Abstract
Free full text

Differential Macrophage Programming in the Tumor Microenvironment
Abstract
Of the multiple unique stromal cell types common to solid tumors, tumor-associated macrophages (TAMs) have been recognized as significant for fostering tumor progression. The protumor properties of TAMs are derived from their ability to regulate angiogenic programming, provide soluble mediators to malignant cells for proliferation, survival and invasion, and for directly and indirectly suppressing activity of cytotoxic T cells. These varied activities are dependent on the polarization state of TAMs that is regulated in part by local concentrations of cytokines and chemokines, as well as varied interactions of TAMs with normal and degraded components of the extracellular matrix. Targeting molecular pathways regulating TAM polarization holds great promise for anti-cancer therapy.
Macrophages in solid malignancies
Macrophages are important residents of all tissues, where they play critical roles in regulating tissue homeostasis. In this context, tissue-resident macrophages assist with combating infection [1, 2], resolving acute inflammation [3], and in regulating metabolic response to tissue stress [4, 5]. This broad range of functions, and the accompanying plasticity required to permit such adaptive responses, also implicates macrophages in a number of chronic pathological conditions including diabetes and atherosclerosis [5–7]. Solid tumors represent an extreme example of a dysregulated tissue, and multiple characteristics of tumors, including hypoxia [8] and abundant cell death [9], help direct macrophage function towards attempting homeostatic restoration. In the context of a tumor however, this represents a maladaptive response that instead helps drive tumor growth through promotion of angiogenic programs, tissue remodeling, ectopic survival of malignant cells, and development of immunosuppressive microenvironments that blunt cytotoxic T cell activities [10]. More recently, it has been demonstrated that polarization of macrophages towards tumor promoting phenotypes, is not exclusively the result of thwarted tissue homeostasis, but instead a more active process driven by what are likely reciprocal interactions with both malignant and stromal cells in the local microenvironment [10, 11]. Thus in addition to discussing well accepted functions for tumor-associated macrophages (TAMs; Box 1), this review will also focus on recently recognized molecular and cellular mechanisms underlying TAM polarization within tumor microenvironments, and the therapeutic implications of these findings.
TAM Function
With the exception of non-small cell lung carcinoma [12, 13], patient prognosis in solid tumors is generally described as correlating inversely with TAM density and TAM expression signatures [10, 14]. TAMs have also been related to particular functional roles in human tumors, with an established association between TAM presence and density of tumor vasculature in several carcinomas [15–18] including breast [19–24], as well as increased local invasion and/or metastasis in melanoma [25], breast [26, 27], ovarian [15, 28], colorectal [29, 30], pancreatic neuroendocrine [31] and bladder cancer [32]. Less established in humans is a role for TAMs in local immune suppression, although there have been several reports in ovarian cancer [33-35]. A potential caveat of these studies is that most rely on immunohistochemical detection of CD68 for measurement of TAM density. However, CD68 is not a specific marker of macrophages and is expressed by other stromal populations (Box 1), including those that may have overlapping function with TAMs (Box 2).
Angiogenesis
Experimental studies using murine carcinoma models have clearly demonstrated that TAMs regulate vascular programming of tumors. Important for this activity is TAM production of vascular endothelial growth factor A (VEGFA), because overexpression of VEGFA partially reverses the effects of macrophage-depletion [36], and macrophage-specific loss of VEGFA results in vascular normalization [37]. In some tumor models, production of matrix metalloproteinase (MMP)-9 by TAMs mediates VEGFA bioavailability, thus providing an alternative, but still VEGF-dependent route for promoting angiogesis [38, 39]. Similarly, the TAM production of placental growth factor (PIGF), a homologue of VEGFA that selectively binds VEGF receptor 1 (VEGFR1), also stimulates angiogenesis in tumors [40] and may in part explain resistance to VEGFA and VEGFR targeted therapies in the clinic [41–43]. Multiple other factors produced by macrophages are also known to regulate vascular programming [44–46], but their roles in tumorigenesis are not firmly established and therefore will not be discussed herein.
Recently a novel subset of macrophages expressing Tie2, a receptor for angiopoeitins (ANG) was described [47]. Tie2+ TAMs tend to be closely associated with tumor vasculature, and have been found crucial for angiogenesis in orthotopic [47] and transgenic tumor models [48]. This activity depends in part on endothelial cell-produced ANG2 and the Tie2 receptor that direct localization of Tie-2-expressing cells along the vasculature [48]. Notably, antagonists that block ANG2 restrict tumor growth in mouse models of de novo mammary carcinogenesis, i.e., MMTV-PyMT mice [49], a property that has thus far not been observed in tumors following blockade of TAM infiltration [50], or by myeloid-specific Vegfa ablation [37], thus hinting at a unique mode of action either downstream or parallel to VEGFA bioactivity [48].
Invasion and metastasis
The most comprehensively described mechanism through which TAMs promote solid tumor development is by providing factors that enhance invasion of malignant cells into ectopic tissue. This activity centers around a paracrine interaction loop involving macrophage-expressed epidermal growth factor (EGF) and epithelial cell-expressed colony-stimulating factor (CSF)1, also known as macrophage CSF [51]. Increased expression of CSF1 is a significant mechanism underlying macrophage recruitment into tissues [50, 52–54] after CSF1 binding to its high affinity receptor (CSF1R) expressed on resident macrophages and some macrophage precursors. This interaction promotes macrophage proliferation, survival, and tissue recruitment during development (e.g., branching morphogenesis in the mammary gland) [55], homeostasis [56], and pathological tissue remodeling processes such as those associated with acute tissue injury [53,57–59] and during solid tumor development [50]. Chemokines such as CCL2 and stromal-derived factor-1 (SDF-1; CXCL12) are also important for TAM recruitment with demonstrated roles in models of glioblastoma, melanoma, cervical and prostate cancer [38, 39, 60–65]. Crucially, in addition to supporting TAM recruitment, activation of intracellular signaling pathways downstream of the CSF1-CSF1R interaction significantly enhance EGF expression, which in turn regulates epithelial cell migration in some tissues [51, 66–68].
Macrophages also regulate composition and structure of extracellular matrix (ECM) through their deposition of ECM components, e.g. various types of collagens, and breakdown of these same components via their release of MMPs, serine proteases and cathepsins [69–71]. Migration on and through ECM is a necessary aspect of cell migration [72], and thus by extension, TAMs are thought to regulate tumor and stromal cell migration/invasion through ECM. This activity has been directly demonstrated in vitro [73, 74] and in vivo with mice genetically deficient for cathepsin B, cathepsin S and urokinase/plasminogen activator (uPA), all of which derive primarily from TAMs in the tumor microenvironment [75–77]. MMP-dependent cleavage of the ECM [69], collagen deposition [78], and alteration of collagen structure [79] are also potential sources of regulation mediated by TAMs, but formal demonstration of these is lacking.
Immune suppression
Solid tumors are well recognized for repressing the activity of cytotoxic T cells, and are thus characterized grossly as immunosuppressive. While regulatory T (Treg) cells have garnered much attention owing to this capability, recent literature also recognizes macrophages as major determinants of immune suppression in solid tumors. Although the mechanisms underlying these activities are less characterized as compared to myeloid-derived suppressor cells for example [80], TAMs do typically express several genes with immunosuppressive potential [68, 81, 82], and are capable of directly limiting T cell proliferation during classic in vitro suppressive assays [8, 34, 50, 83]. TAMs also act through intermediates to regulate immune suppression, as has been reported for recruitment of regulatory T cells by CCL22 [33], and may be important mediators of T cell recruitment based upon the inverse correlation between the presence of CD68+ TAMs and CD8+ T cells in human breast cancer and the enhanced CD8+ T cell infiltration observed during chemotherapy in the MMTV-PyMT model following blockade of the CSF1-CSF1R pathway [50].
In murine tumor models, suppression of CD8+ T cell proliferation by TAMs is at least partly dependent on metabolism of L-arginine via Arginase-1 or inducible nitric oxide synthase (iNOS) [8, 83], and resulting production of oxygen radicals or nitrogen species [84, 85]. In contrast, suppression of CD8+ T cells by human TAMs can occur independent of L-arginine metabolism [34], and instead may rely on the B7 family of molecules as has been described for B7-H1 (PD-L1) in hepatocellular carcinoma [86], and B7-H4 in ovarian cancer [34]. This distinction may be a result of differences between human and murine macrophages, as expression of arginase-1 by the former does not correlate with macrophage polarization. Alternatively, the tissue specific microenvironment could dictate the mechanism of TAM suppression. As an example of this, it was described that ovarian TAMs are exposed to minimal levels of IL-4, which in other circumstances may down-regulate B7-H4 expression [34]. TAMs from murine mammary and human breast carcinomas on the other hand are exposed to significantly higher amounts of IL-4/13 [68, 87], and thus one would anticipate minimal expression of B7-H4 in TAMs from these tissues.
Mechanisms of TAM Polarization
The description of macrophage activation as either classical (M1; IFNγ/LPS-dependent) or alternative (M2; IL-4/IL-13/IL-10/FcγR-dependent) has provided a necessary framework for the understanding of TAM polarization [88]. However, even though the M1/M2 designations represent extreme ends of a scale, the concept is an oversimplification of the diversity of TAM phenotypes evident simply based on their localization within tumors (Figure 1). TAMs do not become polarized by virtue of their location per se, but instead receive signals from the particular microenvironment they reside (Figure 2). These heterotypic signals may overlap, for example, IL-4 promotes both Egf and Arginase-1 expression by TAMs [68], whereas other signals may be unique to particular microenvironments, such as hypoxic zones within solid tumors that also induce Arginase-1 expression [8], but which are not found along vasculature where invasive macrophages are localized. From these two examples, all murine TAMs would be anticipated to express higher levels of arginase-1 than normal tissue macrophages, but the suppressive capabilities of intratumoral TAMs in hypoxic regions would be even further enhanced. Thus, in addition to differentiation of TAMs, from either Tie2+ [89] or other monocyte precursors [83] that may preferentially home to specific localizations, one can envision that integration of these distinct signals result in production of an array of TAM populations/phenotypes with unique tumor-regulating properties [10]. It is important to note that the composition of the immune microenvironment [68, 90, 91] and the overall polarization state of TAMs becomes more favorable towards growth during tumor progression [92]. The functional role of macrophages during tumor initiation [93, 94] therefore differs from that during tumor progression.
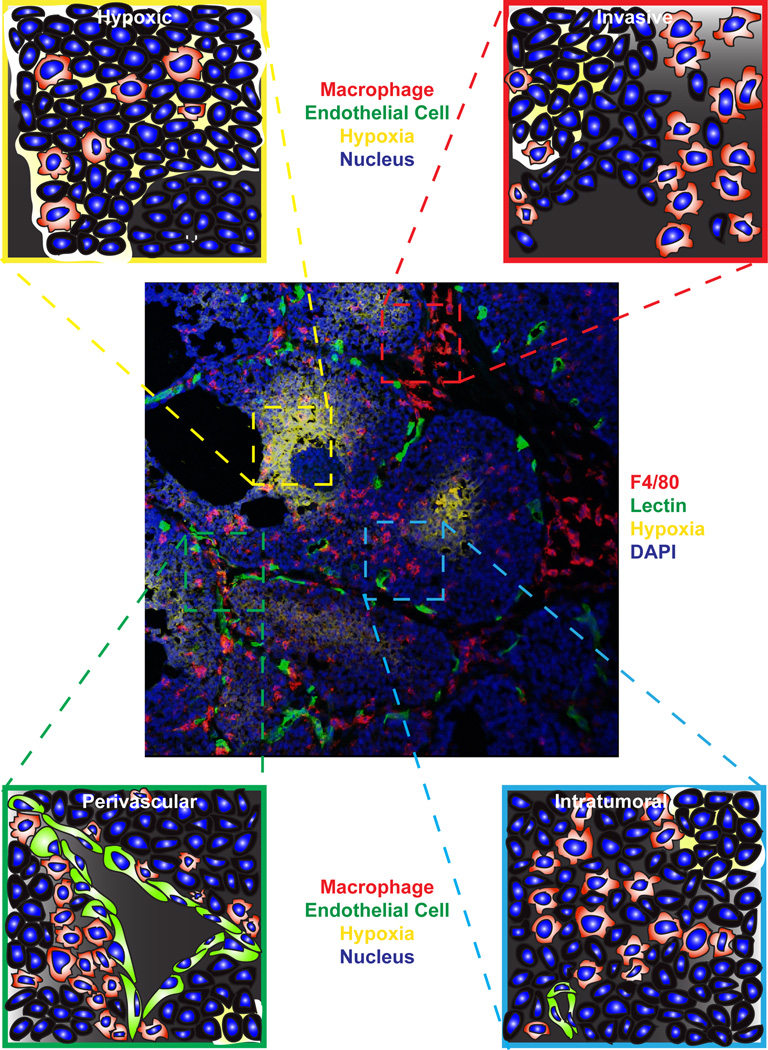
TAM localization within unique tumor microenvironments. Centrally displayed is an immunofluorescent confocal micrograph of F4/80+ macrophages (red) within a late-stage tumor from the MMTV-PyMT mouse model of mammary carcinogenesis. Also shown are areas of hypoxia detected with pimonidazole (yellow), functional vasculature as revealed by perfusion with tomato lectin (green) and DAPI nuclear stain (blue). Clockwise from top left insets display enlarged graphical representations of TAMs within a hypoxic region, at a locally invasive edge, in a normoxic area within the tumor, and associated with the vasculature.
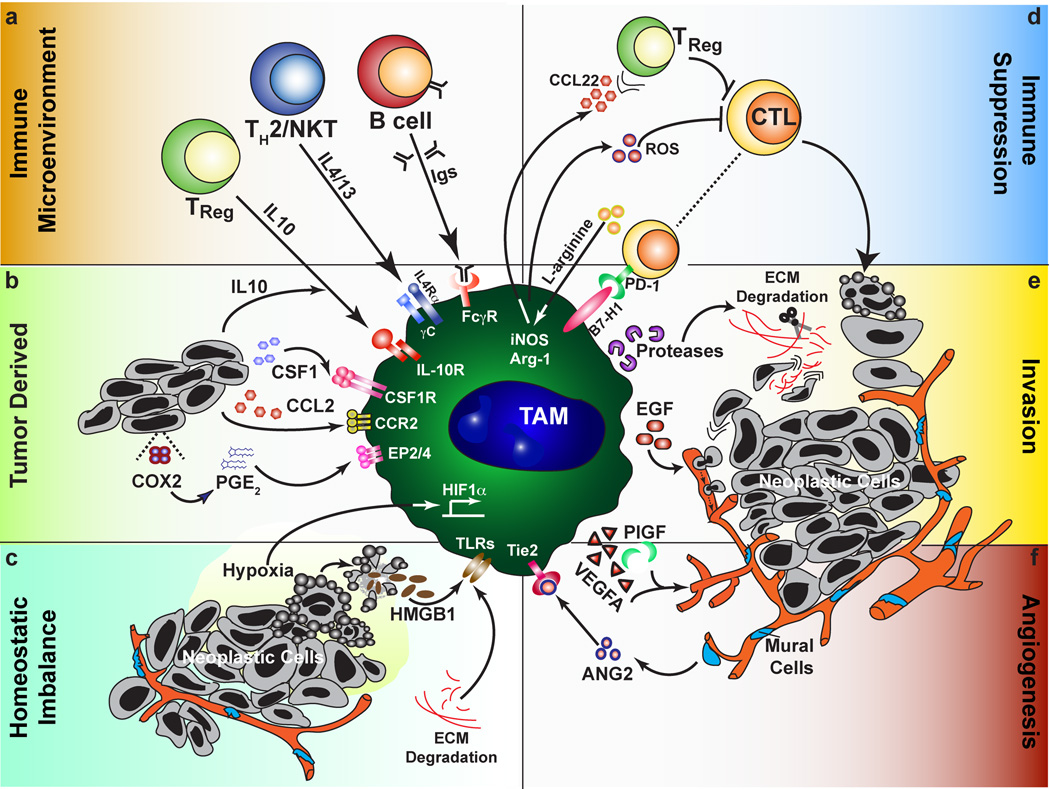
TAMs as central regulators of the tumor microenvironment. Factors that promote the polarization of TAMs towards a pro-tumor phenotype (a–c) can be subdivided into those derived from the immune system, actively produced by tumor cells, or resulting from tissue stress. (a) From leukocytes, this includes cytokines and other soluble factors such as immune complexes. (b) Neoplastic cells can produce chemokines that recruit macrophages, including CSF1 and CCL2 depending on the tissue involved, as well as directly producing immunosuppressive molecules such as IL-10 and PGE2. (c) Signs of dysregulated tissues include leaky vasculature, hypoxia, ECM remodeling and cell death. These signals all direct the pro-tumor functions of TAMs (d–f) including immune suppression, tumor cell dissemination, and promoting angiogenesis. (d) Immune suppression can occur through soluble or cell surface mediators, and may be indirect such as through the recruitment of regulatory T cells. (e) Neoplastic cell invasion of ectopic tissue can be promoted through directed release of cytokines such as EGF, or through protease-dependent ECM remodeling that may directly affect neoplastic migration or increase chemoattractant bioavailability. (f) In addition to the interplay of TAMs with endothelial cells through production of VEGFA and other angiogenic factors, subsets of TAMs expressing the Tie2 receptor interact with mural cells/pericytes to regulate vascular structure.
Immune Microenvironment
Consistent with the original description of alternative activation, the type 2 cytokine IL-4 [68, 77], and immunoglobulin (Ig) signaling through activating-types of Fcγ receptors [91], exert significant regulation on TAMs in their ability to direct TAM pro-tumor phenotype. IL-13 derived from malignant epithelial cells or TH2-polarized CD4+ T cells [87], or CD1d-restricted natural killer T (NKT) cells [95], may have similar effects on TAM polarization due to overlapping IL-13 and IL-4 signaling cascades that lead to STAT6 activation, but this has yet to be proven in vivo. Likewise, activation of STAT3 by IL-10 suppresses IL-12 [96] and tumor necrosis factor-α (TNF-α) expression [97] by TAMs, but the source of IL-10 and the relevance of this pathway to tumor development is unclear. Intriguingly, while IL-4 from TH2-polarized CD4+ T cells is necessary for TAM programming in the MMTV-PyMT mammary carcinoma model for EGF expression and promotion of metastasis, the absence of Igs in B cell-deficient mice does not affect tumorigenesis [68]. Conversely, autoantibodies in the K14-HPV16 mouse model of squamous carcinogenesis drive TAM-dependent angiogenesis by FcRγ-dependent mechanisms [91], whereas CD4+ T cell-deficient animals have only mildly reduced tumor incidence [98]. These paradoxical findings likely indicate tissue-specific dependencies in each respective tumor model, and argue for detailed analysis of counterpart human tissue as a precursor to clinical translation because human tumors could vary considerably in leukocyte composition.
Such human/mouse differences have been described in breast/mammary cancer. Notably, leukocyte composition in murine mammary carcinomas are dominated by TAMs, while lymphocytic infiltrate, predominately CD4+ T cells, comprise the majority of immune cells in human breast carcinomas [68, 99]. CD4+ T cells from human breast cancers express high levels of interferon (IFN)γ resulting in protein concentrations over 10-fold higher than IL-4 or IL-13 in human tumors [87]. This suggests divergent cytokine milieus between human and mouse tissue, as tumor CD4+ T cells from MMTV-PyMT-derived mammary tumors exhibit high expression of IL-4, IL-13 and IL-10, but produce minimal IFNγ [68]. Given the strong polarizing effects of these cytokines, and the potential for synergistic effects on macrophage phenotype [100], transcriptional profiling of purified human TAMs should prove informative in relating functional data in mouse models to correlative studies in patients.
Tumor derived
In addition to its potential production by multiple leukocyte subsets, IL-10 is secreted in vitro by many human carcinoma cell lines [101], which in some instances actually reflects its origin in vivo [102]. Well established as a broad immunosuppressive molecule, in vitro administration of IL-10 to macrophages inhibits production of proinflammatory cytokines and chemokines [103], and reduces surface expression of major histocompatability complex (MHC)II, and the costimulatory molecules CD80 and CD86 [104]. IL-10 also synergizes with IL-4 to induce Arginase-1 expression in macrophages, possibly through induction of IL-4Rα [103]. As mentioned however, the source of IL-10 in tumor microenvironments is unclear, and may even derive from TAMs themselves [96, 97, 105]. Interestingly, the ability of TAMs to produce IL-10 has been associated with another molecule produced by tumor cells, prostaglandin E2 (PGE2), suggesting that this may also regulate TAM polarization [97, 106] through EP2 and EP4 receptors [107]. The importance of PGE2 in cancer is inferred from the preventative effects of cyclooxygenase-2 (COX-2) inhibitors including aspirin in colon [108, 109] and respective transgenic mouse models [108], as well as a less prominent effect in multiple other cancers [110]. In the APCMin/+ mouse model of intestinal tumorigenesis, COX-2 inhibition reduces expression of Arginase-1 and increases that of CXCL1 [111], both changes that are also associated with repolarization of TAMs. Whether this repolarization is important for therapeutic efficacy of COX-2 inhibitors is unknown however, as PGE2 has pleiotropic effects on multiple aspects of tumor development [108].
Homeostatic imbalance
Hypoxic conditions exist within solid tumors in areas distal from functional vasculature (Figure 1). Although nutrient deprivation is the goal of anti-angiogenic therapy, the resulting hypoxia actually seems to promote malignant conversion and metastasis [112]. This response to oxygen availability is mediated primarily through hypoxia-inducible factor (HIF)-1α and HIF-2α, both of which also regulate macrophage function [113]. Using LysM-cre mice to induce myeloid-specific loss of either HIF-1α or HIF-2α, two recent reports have established that the pro-tumor functions of TAMs are likewise dependent on HIFs [8, 114]. Loss of HIF-1α limited arginase-1 expression and the suppressive capabilities of TAMs in the MMTV-PyMT model [8], while loss of HIF-2α reduced TAM recruitment through lower chemokine receptor expression in models of inflammatory hepatocellular and colon carcinoma [114]. Despite the divergent mechanisms implicated in each study, both observed reduced tumor volume and progression, suggesting either tissue specific roles for TAMs and/or involvement of overlapping pathways regulated by HIF-1α or HIF-2α. This includes the possible induction of an angiogenic response that was not thoroughly evaluated in either study, but which has been associated with TAMs localized to hypoxic regions of tumors [83, 115]
The mislocalization of cellular and extracellular components is another prominent feature of tumors due to cell death and dysregulated tissue architecture, respectively. The presence of extracellular ATP, high-mobility group box 1 protein (HMGB1), and other normally intracellular molecules is detected by a class of receptors on the surface of macrophages called Toll-like receptors (TLR) [6]. Although TLR signaling in dendritic cells is important for generating an adaptive immune response following cytotoxic therapies through TLR4 recognition of HMGB1 [116], both TLR4 and TLR2 signaling promote growth of cell lines in the lung through induced TNFα production by macrophages [117, 118]. For TLR2, this can be mediated by tumor-derived versican [118], but other ECM components including biglycan and hyaluronan also induce proinflammatory cytokine expression by macrophages via TLR2 and TLR4 [119], and are potentially important in dictating TAM polarization. Crucially, these ECM components do not bind TLRs in their native form in non-inflamed tissue, but become TLR ligands following degradation by protease cleavage or interaction with reactive oxygen or nitrogen species, thereby forming putative sensory pathways for detection of inflammation and tissue disruption.
Conclusion: Targets for therapy
The pathways that engage and mediate the maladaptive response of TAMs present attractive therapeutic targets, several of which have already shown promise in the preclinical arena, and to improve therapeutic responses to chemotherapy [37, 50, 120]. Therapeutic strategies directed at TAMs can be grouped crudely into four prospective themes: blocking effector function, limiting recruitment, reprogramming, or preventing pro-tumor polarization. Monoclonal antibodies and small molecular inhibitors targeting the VEGF and EGF pathways are already approved for treatment of various carcinomas alone or in combination, although none were designed specifically to target TAM function and their clinical efficacy has been mixed [121, 122]. It has recently been established that blocking TAM recruitment and/or survival in solids tumors (in murine models) improves efficacy of cytotoxic therapies [50, 123], in a manner dependent upon CD8+ T cells [50]. Though monoclonal antibodies against CD11b have been unsuccessful as single agents for the treatment of inflammatory disorders, antagonists targeting the CSF1-CSF1R pathway in breast cancer and the CCL2-CCR2 axis in prostate cancer are now in early phase clinical trials.
We anticipate emerging therapeutics to focus on repolarization as a method to invoke the anti-tumor potential of TAMs, as has been reported in pancreatic ductal adenocarcinoma for agonist antibodies against the costimulatory molecule CD40 [120] and the use of Tie2+ monocytes to delivery IFNα to tumors [124]. Additional approaches include synthetic TLR ligands such as CpG [125] or imiquimod [126], although reports that TLR signaling and NF-κB activation in TAMs promotes tumor growth [117, 118, 127] suggest that TLR ligands must be used in a multi-targeted approach [125]. Based upon our findings in mouse models of mammary and squamous carcinogenesis, we are currently evaluating whether blocking pro-tumor polarization of TAMs, as opposed to direct repolarization, is similarly efficacious in pre-clinical models in combination with chemotherapy. Although this may seem like a distinction without a difference, we hypothesize that this approach may be less prone to refractory responses and adverse autoimmune side effects.
Acknowledgements
The authors thank Dr. Anna Wasiuk for helpful discussion. This work was supported by a Department of Defense Breast Cancer Research Program (BCRP) Fellowship to B.R., and grants from the NIH/NCI (R01CA130980, R01CA132566, R01CA140943, R01 CA155331), the Department of Defense BCRP Era of Hope Scholar Expansion Award (W81XWH-10-BCRP-EOHS-EXP) and W81XWH-08-PRMRP-IIRA to LMC.
Footnotes
This is a PDF file of an unedited manuscript that has been accepted for publication. As a service to our customers we are providing this early version of the manuscript. The manuscript will undergo copyediting, typesetting, and review of the resulting proof before it is published in its final citable form. Please note that during the production process errors may be discovered which could affect the content, and all legal disclaimers that apply to the journal pertain.
References
Full text links
Read article at publisher's site: https://doi.org/10.1016/j.it.2011.12.001
Read article for free, from open access legal sources, via Unpaywall:
https://europepmc.org/articles/pmc3294003?pdf=render
Citations & impact
Impact metrics
Citations of article over time
Alternative metrics
Smart citations by scite.ai
Explore citation contexts and check if this article has been
supported or disputed.
https://scite.ai/reports/10.1016/j.it.2011.12.001
Article citations
Tumour Immunotherapy and Applications of Immunological Products: A Review of Literature.
J Immunol Res, 2024:8481761, 24 Oct 2024
Cited by: 0 articles | PMID: 39483536 | PMCID: PMC11527548
Review Free full text in Europe PMC
Macrophage diversity in cancer dissemination and metastasis.
Cell Mol Immunol, 21(11):1201-1214, 14 Oct 2024
Cited by: 0 articles | PMID: 39402303 | PMCID: PMC11528009
Review Free full text in Europe PMC
Tumor-Associated Macrophages as Major Immunosuppressive Cells in the Tumor Microenvironment.
Cancers (Basel), 16(19):3410, 08 Oct 2024
Cited by: 0 articles | PMID: 39410029 | PMCID: PMC11475569
Review Free full text in Europe PMC
M2 macrophage-derived lncRNA NORAD in EVs promotes NSCLC progression via miR-520g-3p/SMIM22/GALE axis.
NPJ Precis Oncol, 8(1):185, 30 Aug 2024
Cited by: 0 articles | PMID: 39215037 | PMCID: PMC11364787
Knockdown of HM13 Inhibits Metastasis, Proliferation, and M2 Macrophage Polarization of Non-small Cell Lung Cancer Cells by Suppressing the JAK2/STAT3 Signaling Pathway.
Appl Biochem Biotechnol, 29 Aug 2024
Cited by: 0 articles | PMID: 39207680
Go to all (539) article citations
Similar Articles
To arrive at the top five similar articles we use a word-weighted algorithm to compare words from the Title and Abstract of each citation.
Tumor-associated macrophages: role in cancer development and therapeutic implications.
Cell Oncol (Dordr), 42(5):591-608, 29 May 2019
Cited by: 100 articles | PMID: 31144271
Review
The roles of tumor-associated macrophages in tumor angiogenesis and metastasis.
Cell Immunol, 353:104119, 04 May 2020
Cited by: 174 articles | PMID: 32446032
Review
New Mechanisms of Tumor-Associated Macrophages on Promoting Tumor Progression: Recent Research Advances and Potential Targets for Tumor Immunotherapy.
J Immunol Res, 2016:9720912, 16 Nov 2016
Cited by: 79 articles | PMID: 27975071 | PMCID: PMC5128713
Review Free full text in Europe PMC
Targeting tumor-associated macrophages: A potential treatment for solid tumors.
J Cell Physiol, 236(5):3445-3465, 16 Nov 2020
Cited by: 35 articles | PMID: 33200401
Review
Funding
Funders who supported this work.
NCI NIH HHS (19)
Grant ID: R01 CA140943-04
Grant ID: R01CA132566
Grant ID: R01 CA130980-04
Grant ID: R01 CA132566-05
Grant ID: R01 CA140943-01
Grant ID: R01 CA140943-02
Grant ID: R01 CA155331-01
Grant ID: R01 CA130980-02
Grant ID: R01 CA130980-03
Grant ID: R01 CA155331
Grant ID: R01 CA155331-02
Grant ID: R01CA140943
Grant ID: R01 CA130980-01A1
Grant ID: R01 CA140943
Grant ID: R01 CA140943-03
Grant ID: R01CA130980
Grant ID: R01 CA132566
Grant ID: R01 CA130980
Grant ID: R01 CA130980-05