Abstract
Free full text

Human Dendritic Cells and Transplant Outcome
Abstract
Early interest in dendritic cells (DC) in transplantation centered on the role of graft interstitial DC in the instigation of rejection. Much information has subsequently accumulated concerning the phenotypic and functional diversity of these rare, migratory, bone marrow-derived antigen-presenting cells (APC), and their role in the induction and regulation of immunity. Detailed insights have emerged from studies of freshly-isolated or in vitro-propagated DC, and from analyses of their function in experimental animal models. The functional plasticity of these uniquely well-equipped APC is reflected in their ability not only to induce alloimmune responses, but to serve as potential targets and therapeutic agents for the long-term improvement of transplant outcome. Notably, however, a great deal remains to be understood about the immunobiology of DC populations in relation to human transplant outcome. Herein, we briefly review aspects of human DC biology in organ and bone marrow transplantation, the potential of these cells for monitoring outcome, and the role of DC in development of vaccines to protect against infectious disease or to promote allograft tolerance.
Since the first accounts of their morphology and function by Steinman and Cohn in 1973/4 (1, 2), dendritic cells (DC) have been recognized increasingly as critically important inducers and regulators of innate and adaptive immunity. The significance of their discovery and the numerous insights that have been gained subsequently into their basic and applied immunobiology were recognized in 2007 by the Lasker Award for Basic Medical Research to Ralph M. Steinman MD (3).
That DC are rare, bone marrow (BM)-derived leukocytes, that arise from CD34+ stem cells, and that are uniquely well-equipped for antigen (Ag) presentation has long been recognized. DC are distributed ubiquitously throughout the body, especially in proximity to portals of entry, and can be identified in all commonly-transplanted organs. They traffic from peripheral tissue under steady-state or (at enhanced levels) under inflammatory conditions, including organ transplantation, via afferent lymphatics or blood, to secondary lymphoid tissue. Therein they present self or foreign Ag to T cells expressing appropriate receptors. Immature DC, like those found in the periphery under noninflammatory conditions, display low levels of surface major histocompatibility complex (MHC) class II (in the context of which antigenic peptides are presented) and costimulatory molecules (CD40/80/86), and thus have tolerogenic properties. By contrast, DC induced to mature by environmental stimuli (foreign molecules, alarmins, tissue factors and cytokines) express high levels of MHC II, costimulatory molecules, and the chemokine receptors necessary for homing to secondary lymphoid tissue, are potent stimulators of T helper cells.
The derivation, biology, immunoregulatory ability and clinical applications of DC have been reviewed extensively (4–10). Much of what we have learned about DC development and function over the past 35 years has been based largely on studies of cells isolated from rodent models, or propagated in vitro, either from murine BM or human blood-borne precursors, in response to appropriate hematopoietic growth factors. Phenotypic differences between murine and human DC can make direct extrapolation of function difficult, and there is much that remains unknown about human DC development and function, especially in vivo.
In rodents and humans, multiple DC subsets have been described, in addition to the conventional myeloid (m)DC, described initially in mouse spleen (1). These include epidermal Langerhans cells (LC), and the more recently characterized plasmacytoid (p)DC, the principal interferon (IFN)α-producing cells in the body. The ability of murine DC induce and to regulate T cell responses in vitro and in vivo, and similar properties of human DC reported in vitro, have prompted investigation of the role of these cells in transplant recipients. Particular attention has focused on (1) the role/ fate of donor-derived and host DC, in relation to allorecognition/regulation of T cell responses, (2) monitoring of DC subsets, especially in relation to transplant outcome, (3) the impact of immunosuppressive agents on DC subsets, (4) the potential of DC-based vaccines for therapy of transplant-associated infectious disease (e.g. Epstein-Barr virus [EBV] or cytomegalovirus [CMV] infection) and (5) the potential of “tolerogenic” DC for therapy of allograft rejection. Herein, we shall briefly review each of these topics.
Identification and Characterization of Human DC in Blood and Tissues
Circulating human DC precursors can be identified readily in whole blood or in mononuclear cell (MNC) populations harvested from blood (11), organ perfusion (12), or bronchiolar lavage fluid (13) by monoclonal antibody (mAb) staining and multi-color flow cytometry. Monocytoid DC are lineage (lin)-MHC II+CD11c+CD123 (IL-3R)−, whereas pDC are lin−MHC II+CD11c–CD123+. Alternatively, DC subsets in blood can be identified using a panel of mAbs directed against blood DC Ag (BDCA-1) (CD1c) expressed on mDC, BDCA-2 (a type II C-type lectin) expressed specifically on pDC (14), and CD14 and CD19 (15, 16) (Fig. 1). Traditionally, as in rodents, human DC have been identified as MHC II+ (HLA-DR+) cells in the kidney (17), liver (18, 19), heart, and pancreas (20, 21).Contemporary immunofluorescent/histochemical staining for interstitial DC-expressed Ags allows the distinction and quantification of HLA-DR+ DC subsets in tissues such as kidney, based on their differential expression of BDCA-1/2 and DC-specific intercellular adhesion molecule-3 grabbing non-integrin (DC-SIGN; CD209) (22). Intracellular cytokine production can be measured in circulating human DC and IL-12-and IL-10-producing DC have been associated with time post-transplant, dose of immunosuppression and plasma cytokine levels in renal allograft patients (23).
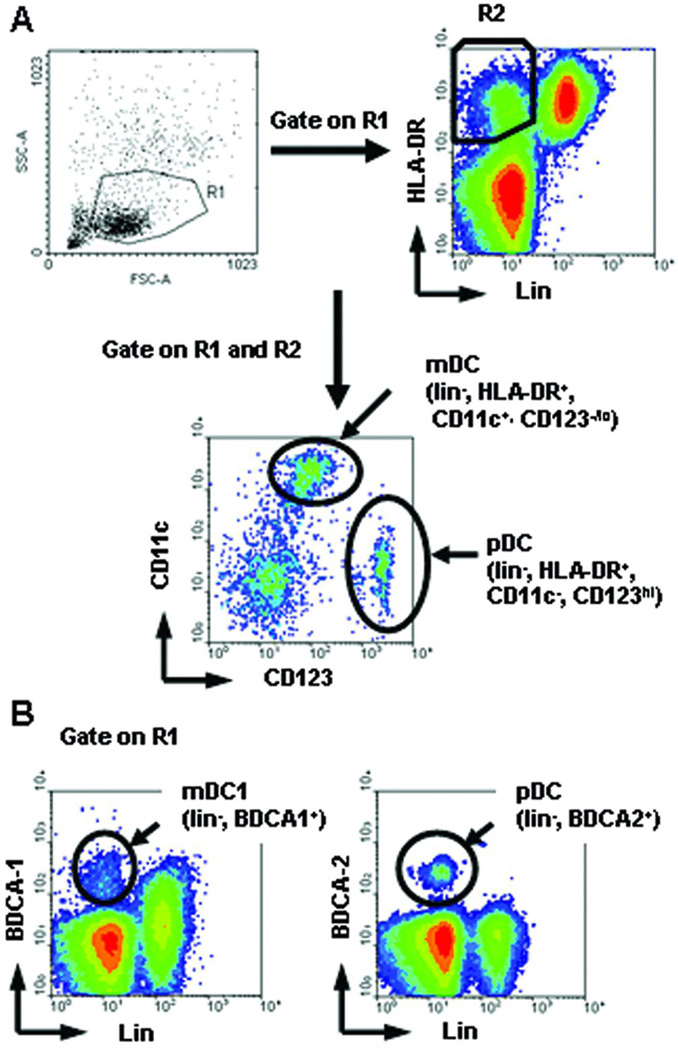
Strategies used for the identification of circulating human DC subset precursors. (A) Historical 4-color staining method: cluster resolution and phenotypic characteristics of mDC and pDC subsets. DC subsets were stained with lineage (lin) mAb cocktail (anti-CD3,−14,−19,−20) and with anti-CD11c and anti-CD123 (IL-3R α). mDC are lin- CD11c+ HLA-DR+ cells; pDC are lin-, CD123bright HLA-DR+. (B) Direct DC subset-specific mAb staining method: cluster resolution and phenotypic characteristics of mDC1 and pDC subset precursors. PBMC were stained with lin mAbs, BDCA-1 and BDCA-2. BDCA-1+ lin−cells are considered mDC1. Lin- BDCA-2+ cells are pDC. (Reproduced from Tokita D. et al Transplantation 2008; 85:369)
The Fate and Function of Donor-Derived DC in Human Organ Transplantation
Early rodent work described the trafficking of donor (heart or skin)-derived MHC II+ DC (“passenger leukocytes”) to host secondary lymphoid tissue and the disappearance of these cells around the time of acute allograft rejection in non-immunosuppressed recipients (24, 25). Consistent with the prevailing paradigm of DC function at the time, and with earlier experimental studies of the role of interstitial donor DC in kidney graft rejection (26), these donor-derived DC were implicated as the principal instigators of rejection. In keeping with this view, efforts to deplete donor MHC II+ or CD45+ passenger leukocytes by perfusion of human kidney grafts with mAbs before transplantation, led to a reduced incidence of rejection (27, 28). However, later studies of stable human organ allograft recipients, including patients off all anti-rejection therapy, revealed donor-derived DC and other hematopoietic cells (‘microchimerism’ [<1% chimerism]) in lymphoid and non-lymphoid tissues (29, 30). These donor cells, detected by immunohistochemical and molecular analyses, and likely derived from hematopoietic precursors, were linked to allograft acceptance and the regulation of anti-donor immune reactivity (30– 32). As in unmanipulated mouse liver allograft recipients (33), that accept grafts across MHC barriers without immunosuppressive therapy, donor-derived DC could be propagated from circulating progenitors within (donor BM-augmented) human liver transplant recipients (34), providing a basis for their persistence and expansion.
These observations in humans raised fundamental questions about the role of donor-derived (and host) DC in organ transplant outcome. Subsequent observations of the tolerogenic potential of donor-derived DC in animal models (reviewed in (9, 35)) have shifted the perception of DC from solely that of instigators of rejection, to potential instigators and regulators of alloimmunity. However, direct functional assessment of these rare cells in human transplant patients is very difficult. On the other hand, observational studies of human organ allograft survival have implicated donor DC in either immune activation or the maintenance of tolerance (36). In studies of small numbers of patients, Burlingham and colleagues (37) have implicated donor DC in infectious tolerance in human renal transplantation, and have suggested a role for pDC in the regulation of bystander immune suppression by adaptive regulatory T cells (Treg) in tolerant patients (38).
Impact of Disease and Immunosuppressive Drugs on DC in Human Transplant Recipients
Enumeration of DC and their distribution within known subsets in healthy, age- and gender-matched individuals, and in patients pre- and post-transplant, provides the basis for assessment of their value as correlates/predictors of graft outcome. Patients in chronic renal failure display decreased numbers of DC in peripheral blood (39, 40) and of LC in the skin and cervix (41) compared with healthy controls. In the first week post-transplant, blood mDC and pDC drop substantially, with reductions in the pDC subset being more profound (42). It has been reported that by approximately four months after renal transplant, DC levels return to pre-transplant values, likely due to tapering of immunosuppressive drugs (42). However, significant reductions in circulating DC subsets have been documented in renal transplant patients on long-term (>1yr) immunosuppression (15). Similar trends have been observed in liver transplant patients, in whom several-fold reductions in mDC immediately post-transplant normalized between three and twelve months (43). Many factors may alter the numbers and balance of peripheral blood and tissue DC subsets. Thus, depleting agents, such as anti-thymocyte globulin (ATG) and Campath-1H (anti-CD52 mAb), maintenance immunosuppression, infection, and time post-transplant may contribute to the variable results that have been reported.
In vivo effects of immunosuppressive and anti-inflammatory drugs on DC numbers, differentiation and function (44) may contribute to (i) a patient’s net state of immunosuppression, (ii) the probability of successful immunosuppressive drug weaning, (iii) susceptibility to viral infectious, (iv) tumor development, and (v) transplant outcome. Corticosteroids appear to be particularly detrimental to pDC numbers and function. A four-day course of corticosteroids in healthy volunteers was found to cause a precipitous drop in the number of pDC that began to recover immediately after steroid withdrawal (45). In vitro, clinically-relevant concentrations of prednisolone, but not calcineurin inhibitors (CNI) (tacrolimus or cyclosporine), induce pDC apoptosis (46). Most studies indicate that both mDC and pDC are decreased significantly in the months post-transplant, and do not necessarily recover completely in patients on long-term immunosuppression (15). Interestingly, neither the dose of prednisone nor tacrolimus, nor the tacrolimus trough level, appears to affect the incidence or ratio of DC subsets in liver allograft recipients (47). Furthermore, these DC exhibit maturational impairment, that is partially or fully reversible in vitro after removal of the cells from the immunosuppressive environment (43, 48).
Certain drugs require special attention, as their influence may confound interpretation of DC subset levels. Most peripheral blood mDC, and some pDC, express CD52, but progressively lose this marker as they mature (e.g. LC do not express CD52) (49). The peripheral DC repertoire has been evaluated in kidney transplant recipients receiving Campath-1H (alemtuzumab), followed by tacrolimus monotherapy, and in those given conventional triple drug therapy (tacrolimus, mycophenolate mofetil, and steroids) (50). Campath-1H caused a strong, sustained reduction in the total number of peripheral DC, with a shift in the pDC/mDC ratio towards pDC. A study comparing heart transplant recipients on rapamycin (sirolimus) with those on a CNI revealed a higher incidence of pDC in those patients on sirolimus maintenance therapy, with a shift in the pDC/mDC ratio toward pDC (51). Patients converted from a CNI to either sirolimus or everolimus exhibited an even greater shift in the pDC/mDC ratio compared to those in the CNI and sirolimus maintenance groups.
DC Enumeration in Human Blood: Variables and Limitations
The recent literature suggests a potential role for peripheral blood DC subset analysis in monitoring of transplant outcome. Widespread application of this new tool faces several obstacles, including variability in technique, absence of reference standards, and as yet, incomplete data regarding the influence of disease, medications, and patient-related factors on blood DC subsets (Table 1) (39, 40, 42, 50–59); see also Field et al (60). A potential confounding factor when comparing published data, is the variation in methods used for DC isolation/analysis that can significantly affect DC recovery, the blood pDC/mDC ratio, and the level of expression of DC surface markers, such as chemokine receptors. The most commonly-studied human blood DC subsets are the CD11c+CD123low mDC and the CD11c−CD123high pDC. Not only are there various markers that can be used to identify these subsets, but new and overlapping subsets (based more on phenotype than function) are emerging (61). Specific techniques are known to alter blood DC measurements. The staining of Ficoll gradient-isolated MNC may result in a higher pDC/mDC ratio compared to whole blood staining (59). Cryopreservation may further affect this ratio. Vuckovic et al (61) have described a highly reproducible, flow cytometric whole blood assay based on TruCOUNT™ beads, that requires only 50µL of whole blood and does not produce DC-T cell conjugates that can result in counting abnormalities in conventional density gradient separation procedures. Such a protocol may aid in global standardization of DC monitoring.
Table 1
Common Potential Confounders of Blood DC Monitoring in Transplant Patients
AGENT/EVENT | EFFECT ON BLOOD DC SUBSETS | CHANGE IN pDC/mDC RATIO | REFERENCE | |
---|---|---|---|---|
Drugs | Campath-1H (anti-CD52) | Greater depletion of mDC over pDC | ↑ | Kirsch et al (50) |
ATGa | Greater depletion of mDC over pDC | ↑ | Womer et al (42); Fang et al (53) | |
Steroids | Complete depletion of both mDC and pDC; mDC recover faster | ↓ | Suda et al (52) | |
Rapamycin | May increase pDC | ↑ | Barten et al (51) | |
G-CSFb | Mobilizes greater numbers of pDC | ↑ | Vuckovic et al (54) | |
CNIc | Increases mDC compared to CNI-free regimens | ↓ | Lim et al (40) | |
Comorbitities/demographics | Chronic kidney disease; no renal replacement therapy | Decreases pDC | ↓ | Hesselink et al (39) |
Hemodialysis | Reduces mDC but not pDC | ↑ | Hesselink et al (39); Womer et al (42) | |
Coronary artery disease | Increases mDC | ↓ | Shi et al (55) | |
Chronic heart failure | Increases mDC | ↓ | Athanassopoulos et al (56) | |
Age | mDC is unchanged while pDC decreases with age (8m to 18y) | ↓ | Teig et al (57) | |
Surgical trauma | Absolute DC counts increase acutely, then drop below pre-operative levels on days 2–3 | none | Ho et al (58) | |
DC processing | Ficoll isolation | Relative increase in pDC | ↑ | Gerrits et al (59) |
Cryopreservation of PBMC | - | ↑ | Gerrits et al (59) | |
Lack of reference standard | n/a | n/a | ||
DC survival after isolation | unknown | unknown | ||
Choice of marker | variable | variable | Field et al (60) |
Human DC and Correlation With/Prediction of Outcome in Allogeneic BM or Organ Transplantation
Absolute numbers of DC, their origin, maturation or activation status, and DC subset distribution, may have potential as predictive indices of human transplant outcome (Table 2) (23, 47, 61–68).
Table 2
Dendritic Cell Assays and Correlation with HSC and Organ Transplant Outcome
DC MEASUREMENT | OUTCOME | ANALYSIS | PATIENTS STUDIED | REFERENCE | |
---|---|---|---|---|---|
Hematopoietic Stem Cell Transplantation | CMRF-44 expression on blood mDC (>7.9%) prior to GVHD onset | grade 2–4 aGVHD | PPVa= 73.7%, NPVb= 90.5% Sensitivity= 87.5%, Specificity= 79.2% 95% CIc (0.831–1.002) p= 0.0001 | 40 adult allo-HSCT recipients | Lau et al. (62) |
Total blood DC count (low) 3 months post HSCT | 1. death 2. poor EFSd 3. relapse | 1. RRe= 8.2; 95% CI (1.9–34.5) p= 0.004 2. RR= 6; 95% CI (2.1–17.25) p= 0.001 3. RR= 4.1; 95% CI (1.3–12.5) p= 0.01 | 79 adult RICf-allo-HSCT recipients | Talarn et al. (63) | |
Blood pDC count (low) 3 months post HSCT | aGVHD | RR= 2.4; 95% CI (.091–6.5) p= 0.06 | 79 adult RIC-allo-HSCT recipients | Talarn et al. (63) | |
Blood pDC (>0.725/ µL) 3 months post RIC | death | RR= 0.34; 95% CI (0.12–0.96) p= 0.04 | 54 adult RIC-allo-HSCT recipients | Mohty et al. (64) | |
Total blood DC count (<4.97/µL) around time of engraftment | 1. death 2. aGVHD 3. relapse | 1. RR= 3.67; 95% CI (1.50–8.94) p= 0.0023 2. RR= 3.21; 95% CI (1.61–6.40) p= 0.0005 3. RR= 5.07; 95% CI (1.62–15.89) p= 0.0021 | 50 adult allo-HSCT(92%) or BMT(8%) recipients | Reddy et al. (65) | |
Presence of host DC at day 100 post HSCT | 1. grade 2–4 aGVHD and extensive cGVHD 2. death | 1. p= 0.001 2. p= 0.01 | 24 adult HSCT recipients after RIC (n=21) or conventional conditioning (n=3) | Chan et al. (66) | |
Solid Organ Transplantation | Blood pDC/mDC ratio | n/a | Tolerant and weaning patients had 4-4.5 fold higher pDC/mDC ratio compared with patients on maintenance immunosuppression p<0.001 | 75 (58 pediatric, 17 adult) clinically stable liver recipients | Mazariegos et al. (47) |
Blood mDC/pDC ratio at the time of EMBg | 1. grade 2 rejection 2. grade 3A/B rejection | 1. p<0.05 2. p<0.01 | 20 adult heart transplanth recipients | Athanassopoulos et al. (67) | |
CD83 expression or total infiltrating graft from EMB | 1. grade 1 rejection 2. grade 2 rejection | 1. p< 0.05 compared with grade 0 2. p< 0.05 compared with grade 1 | 21 adult heart transplant recipients | Sorrentino et al. (68) | |
Blood IL-10+DC and IL-12+DC levels | n/a | IL-10+DC dominate late post-transplant period in complication-free recipients | 57 renal transplant recipients | Daniel et al. (23) | |
Total blood DC and DC subsets | n/a | This single-platform TruCOUNT™ bead assay enumerates DC subsets from 50 µL of whole blood 24h after collection in EDTA tubes. | n/a | Vuckovic et al. (61) |
Number and origin (donor versus host) of DC are associated with acute (a) graft-versus-host disease (GVHD), relapse, and graft failure after human allogeneic hematopoietic stem cell transplantation (HSCT). Patients with aGVHD after HSCT have lower numbers of circulating mDC and pDC compared to healthy volunteers (69, 70), but a higher incidence of DC in affected tissue, such as skin (71). A dominant donor chimerism of migratory LC and dermal DC was detected, even early after transplantation (71). DC monitoring may be used clinically to predict outcomes in HSC/BM transplantation. Thus, Reddy et al (65) have demonstrated that the absolute number of circulating DC after allogeneic HSC transplantation is an independent predictor of post-transplant aGVHD, relapse, and even mortality. Subsequent studies have further analyzed the predictive value of DC subsets and the expression of DC activation markers. CMRF44 is a human DC early activation marker, that is absent from peripheral blood DC in healthy volunteers. However, Lau et al (62) have demonstrated that CMRF44 expression on >7.9% peripheral blood CD11c+ DC identifies patients who will develop grade 2–4 aGVHD, with positive and negative predictive values of 73.7% and 90.5% respectively. This approach may be useful as a prognostic tool to guide early therapeutic intervention in human HSC transplantation, with CMRF44+ CD11c+ DC as potential therapeutic targets (72).
GVHD is mediated by the response of donor T cells to host tissues, particularly skin, mucosa, bowel, and liver. Despite a 10-fold higher dose of T cells in HSCT vs BMT, the risk of aGVHD is only slightly higher, but chronic (c)GVHD is increased significantly (73). Leukemia relapse rate is lower and overall survival is improved (74). DC have been attributed a central role in this complex balance of graft-host, graft-tumor, and host-graft interactions. The first report came from Waller et al (75) who reported that patients given larger numbers of CD4bright DC (putative pDC) had significantly lower event-free survival, increased incidence of relapse, and lower incidence of cGVHD. Granulocyte- colony-stimulating factor (CSF) mobilizes greater numbers of pDC to the peripheral blood (76), resulting in a higher pDC:mDC ratio in HSCT compared to BMT. Arpinati et al (77) refer to data suggesting that greater graft pDC numbers significantly increase the risk of cGVHD. Significantly higher numbers of pDC of exclusive donor origin were found in patients with cGVHD, while the persistence of host DC was observed only in patients free of cGVHD (78). Another clinical study evaluating the relationship between the cellular composition of HSC graft and outcome suggests that a lower number of pDC in the graft (<1.7×106/kg) results in better disease-free survival and overall survival (79). The nature of interactions between DC subsets and other immune cells in HSC grafts and BM grafts is largely unknown. The significance of host vs donor origin of DC, and the relationship between graft DC content and host DC reconstitution post-transplant also have yet to be clarified. There are as yet no reports of therapeutic DC manipulation in clinical HSCT/BMT.
The solid organ transplantation literature also contains recent reports on the potential of blood and tissue DC monitoring to predict and improve graft outcome. In a cross-sectional study of pediatric liver allograft recipients, Mazariegos et al (11) found a significantly higher circulating pDC/mDC precursor ratio in patients undergoing weaning or successful withdrawal of immunosuppression, compared to patients on maintenance immunosuppressive therapy. The higher relative incidence of pDC may reflect a state of low anti-donor responsiveness and could be used to select patients for drug weaning. Subsequent work from this group has confirmed the earlier findings with a larger sample size, and suggested a pDC/mDC subset ratio of 0.1 as a possible threshold for weaning (47). The results of sequential monitoring of peripheral blood DC subsets (pDC/mDC ratio) in relation to clinical history are awaited with interest, as these studies will determine whether the assay can be used to predict safe drug withdrawal. Interestingly, a high ratio of co-inhibitory programmed death ligand (PD-L)1 (=B7-H1) to costimulatory CD86 (B7-2) on circulating pDC correlates with elevated levels of Treg in clinically tolerant, liver transplant patients (16).
DC monitoring may also have a role in the prediction of acute organ allograft rejection. During acute rejection, heart transplant recipients displayed a marked decrease in peripheral blood mDC, that correlated with rejection grade in endomyocardial biopsies (EMB) (67, 80). A similar finding was reported in three kidney transplant recipients who experienced at least one rejection episode and demonstrated decreased circulating DC compared to non-rejecting controls (81). This raises the question of the role/significance of peripheral blood DC in acute rejection, their migratory function, and the immunologic consequences of incomplete reconstitution of circulating DC. DC were distributed evenly in Grade 0 (G0) EMBs from heart graft recipients, while G1 histology revealed perivascular accumulation and G2 showed the same accumulation, but with significantly increased DC numbers (68). Expression of the human DC maturation marker CD83 was almost undetectable in G0 samples, but increased progressively in G1 and G2. Increased mDC were also noted in acutely-rejecting kidney allografts compared to healthy renal tissue (22). Though draining lymph nodes were not sampled in these studies, it is likely that DC participate in the acute rejection response by migrating (as precursors) from peripheral blood to the allograft and subsequently to secondary lymphoid tissue, accounting for the decrease in circulating DC.
Aside from the potential prognostic value of monitoring DC subsets, recent data suggest that, in allogeneic HSCT, reconstituting certain DC populations post-transplant might improve outcome. Mohty et al (64) have demonstrated that patients with a high pDC recovery after SCT show improved overall survival, and a reduced incidence of late viral, bacterial, and fungal infections. Whether pDC levels are merely a marker for transplant outcome, or pDC are involved directly in determining outcome has not been established. Their role as the body’s principal producers of type-I IFNs suggests they may be important in mediating innate immune responses following HSCT.
Human DC as Therapeutic Agents
Based on preclinical studies, immature, ‘semi-mature’ or ‘alternatively-activated’ DC, and DC that have been exposed to immunosuppressive agents in vitro to preserve/potentiate their tolerogenicity, offer potential for therapy of GVHD and leukemia relapse after allogeneic BMT (82), or for promotion of long-term organ allograft survival (9). Proof-of-principle that immature (autologous) Ag-pulsed human mDC can induce Ag-specific T cell tolerance, associated with the induction of Ag-specific Treg, has been demonstrated in human volunteers (83, 84).
In these studies, DC were generated from monocytes isolated from T cell-depleted peripheral blood MNC by plate adherence. The monocytes were cultured with granulocyte-macrophage (GM)-CSF and IL-4 to produce immature DC. Two volunteers received a single subcutaneous injection of 2×106 immature DC pulsed with keyhole limpet hemocyanin and influenza matrix peptide, resulting in Ag-specific inhibition of effector T cells. This group later reported that CD8+ T cells detected in the blood, one week after immature DC injection, were able to block the function of IFN-γ -secreting CD8+ T effector cells (84). Though these studies with immature DC therapy were terminated due to fear of reducing influenza-specific effectors in the volunteers, their implications for the therapeutic potential of ‘tolerogenic’ DC in transplantation are clear.
Reliable, large-scale generation of clinical grade human DC with tolerogenic potential is imperative for their testing as therapeutic agents in allograft recipients. Excellent progress has been made in human DC expansion and purification in the field of cellular vaccines for cancer treatment (85–87). While maturation of DC improves their ability to stimulate Ag-specific T cells,- a pre-requisite for immunization, immature DC have a propensity to downregulate immune responses, making them attractive as ‘negative cellular vaccines’ for the promotion of donor-specific tolerance (88).
The two major sources for expansion of human DC precursors are peripheral blood and BM. CD34+ HSC can be collected from BM or blood after cytokine mobilization. These DC precursors have the advantage of high proliferative capacity, but may produce a heterogeneous population of DC and lower yields compared to other methods. DC constitute < 1% of peripheral blood MNC (89). They can, however, be generated from circulating CD14+ monocytes. Closed systems for the enrichment of monocyte-derived DC that meet current good manufacturing practice (cGMP) guidelines are available. Once the MNC are collected by leukapheresis, they can be enriched for monocytes by filtration, elutriation, adherence, or negative or positive selection. Closed-system protocols employing leukapheresis, elutriation or negative selection, followed by 7-day culture in serum-free medium with GM-CSF and IL-4 can yield 5×108 immature mDC from 5×109 MNC collected from a 7.5L blood volume leukapheresis (90). Similar strategies have been used to generate maturation-resistant rhesus monkey mDC that, when infused systemically into allogeneic recipients in conjunction with costimulation blockade, modulate alloimmune reactivity and induce T-cell hyporesponsiveness to donor (91).
Immature, monocyte-derived DC have high endocytic activity and are poor T cell stimulators. Recent studies have characterized maturation and migration markers on these cells. While maturation status is likely the most important DC characteristic with respect to their use in human immunotherapy, pDC are thought to be inherently tolerogenic and can induce Treg in humans (92). It has not yet been ascertained whether immature human pDC may be more efficacious than immature mDC for transplant tolerance induction.
DC and the Prevention/Treatment of Transplant-Associated Infection
Recipients of allogeneic HSC or solid organ transplants are at increased risk of viral infection. EBV and CMV are two prevalent and problematic viruses affecting this population. EBV-associated post-transplant lymphoproliferative disease (PTLD) occurs in up to 20% of graft recipients, depending on the type of allograft (93). Chemical prophylaxis has reduced the incidence and severity of CMV infection, but it is still associated with both acute and chronic rejection (94), as well as other morbidities and mortality (95, 96). New experimental DC-based therapies have been employed to target these viruses.
EBV-associated PTLD has been treated successfully by adoptive transfer of EBV-specific cytotoxic T lymphocytes (CTL) (97). These CTL were prepared by repeated stimulation with autologous lymphoblastoid cells (LCL). However, EBV peptide-pulsed mature autologous DC were found to be 10-fold more efficient at expanding CTL compared to LCL (98). Interestingly, unlike healthy controls, where DC loaded with MHC class I EBV peptides preferentially reactivated specific type-1 CD8+ T cells, DC generated from transplant patients reactivated EBV-specific CD8+ Treg that produced both IFNγ and IL-10, upregulated Foxp3, and suppressed non-cognate CD4+ T cell proliferation (99). These findings suggest a novel regulatory pathway for T-cell-mediated anti-EBV responses in immunosuppressed transplant recipients, with implications for the design of adoptive cellular immunotherapy in the clinical setting. CTL expanded by DC genetically modified to express LMP2, an EBV-derived Ag, have been shown recently to effect a complete clinical response in 4/6 patients with active, relapsed lymphoma (100). Similar techniques using DC pulsed with viral Ag have been employed for the treatment and prevention of CMV infection (101–103). In vivo injection of Ag-pulsed DC, rather than the CTL that they expand in vitro, has also been studied. Small clinical trials with EBV-(104) and CMV- Ag-pulsed DC vaccines (101) demonstrate the promise of this therapy. DC vaccine research continues with other diseases that affect transplant patients, such as hepatitis B (105), hepatitis C (106), HIV (107), BK virus (108), and various fungal infections (109–111).
Conclusion
Much of what we have learned about human DC immunobiology has been based on studies of isolated cells or tissues, or of DC propagated in vitro. Functional analysis of these cells in blood and tissues is challenging because of their rarity, but valuable reagents and techniques that should enhance insights are now available to interrogate the principal DC subsets. In human transplantation, evidence has emerged that characterization/quantification of DC subsets may be useful in predicting outcome, and that specific DC populations may correlate with graft survival. Immunosuppressive drugs can markedly inhibit DC numbers and function (particularly pDC) with implications for resistance to viral infection and cancer. Human DC expanded in vitro have therapeutic potential for the management of viral infection and cancer and the promotion of tolerance in allograft recipients.
ACKNOWLEDGMENTS
We thank Ms. Miriam Freeman for administrative support and Dr. Daisuke Tokita for generating the data depicted in Fig. 1. We acknowledge the valuable input of current colleagues and laboratory alumni to these studies, and the important contributions of those investigators whose work has or (due to space limitations) may not have been cited in this Overview.
Supported by National Institutes of Health (NIH) NRSA Postdoctoral Fellowship F32 AR054225 (to MGS) and by NIH grants R01 AI60994, R01 AI67541 and U01 AI51698 (to AWT)
Glossary
Ag | antigen |
BM(T) | bone marrow (transplantation) |
DC | dendritic cell(s) |
GVHD | graft-versus-host disease |
HSC(T) | hematopoietic stem cell (transplantation) |
LC | Langerhans cell(s) |
mDC | myeloid/monocytoid DC |
pDC | plasmacytoid DC |
PTLD | post-transplant lymphoproliferative disease |
REFERENCES
Full text links
Read article at publisher's site: https://doi.org/10.1097/tp.0b013e318173a768
Read article for free, from open access legal sources, via Unpaywall:
https://europepmc.org/articles/pmc3780572?pdf=render
Subscription required at www.transplantjournal.com
http://content.wkhealth.com/linkback/openurl?issn=0041-1337&volume=85&issue=11&spage=1513
Citations & impact
Impact metrics
Citations of article over time
Article citations
Overexpression of miR-223 Promotes Tolerogenic Properties of Dendritic Cells Involved in Heart Transplantation Tolerance by Targeting Irak1.
Front Immunol, 12:676337, 05 Aug 2021
Cited by: 8 articles | PMID: 34421892 | PMCID: PMC8374072
Standardisation of flow cytometry for whole blood immunophenotyping of islet transplant and transplant clinical trial recipients.
PLoS One, 14(5):e0217163, 22 May 2019
Cited by: 8 articles | PMID: 31116766 | PMCID: PMC6530858
Dendritic Cell Therapy in Transplantation, Phenotype Governs Destination and Function.
Transplantation, 102(10):1593-1594, 01 Oct 2018
Cited by: 1 article | PMID: 29677071 | PMCID: PMC6153052
CD83 Antibody Inhibits Human B Cell Responses to Antigen as well as Dendritic Cell-Mediated CD4 T Cell Responses.
J Immunol, 200(10):3383-3396, 11 Apr 2018
Cited by: 10 articles | PMID: 29643191
Defective CD8 Signaling Pathways Delay Rejection in Older Recipients.
Transplantation, 100(1):69-79, 01 Jan 2016
Cited by: 9 articles | PMID: 26356176
Go to all (22) article citations
Similar Articles
To arrive at the top five similar articles we use a word-weighted algorithm to compare words from the Title and Abstract of each citation.
Microchimerism, dendritic cell progenitors and transplantation tolerance.
Stem Cells, 13(6):622-639, 01 Nov 1995
Cited by: 111 articles | PMID: 8590864 | PMCID: PMC2963943
Review Free full text in Europe PMC
Preferential induction of Th1 responses by functionally mature hepatic (CD8alpha- and CD8alpha+) dendritic cells: association with conversion from liver transplant tolerance to acute rejection.
Transplantation, 69(12):2647-2657, 01 Jun 2000
Cited by: 41 articles | PMID: 10910289
Impact of Flt-3 ligand on donor-derived antigen presenting cells and alloimmune reactivity in heart graft recipients given adjuvant donor bone marrow.
Transpl Immunol, 6(4):225-234, 01 Dec 1998
Cited by: 1 article | PMID: 10342736
Dendritic cells,tolerance and therapy of organ allograft rejection.
Contrib Nephrol, 146:105-120, 01 Jan 2005
Cited by: 24 articles | PMID: 15567925
Review
Funding
Funders who supported this work.
NIAID NIH HHS (6)
Grant ID: U01 AI051698
Grant ID: R01 AI 67541
Grant ID: U01 AI 51698
Grant ID: R01 AI060994
Grant ID: R01 AI 60994
Grant ID: R01 AI067541
NIAMS NIH HHS (2)
Grant ID: F32 AR054225
Grant ID: F32 AR 054225