AAA lesion adventitia (A) and medial (B) TUNEL-positive cell numbers were significantly lower in Ctsk−/− mice than in Ctsk+/+ mice at 14 days post-perfusion. At the same time point, lesion medial SMC loss was reduced in Ctsk−/− mice (C). The number of mice per group is indicated in each bar. Aortic SMC from Ctsk−/− mice were resistant to PDTC-induced apoptosis (D). All data are mean ± SE. P<0.05 is considered statistically significant; Mann-Whitney U test. Representative images are shown to the right in panels A, B, and C.
CatK deficiency protected medial elastin fragmentation
Elastin fragmentation is an important hallmark of human AAA29 and is mediated by elastases in the media. As one of the most potent elastases, CatK may participate directly in medial elastin degradation. Verhoeff–van Gieson staining demonstrated significantly reduced elastin fragmentation in AAA lesions from Ctsk−/− mice compared with those from Ctsk+/+ mice at 7 days post-perfusion. Elastin degradation was enhanced at the later time point and remained lower in Ctsk−/− mice than in Ctsk+/+ mice at 14 days post-perfusion, although it did not reach statistical significance (2.39±0.26 vs. 2.79±0.22, P=0.171) (Figure 4A). Insignificance in elastin degradation between the groups at this time point was due to an outlier aorta in each group of mice. Significant differences in AAA expansion (Figure 1B) but insignificant medial elastin fragmentation (Figure 4A) between Ctsk−/− mice and Ctsk+/+ mice at 14 days post-perfusion suggest that medial elastin degradation is merely part of the mechanism of murine AAA formation. Other mechanisms, such as inflammatory cell infiltration, medial cell apoptosis, and SMC loss also contribute to the pathogenesis.
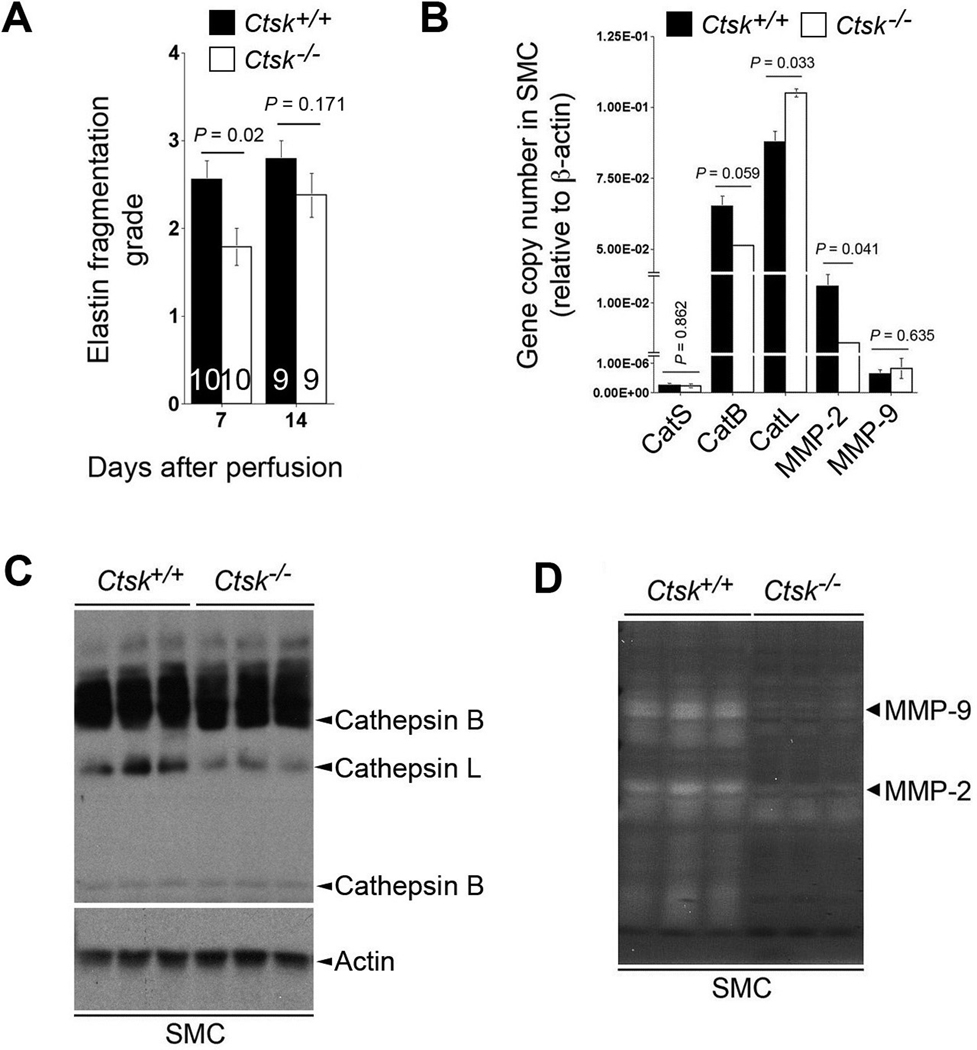
CatK activities on medial elastin fragmentation and aortic SMC protease expression. At both time points (7 days and 14 days post-perfusion), medial elastin fragmentation in AAA lesions from Ctsk−/− mice were lower than in Ctsk+/+ mice (A). The number of mice per group is indicated in each bar. Data are mean ± SE. Cathepsin and MMP transcripts in aortic SMC were examined by RT-PCR (B). Data are mean ± SE of four independent experiments. P<0.05 is considered statistically significant; Mann-Whitney U test. Cysteinyl cathepsin activities in SMC were examined by active site JPM labeling (C). Actin immunoblot was used as protein loading control. MMP activities were assessed by gelatin gel zymogram assay (D).
CatK deficiency can alter expression or activities of other elastases — and consequently, impair elastin fragmentation — in AAA lesions from Ctsk−/− mice. RT-PCR showed increased CatL transcript and decreased MMP-2 transcripts in SMC from Ctsk−/− mice, as compared with Ctsk+/+ mice (Figure 4B). Although SMC routinely express MMP-2, they also express MMP-9 at much lower levels,30,31 and CatK deficiency did not affect MMP-9 mRNA levels significantly (Figure 4B). Cysteinyl cathepsin active site labeling with JPM in SMC from Ctsk−/− mice, however, revealed reduced activity of CatL (Figure 4C), another potent elastase that is abundant in human AAA.32 In a separate study, we proved that the absence of CatL prevented mice from elastase perfusion-induced AAA, with concomitant reduction of media elastin fragmentation (Shi, unpublished data). Furthermore, MMP-2 and MMP-9 activities were reduced in SMC from Ctsk−/− mice, as demonstrated in a gelatin gel zymogram assay (Figure 4D). MMP-2 and MMP-9 may play essential roles in murine experimental AAA formation.14,33 Reduced MMP activities in Ctsk−/− mouse SMC may indirectly contribute to the protection of elastase perfusion-induced AAA.
Impaired elastinolytic cathepsin activities in AAA lesions from Ctsk−/− mice was further illustrated in an in situ elastase zymogram assay to detect lesion elastinolytic cathepsin activity3,15,16 and immunostaining to detect two common elastinolytic CatL and CatS in the media. When 8-µm unfixed cross sections from AAA lesions were used to digest flurogenic DQ-elastin,5 AAA lesions from Ctsk+/+ mice contained elastinolytic cathepsin activities in the adventitia regions that could be inhibited by 20 µM of a non-selective cathepsin inhibitor E64d (Figure 5A, top panels). Reduced elastinolytic cathepsins in adventitia from Ctsk−/− AAA lesions may result from impaired lesion inflammatory cell infiltration and proliferation (Figure 2B–2E). We focused on elastinolytic cathepsin activities in the adventitia, separated from media by dotted lines in Figure 5A, mainly because of the interference of internal elastica autofluorescence. In contrast, AAA sections from Ctsk−/− mice contained much lower adventitia elastinolytic cathepsin activities that were further inhibited by E64d (Figure 5A, bottom panels). To examine elastinolytic cathepsin activities in the media, we subtracted the percentages of media fluorescence of E64d-treated sections from those of untreated sections. Using this method, we found that the percentages of medial cathepsin activity from Ctsk+/+ mice were significantly higher than those from Ctsk−/− mice (P<0.0001, Figure 5B). To confirm further altered medial cathepsin expression and activity differences between the groups, we performed immunostaining for CatL and CatS, two potent mammalian elastases. Medial expressions of CatL and CatS were reduced in AAA lesions from Ctsk−/− mice compared with those from Ctsk+/+ mice (Figure 5C), which is consistent with reduced medial elastase activity (Figure 5B) and elastinolysis in these AAA lesions (Figure 4A).

Elastinolytic cysteinyl cathepsins in AAA lesions at 14 days post-perfusion. A. In situ cysteinyl elastastinolytic activity fluorescent zymogram assay. High cathepsin activities in the adventitia of AAA lesions in Ctsk+/+ mice (top left) can be largely inhibited by E64d (top right). Elastinolytic cathepsin activities were reduced in AAA lesion adventitia from Ctsk−/− mice (bottom left), and E64d further reduced cathepsin activities (bottom right). The percentages of adventitia fluorescence yielded from fluorogenic elastin degradation represented cysteinyl cathepsin activities. The white dotted lines separate medial elastin autofluorescence from the adventitia. B. Medial fluorescence subtractions between E64d-treated and untreated sections determined medial elastinolytic cathepsin activities, which were significantly reduced in the absence of CatK. The number of mice per group is indicated in each bar. C. Immunostaining detected medial CatL and CatS expression in AAA lesions from Ctsk+/+ and Ctsk−/− mice. Med.: media; Adv.: adventitia.
CatK expression and activities in EC and angiogenesis
Increased angiogenesis associates with AAA growth.34 Neovascularization provides paths for inflammatory cell accumulation in human AAA lesions.35 Reduced AAA growth in Ctsk−/− mice might be caused by impaired angiogenesis. To test this hypothesis, we immunostained aortic sections with anti-mouse CD31 antibodies, and found lower CD31+ microvessel numbers in lesions from Ctsk−/− mice than in those from Ctsk+/+ mice at 14 days post-perfusion, but the difference did not reach statistical significance (Figure 6A). These observations suggest that CatK activities in angiogenesis contribute partially to pathogenesis in this experimental AAA. In vitro aortic ring assays yielded similar results. When aortic rings from Ctsk+/+ mice and Ctsk−/− mice were used, we did not see significant differences in microvessel growth area in the absence (0.44±0.39 vs. 0.35±0.32 mm2, P>0.05) or presence (5.34±1.86 vs. 5.34±2.59 mm2, P>0.05) of pro-angiogenic factor bFGF. A mild effect of CatK in angiogenesis is consistent with nearly “unchanged” EC protease activities. Although RT-PCR revealed decreased CatB transcript and increased MMP-2 transcript in EC from Ctsk−/− mice, cathepsin active site labeling with JPM demonstrated that CatK deficiency impaired EC CatS activity, with compensatory increase of CatL activity (Figure 6C). Gelatin gel zymogram assay showed that CatK deficiency did not affect EC MMP-9 or MMP-2 activities (Figure 6D).
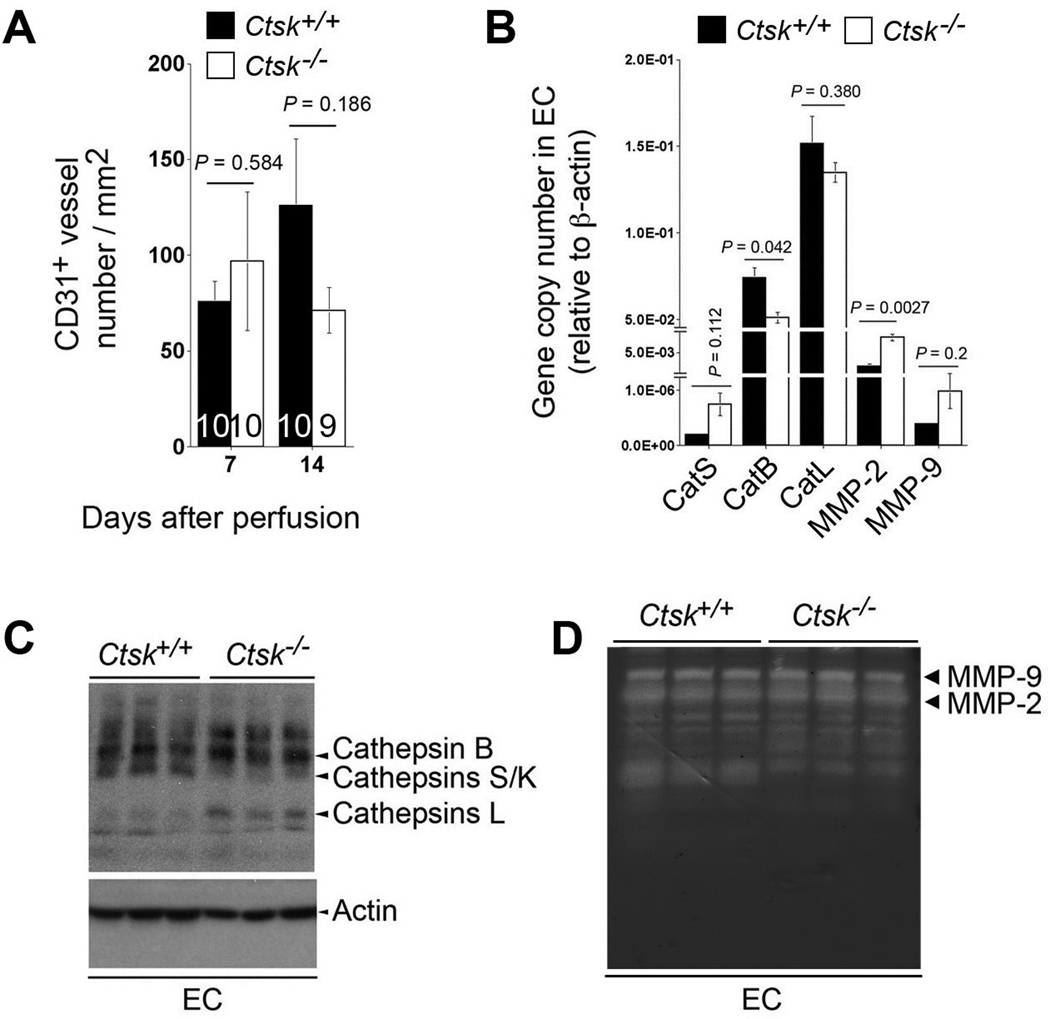
CatK activities on angiogenesis and EC protease expression. CD31+ microvessel numbers were not significantly different in AAA lesions between the genotypes at both time points (A). EC cathepsin and MMP transcription levels were assessed by RT-PCR (B). Cysteine protease active site labeling with JPM (C) and gelatin gel zymogram (D) were used to assess EC cathepsin and MMP activities, respectively.
DISCUSSION
Cathepsins have been implicated in human AAA formation despite a lack of direct proof. This study provides the first evidence that cathepsins participate directly in AAA pathogenesis. Although many more mechanisms remain unknown, we demonstrated that CatK contributed to AAA formation by regulating lesion T-cell proliferation, SMC apoptosis, and medial vascular cell protease expression or activities. CatK deficiency protected mice from elastase perfusion-induced experimental AAA.
An earlier study from Bai and colleagues, using apolipoprotein E and CatK double-deficient (Apoe−/−Ctsk−/−) mice, showed that the absence of CatK did not affect AAA progression in Ang-II–induced experimental AAA.36 These prior observations did not support a role for CatK in mouse AAA pathogenesis. Compensatory induction of other cathepsins in Apoe−/−Ctsk−/− mice, although not tested in the study by Bai et al., may explain their findings; different experimental models also may contribute to the insignificant effect of CatK in Ang-II–induced AAA between Apoe−/− mice and Apoe−/−Ctsk−/− mice. Bai et al. discovered unexpectedly that Ang-II infusion enhanced peripheral active CD4+CD25+ T cells and Ly6G+ leukocytes in Apoe−/−Ctsk−/− mice. Such Ang-II–induced peripheral inflammatory cell population changes may contribute in part to increased AAA lesion CD45+ leukocytes and Mac-3+ macrophages, thereby increasing expression of CatS and CatC in AAA lesions from Apoe−/−Ctsk−/− mice.36 The absence of a mutant Apoe allele and Ang-II treatment in this study may eliminate these confounding factors, and therefore reflect the true function of CatK in AAA pathogenesis.
To assess whether Ang-II treatment alone may alter the peripheral inflammatory cell population, we treated both Ctsk+/+ and Ctsk−/− mice with Ang-II for 28 days, and then analyzed peripheral blood and spleen leukocyte populations. Blood total Ly6G+ neutrophils and Ly6G+CD11b+ activated neutrophils37 were not different between untreated Ctsk+/+ mice and Ctsk−/− mice. Although Ang-II infusion did not yield appreciable AAA in either Ctsk+/+ mice or Ctsk−/− mice, it significantly increased blood total neutrophils and activated neutrophils in Ctsk+/+ mice, while showing no effects on Ctsk−/− mice. Blood CD11b+ cells (e.g., monocytes, macrophages, and NK T cells) were significantly fewer in Ctsk−/− mice than in Ctsk+/+ mice, and Ang-II treatment did not affect these populations in blood (Supplementary Figure IA). Blood CD4+ and CD8+ T-cell populations were also affected by Ang-II. Before Ang-II infusion, Ctsk+/+ mouse blood contained significantly fewer CD4+ cells and CD4+CD25+ activated CD4+ cells, but more CD8+ cells and CD4+CD8+ cells. After 28 days of Ang-II infusion, all of these differences were impaired at different levels (Supplementary Figure IB/IC). In spleen, Ly6G+ cells, CD11b+ cells, and Ly6G+CD11b+ cells were similar between Ctsk−/− mice and Ctsk+/+ mice. Ang-II infusion increased Ly6G+ and Ly6G+CD11b+ cells and reduced CD11b+ cells similarly in both Ctsk−/− mice and Ctsk+/+ mice (Supplementary Figure IIA). Furthermore, more CD4+ cells and fewer CD8+ cells in Ctsk+/+ spleen were reversed after Ang-II treatment (Supplementary Figure IIB), which also reduced spleen activated CD4+CD25+ cells in both Ctsk−/− mice and Ctsk+/+ mice (Supplementary Figure IIC). Altered peripheral inflammatory cell profiles in Apoe−/− mice after Ang-II infusion therefore may have confounded AAA progression, thus obscuring the contribution of CatK.36
Although we did not examine them in this study, cysteinyl cathepsins induce cell apoptosis by cleaving the anti-apoptotic protein Bcl-2 member Bid and creating pro-apoptotic signal for mitochondrial cytochrome C release.38 For example, CatB-deficient mice showed reduced cerebral cell losses, whereas a mutation on cystatin resulted in increased apoptosis of cerebellar granule cells.39 Osteoclasts from Ctsk−/− mice showed increased growth due to reduced apoptosis and senescence.40 Reduced apoptosis in AAA lesions from Ctsk−/− mice may utilize the same mechanism. Cysteinyl cathepsins also regulate lymphocyte proliferation with complicated mechanisms. Prior studies have suggested that cathepsins promote T-cell proliferation by degrading matrix proteins in the lymphoid organs,41 activating transcription factor NF-κB,42 and regulating β2 integrin receptor lymphocyte function-associated antigen-1 activity.43,44 Consistent with these prior studies, our study showed impaired proliferation of CD4+ T cells isolated from Ctsk−/− splenocytes compared with those from Ctsk+/+ splenocytes, which helped to explain reduced CD4+ T cells in aortic tissues from Ctsk−/− mice (Figure 2B/2C). Total CD4+ cells in spleen, however, were fewer in Ctsk+/+ mice than in Ctsk−/− mice. Ang-II infusion reversed splenic CD4+ cell profiles. Similar mechanisms may occur in the aortic wall during the progression of AAA. All such speculations merit further investigation.
Acknowledgements
The authors thank Mrs. Eugenia Shvartz for technical assistance, and Ms. Sara Karwacki for editorial assistance.
Sources of Funding
This study is supported by National Institutes of Health grants HL60942, HL81090, HL88547 (GPS) and HL56985 (PL); and by EIA award 0840118N from the American Heart Association (GPS).
Footnotes
Publisher's Disclaimer: This is a PDF file of an unedited manuscript that has been accepted for publication. As a service to our customers we are providing this early version of the manuscript. The manuscript will undergo copyediting, typesetting, and review of the resulting proof before it is published in its final citable form. Please note that during the production process errors may be discovered which could affect the content, and all legal disclaimers that apply to the journal pertain.
Disclosures
None
REFERENCES
Full text links
Read article at publisher's site: https://doi.org/10.1161/atvbaha.111.235002
Read article for free, from open access legal sources, via Unpaywall:
https://www.ahajournals.org/doi/pdf/10.1161/ATVBAHA.111.235002
Citations & impact
Impact metrics
Citations of article over time
Article citations
CD8 + T-cell deficiency protects mice from abdominal aortic aneurysm formation in response to calcium chloride 2.
J Hypertens, 42(11):1966-1975, 13 Aug 2024
Cited by: 0 articles | PMID: 39146540 | PMCID: PMC11451972
Novel pharmacological approaches in abdominal aortic aneurysm.
Clin Sci (Lond), 137(15):1167-1194, 01 Aug 2023
Cited by: 4 articles | PMID: 37559446 | PMCID: PMC10415166
Review Free full text in Europe PMC
Overview of multifunctional cysteinyl cathepsins in atherosclerosis-based cardiovascular disease: from insights into molecular functions to clinical implications.
Cell Biosci, 13(1):91, 19 May 2023
Cited by: 7 articles | PMID: 37202785 | PMCID: PMC10197855
Review Free full text in Europe PMC
Cysteine Cathepsins as Therapeutic Targets in Immune Regulation and Immune Disorders.
Biomedicines, 11(2):476, 07 Feb 2023
Cited by: 8 articles | PMID: 36831012 | PMCID: PMC9953096
Review Free full text in Europe PMC
Role of Extracellular Matrix and Inflammation in Abdominal Aortic Aneurysm.
Int J Mol Sci, 23(19):11078, 21 Sep 2022
Cited by: 18 articles | PMID: 36232377 | PMCID: PMC9569530
Review Free full text in Europe PMC
Go to all (66) article citations
Data
Data behind the article
This data has been text mined from the article, or deposited into data resources.
BioStudies: supplemental material and supporting data
Similar Articles
To arrive at the top five similar articles we use a word-weighted algorithm to compare words from the Title and Abstract of each citation.
Cathepsin L activity is essential to elastase perfusion-induced abdominal aortic aneurysms in mice.
Arterioscler Thromb Vasc Biol, 31(11):2500-2508, 01 Nov 2011
Cited by: 52 articles | PMID: 21868704 | PMCID: PMC3312037
Deficiency of cathepsin S attenuates angiotensin II-induced abdominal aortic aneurysm formation in apolipoprotein E-deficient mice.
Cardiovasc Res, 96(3):401-410, 07 Aug 2012
Cited by: 65 articles | PMID: 22871592 | PMCID: PMC3500043
Cathepsin K gene disruption does not affect murine aneurysm formation.
Atherosclerosis, 209(1):96-103, 06 Sep 2009
Cited by: 18 articles | PMID: 19775691
Three-dimensional microstructural changes in murine abdominal aortic aneurysms quantified using immunofluorescent array tomography.
J Histochem Cytochem, 60(2):97-109, 01 Dec 2011
Cited by: 9 articles | PMID: 22140132 | PMCID: PMC3351119
Review Free full text in Europe PMC
Funding
Funders who supported this work.
NHLBI NIH HHS (11)
Grant ID: HL60942
Grant ID: HL81090
Grant ID: HL88547
Grant ID: P50 HL056985
Grant ID: R01 HL060942-13
Grant ID: HL56985
Grant ID: R01 HL081090-04
Grant ID: R01 HL088547
Grant ID: R01 HL088547-04
Grant ID: R01 HL060942
Grant ID: R01 HL081090