Abstract
Free full text

In vivo imaging using polymeric nanoparticles stained with near-infrared chemiluminescent and fluorescent squaraine catenane endoperoxide†
Associated Data
Abstract
Polystyrene nanoparticles stained with squaraine catenane endoperoxide exhibit remarkably high chemiluminescence and enable optical imaging of biodistribution in living mice. Whole-body chemiluminescence imaging was much more effective than fluorescence at identifying lung accumulation of the nanoparticles.
The widespread introduction of relatively cheap, technically-straightforward, optical imaging stations has made it possible to incorporate small animal optical imaging into mainstream biomedical research and drug discovery programs.1 Planar optical imaging of small animals is amenable to longitudinal and high throughput studies because images can be recorded rapidly, using harmless near-infrared light, and multiple animals can be imaged simultaneously.2 Whole-body images can be acquired in either; (a) fluorescence mode, which typically involves illumination of the entire living animal to achieve excitation of targeted or activated fluorescent probes, or (b) chemiluminescence mode, which usually employs genetically modified cells expressing luciferase enzymes that consume chemical fuel and produce light.3 A common drawback with both optical modalities is limited penetration of the light through skin and tissue, a problem that is minimized by employing wavelengths in the window of 650–900 nm. We are pursuing a new optical molecular imaging method using small polymeric particles that incorporate synthetic dyes that we call CLF dyes because they are simultaneously chemiluminescent and fluorescent. Unlike, inorganic nanoparticles with persistent luminescence,4 the chemiluminescence with CLF dyes is thermally-activated (that is, no chemical or electrical stimulus is needed) which means that the dyes can be stored at low temperature and they only become self-illuminating when warmed to body temperature.
This current study compares two types of related CLF dyes; recently described Squaraine Rotaxane Endoperoxide (SREP),5 and previously unreported Squaraine Catenane Endoperoxide (SCEP) (Fig. 1). They are interlocked molecules with two components, a macrocyclic endoperoxide that is the primary energy source for the chemiluminescence, and an encapsulated fluorescent squaraine chromophore. We have previously shown that SREPs undergo a thermally-activated cycloreversion reaction that releases excited-state singlet oxygen and emits 733 nm light.6 The chemiluminescence apparently comes from the encapsulated squaraine chromophore after energy transfer from the released singlet oxygen.7 The flux of singlet oxygen that is produced by this thermal process is quite low and SREPs are not toxic to cells.8 A preliminary, highly controlled study of SREP-stained microparticles simply injected at different depths into mouse leg muscle (the microparticles did not enter the animal’s systemic circulation and were imaged immediately after injection) indicated that target signal contrast with fluorescence imaging was much more “surface weighted” than chemiluminescence imaging. With superficial target sites (<2 mm deep), fluorescence imaging produced the highest Target-to-Background Ratio (TBR). But TBR for fluorescence imaging quickly dropped towards unity with increasing tissue depth due to increased contribution of the background signal.9 With chemiluminescence imaging, the attenuation of TBR with tissue depth was more linear and gradual, such that chemiluminescence imaging produced the highest TBR in deeper target sites. These results suggest a new paradigm for optical molecular imaging using nanoparticle probes containing these CLF dyes. First, high contrast chemiluminescence imaging is employed to locate the probe in vivo at relatively deep target sites and then there is a switch to fluorescent mode for subsequent microscopic studies of thin biopsy or histopathology sections taken from the exact same specimen. A necessary requirement for further development of this imaging paradigm is the production of nanoparticle probes with very high chemiluminescence intensity. Here, we report that SCEP-stained carboxylate-functionalized polystyrene nanoparticles exhibit substantially more chemiluminescence intensity than analogous SREP-nanoparticles. We utilize this crucial technical breakthrough to directly compare the capabilities of whole-body fluorescence and chemiluminescence imaging to track systemic biodistribution of polymeric nanoparticles in a living mouse after intravenous injection. The work has relevance in nanomedicine since small polymeric particles are used clinically as embolization agents,10 and they are under active preclinical investigation as pulmonary drug delivery vehicles,11 in vivo imaging probes,12 and adjuvants for vaccination.13
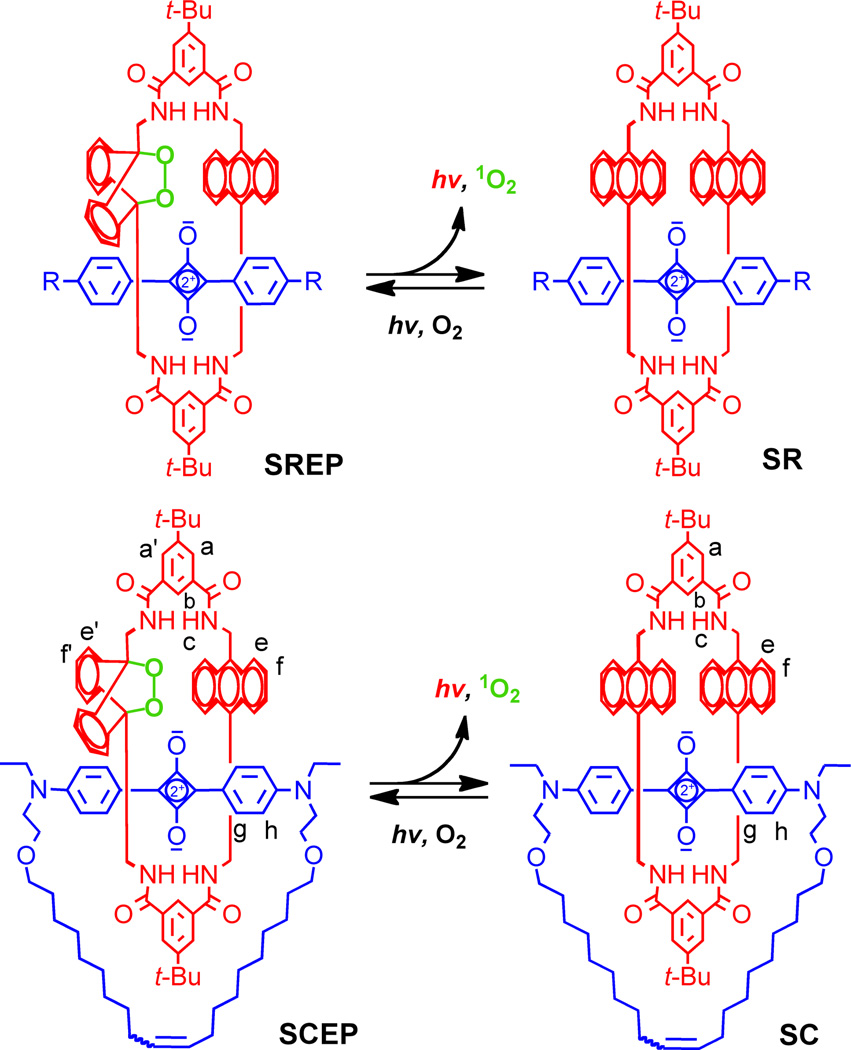
Thermal cycloreversion of SREP or SCEP releases singlet oxygen and emits near-infrared light. The reverse process of light irradiation in the presence of air is employed to prepare the storable endoperoxide compounds.
The synthesis of SREP has been reported before, and the same photoxidation method was employed to make SCEP. That is, simple irradiation of the known parent squaraine catenane (SC)14 with red light in the presence of air produced SCEP cleanly and in essentially quantitative yield with no evidence of over oxidation (Fig. 2). The room temperature 1H NMR spectrum of SCEP exhibits coalesced signal patterns that imply rapid circumrotation of one interlocked macrocycle relative to the other. In organic solution, the chemical and photophysical properties of SCEP and SREP are very similar. They both can be stored at −20 °C until needed and upon warming they undergo chemiluminescent cycloreversion reactions that hardly change the fluorescence profile of the encapsulated squaraine dye. The cycloreversion processes were conveniently monitored by the changes in 1H NMR spectra, and the first-order rate constant for SCEP cycloreversion (k1 = 0.39 h−1, t1/2 = 1.8 h) was found to be roughly two times faster than SREP (k1 = 0.17 h−1, t1/2 = 4.0 h) at 38 °C in C2D2Cl4. In each case, standard chemical trapping experiments showed that almost two thirds of the released oxygen was excited state singlet oxygen (see ESI). A straightforward particle-swelling procedure was employed to stain 20 nm carboxylate-functionalized polystyrene nanoparticles with SREP or SCEP at 4 °C.5 In both cases, equally high loadings of fluorescent nanoparticles were obtained, but chemiluminescence intensity with the SREP-nanoparticles was disappointingly low. In comparison, the SCEP-nanoparticles emitted thirty-fold higher chemiluminescence, a key discovery that permitted the mouse imaging studies described below.‡ Shown in Fig. 3 are representative chemiluminescent and fluorescent pixel intensity images of a vial, containing an aqueous dispersion of SCEP-nanoparticles, that was warmed from 4 °C to 38 °C. As expected, both the chemiluminescence and the fluorescence signals were observed selectively in the 695–770 nm emission filter channel. The chemiluminescence signal became more intense over the first few minutes as the cycloreversion rate increased with warming; subsequently, the emission decayed exponentially with a detectable signal persisting beyond two hours. The integrity of the dye-stained nanoparticles under physiological conditions was evaluated by in vitro experiments that incubated the particles with red blood cells and looked for transfer of the dye to the cells (see ESI). Essentially no dye uptake was observed over 45 minutes suggesting that significant leakage of SCEP from the nanoparticles was unlikely to occur over the shorter time required for in vivo mouse imaging studies.
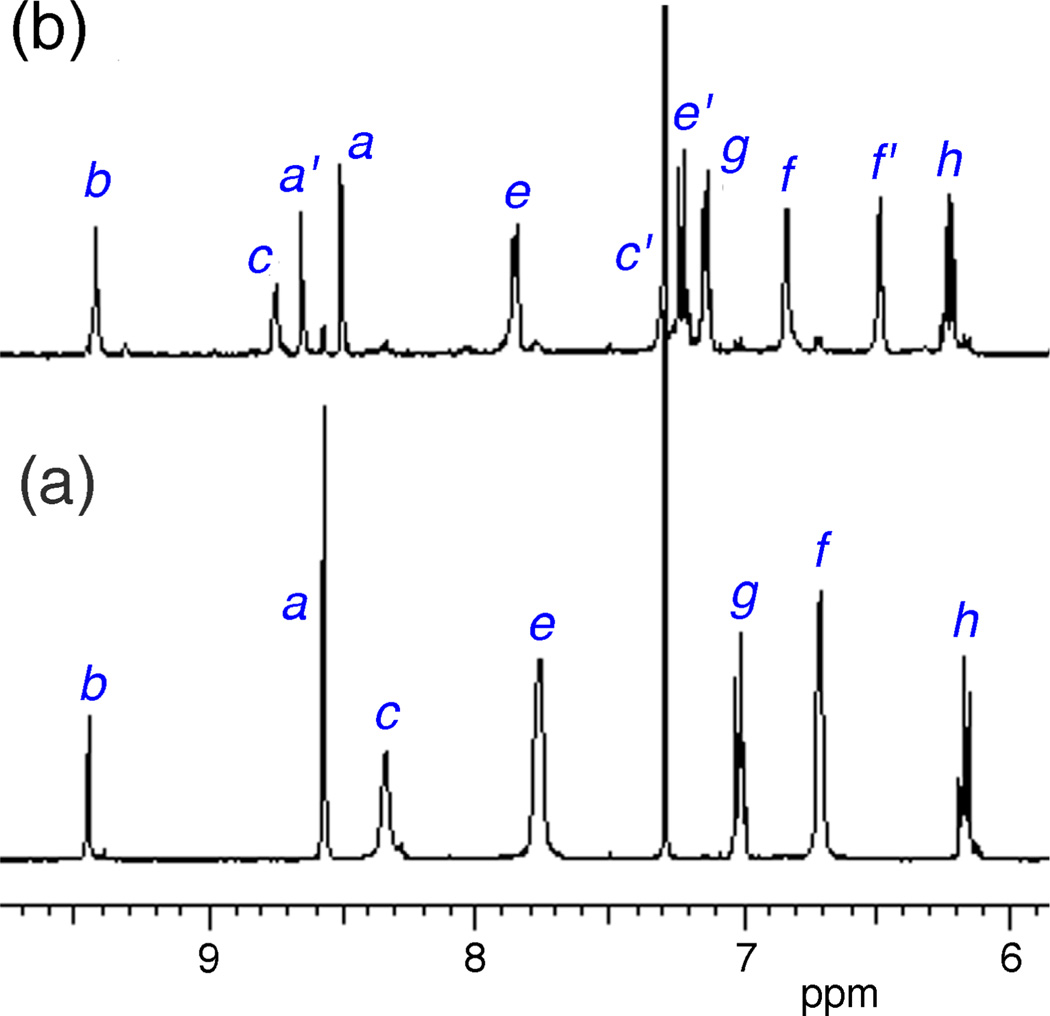
Partial 1H NMR spectra in CDCl3 (25 °C) showing clean and quantitative photoxidation of SC (a) to produce SCEP (b), achieved by irradiating an NMR tube with red light for two hours in the presence of air.
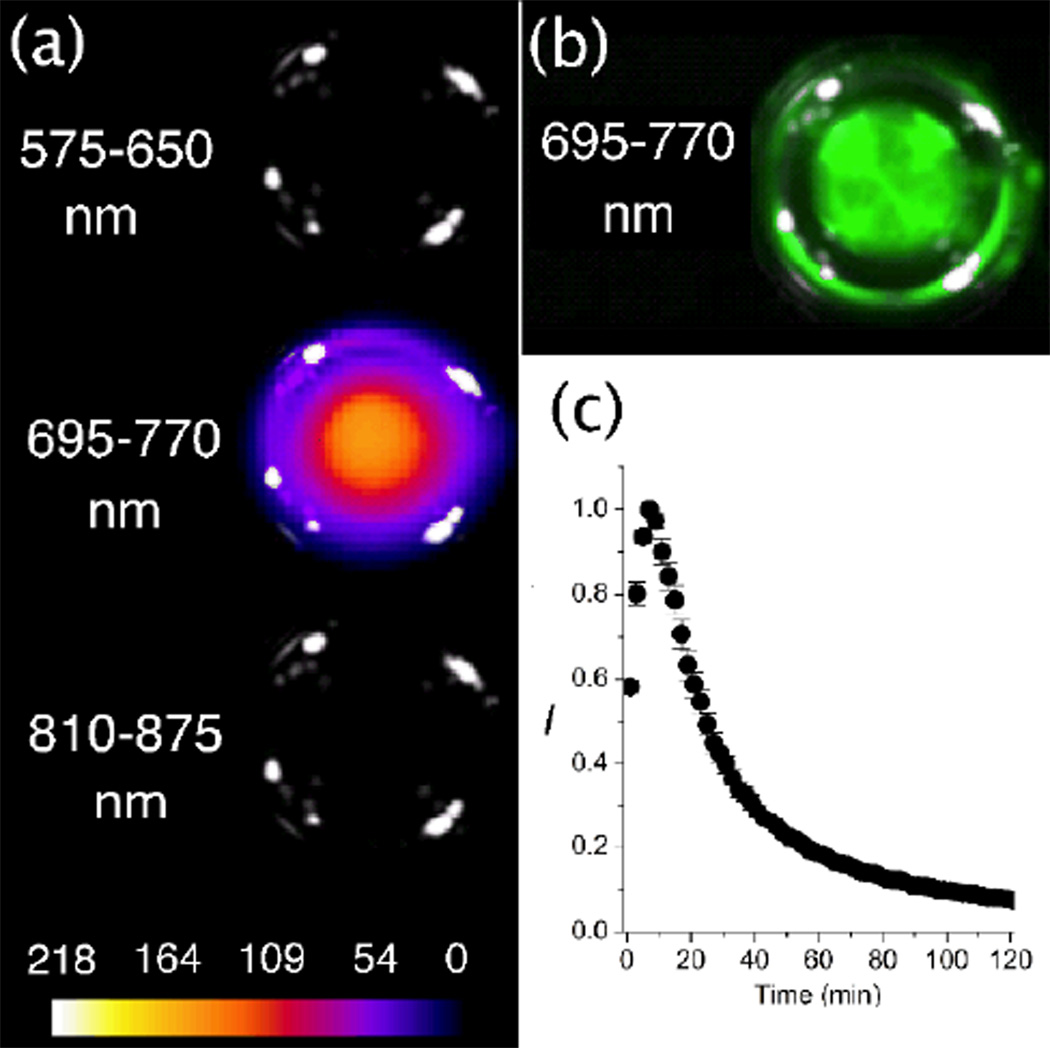
(a) Chemiluminescence pixel intensity maps of a vial at 38 °C containing an aqueous dispersion of SCEP-nanoparticles (20 nm carboxylate-functionalized polystyrene) and emitting only in the 695–770 nm channel of the imaging station. (b) The same vial emitted strong fluorescence in the 695–770 nm channel. (c) Chemiluminescence decay profile of the vial.
Fig. 4a shows the results of a typical whole-body imaging experiment with chemiluminescence and fluorescence pixel intensity maps of an anesthetized mouse at 10 minutes after tail vein injection of SCEP-nanoparticles (20 nm carboxylate-functionalized polystyrene). The TBR for each image was calculated by dividing the mean pixel intensity of the target lung region (T) by the mean pixel intensity of a non-target background region (B). Even a cursory inspection of the chemiluminescence and fluorescence images reveals that they are quite different. Although the chemiluminescence mean pixel intensity is substantially weaker, the average TBR for each lung is 6.3±0.3 (N=3) and more than double the average lung fluorescence TBR of 2.7±0.3 (N=3). Furthermore, the chemiluminescence image suggests that most of the nanoparticles are in the lungs, whereas the fluorescence image indicates that the particles are primarily in the liver (Fig. 4b). Ex vivo analysis of the organs after animal sacrifice proved that most of the nanoparticles were indeed in the lungs (Fig. 4c). In other words, in vivo chemiluminescence imaging was substantially more accurate than in vivo fluorescence imaging. The fluorescence imaging is misleading because it is highly “surface weighted” – even though the liver contained fewer SCEP-nanoparticles, the liver is closer to the animal surface and produces a much brighter fluorescence signal compared to the lungs which are located more deeply in the thoracic cavity partly behind the heart. The superior in vivo quantification provided by chemiluminescence imaging, compared to fluorescence imaging, is mentioned occasionally in the imaging literature,15 but the practical implications of this disparity have not previously been described. The whole-body images in Fig. 4b provide a striking demonstration of the difference in capabilities to accurately image deep-tissue sites. The direct comparison is enabled by the unique optical properties of SCEP-nanoparticles, which are both highly fluorescent and chemiluminescent.
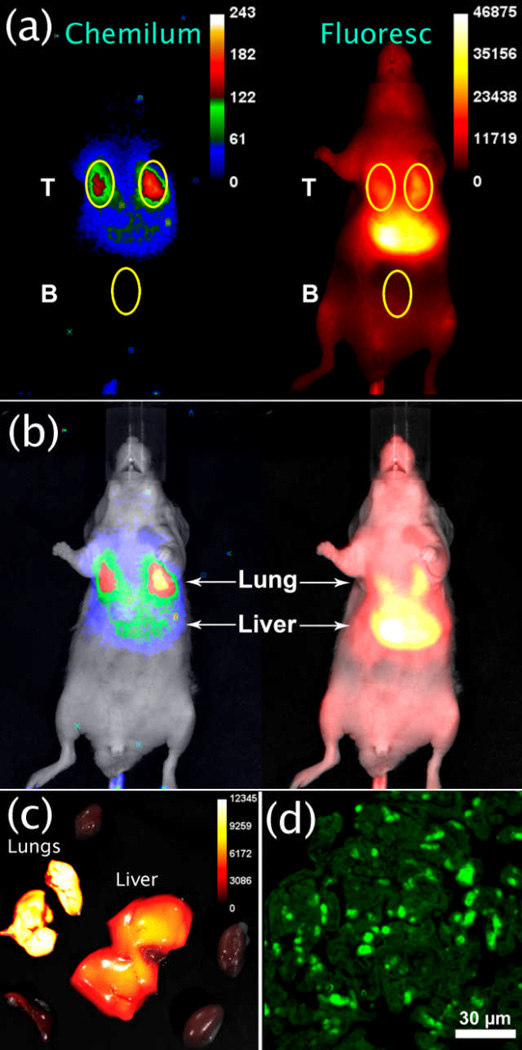
(a) Ventral chemiluminescence and fluorescence pixel intensity images of an anesthetized mouse at 10 mins after tail vein injection of SCEP-nanoparticles (20 nm carboxylate-functionalized polystyrene). (b) Overlay with bright field image highlights the different ratios of lung and liver signals. (c) Ex vivo fluorescence image of excised organs showing that most of the nanoparticles are in the lungs. (d) Fluorescence micrograph (695–770 nm emission channel) of lung histology section showing micrometer diameter aggregates of nanoparticles.
Extensive lung retention of these 20 nm nanoparticles was not expected since the average diameter of mouse lung capillaries is 5.7 µm,16 and several previous reports have shown unimpeded passage of micron-sized polymeric particles.17 Shown in Figure 4d is a fluorescent micrograph of a thin, histological section taken from excised lung tissue. The micrograph was acquired several days after the mouse was sacrificed, so the nanoparticles were no longer chemiluminescent but they were still highly fluorescent. The SCEP signal appears as micrometer-scale, fluorescent aggregates which is consistent with self-aggregation of the nanoparticles in lung capillaries after dosage;¶ however, alternative explanations, such as nanoparticle uptake by lung epithelial cells, cannot be ruled out at this point. In any case, the results show clearly that chemiluminescence imaging can be employed to accurately identify lung accumulation in living mice. This finding is justification for ongoing community efforts to develop improved chemiluminescent methods for in vivo imaging of deep-tissue sites.18 With further refinement we are hopeful that our optical imaging paradigm using CLF dyes can be utilized to monitor, in real-time, the progress of various types of lab animal experiments and nanoparticle delivery procedures.
Acknowledgments
This work was supported by the Notre Dame Integrated Imaging Facility and NIH grants R01GM059078 (B.D.S.) and T32GM075762 (D.R.R.). The animal studies were approved by the Notre Dame Institutional Advisory Care and Use Committee.
Footnotes
†Electronic Supplementary Information (ESI) available: experimental details and additional information. See DOI: 10.1039/b000000x/
‡Comparative control studies of micron-sized polystyrene particles, stained with either SREP or SCEP, showed very similar chemiluminescence intensities. We tentatively attribute the enhanced chemiluminescence observed with 20 nm SCEP-nanoparticles to the relatively compact and spherical molecular shape of SCEP. This might allow deeper penetration of the dye into the core of the highly curved nanoparticles, away from the surrounding quenching water, with a concomitant increase in energy transfer efficiency from the singlet oxygen released during the cycloreversion.
¶Dynamic light scattering studies showed that the SCEP-nanoparticles do not self-aggregate in the stock solution.
Notes and references
Full text links
Read article at publisher's site: https://doi.org/10.1039/c3cc40630j
Read article for free, from open access legal sources, via Unpaywall:
https://europepmc.org/articles/pmc3633569?pdf=render
Citations & impact
Impact metrics
Citations of article over time
Article citations
Nano-Theranostics for the Sensing, Imaging and Therapy of Prostate Cancers.
Front Chem, 10:830133, 12 Apr 2022
Cited by: 8 articles | PMID: 35494646 | PMCID: PMC9039169
Review Free full text in Europe PMC
Distinctive features and challenges in catenane chemistry.
Chem Sci, 13(12):3315-3334, 07 Feb 2022
Cited by: 5 articles | PMID: 35432874 | PMCID: PMC8943846
Review Free full text in Europe PMC
A Rolling Circle-Amplified G-Quadruplex/Hemin DNAzyme for Chemiluminescence Immunoassay of the SARS-CoV-2 Protein.
Anal Chem, 93(28):9933-9938, 06 Jul 2021
Cited by: 14 articles | PMID: 34227801
TADF Dye-Loaded Nanoparticles for Fluorescence Live-Cell Imaging.
Front Chem, 8:404, 08 May 2020
Cited by: 4 articles | PMID: 32457878 | PMCID: PMC7227253
Ultrasound-Enhanced Chemiluminescence for Bioimaging.
Front Bioeng Biotechnol, 8:25, 06 Feb 2020
Cited by: 3 articles | PMID: 32117914 | PMCID: PMC7016203
Review Free full text in Europe PMC
Go to all (15) article citations
Data
Data behind the article
This data has been text mined from the article, or deposited into data resources.
BioStudies: supplemental material and supporting data
Similar Articles
To arrive at the top five similar articles we use a word-weighted algorithm to compare words from the Title and Abstract of each citation.
Storable, thermally activated, near-infrared chemiluminescent dyes and dye-stained microparticles for optical imaging.
Nat Chem, 2(12):1025-1030, 24 Oct 2010
Cited by: 102 articles | PMID: 21107365 | PMCID: PMC3043620
Non-Covalently Pre-Assembled High-Performance Near-Infrared Fluorescent Molecular Probes for Cancer Imaging.
Chemistry, 24(52):13821-13829, 19 Aug 2018
Cited by: 15 articles | PMID: 30022552 | PMCID: PMC6415912
Internal and external stereoisomers of squaraine rotaxane endoperoxide: synthesis, chemical differences, and structural revision.
J Org Chem, 79(3):1120-1130, 01 Feb 2014
Cited by: 6 articles | PMID: 24428682
Squaraine Dyes: Molecular Design for Different Applications and Remaining Challenges.
Bioconjug Chem, 31(2):194-213, 12 Aug 2019
Cited by: 46 articles | PMID: 31365819 | PMCID: PMC7845514
Review Free full text in Europe PMC
Funding
Funders who supported this work.
NIGMS NIH HHS (4)
Grant ID: R01 GM059078
Grant ID: T32 GM075762
Grant ID: R01GM059078
Grant ID: T32GM075762