Abstract
Purpose
The sclera forms the fibrous outer coat of the eyeball and acts as a supportive framework. The purpose of this study was to examine whether the sclera contains mesenchymal stem/progenitor cells.Method
Scleral tissue from C57BL6/J mice was separated from the retina and choroid and subsequently enzyme digested to release single cells. Proliferation capacity, self-renewal capacity, and ability for multipotent differentiation were analyzed by BrdU labeling, flow cytometry, reverse transcriptase-polymerase chain reaction, immunocytochemistry, and in vivo transplantation.Results
The scleral stem/progenitor cells (SSPCs) possessed clonogenic and high doubling capacities. These cells were positive for the mesenchymal markers Sca-1, CD90.2, CD44, CD105, and CD73 and negative for the hematopoietic markers CD45, CD11b, Flk1, CD34, and CD117. In addition to expressing stem cell genes ABCG2, Six2, Notch1, and Pax6, SSPCs were able to differentiate to adipogenic, chondrogenic, and neurogenic lineages.Conclusions
This study indicates that the sclera contains multipotent mesenchymal stem cells. Further study of SSPCs may help elucidate the cellular and molecular mechanism of scleral diseases such as scleritis and myopia.Free full text

Identification of Multipotent Stem/Progenitor Cells in Murine Sclera
Abstract
Purpose.
The sclera forms the fibrous outer coat of the eyeball and acts as a supportive framework. The purpose of this study was to examine whether the sclera contains mesenchymal stem/progenitor cells.
Method.
Scleral tissue from C57BL6/J mice was separated from the retina and choroid and subsequently enzyme digested to release single cells. Proliferation capacity, self-renewal capacity, and ability for multipotent differentiation were analyzed by BrdU labeling, flow cytometry, reverse transcriptase–polymerase chain reaction, immunocytochemistry, and in vivo transplantation.
Results.
The scleral stem/progenitor cells (SSPCs) possessed clonogenic and high doubling capacities. These cells were positive for the mesenchymal markers Sca-1, CD90.2, CD44, CD105, and CD73 and negative for the hematopoietic markers CD45, CD11b, Flk1, CD34, and CD117. In addition to expressing stem cell genes ABCG2, Six2, Notch1, and Pax6, SSPCs were able to differentiate to adipogenic, chondrogenic, and neurogenic lineages.
Conclusions.
This study indicates that the sclera contains multipotent mesenchymal stem cells. Further study of SSPCs may help elucidate the cellular and molecular mechanism of scleral diseases such as scleritis and myopia.
The sclera is a dense fibrous and viscoelastic connective tissue. It forms the outer coat frame of the eye and acts to retain the shape of the eyeball by withstanding the pressure from both outside and inside. In eutherian mammals, by far the main component of the sclera (over 90%) is fibrous type I collagen.1 Recent research has shown that the sclera is a dynamic tissue, capable of responding rapidly to changes in the visual environment to alter ocular size and refraction.2,3 The sclera's biochemical and biomechanical properties determine the shape and size of the eyeball and therefore play an important role in determination of refraction status.4 Scleral extracellular matrix remodeling is responsible for changes in ocular elongation resulting in myopic disease.5,6 Diseases affecting the sclera, such as refractory scleritis and scleromalacia, are all difficult to cure and often lead to blindness.7–9 It is thought that sclera cells may play an important role in scleral remodeling or sclera inflammation. Although tissue-specific postnatal stem/progenitor cells have been isolated from a variety of organs and tissues, it is still unknown whether the sclera contains these subsets of cells. The purpose of this study was to identify and culture multipotent scleral stem/progenitor cells (SSPCs) from the murine sclera.
Materials and Methods
Mice
Male wild-type C57BL/6 mice (Jackson Laboratories, Bar Harbor, ME) were used in the study. All procedures were performed in accordance with an IACUC-approved protocol as well as the ARVO Statement for the Use of Animals in Ophthalmic and Vision Research.
Cell Isolation and Culture
Six- to 8-week-old mouse scleras (10 scleras/batch) were obtained from eyes postmortem. The sclera was carefully dissected away from the corneal limbus and optic disc under a dissecting microscope. The scleral tissue was cut into small pieces and digested with 1.5 mg/mL collagenase type I (Worthington, Lakewood, NJ) and Dispase (Roche, Indianapolis, IN) in PBS 2 mg/mL for 1 hour at 37°C to release the individual cells. The cells were cultured in α-MEM (Invitrogen-Gibco, Grand Island, NY), supplemented with 20% lot-selected FBS (Equitech-Bio, Kerrville, TX), glutamine, penicillin/streptomycin, and 100 mM 2-mercaptoethanol (Gibco) for 8 to 10 days at 37°C in 5% CO2. Mouse bone marrow mesenchymal stem cells (BMMSCs) were also cultured.10
Proliferation Capacity of SSPCs
SSPCs (5000/well) were plated into a two-well chamber slide (Nalge Nunc International, Naperville, IL) and cultured (5% CO2, 37°C) overnight. The cells were labeled with bromodeoxyuridine (BrdU) labeling reagent (Zymed Laboratories, South San Francisco, CA) for 24 hours and fixed in 4% PFA for 10 minutes at room temperature. The BrdU-labeled cells were visualized using a BrdU staining kit (Zymed Laboratories Inc.) according to the procedures recommended by the manufacturer.11
Population-Doubling Assay
Population doubling (PD) for each passage was determined by using the formula PD ≈ log2[Nc/No], where No is the original cell population and Nc is the number of cells at confluence, and by adding the PD from each passage together to obtain the PD values. The PD assay for mouse SSPCs began with passage 1.12,13
Adipogenic Differentiation
For adipogenic induction in vitro, the cells were cultured in αMEM as previously described until confluent, at which time they were treated with adipogenic medium for 1 week.14,15 Some cultures were stained with 0.3% oil-red-O (Sigma-Aldrich, St. Louis, MO) to detect lipid droplets. Total RNA was also extracted and analyzed for adipocyte-specific markers by RT-PCR.
Chondrogenic Differentiation
For chondrogenic induction in vitro, the second-passage SSPCs or BMMSCs (1 × 106 cells) were spun down, and the pelleted cells were incubated at 37°C with 5% CO2 in medium consisting of DMEM with ITS/premix (Collaborative Biomedical Products, Bedford MA), containing insulin (6.25 μg/mL), transferrin (6.25 μg/mL), selenous acid (6.25 g/mL), and linoleic acid (5.35 mg/mL), with bovine serum albumin (1.25 mg/mL), pyruvate (1 mM), dexamethasone (10−7M), TGF-β1 (10 ng/mL, recombinant human, R&D Systems, Minneapolis, MN), and ascorbate 2-phosphate (37.5 mg/mL).16 Cell aggregates were harvested at time points up to 28 days. Chondrogenic differentiation was assessed by comparing SSPCs with BMMSCs for staining by Alcian blue or immunohistochemical staining for type II collagen, SOX9, and aggrecan.
Neurogenic Differentiation
For neurogenesis induction in vitro, neuronal cell culture medium (Neurobasal A; Invitrogen-Gibco) conditioned with B27 supplement (Invitrogen-Gibco), 1% penicillin, 20 ng/mL epidermal growth factor (BD Biosciences, Franklin Lakes, NJ), and 40 ng/mL fibroblast growth factor (FGF; BD Biosciences) was used to culture SSPCs on laminin-coated dishes.17,18 After 4 weeks, total RNA was extracted and analyzed for beta3-tubulin, nestin, and NFM (gene for neurofilament medium) by RT-PCR. Neurogenic differentiation was also assessed by immunofluorescence staining for these antigens.
Osteogenic Differentiation
For osteogenic induction in vitro, SSPCs (1.25 × 105 per dish) were seeded on 35-mm dishes (Corning, Corning, NY) and cultured in the growth medium until the cells reached confluence. To induce osteogenic differentiation, the medium was changed to an osteogenic medium consisting of l-ascorbic acid phosphate and β-glycerophosphate. One week after osteogenic induction, total RNA was extracted and analyzed for osteogenic markers by RT-PCR. After 8 weeks, calcium deposits formed by osteoblast on the dishes were detected by staining with 2% alizarin red S (pH 4.2; Sigma-Aldrich).19,20
Flow Cytometric Analysis of SSPCs
Passage 2 stem cells were cultured under the growth medium. Single-cell suspensions (2 × 105/100 μL each marker) were incubated with R-phycoerythrin (PE) or fluorescein isothiocyanate (FITC) conjugated mouse monoclonal antibodies specific to cell surface markers (1 μg/100 μL each) for 30 minutes on ice. As negative controls, isotype-matched mouse immunoglobulins were incubated instead of the primary antibodies. We analyzed the samples and calculated the data using flow cytometry (FACSCalibur; BD Biosciences).
Semiquantitative RT-PCR
Primers were designed with Primer-BLAST software (http://www.ncbi.nlm.nih.gov/tools/primer-blast/, National Center for Biotechnology Information [NCBI], Bethesda, MD). Total RNA was isolated from the cultures (TRIzol Reagent; Invitrogen), according to the manufacturer's protocols. The cDNA was synthesized from 500 ng of total RNA (Superscript III; Invitrogen). PCR was then performed with gene specific primers and PCR supermix (Platinum; Invitrogen). The primers used are shown in Table 1. The amplified PCR products were subjected to 2% agarose gels which contain ethidium bromide and visualized under UV light. Band intensity was measured by using NIH image-J software and normalized to GAPDH (developed by Wayne Rasband, National Institutes of Health, Bethesda, MD; available at http://rsb.info.nih.gov/ij/index.html).
Table 1.
Primers for Reverse Transcription-Polymerase Chain Reaction
Gene | Primer Sequences | Accession Number |
---|---|---|
ABCG2 | F: 5′-ATGAACTCCAGAGCCGTTAG-3′ | NM_011920.3 |
R: 5′-TTGAAATGGGCAGGTTGAGG-3′ | ||
Notch1 | F: 5′-GGATCACATGGACCGATTGC-3′ | NM_008714.2 |
R: 5′-ATCCAAAAGCCGCACGATAT-3′ | ||
Six2 | F: 5′-GCAAGTCAGCAACTGGTTCA-3′ | NM_011380.2 |
R: 5′-AACTGCCTAGCACCGACTTG-3′ | ||
Pax6 | F: 5′-GCAACCTGGCTAGCGAAAAG-3′ | NM_013627.3 |
R: 5′-CCCGTTCAACATCCTTAGTTTATCAT-3′ | ||
α-SMA | F: 5′-ATGCCTCTGGACGTACAACTG-3′ | NM_007392.2 |
R: 5′-CGGCAGTAGTCACGAAGGAAT-3′ | ||
Vimentin | F: 5′-GTGCGCCAGCAGTATGAAAG-3′ | NM_011701.3 |
R: 5′-GCATCGTTGTTCCGGTTGG-3′ | ||
COLI | F: 5′-GAGCGGAGAGTACTGGATCG-3′ | NM_007742.3 |
R: 5′-TACTCGAACGGGAATCCATC-3′ | ||
COL II | F: 5′-GTCCTTCTGGCCCTAGAGGT-3′ | NM_031163.2 |
R: 5′-TGTTTCTCCTGAGCGTCCA-3′ | ||
CK12 | F: 5′-ACTAGAGCCGACCTGGAAGC-3′ | NM_010661.2 |
R: 5′-ACCTTGGTGAGATCCACTCC-3′ | ||
CK19 | F: 5′-AAGACCATCGAGGACTTGC-3′ | NM_008471.2 |
R: 5′-AATCCACCTCCACACTGACC-3′ | ||
Desmin | F: 5′-GTACCAGGTGTCGCGCACGTCGGG-3′ | NM_010043.1 |
R: 5′-GCTCGGAAGGCAGCCAAGTTGTTC-3′ | ||
LPL | F: 5′-GGGCTCTGCCTGAGTTGTAG-3′ | NM_008509.2 |
R: 5′-AGAAATTTCGAAGGCCTGGT-3′ | ||
PPARg | F: 5′-GCTGTTATGGGTGAAACTCTG-3′ | NM_011146.2 |
R: 5′-ATAAGGTGGAGATGCAGGTTC-3′ | ||
β3-Tubulin | F: 5′-TGAGGCCTCCTCTCACAAGT-3′ | NM_023279.2 |
R: 5′-GGCCTGAATAGGTGTCCAAA-3′ | ||
Nestin | F: 5′-GAGGGGACCTGGAACATGAA-3′ | NM_010277.3 |
R: 5′-GTCCATTCTCCATCCTCCCA-3′ | ||
NFM | F: 5′-GCTGGGTGATGCTTACGACC-3′ | NM_008691.2 |
R: 5′-GCGGCATTTGAACCACTCTT-3′ | ||
GFAP | F: 5′-GAGAACAACCTGGCTGCGTAT-3′ | NM_010277.3 |
R: 5′-GCCTCGTATTGAGTGCGAAT-3′ | ||
Runx2 | F: 5′-GCAGTTCCCAAGCATTTCAT-3′ | NM_009820.2 |
R: 5′-CACTCTGGCTTTGGGAAGAG-3′ | ||
ALP | F: 5′-GCCCTCTCCAAGACATATA-3′ | NM_007431.1 |
R: 5′-CCATGATCACGTCGATATCC-3′ | ||
GAPDH | F: 5′-AACTTTGGCATTGTGGAAGG-3′ | NM_008084.2 |
R: 5′-ACACATTGGGGGTAGGAACA-3′ |
F, forward; R, reverse.
In Vivo Transplantation
Approximately 2 × 106 cells were mixed with 40 mg of HA/TCP ceramic powder (Zimmer, Warsaw, IN) and inserted subcutaneously into the dorsal surface of 8- to 10-week-old female immunocompromised athymic nude-Foxn1nu mice (Harlan; Indianapolis, IN). The transplants were harvested 8 to 10 weeks later.21 For histologic analysis, the tissue samples were fixed with 4% PFA in PBS and decalcified with 5% EDTA solution (pH 7.4). The paraffin-embedded sections were stained with hematoxylin and eosin (H&E).
Histochemistry and Immunohistochemistry
Paraffin-embedded sections were histochemically stained with H&E or Alcian blue. For immunohistochemical analysis, immunolabeled sections using primary antibodies at 4°C overnight, including antibody to type II collagen (mouse IgG1, 1 μg/mL; Chemicon International, Temecula, CA), rabbit antibodies to aggrecan (10 μg/mL, Millipore, Billerica, MA), and antibody to Sox9 (rabbit IgG, 2.5 μg/mL; Abcam, Cambridge, MA), and horseradish peroxidase (HRP)-conjugated secondary (1:200; Santa Cruz Biotechnology, Santa Cruz, CA) antibodies for 1 hour. The DAB reagent (diaminobenzidine tetrahydrochloride) was subsequently used to detect the immunoactivity. The sections were counterstained with hematoxylin.
Immunofluorescent Microscopy
SSPCs were subcultured on eight-well chamber slides (Nunc, Rochester, NY) (104 per well) under the growth medium for 1 day, fixed with 4% paraformaldehyde (PFA; Merck, Darmstadt, Germany), and blocked with normal serum matched to secondary antibodies. The samples were incubated with the specific antibodies to cell surface markers at 4°C overnight and treated with rhodamine or FITC-conjugated secondary antibodies for 1 hour (1:200; Jackson ImmunoResearch, West Grove, PA; Southern Biotechnology, Birmingham, AL). Finally, they were mounted with mounting medium containing 4′,6-diamidino-2-phenylindole (DAPI, Vectashield; Vector Laboratories, Burlingame, CA). For the neurogenesis induced or noninduced SSPCs, the cultured cells were also fixed with 4% paraformaldehyde and followed the above procedures for immunofluorescent staining.
Results
Isolation of SSPCs
The commonly used criteria to define mesenchymal stem cells are clonogenicity, multipotency, and self-renewal. To examine whether SSPCs are clonogenic, we generated and cultured cells isolated from C57BL/6 mouse scleras. A portion of sclera-derived cells attached onto the culture plate forming single colony cluster as evidenced by methyl violet staining (Fig. 1a). SSPCs showed a higher proliferation rate when compared to bone marrow mesenchymal stem cells, as assessed by a BrdU incorporation assay (Fig. 1b). Population-doubling analysis showed that SSPCs possess the ability to self-renew for an extensive period in vitro (Fig. 1c).
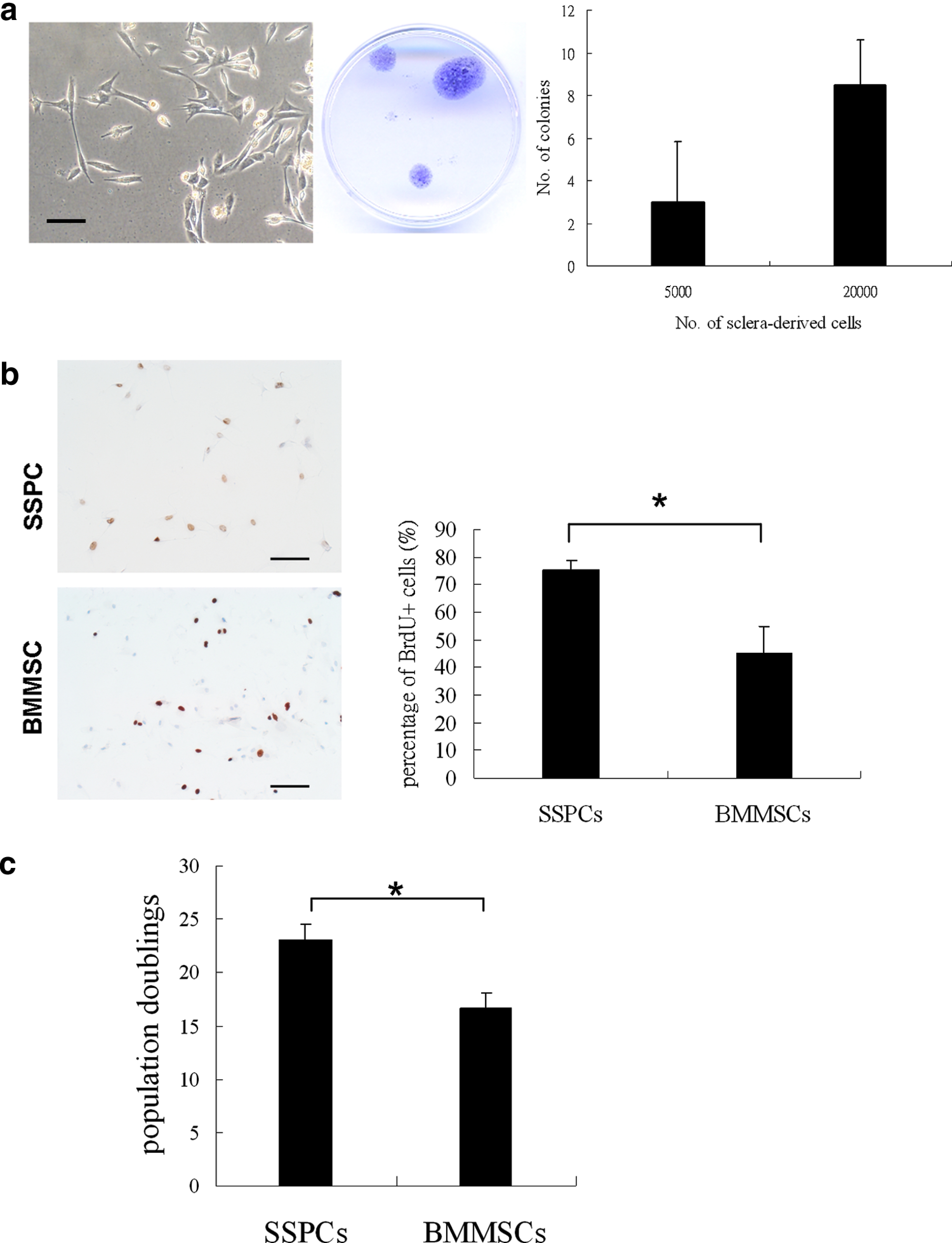
Isolation and characterization of mouse sclera stem/progenitor cells (SSPCs). (a) The colony-forming efficiency of mouse sclera–derived cells. The results shown are mean ± SD of three disks (right). Under a phase-contrast microscope, SSPCs exhibited a fibroblast-like spindle shape (left, bar 200 μm). SSPCs formed single colony clusters as shown by methyl violet staining (middle). (b) Proliferation rate of SSPCs and BMMSCs as measured by BrdU incorporation. Data are mean ± SD of 10 fields, *P < 0.005. (c) Population doublings of multicolony-derived mouse SSPCs and BMMSCs. Data are mean ± SD of three samples. *P < 0.05 SSPCs versus BMMSCs.
Next, we compared the gene expression profile of SSPCs to that of BMMSCs to identify putative SSPC-specific markers. Semiquantitative reverse transcription (RT)-PCR analysis showed that SSPCs express high levels of stem cell markers ABCG2, Six2, Pax6, and Notch1. With regard to lineage-associated genetic markers, SSPCs expressed Pax6 (neurogenic and ocular developing marker), vimentin (mesenchymal cell marker), a-SMA (α-smooth muscle actin, cellular activation, and myogenic marker), and type I collagen (major composition protein of sclera), but failed to express cytokeratin 12 (corneal epithelium marker), cytokeratin 19 (corneal limbal stem cell specific marker), and desmin (mesoderm stem cell marker) transcripts. This implies that the isolated cells were not from a corneal epithelial, corneal limbal stem cell, or muscular origin. Immunocytochemical staining further confirmed that all SSPCs expressed high levels of type I collagen and α-SMA compared with BMMSCs (Fig. 2b).
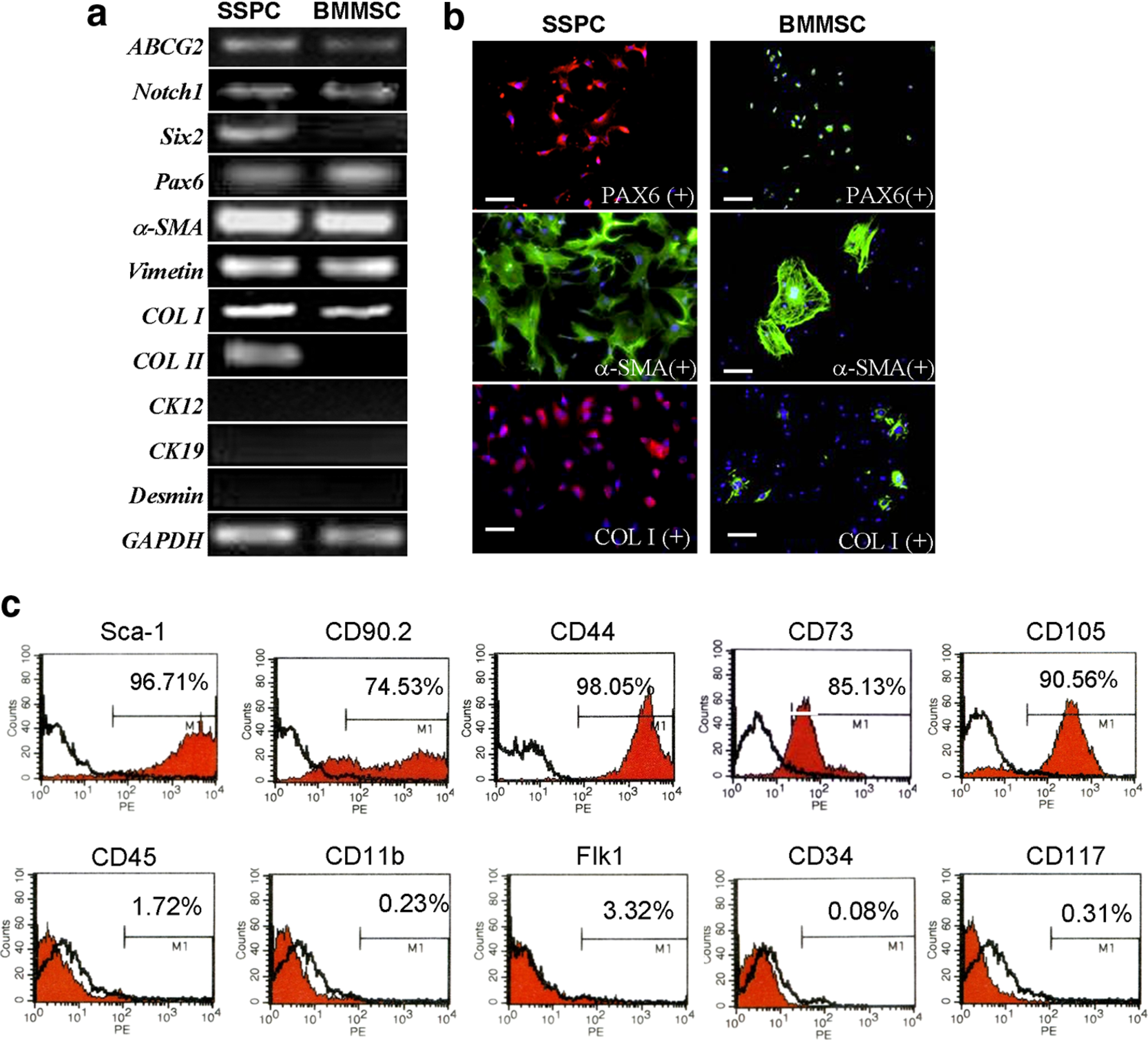
Phenotype for SSPCs. (a) RT-PCR analysis of gene expression profiles related to stem cells (ABCG2 and Notch1), neural crest origin (Six2), eye development (Pax6), sclera (a-SMA, vimentin, and collagen I), and keratocytes (Ck12, Ck19, and desmin). (b) Immunostaining for proteins related to scleral tissue and stem cells in mouse SSPCs and BMMSCs. Bars, 100 μm. (c) Flow cytometry analysis for the expression of cell surface markers related to mesenchymal stem cells (Sca-1, CD90.2, CD44, CD73, and CD105), hematopoietic stem cells (CD34, CD117), leukocytes (CD45), macrophages (CD11b), and endothelial cells (Flk-1) on mouse SSPCs.
To examine surface molecule expression on SSPCs, flow cytometric analysis was used to show that more than 96% of SSPCs at passage 2 were positive for stem cell antigen-1 (Sca-1), and more than 70% of SSPCs were positive for a mesenchymal cell marker, CD90.2. SSPCs also expressed high levels of CD44, a cell-surface glycoprotein involved in cell–cell interactions, cell adhesion, and migration. CD44 is also a receptor for hyaluronic acid and can interact with other ligands, such as osteopontin, collagens, and matrix metalloproteinases (MMPs). Approximately 90% of SSPCs were positive for anti-CD105 antibody staining. CD105, also named Endoglin, is a type I membrane glycoprotein located on the cell surface and is part of the TGF-β receptor complex. It may be involved in the cytoskeletal organization affecting cell morphology and migration. SSPCs were negative for leukocyte marker CD45, endothelial cell markers Flk-1, macrophages/monocyte antigen CD11b, and hematopoietic stem cell markers CD34 and CD117(c-kit), thus verifying the lack of contaminating hematopoietic cells and endothelial cells.
Our data showed that the surface markers of SSPCs were in agreement with the definition of mesenchymal stem cells (MSCs).
Multipotent Differentiation of SSPCs
The differentiation potentials of SSPCs toward adipogenesis, chondrogenesis, and osteogenesis lineages were determined by comparison to those of BMMSCs. Oil-red-O staining showed that SSPCs were analogous to BMMSCs with regard to forming lipid droplet containing adipocytes and upregulated expression of the adipogenic genes peroxisome proliferator-activated receptor-γ (PPAR-g) and lipoprotein lipase (Lpl/LPL).
Chondrogenic differentiation was induced by a pellet culture approach16,22 and assessed by immunostaining. We found that SSPCs were similar to BMMSCs in terms of expressing chondrogenic markers type II collagen, aggrecan, and Sox9 when cultured under the chondrogenic induction conditions (Fig. 3b). In addition, SSPCs showed positive Alcian blue staining when differentiated into chondrocytes in vitro.
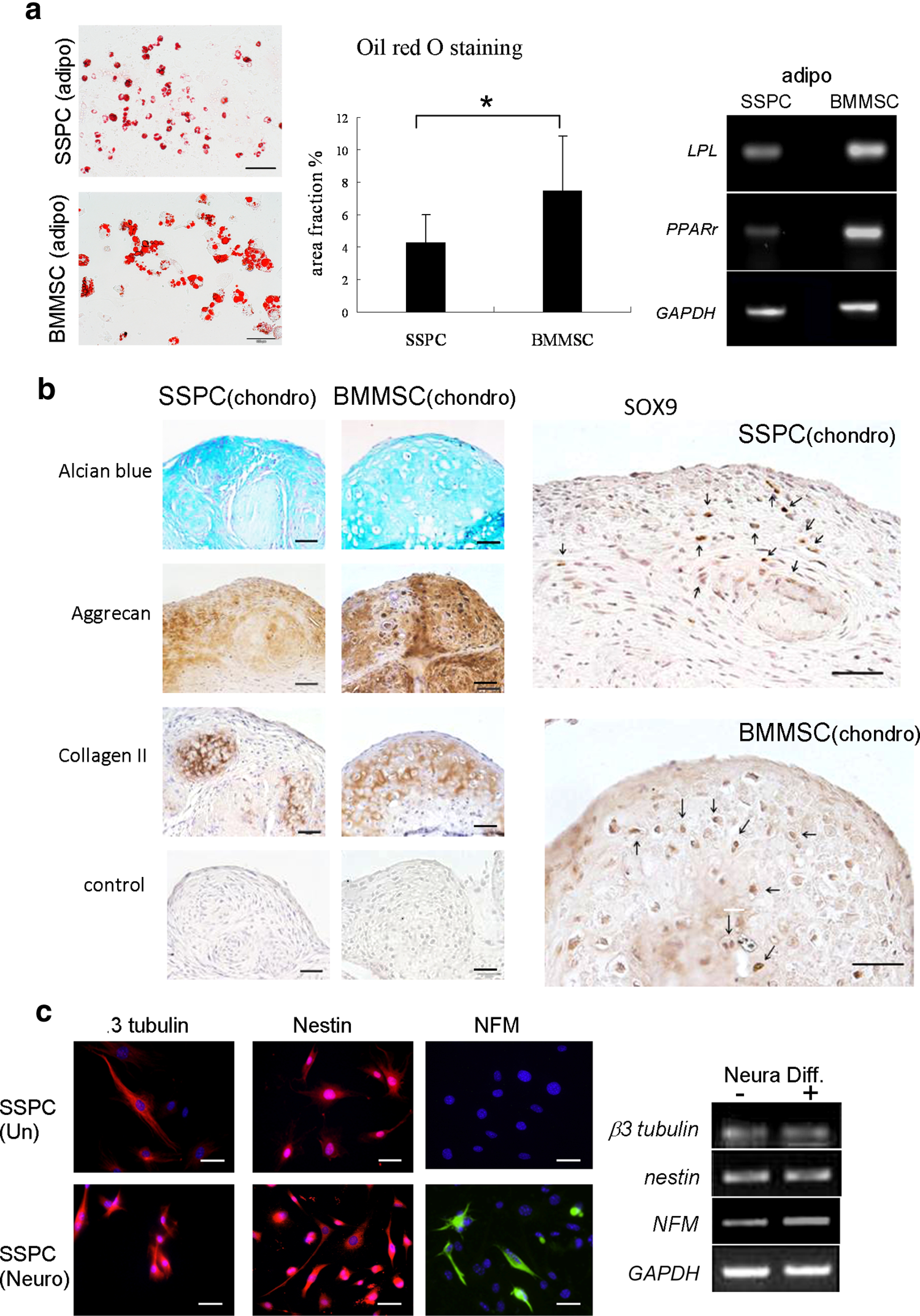
Multipotent differentiation capabilities of putative mouse SSPCs in vitro. (a) Oil-red-O staining showed adipogenic differentiation in SSPCs (top left) and BMMSCs (lower left). Bar, 100 μm. The number of Oil-red-O–positive droplet-containing cells were counted and shown as a percentage of Oil-red-O–positive area over total area. (middle) *P < 0.005. RT-PCR (right) showed gene expression profiles related to adipogenesis in SSPCs compared to BMMSCs. (b) Chondrogenic differentiation of SSPCs and BMMSCs. Chondrogenic differentiation was assessed by Alcian blue staining for proteoglycans and the expression of aggrecan, type II collagen, and SOX9 (arrows: positive nuclear stain). Bar, 100 μm. (c) Neurodifferentiation of SSPCs. Immunofluorescent staining showed SSPCs expressing β3-tubulin, Nestin, and NFM. RT-PCR (right) confirmed that gene expression profiles of SSPCs expressed neural markers as described above. After 4 weeks of culture in the presence of B27 supplement, bFGF (40 ng/mL), and epidermal growth factor (20 ng/mL; Neural Diff.+), expression levels of NFM and β3-tubulin were upregulated when compared with levels in regular culture conditions (Neural Diff.−). However, expression levels of Nestin remained the same after treatment.
When cultured under neurogenesis conditions, SSPCs lost their fibroblastic morphology, developed multicytoplasmic processes, and formed a bipolar axon neuronlike morphology (Fig. 3c). To elucidate the neural-differentiation potential of SSPCs, we examined the expression of neural markers in SSPCs. We found that cultured SSPCs expressed several neural cell markers including β3-tubulin, nestin, and NFM, as measured by immunocytochemical staining and RT-PCR analysis. After 4 weeks of neural inductive culture, expression levels of nestin remained the same, but β3-tubulin and NFM increased (Fig. 3c).
To induce osteogenic differentiation, confluent cells were cultured in osteo-inductive medium for 4 weeks. SSPCs failed to form mineralized nodules, as observed in BMMSCs by alizarin red staining (Fig. 4a, left). To further confirm that SSPCs lack osteogenic differentiation potential, we showed that SSPCs express lower levels of alkaline phosphatase (Alp) and osteocalcin (Ocn) than BMMSCs under the osteoinductive cultures.
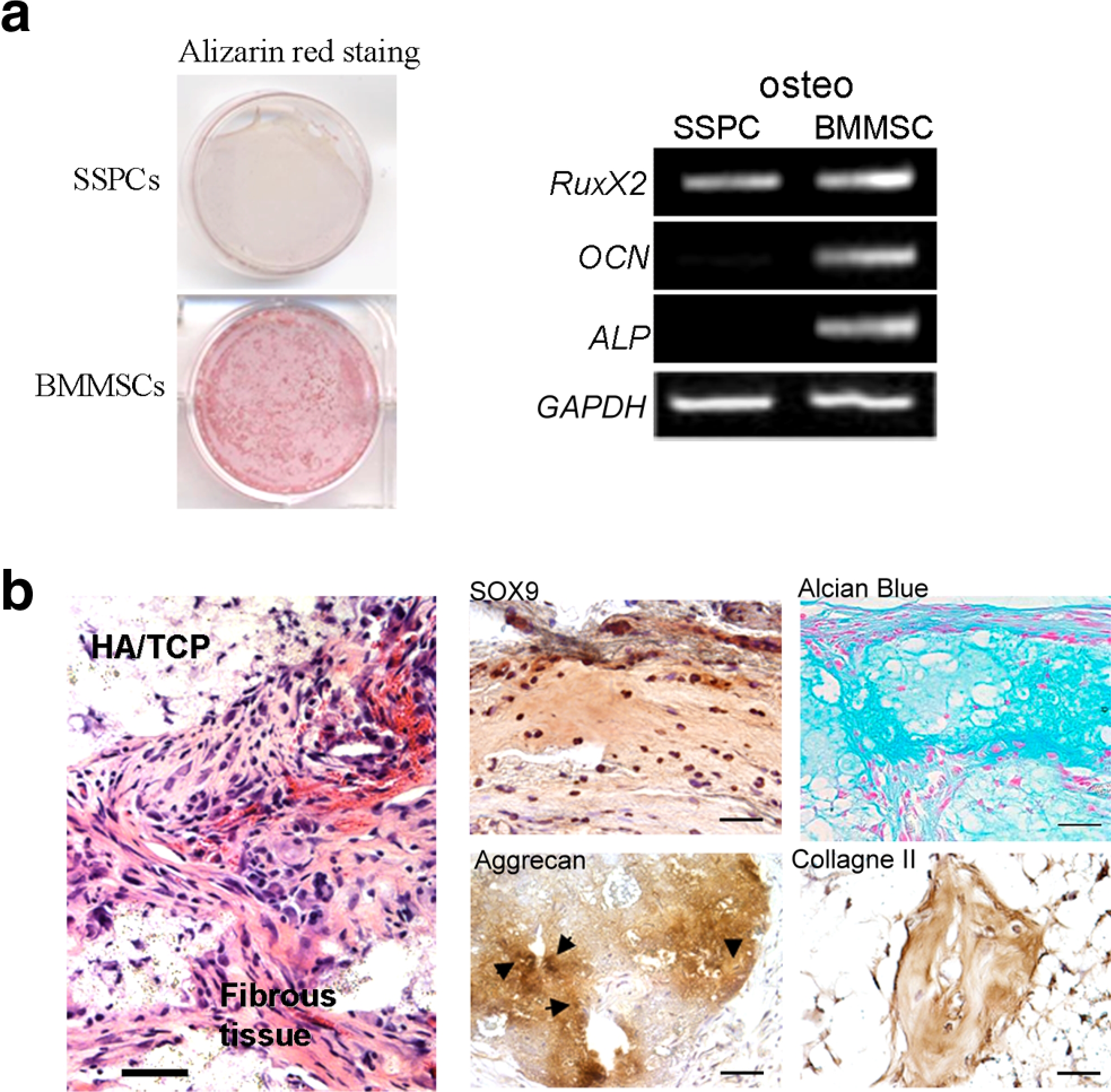
SSPCs failed to develop osteogenic tissue in vitro and in vivo. (a) Osteogenic differentiation of SSPCs and BMMSCs. The Alizarin red S staining showed that SSPCs (top left) could not be induced to form red mineralizing areas in comparison with BMMSCs (lower left). RT-PCR (right) showed that the osteogenic gene expression profile was far less in osteogenic SSPCs compared to BMMSCs. (b) Multipotential differentiation of mouse SSPCs in vivo. Bar, 50 μm. H&E-stained sections of transplant (left) showed sclera-like connective tissue around the carrier (HA/TCP). The tissue was positively stained for Sox9 (top middle, positive nuclear stained) and aggrecan (bottom middle) along the margin of HA/TCP and some cartilage lacuna-like tissue (arrow) was found in the aggrecan-positive area. Alcian blue–positive staining showed that this tissue contained proteoglycans (top right). Collagen II-expressing tissue may be found in the HT/TCP area. The immunostaining showed that fibrocartilage had formed.
In Vivo Tissue Regeneration
To determine the multidifferentiation potential of SSPCs in vivo, we transplanted SSPCs into immunocompromised mice SC using hydroxyapatite tricalcium phosphate as a carrier. At 8 weeks after implantation, there was no bone formation observed in SSPC implants (Fig. 4b). Connective tissue in the implants was morphologically similar to scleral tissue, but was positive for Sox9 and aggrecan antibody staining. Positive staining for proteoglycans by Alcian blue was also seen in the connective tissues.
Discussion
In this study, we identified a unique MSC population residing in the mouse sclera, which we have termed SSPCs. SSPCs posses the capacity for self-renewal and multipotent differentiation. The isolation and characterization of SSPCs may provide a new tool for the study of basic biology of the sclera.
The sclera exhibits low cellularity compared with most vascularized tissues. It was considered that sclera cells are quiescence residents between fibrous layers.23 Cell-based studies of the sclera often use explants to obtain cell cultures.24–26 Approximately 10 to 14 days after explantation, the cells, termed sclerocytes, rapidly transform into active “scleral fibroblasts.” These characteristics of cell proliferation and transformation hint at the possibility that stem/progenitor cells reside in the sclera. These scleral fibroblasts or part of them may be stem/progenitor cells that have not been identified.
SSPCs failed to undergo osteogenic differentiation when cultured in osteoinductive medium or after in vivo transplantation. It has been reported that gingival stem/progenitor cells also cannot form mineralized tissue in vivo.27 SSPCs may need additional supplemental growth factors, such as BMPs, for further induction of osteocytes rather than only osteoinductive medium. The fate of in vivo lineage commitment by MSCs depends on multiple factors such as different tissue origins, the hierarchy of lineage commitment, cell culture conditions, local growth factors, and transplantation conditions (carriers and recipients).28,29
Seko et al.13 showed that explants from human scleral tissue possessed a chondrogenic lineage potential. They described how the sclera of certain vertebrates, such as birds and fish, is composed of hyaline cartilage. Although the human sclera is not a cartilaginous tissue, the human sclera has maintained chondrogenic potential throughout evolution. In addition, they directly explain the enigma of why the sclera and the joint cartilage are common targets of inflammatory cells in rheumatic arthritis. These cells may play a role in inflammatory and immune response of scleritis. Further study for the relationship between SSPCs and immune response is worth performing.
SSPCs may be a resource for the bioengineering of artificial scleras and for cell-based therapy. Myopia has become a public health issue worldwide.30–34 High myopia is associated with an increased risk for several ocular diseases such as cataract, glaucoma, retinal detachment, myopic retinal degeneration, visual impairment, and blindness.35–38 The detailed mechanism of myopia development is unknown. Scleral tissue may be responsible for myopia development due to its significant structure alteration in myopia.39 SSPCs may help to clarify the pathogenesis of developmental diseases such as childhood myopia and remain a source for further studies.
A mouse model of myopia was established in 2008,40 and as a result of the recent complete genomic sequence disclosure, the mouse eye has become a very important model for research on eye growth and myopia. In addition, sclera diseases such as refractory scleritis and scleromalacia are also difficult to cure and lead to blindness. Scleritis is often the initial or only indication of severe and life-threatening systemic autoimmune diseases such as rheumatoid arthritis, Wegener's granulomatosis, systemic lupus erythematosus, and relapsing polychondritis.41,42 An autoimmune dysregulation in a genetically predisposed host is presumed to cause scleritis. Recently, mesenchymal stem cells were reported to have abilities and functions in immunomodulation,43–45 and we propose that SSPCs may play an important role in the development of scleritis. Identification of a multipotent stem cell in the sclera is an important first step in developing future cell-based studies and/or treatments for myopia, scleritis and other scleral disease.
We present a concept that the mouse sclera contains a unique group of multipotent mesenchymal stem cells. This finding may provide a tool to help clarify mechanisms of ocular development and disease and will allow us to investigate the possible role of this group of cells in further studies.
Acknowledgments
The authors thank Gabriel Gordon for reading and editing the manuscript and Yan Chen, Yi Liu, and Chider Chen for technical assistance.
Footnotes
Supported by National Institute of Dental and Craniofacial Research Grants R01 DE017449, R01 DE019932, and R01 DE019413 (SS) and National Eye Institute Grants and R01 EY09828 (MEF) and P30 EY003040 to Doheny Eye Institute and Grant RN1–00572 from the California Institute for Regenerative Medicine (SS).
Disclosure: C.-L. Tsai, None; P.-C. Wu, None; M. Elizabeth Fini, None; S. Shi, None
References
Articles from Investigative Ophthalmology & Visual Science are provided here courtesy of Association for Research in Vision and Ophthalmology
Full text links
Read article at publisher's site: https://doi.org/10.1167/iovs.11-7676
Read article for free, from open access legal sources, via Unpaywall:
https://europepmc.org/articles/pmc3262553?pdf=render
Citations & impact
Impact metrics
Citations of article over time
Alternative metrics
Smart citations by scite.ai
Explore citation contexts and check if this article has been
supported or disputed.
https://scite.ai/reports/10.1167/iovs.11-7676
Article citations
RAD21 deficiency drives corneal to scleral differentiation fate switching via upregulating WNT9B.
iScience, 27(6):109875, 03 May 2024
Cited by: 0 articles | PMID: 38774716 | PMCID: PMC11107359
Biofabrication of Artificial Stem Cell Niches in the Anterior Ocular Segment.
Bioengineering (Basel), 8(10):135, 30 Sep 2021
Cited by: 4 articles | PMID: 34677208 | PMCID: PMC8533470
Review Free full text in Europe PMC
Effects of carbon and nitrogen plasma immersion ion implantation on bioactivity of zirconia.
RSC Adv, 10(59):35917-35929, 30 Sep 2020
Cited by: 9 articles | PMID: 35517098 | PMCID: PMC9056952
Distribution of Stromal Cell Subsets in Cultures from Distinct Ocular Surface Compartments.
J Ophthalmic Vis Res, 15(4):493-501, 25 Oct 2020
Cited by: 2 articles | PMID: 33133440 | PMCID: PMC7591840
Dasatinib inhibits peripapillary scleral myofibroblast differentiation.
Exp Eye Res, 194:107999, 13 Mar 2020
Cited by: 4 articles | PMID: 32179077 | PMCID: PMC7217736
Go to all (12) article citations
Data
Data behind the article
This data has been text mined from the article, or deposited into data resources.
BioStudies: supplemental material and supporting data
RefSeq - NCBI Reference Sequence Database (Showing 19 of 19)
- (2 citations) RefSeq - NM_010277.3
- (1 citation) RefSeq - NM_011920.3
- (1 citation) RefSeq - NM_013627.3
- (1 citation) RefSeq - NM_011701.3
- (1 citation) RefSeq - NM_007431.1
- (1 citation) RefSeq - NM_008084.2
- (1 citation) RefSeq - NM_011380.2
- (1 citation) RefSeq - NM_010661.2
- (1 citation) RefSeq - NM_007392.2
- (1 citation) RefSeq - NM_007742.3
- (1 citation) RefSeq - NM_008691.2
- (1 citation) RefSeq - NM_031163.2
- (1 citation) RefSeq - NM_008471.2
- (1 citation) RefSeq - NM_008509.2
- (1 citation) RefSeq - NM_010043.1
- (1 citation) RefSeq - NM_011146.2
- (1 citation) RefSeq - NM_023279.2
- (1 citation) RefSeq - NM_008714.2
- (1 citation) RefSeq - NM_009820.2
Show less
Similar Articles
To arrive at the top five similar articles we use a word-weighted algorithm to compare words from the Title and Abstract of each citation.
Culture and identification of multipotent stem cells in guinea pig sclera.
Int Ophthalmol, 43(1):113-120, 06 Jul 2022
Cited by: 0 articles | PMID: 35794406
Isolation and characterization of multipotent mesenchymal stem cells in nasal polyps.
Exp Biol Med (Maywood), 240(2):185-193, 06 Oct 2014
Cited by: 14 articles | PMID: 25294891 | PMCID: PMC4935324
Chondrogenesis in scleral stem/progenitor cells and its association with form-deprived myopia in mice.
Mol Vis, 21:138-147, 06 Feb 2015
Cited by: 14 articles | PMID: 25684979 | PMCID: PMC4323720
PDGFR-β (+) perivascular cells from infantile hemangioma display the features of mesenchymal stem cells and show stronger adipogenic potential in vitro and in vivo.
Int J Clin Exp Pathol, 7(6):2861-2870, 15 May 2014
Cited by: 8 articles | PMID: 25031705 | PMCID: PMC4097281
Funding
Funders who supported this work.
NEI NIH HHS (3)
Grant ID: R01 EY009828
Grant ID: P30 EY003040
Grant ID: R01 EY09828
NIDCR NIH HHS (3)
Grant ID: R01 DE017449
Grant ID: R01 DE019413
Grant ID: R01 DE019932