Abstract
Free full text
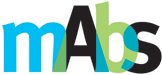
A strategy for risk mitigation of antibodies with fast clearance
Abstract
A majority of human therapeutic antibody candidates show pharmacokinetic properties suitable for clinical use, but an unexpectedly fast antibody clearance is sometimes observed that may limit the clinical utility. Pharmacokinetic data in cynomolgus monkeys collected for a panel of 52 antibodies showed broad distribution of target-independent clearance values (2.4–61.3 mL/day/kg), with 15 (29%) having clearance > 10 mL/day/kg. Alteration in the interaction with the recycling FcRn receptor did not account for the faster than expected clearance observed for the antibodies; off-target binding was presumed to account for the fast clearance. We developed an assay based on ELISA detection of non-specific binding to baculovirus particles that can identify antibodies having increased risk for fast clearance. This assay can be used during lead generation or optimization to identify antibodies with increased risk of having fast clearance in both humans and cynomolgus monkeys, and thus increase the likelihood of obtaining a suitable drug candidate.
Introduction
Human or humanized monoclonal antibodies have been broadly successful as therapeutic agents for human disease. Over 30 antibodies have received FDA-approval for treatment of a variety of disorders and hundreds more are currently in clinical development.1 In addition to the exquisite selectivity and high potency that can be achieved with an antibody therapeutic, the success of antibodies as drugs has greatly benefited from their typically long circulating half-life. Slow clearance from blood enables desired drug concentrations to be realized with infrequent dosing. Antibody drugs are usually administered via intravenous infusion or subcutaneous injection; less frequent administration improves patient compliance and clinical benefit.
Antibodies can be eliminated from systemic circulation by several mechanisms,2,3 including intracellular catabolism following fluid phase endocytosis (non-specific clearance), antigen-mediated clearance,4 and, in some cases, formation of anti-therapeutic antibodies (ATA). Antigen-mediated clearance is generally observed when antibodies target cell-bound antigens; it is most prominent at low concentrations of antibody and can usually be saturated by increasing the antibody dose. ATA have been observed to appear as early as 4–7 d after dosing, involve formation of immune complexes that can be rapidly cleared, and can sometimes be easily detected from the atypical shape of the antibody’s plasma concentration-time profile.
Antibody elimination is slowed through an FcRn-dependent recycling mechanism.5,6 As an antibody traverses the endocytic pathway, it can bind to FcRn at pH < 6. Binding to FcRn protects IgG from catabolism and promotes return to the apical cell surface where it can be rapidly released at the pH (> 7) of blood. These features result in half-lifes of 6–32 d for human or humanized antibodies in humans.7 On the basis of pharmacokinetic (PK) data with 12 IgG1 antibodies, a mean value for clearance in humans of 3.9 ± 1.2 (s.d.) mL/day/kg with range of ~2–6 mL/day/kg was determined.8 Similarly, studies of these antibodies in cynomolgus monkeys yielded a mean clearance of 6.5 ± 2.9 (s.d.) mL/day/kg. Engineering of the antibody Fc region to increase binding to FcRn at pH 6.0 can increase half-life of potential therapeutic antibodies in cynomolgus monkeys and in mice.9-12
Preclinical testing of a potential therapeutic antibody in a relevant non-human species is important to gain understanding of the expected dosing regimen in humans, and to assess potential toxicities. Given the high target antigen sequence homology between human and non-human primates (NHP), and similar binding affinities for the recycling FcRn receptor,9 cynomolgus monkey is the preferred species for preclinical PK studies. Previously we have shown that the non-specific clearance of therapeutic IgG1 antibodies determined in humans is commonly about half that measured in cynomolgus monkeys.8
One potential mechanism that can contribute to faster than expected clearance is off-target binding.13-16 Although highly specific off-target binding can sometimes be identified and eliminated,14 off-target binding is often of unknown origin and difficult to saturate with an increase in dose. In vitro systems to evaluate and predict the in vivo absorption, distribution, metabolism, and elimination mechanisms or the in vivo PK behavior of antibodies are not yet established. We sought to develop in vitro assays of non-specific binding that would be useful for identifying antibodies likely to show fast clearance in vivo. Assays of non-specific binding based on binding to glass surfaces or elution times on a gel filtration column did not show an association with fast clearance (Figs. S1 and S2). Here, we describe an assay based on ELISA detection of binding to baculovirus (BV) particles that is useful to evaluate the non-specific binding of therapeutic proteins. Using a large panel of antibodies, we demonstrate that the assay readout is associated with the non-specific clearance measured in non-human primate (n = 45) and human (n = 16), and can be employed to mitigate the risk of fast clearance for potential therapeutic antibodies.
Results
Predictive scaling of human clearance from clearance in monkeys
Using a slightly larger data set than previously available,8 we confirmed a strong correlation between the clearance values measured in cynomolgus monkeys and humans [Fig. 1, Spearman’s correlation coefficient (ρ) = 0.74]. A simple scaling guideline is obtained in that the clearance measured in humans is about two-fold slower than the clearance measured in cynomolgus monkeys, with the anti-NRP1 antibody as an outlier.
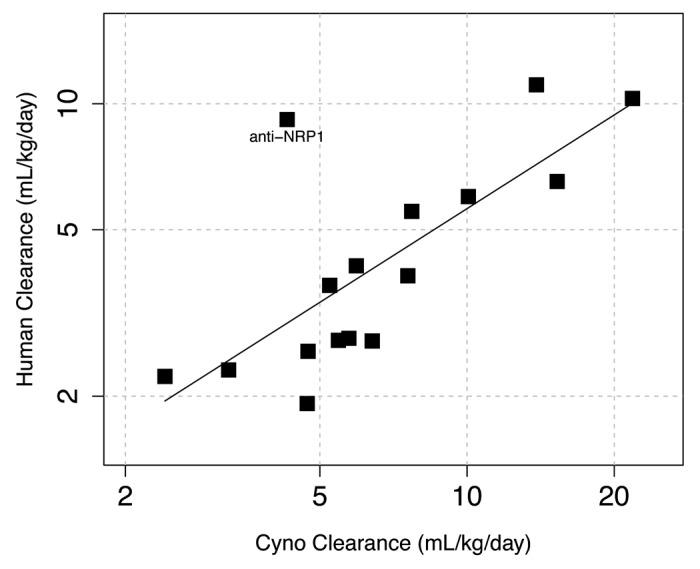
Figure 1. Correlation of antibody clearance values measured in humans and cynomolgus monkeys (ρ = 0.74, n = 16). For our analyses, antibody doses were chosen that were believed to saturate any target-dependent clearance. The solid line is a linear regression fit of the logarithm of human clearance to the logarithm of cynomolgus monkey clearance [log(Human clearance) = 0.0123 + 0.744 * log(Cyno clearance)]. For most antibodies shown, human clearance is about two-fold slower than the corresponding cynomolgus monkey clearance. By contrast, for anti-NRP1 the clearance in human (9.2 mL/day/kg) is ~2-fold faster than the clearance in cynomolgus monkey (4.3 mL/day/kg).
Characterization of structural variants and clearance in cynomolgus monkeys for a panel of therapeutic antibodies
In the normal course of preclinical development, PK data in cynomolgus monkeys were collected for 52 humanized and human antibodies. The predominant isotype of these antibodies was IgG1, κ (n = 46). The antibody panel also included 4 IgG1λ and 2 IgG4κ. The IgG4 antibodies incorporate the S228P hinge-stabilizing substitution.17 Six of the 52 antibodies are aglycosylated to reduce effector function, one is afucosylated to increase ADCC activity, and three have Fc amino acid substitutions to ablate or increase FcγR binding. The variable domains of these antibodies are either humanized (n = 32), derived from synthetic human antibody phage libraries18 (n = 16), human (n = 3), or mouse (n = 1; rituximab). Twenty-six of the antibodies recognize membrane associated targets with the remainder directed against soluble antigens. These antibodies showed a wide range of target-independent clearance values (Fig. 2), with 15 (29%) antibodies having clearance faster than 10 mL/day/kg.
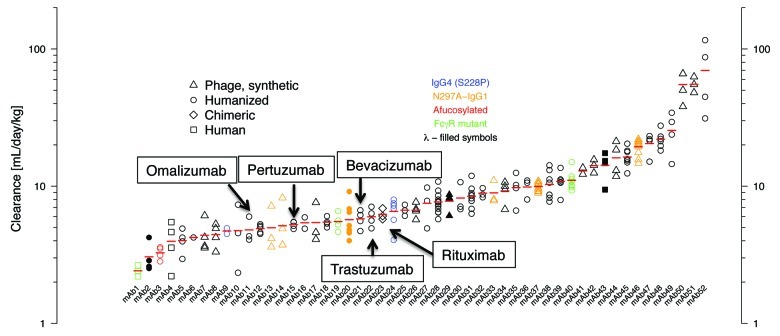
Figure 2. Clearance values of antibodies (n = 52) in cynomolgus monkeys. Individual animal data and geometric mean values (red bars) are shown from dose groups where contribution of any specific clearance to the reported clearance was assumed to be negligible. Humanized (circle), human (square), synthetic human phage derived (triangle), and chimeric (diamond) antibodies are shown. Filled symbols indicate antibodies with a λ light chain (mAbs 2, 20, 29, 43), all others have a κ light chain. IgG4 isotype antibodies having the hinge-stabilizing mutant S228P are colored blue (mAbs 9, 24), all others are IgG1 isotype. Red symbol indicates an afucosylated antibody (mAb 3). Aglycosylated antibodies obtained via replacement of Asn297 with Ala are colored orange (mAbs 13, 14, 20, 33, 37, 46). Antibodies with Fc amino acid substitutions to modulate FcγR binding are colored green (mAbs 1, 19, 40).
The hypothesis that fast clearance is associated with the synthetic library antibodies was investigated. Antibodies derived from synthetic human antibody phage libraries were selected and optimized through in vitro selection.18 Most of these required affinity maturation through amino acid changes in their complementarity–determining regions (CDRs) to achieve the required affinity and potency. Although many of the humanized antibodies also have CDR substitutions relative to the rodent sequence either to improve affinity or chemical stability, in general the humanized antibodies were subjected to less in vitro optimization. A concern with in vitro selection is that it may inadvertently result in off-target interactions since the negative selection that can be performed in vitro is less extensive than can occur in vivo.13 Nonetheless, a comparison of humanized and synthetic library-derived human antibodies indicates an overlapping range of clearance values (Fig. 3). The median clearance value is 6.5 mL/day/kg for humanized antibodies and 9.0 mL/day/kg for human antibodies from synthetic phage libraries. These data do not provide evidence for an association between the synthetic library antibodies and fast clearance (p = 0.28, Wilcoxon test).
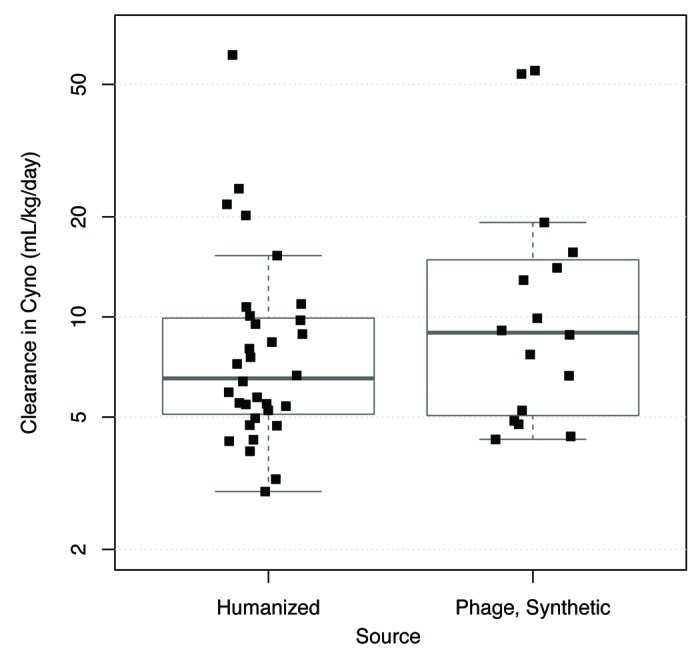
Figure 3. Comparison of clearance values in cynomolgus monkeys of humanized and synthetic human antibodies derived from phage display libraries. Chimeric antibodies (n = 1) and human antibodies from non-phage sources (n = 3) are not shown because of the very small size of the data sets. Plots show individual data values overlaid by boxplots. Boxplot rectangle shows the interquartile range (IQR) between the first quartile (25th percentile) and the third quartile (75th percentile); the thicker horizontal line within the boxplot rectangle is at the median (50th percentile). The lower boxplot whisker extends to the lowest data value that is still within 1.5 IQR of the lower quartile, and the upper whisker extends to the highest data value within 1.5 IQR of the upper quartile.
Binding affinity to cynomolgus monkey FcRn
To test whether variations in FcRn binding could account for the range of clearance values observed, we used surface plasmon resonance (SPR) methods to determine KD values for binding of these antibodies to purified cynomolgus monkey FcRn at pH 5.8. Since IgG-FcRn binding is a relatively weak interaction, we used a steady-state approach described previously11 to determine binding constants. Avidity effects arising from 2:1 FcRn:IgG interaction were avoided by immobilizing each of the antibodies (n = 44) that were available for analysis. KD values determined in this fashion ranged from about 250 nM to 1,500 nM. Such a range was not unexpected because each antibody was separately immobilized using random coupling via amino groups, with a concomitant uncertainty in the orientation on the sensor chip surface. Multiple (n = 7) determinations of the KD for pertuzumab indicated a mean of 940 ± 140 (s.d.) nM. The measurement method can detect changes in FcRn affinity because we could reproduce binding effects for Fc mutants known to increase affinity for FcRn.19
The faster clearance observed for some antibodies was not due to an altered low pH binding affinity for cynomolgus monkey FcRn (Fig. 4). Indeed, a comparison of several antibodies with approximately identical FcRn binding affinities gave a nearly 30-fold range in clearance values. Separate experiments (Fig. S3) indicated that the rate of dissociation of cynomolgus monkey FcRn from these antibodies at pH 7.4 was too fast for accurate measurement by Biacore technology. Although these experiments are insufficient to rule out a change in the recycling pathway for some antibodies as an explanation for the fast clearance observed, it appears unlikely that a significant change in binding affinity at pH 5.8 or in release kinetics at neutral pH played a major role in the fast clearance.
Development of in vitro assay for risk mitigation of fast clearance
Fast clearance observed for a few antibodies has been associated with specific14,15 or non-specific13,16 off-target binding. During antibody generation and optimization, most of the antibodies in our panel were screened for lack of binding to closely related antigen homologs. In addition, many of the antibodies derived via phage display were selected in the presence of additives to prevent enrichment for non-specific binding.20 As noted by Wu et al.,13 screening by ELISA with a limited variety of antigens may not detect the kind of specific or non-specific off-target binding that may influence in vivo behavior. Therefore, we sought to develop an in vitro assay useful for identifying candidate antibodies likely to show fast clearance. In the course of experiments using intact BV particles bearing specific membrane bound targets as antigen in ELISA assays,21 we noted that some antibodies bound to BV particles that did not express the target. Many of these antibodies were also shown to bind non-specifically to non-expressing human 293 cells by FACS (Fig. S4). However, expression of some cognate antigens on 293 cells made it challenging to distinguish specific from non-specific binding.
An initial screen against a test set of 15 antibodies selected from the panel used to generate data shown in Figure 2 suggested that fast clearing antibodies bound non-specifically in the BV ELISA. Subsequently, a larger group of 45 antibodies was tested in the assay. While there is considerable variability, a higher score in the in vitro assay is associated with faster clearance in cynomolgus monkey (Fig. 5). Thresholds of > 10 mL/day/kg for “fast” clearance and > 5 for normalized BV score were useful for quantifying these data. A clearance value of 10 mL/day/kg is more than 1 standard deviation from the mean value (6.5 ± 2.9) determined for 12 human IgG1 antibodies in monkeys.8 With these thresholds, 9 antibodies were correctly identified (“true positives”) as having fast clearance in monkeys, with only 3 “false positives”—antibodies with clearance values < 10 mL/day/kg that showed a normalized BV score > 5. Four antibodies were “false negatives,” having clearance greater than 10 mL/day/kg and a BV score < 5. The remaining 29 antibodies were correctly identified as “true negatives.”

Figure 5. Association of antibody clearance value in (A) cynomolgus monkey and (B) human with normalized BV ELISA score. A BV ELISA score > 5 is associated with increased risk of fast clearance in cynomolgus monkey (ρ = 0.53, n = 45). The BV score was calculated from the mean of 6 determinations; each determination was normalized by dividing by the signal observed for non-coated wells on the same assay plate. Of antibodies with a BV score < 5, 12% have clearance > 10 mL/day/kg in cynomolgus monkey, while clearance exceeds 10 mL/day/kg for 75% of antibodies with BV score > 5. A maximum likelihood estimate for the odds ratio is 19.5 [Fisher’s Exact Test, 95% Confidence Interval (3.3, 165.7)]. The confidence interval suggests a 3.3 to 166-fold increase in the odds of faster clearance for BV > 5; that the interval does not contain 1 implies statistical significance. (B) BV ELISA score > 5 is associated with increased risk of fast clearance in human (ρ = 0.83, n = 16).
Although the data set (n = 16) was smaller, an increased risk of fast clearance in humans was also associated with high BV score (Fig. 5B; Spearman’s ρ = 0.83). Five antibodies with clearance faster than 5 mL/day/kg in humans were correctly identified in the assay. Notably, one antibody, the anti-NRP1 antibody that had clearance < 10 mL/day/kg in cynomolgus monkeys was detected as a true positive for human clearance in the BV ELISA. One antibody having clearance > 5 mL/day/kg in humans, and > 10 mL/day/kg in cynomolgus monkeys, was not identified in the assay. The remaining 10 antibodies were correctly classified as having slow clearance in humans.
We tested whether the BV ELISA could be used prospectively to help aid candidate selection through further engineering of antibody 47. This is a humanized antibody with amino acid changes in the CDRs both for affinity improvement and to remove potential sequence liabilities. Cynomolgus monkey is a non-target-binding species for antibody 47. No reproducible tissue staining was observed in monkeys with antibody 47, but a clearance of 20.2 mL/day/kg in cynomolgus monkeys was determined for this antibody. A variant of antibody 47 that retained 2 of the affinity maturation changes, and an amino acid change to remove a potential N-linked glycosylation site in one of the heavy chain CDRs (antibody 47b), showed reduced binding in the BV ELISA (Table 1). Antibody 47c retains only the amino acid change to remove the potential N-linked glycosylation site and also had reduced BV binding. Separate experiments indicated that a large contribution to the BV binding of antibody 47 was made by a single amino acid change to remove a potential Asp-Gly isomerization site. Antibodies 47b and 47c had slower clearance relative to antibody 47 in cynomolgus monkeys (Table 1).
Variant | Number and purpose of CDR amino acid substitutions | Normalized BV ELISA Score | Clearance1 (mL/day/kg) |
---|---|---|---|
antibody 47 | 3-affinity maturation 2-chemical stability 1-removal of potential N-linked glycosylation | 8.6 | 20.4 ± 3.6 (n = 4) |
antibody 47b2 | 2-affinity maturation 1-removal of potential N-linked glycosylation site | 3.1 | 9.3, 8.2 (n = 2) |
antibody 47c | 1-removal of potential N-linked glycosylation site | 2.0 | 4.2 ± 1.4 (n = 4) |
1Pharmacokinetic data were collected for a single IV dose of 5 mg/kg, mean (± s.d.) values for clearance are shown. Serum concentrations of antibody were determined using ELISA with antigen coat. The non-specific clearance was calculated from the serum concentration data to day 42 by using a two-compartment model. 2Antibody 47b: 2 of 4 animals had evidence of ATA and data from these animals were not used in the calculation of clearance.
Discussion
The broad distribution of clearance values in cynomolgus monkeys measured for our panel of 52 antibodies, as well as the faster clearance (> 10 mL/day/kg) observed for 15 of the antibodies, was unexpected. Fast clearance likely involves internalization followed by catabolism, but we have not performed experiments to address this question. Since 40 out of the 52 antibodies have identical human IgG1 Fc sequences, it was expected that faster clearance would not be associated with changes in FcRn interaction. Indeed, although the KD measured for FcRn binding at pH 5.8 varied over a seven-fold range, this was not associated with a trend in clearance values. Given that relatively large increases in pH 5.8 FcRn affinity lead to modest improvement in clearance,19 it is not surprising that the small differences in FcRn affinity determined here did not have an effect on clearance. All of the antibodies tested showed equivalent and rapid release from FcRn at neutral pH. In contrast to the results reported by Wang et al.,22 we did not detect an association between clearance and an affect of the Fab portion of the antibody on FcRn release kinetics at neutral pH. Our study used a larger panel of antibodies and a different orientation of the SPR experiments to minimize avidity effects on binding.
Given that the greatest difference between antibodies in our panel is in the variable domains, and in particular in the CDRs, it is likely that differences in the composition of these domains contributed to the variability in clearance behavior. Other groups have observed effects on clearance associated with changes in CDR sequence that were not related to differences in binding affinity to the target antigen.13,16 We did not find that clearance was associated with isoelectric point (pI) or hydrophobicity of the intact antibody (Fig. 6A and B). Igawa et al.23 have previously shown that clearance of a human IgG1 antibody in cynomolgus monkeys can be reduced through changes in the variable domain that result in a lower pI. This effect was observed at a dose where significant antigen-dependent clearance was operating. The mechanism was presumed to be non-FcRn dependent. For our large panel of antibodies, PK data was collected at doses where half-life is FcRn-dependent, and we found that clearance rates were not dependent on pI. A caveat to this conclusion is that the pI values of a majority of antibodies in this analysis are clustered in a relatively narrow range (7.5–9.5); it is possible that a trend would be observed if a wider range of pI was tested. In addition, we find that clearance is not correlated with total charge calculated for the antibody Fv (Fig. 6C). Although the absolute value of calculated and experimentally determined charge may differ,24 the relative charge ranking of antibodies by experiment and calculation is similar,25 suggesting that calculated charge is sufficient for this comparison. Thus, the physiochemical properties of the antibody that result in fast clearance are not easily recognized from sequence comparisons alone and may require more detailed structure-function analysis.
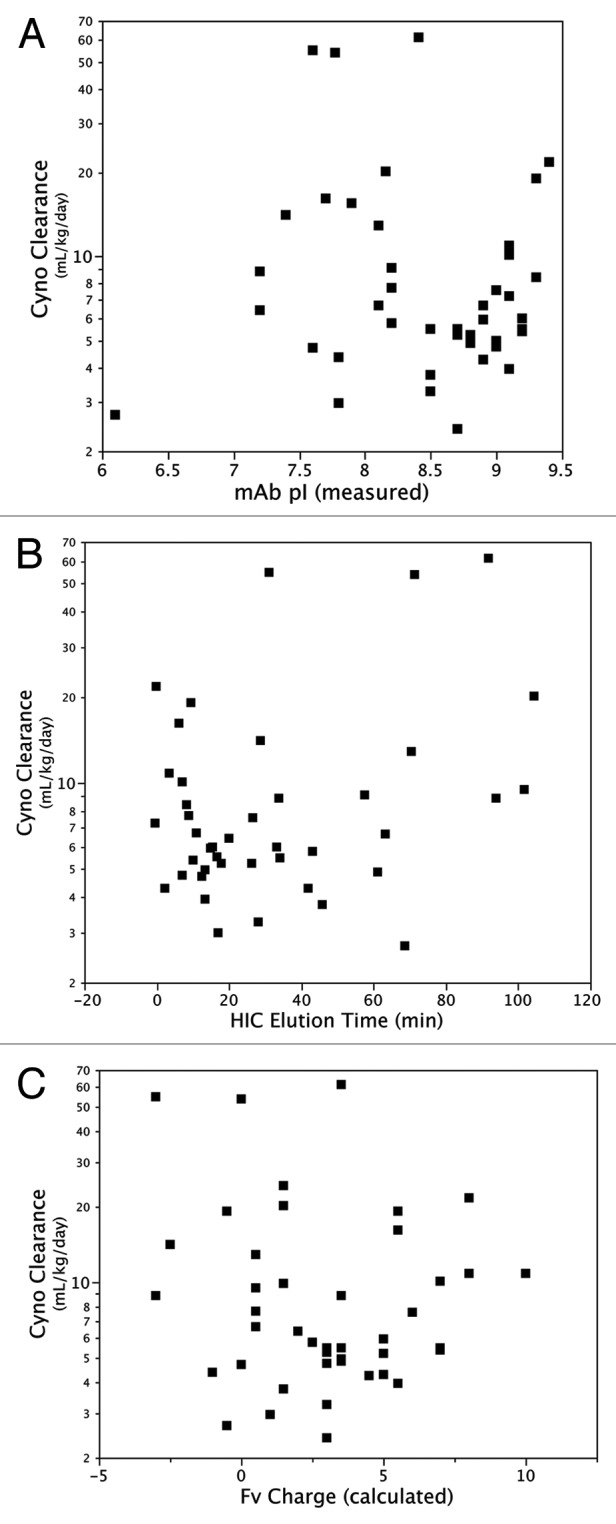
Figure 6. Relationship between antibody clearance in cynomolgus monkeys and antibody (A) pI, (B) hydrophobicity as measured by HIC, and (C) charge calculated for Fv domain.
We show here that a simple assay of non-specific binding can be used to identify antibodies with increased risk of having fast clearance in both humans and cynomolgus monkeys. Preliminary results indicate a similar correlation with clearance measured in rodents; however, the data set of clearance values in rodents is smaller, precluding a robust statistical analysis of the data. This assay, based on antibody binding to virus particles prepared from BV-infected insect cells, employs a simple ELISA format and can be applied in high throughput fashion. Because PK data are determined in mammalian species, an assay based on non-specific binding to mammalian cells might have been expected to show a stronger correlation with fast clearance. Preliminary results indicated a correlation between fast clearance and non-specific binding to human 293 cells detected by FACS, but broad application of this assay was precluded because many of the cognate antigens for this collection of antibodies are expressed on 293 cells. The BV ELISA assay we developed does not suffer from this target-expression limitation. For antibodies that can be evaluated with both assays, a similar trend in non-specific binding was observed (Fig. S4). Budded BV virions are stable nanoparticles that mimic infected cell surfaces, presenting a complex mixture of phospholipid, carbohydrate, glycoproteins, extracellular matrix and nucleic acids, as well as the viral capsid.26,27 The antigen presumably also contains some insect cell debris, including protein, membranes and nucleic acids. Such a mixture that lacks the specific targets of therapeutic antibodies while retaining the same overall biochemical complexity as human cells and tissues has advantages in a non-specific binding assay because it allows detection of different kinds of interactions, e.g., electrostatic, hydrophobic, with different antibodies.
An important caveat to the association between antibody clearance in cynomolgus monkeys and binding to BV particles is that, other than for antibody 47, the observation is based on data collected through normal preclinical development, rather than a designed experiment in which BV-particle binding was systematically varied. Clearance values were obtained from separate studies with widely-varying dose levels and using varying PK assay types; however, where sufficient data was available to make an assessment, the association between fast clearance in cynomolgus monkeys and BV binding was found to persist across dose levels, assay types and antibody sources and target types.
The risk of fast clearance can be reduced by using our assay to minimize introduction of non-specific binding during antibody engineering. In antibody 47, both charge reduction and increased hydrophobicity were associated with increased non-specific binding to BV particles. Variants with decreased non-specific binding had slower clearance. The BV ELISA, however, is not expected to be a reliable assay of specific off-target effects. For example, the humanized antibody previously shown to have fast clearance in mice due to unintended specific binding to a complement protein14 had no detectable binding to the BV particles. In our experience, specific off-target effects are less prevalent than antibodies with broader off-target binding. Moreover, specific off-target effects can usually be identified through mechanistic studies of fast clearance.
The BV particle ELISA detects most, but not all, antibodies with rapid clearance in cynomolgus monkeys, with few false positives. Therefore, the assay should be considered as a screening tool to reduce the number of therapeutic antibodies that need to be tested in NHP PK experiments and not as an assay that consistently predicts clearance in cynomolgus monkeys. When multiple candidates with similar potencies against a target are available, such that a small but non-zero rate of false positives is acceptable, this assay is a cost effective tool to minimize risk of fast clearance. The BV ELISA assay could be enhanced if the basis for the false-negative results could be identified. Relative to membranes from mammalian cells, the phospholipid composition of insect cell membranes is enriched in phosphatidyl inositol, phosphatidyl choline, and phosphatidyl ethanolamine, with lower levels of the acidic phospholipid phosphatidyl serine, cholesterol, glyco- and sphingolipids.26 The higher amounts of neutral phospholipids may account for the false negatives in the assay if the faster clearance of these antibodies is related to binding to acidic phospholipids on mammalian membranes. Two of the four false negative antibodies have a high net positive charge calculated for the Fv domain. It will be interesting to explore whether the phospholipid composition of the BV particles can be manipulated through growth conditions or cell line differences with a concomitant improvement in the veracity of the non-specific binding assay. These studies could further illuminate features to be avoided during antibody engineering to maintain acceptable PK behavior.
Materials and Methods
Determination of clearance in cynomolgus monkeys
Antibody PK data were determined following intravenous administration of a single dose or multiple doses of the therapeutic antibody in cynomolgus monkeys. Serum samples were prepared from blood collected at various time points and antibody concentration was determined by ELISA. In most cases, the ELISA consisted of capture with coated antigen followed by detection with anti-human Fc antibody. ELISA assay of antibody 26 employed an anti-idiotype antibody for capture. Antibodies 14, 17, 24, 33 and 44 used an anti-human Fc sandwich ELISA for determination of serum concentration. Serum concentration vs. time profiles were analyzed using non-compartmental analysis to calculate the total clearance.28 For most of the mAbs, the relationship of total clearance vs. dose was explored to obtain evidence of saturation of target-mediated clearance suggested by clearance estimates independent of dose escalation. The clearance at the highest dose tested for each antibody was reported as the non-specific clearance, and it was assumed that the contribution of specific clearance to the total clearance was negligible.
Determination of FcRn affinity
Equilibrium dissociation constants (KD) for binding of cynomolgus monkey FcRn to immobilized antibody were determined from surface plasmon resonance (SPR) measurements on a Biacore® 4000 instrument (GE Healthcare).29 Cynomolgus monkey FcRn was prepared as described previously.11 A series S sensor chip was docked, normalized and prepared for hydrodynamic addressing using a protocol supplied by the manufacturer. All 5 spots of each of 4 flow cells were activated for amine coupling through 10 min exposure to EDC/NHS solution. Antibody was coupled to spots 1, 2, 4 and 5 through 7 min exposure to a solution containing 2 µg/mL antibody and 10 mM NaOAc, pH 5. Unreacted sites were then blocked through 7 min exposure of all 5 spots to 1 M ethanolamine. In this format spot 3 is the reference cell, 4 different antibodies are coupled per flow cell, with a total of 16 per sensor chip. Coupling densities were in the range of 200–1000 RU of antibody. Separate experiments indicated that calculated KD did not vary with coupling density in this range. A series of solutions of cynomolgus monkey FcRn varied in concentration from 10 µM to 0.04 µM in two-fold increments were prepared in a running buffer containing 25 mM MES, 25 mM HEPES, 150 mM NaCl, 0.05% Polysorbate-20, pH 5.8. Sensorgrams were collected for injection of 60 µL of these solutions, and a buffer only control, over the sensor chip at a flow rate of 30 µL/min. The temperature of measurement was 25°C, dissociation was monitored for 180 sec and then any FcRn remaining bound was eluted by injection of 30 µL of a solution containing 50 mM TRIS-HCl, 150 mM NaCl, 0.05% Polysorbate-20, pH 8. Dissociation constants were calculated from Steady State Affinity Analysis using Biacore 4000 Evaluation Software 1.0.
Production of antigen for ELISA
BV particles were obtained by infecting 1.8 × 109 Sf9 insect cells in 600 ml of serum-free ESF media (Expression Systems) with a recombinant Autographa californica nucleopolyhedrovirus expressing green fluorescent protein (Bac-to-Bac, Invitrogen) at a multiplicity of infection of about 1. Infected cultures were incubated for 40 h at 27°C under agitation (200 rpm), harvested and cells removed by centrifugation at 5,000 × g for 10 min. The virus in the supernatant was pelleted by centrifugation at 25,000 × g for 4 h at 4°C, resuspended in PBS buffer (150 mM NaCl, 10 mM sodium phosphate, pH 7.4), layered on a 4 mL 35% (w/v) sucrose cushion in PBS and centrifuged in an SW40Ti rotor (Beckman) at 30,000 rpm for 1 h at 4°C. The supernatant with debris was discarded, the virus pellet gently rinsed once with PBS, resuspended in 1.2 mL of PBS with protease inhibitor cocktail (Roche) and stored at 4°C for up to 4 mo.
Crude Sf9 cell membranes fractions were obtained from uninfected cells. A total of 2 × 108 uninfected Sf9 cells grown in serum-free ESF media were washed once in PBS and resuspended in 4 mL of ice-cold lysis buffer (1 mM EDTA, 50 mM HEPES buffer, pH 7.4, complete protease inhibitor cocktail). The cells were transferred to a Dounce homogenizer and broken up with 8 strokes of a loose-fitting pestle. An additional 4 ml of lysis buffer containing 0.5 M sucrose were added to the lysed cells and cells were further broken up with 8 strokes of a tight-fitting pestle. The lysate was centrifuged for 10 min at 500 × g at 4°C to remove mitochondria, nuclei and coarse debris. The supernatant was transferred to a new tube and centrifuged at 25,000 × g for 20 min at 4°C. The pellet was rinsed once with lysis buffer and resuspended in 5 ml of the same buffer using a Dounce homogenizer with a tight-fitting pestle. Large debris were removed by centrifugation at 500 × g at 4°C.
ELISA assay
Antigen was immobilized in 384-well ELISA plates (Nunc Maxisorp) by adding 25 µL of a 1% BV suspension in 50 mM sodium carbonate buffer pH 9.6 to each well, allowing the particles to adsorb to the plates overnight at 4°C. The wells were blocked with 50 µl of blocking buffer (PBS containing 0.5% BSA) for 1 h at room temperature. After rinsing the plates three times with PBS, purified antibodies were serially diluted in blocking buffer, 25 µl were added in duplicate to the ELISA wells and incubated for 1 h at room temperature. Plates were washed 6 times with PBS and 25 µL of 10ng/mL goat anti-human IgG, (Fcγ fragment specific) conjugated to horseradish peroxidase (Jackson ImmunoResearch) were added to each well. The plates were incubated for 1 h at room temperature, washed 6 times in PBS and 25 µl of TMB substrate added to each well. Reactions were stopped after 15 min by adding 25 µl of 1 M phosphoric acid to each well and absorbances were read at 450 nm, referenced at 620nm. BV score was calculated from the mean of 6 optical density determinations each of which had been normalized by dividing by the average signal observed for non-coated wells. For assays with Sf9 cell membranes, a 2% suspension of the crude membranes was used to coat ELISA plates instead of BV particles. Detergents were not added to buffers in any step.
FACS analysis
HEK293 cells were resuspended in DMEM-10% fetal bovine serum at 5 × 106 cells/mL and dispensed in U-bottom 96-well plates at 100 µL/well. An equal volume of IgG (30 to 50 nM final concentration) diluted in PBS (150 mM NaCl, 10 mM sodium phosphate pH7.4) was added to the cells and incubated for 1 h a 4°C. Cells were then washed 3 times with ice-cold PBS, incubated with an anti-human IgG Fc-R-phycoerythrin conjugate (Jackson ImmunoResearch) or an anti-human IgG-Alexa488 (Molecular Probes) conjugate for 30 min at 4°C, washed twice in ice-cold PBS and fixed in PBS with 0.1% paraformaldehyde. Cells were analyzed in a FACSCalibur flow cytometer (BD Biosciences) with a high-throughput sampler.
Statistical analysis
All statistical analyses were done using R [R Development Core Team (2011). R: A language and environment for statistical computing. R Foundation for Statistical Computing, Vienna, Austria. ISBN 3-900051-07-0, www.R-project.org]. Assessment of the performance of the BV score in correctly identifying fast- and slow-clearing antibodies using the odds ratio as described in the legend to Figure 5 is optimistic because it was made using the same data set used to determine the binning threshold of BV = 5. A more conservative assessment of the in vitro assay’s utility was obtained for the clearance threshold of 10 mL/day/kg using five-fold cross-validation30,31 and regression modeling to determine the BV score threshold; this approach gave a more modest odds ratio estimate of 14.1 [95% Confidence Interval (2.4,113.3)].
Acknowledgments
We thank Tim Spraikes, Brian Hosken, and Boyan Zhang for supplying HIC data, and Will McElroy, David Michels for antibody pI values. Kapil Gadkar helped with figure preparation. Gerald Nakamura, Mary Mathieu, Justin Scheer, Mike Elliott, Sree Ramani, Lino Gonzalez, and Sharookh Kapadia provided useful discussion and other characterization data on the panel of antibodies.
Disclosure of Potential Conflicts of Interest
All authors are current or former employees of Genentech, a member of the Roche group, and hold a financial interest in Roche.
References
Articles from mAbs are provided here courtesy of Taylor & Francis
Full text links
Read article at publisher's site: https://doi.org/10.4161/mabs.22189
Read article for free, from open access legal sources, via Unpaywall:
https://www.tandfonline.com/doi/pdf/10.4161/mabs.22189?needAccess=true
Citations & impact
Impact metrics
Article citations
A bispecific CD40 agonistic antibody allowing for antibody-peptide conjugate formation to enable cancer-specific peptide delivery, resulting in improved T proliferation and anti-tumor immunity in mice.
Nat Commun, 15(1):9542, 05 Nov 2024
Cited by: 0 articles | PMID: 39500897 | PMCID: PMC11538452
Human antibody polyreactivity is governed primarily by the heavy-chain complementarity-determining regions.
Cell Rep, 43(10):114801, 10 Oct 2024
Cited by: 0 articles | PMID: 39392756 | PMCID: PMC11564698
Rapid affinity optimization of an anti-TREM2 clinical lead antibody by cross-lineage immune repertoire mining.
Nat Commun, 15(1):8382, 27 Sep 2024
Cited by: 1 article | PMID: 39333507 | PMCID: PMC11437124
Translational PK/PD and the first-in-human dose selection of a PD1/IL15: an engineered recombinant targeted cytokine for cancer immunotherapy.
Front Pharmacol, 15:1380000, 03 Jun 2024
Cited by: 0 articles | PMID: 38887559 | PMCID: PMC11181026
Protein language models enable prediction of polyreactivity of monospecific, bispecific, and heavy-chain-only antibodies.
Antib Ther, 7(3):199-208, 30 May 2024
Cited by: 0 articles | PMID: 39036071 | PMCID: PMC11259759
Go to all (156) article citations
Data
Data behind the article
This data has been text mined from the article, or deposited into data resources.
BioStudies: supplemental material and supporting data
Similar Articles
To arrive at the top five similar articles we use a word-weighted algorithm to compare words from the Title and Abstract of each citation.
Framework selection can influence pharmacokinetics of a humanized therapeutic antibody through differences in molecule charge.
MAbs, 6(5):1255-1264, 30 Oct 2014
Cited by: 78 articles | PMID: 25517310 | PMCID: PMC4623330
The interplay of non-specific binding, target-mediated clearance and FcRn interactions on the pharmacokinetics of humanized antibodies.
MAbs, 7(6):1084-1093, 04 Sep 2015
Cited by: 51 articles | PMID: 26337808 | PMCID: PMC4966429
pH-dependent binding engineering reveals an FcRn affinity threshold that governs IgG recycling.
J Biol Chem, 290(7):4282-4290, 23 Dec 2014
Cited by: 57 articles | PMID: 25538249 | PMCID: PMC4326836
Pharmacokinetic de-risking tools for selection of monoclonal antibody lead candidates.
MAbs, 9(5):756-766, 02 May 2017
Cited by: 23 articles | PMID: 28463063 | PMCID: PMC5524161
Review Free full text in Europe PMC