Abstract
Free full text

Monocyte recruitment during infection and inflammation
Abstract
Monocytes originate from progenitors in the bone marrow and traffic via the bloodstream to peripheral tissues. During both homeostasis and inflammation, circulating monocytes leave the bloodstream and migrate into tissues where, following conditioning by local growth factors, pro-inflammatory cytokines and microbial products, they differentiate into macrophage or dendritic cell populations. Recruitment of monocytes is essential for effective control and clearance of viral, bacterial, fungal and protozoal infections, but recruited monocytes also contribute to the pathogenesis of inflammatory and degenerative diseases. The mechanisms that control monocyte trafficking under homeostatic, infectious and inflammatory conditions are being unravelled and are the focus of this Review.
Monocytes are a subset of circulating white blood cells that can further differentiate into a range of tissue macrophages and dendritic cells (DCs)1. Bloodstream monocytes are derived from precursors in the bone marrow2 and are subdivided into subsets that differ in size, trafficking and innate immune receptor expression and in their ability to differentiate following stimulation with cytokines and/or microbial molecules1. Monocytes mediate host antimicrobial defence3 and are also implicated in many inflammatory diseases, including atherosclerosis4. In addition, monocytes can be recruited to tumour sites and can inhibit tumour-specific immune defence mechanisms5.
Studies of these different diseases have revealed the remarkable multipotency of monocytes in different inflammatory environments. The ability of monocytes to mobilize and traffic to where they are needed is central for their functions in promoting immune defence during infection and in driving inflammatory diseases. This Review focuses on the mechanisms that allow monocytes to traffic from their site of origin — the bone marrow — to distinct tissue sites. We discuss the mechanisms involved in homeostatic trafficking of monocytes and in their ‘patrolling’ functions, and compare these with the mechanisms that are used to recruit monocytes to inflamed tissues.
Monocyte subsets and chemokine receptors
Monocytes are divided into subsets on the basis of chemokine receptor expression and the presence of specific surface molecules6,7. In mice, expression of LY6C and CD11b identifies a monocyte subset that expresses high levels of CC-chemokine receptor 2 (CCR2) but low levels of CX3C-chemokine receptor 1 (CX3CR1). These monocytes — which are often referred to as inflammatory or LY6Chi monocytes — represent approximately 2–5% of circulating white blood cells in an uninfected mouse and are rapidly recruited to sites of infection and inflammation3. CCR2 deficiency markedly reduces LY6Chi monocyte trafficking to sites of inflammation, indicating a crucial role for this chemokine receptor in the trafficking of these monocytes8,9. CCR2 is also expressed by haematopoietic stem cells (HSCs) and a subset of natural killer (NK) cells, and CCR2-mediated recruitment of HSCs contributes to the generation of inflammatory monocyte populations during peritonitis and acetaminophen-induced hepatitis10.
A second major subset of circulating monocytes in mice expresses high levels of CX3CR1 and low levels of CCR2 and LY6C6,7. In the bloodstream, these LY6Clow monocytes (also referred to as CX3CR1hi monocytes) are less prevalent than LY6Chi monocytes, and intravital microscopy studies demonstrate that they adhere to and migrate along the luminal surface of endothelial cells that line small blood vessels in a process referred to as patrolling11 (FIG. 1).
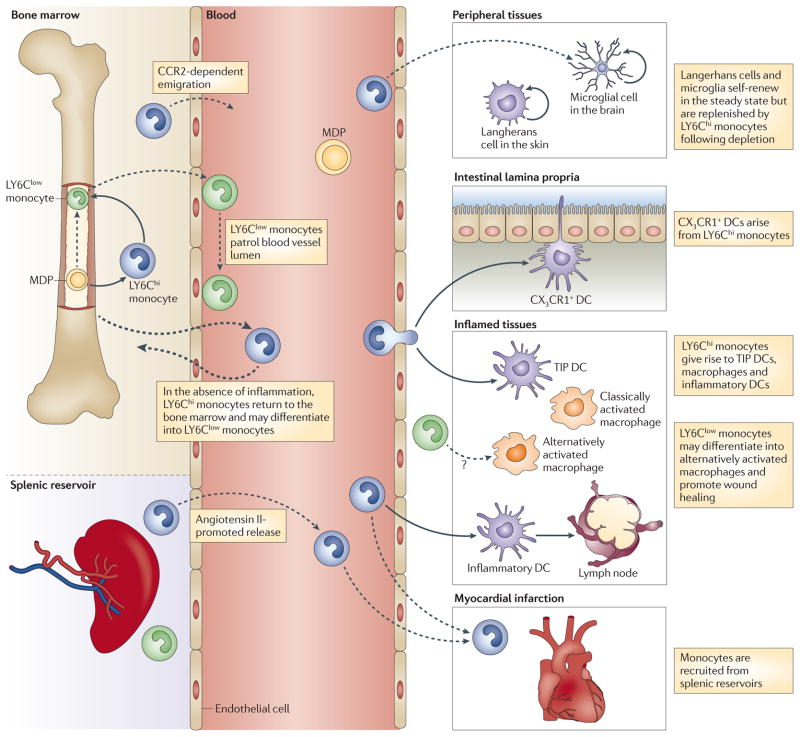
Macrophage and dendritic cell precursors (MDPs) in the bone marrow give rise to LY6Chi monocytes, which can serve as an intermediate for LY6Clow monocyte generation. LY6Chi monocytes exit the bone marrow in a CC-chemokine receptor 2 (CCR2)-dependent manner and are recruited to inflamed tissues. Here, they can differentiate into TNF- and iNOS-producing dendritic cells (TIP DCs), inflammatory macrophages or inflammatory DCs, some of which can subsequently migrate to draining lymph nodes. LY6Clow monocytes patrol the blood vessel lumen by associating with the vascular endothelium. LY6Clow monocytes are also recruited to sites of inflammation and possibly contribute to wound healing by differentiating into alternatively activated macrophages. Under certain experimental conditions, LY6Chi monocytes can give rise to Langerhans cells in the skin, microglia in the central nervous system, and CX3C-chemokine receptor 1 (CX3CR1)+ mononuclear phagocytes in the intestinal lamina propria, where they can sample the gut lumen by extending dendrites between intestinal epithelial cells. In the absence of inflammation, some circulating LY6Chi monocytes return to the bone marrow. The spleen functions as a reservoir for monocytes outside the bone marrow. Following myocardial infarction, monocytes are released from splenic reservoirs, and LY6Chi monocytes are selectively recruited to the injured heart.
Human monocytes are divided into subsets on the basis of surface CD14 and CD16 expression12. CD14++CD16− monocytes, which are also referred to as classical monocytes13, are the most prevalent monocyte subset in human blood and, similarly to mouse LY6Chi monocytes, these cells express CCR2 (REF. 7). The CD16+ monocyte population comprises two subsets, CD14+CD16++ and CD14++CD16+ monocytes; these are also referred to as non-classical and intermediate monocytes, respectively13. The CD14+CD16++ subset, in terms of in vivo patrolling, is similar to the mouse LY6Clow monocyte population14 (TABLE 1). Although the monocyte subsets identified in humans and mice are not precisely overlapping, their differentiation and contribution to immune defence appear to be similar15–17.
Table 1
Mouse and human monocyte subsets
Subset | Markers | Chemokine receptors | Functions |
---|---|---|---|
Mouse | |||
LY6Chi | CD11b+CD115+LY6Chi | CCR2hiCX3CR1low | Pro-inflammatory7 and antimicrobial3 roles |
LY6Clow | CD11b+CD115+LY6Clow | CX3CR1hiCCR2low | Patrolling11; early responses11; tissue repair128 |
Human | |||
Classical | CD14++CD16− | CCR2hiCX3CR1low | Resemble LY6Chi monocytes based on gene-expression arrays7,17,140 |
Intermediate | CD14++CD16+ | CX3CR1hiCCR2low | Pro-inflammatory roles12,15 |
Non-classical | CD14+CD16++ | CX3CR1hiCCR2low | Patrolling14; antiviral roles14 |
CCR2, CC-chemokine receptor 2; CX3CR1, CX3C-chemokine receptor 1.
Chemokines and monocyte trafficking
CCR2 and its ligands
CC–chemokine ligand 2 (CCL2; also known as MCP1) and CCL7 (also known as MCP3) are CC-chemokines that bind to CCR2 and mediate LY6Chi monocyte recruitment18. Although expression of CCR2 is restricted to only a few cell types, most (if not all) nucleated cells express CCL2 in response to activation by pro-inflammatory cytokines or stimulation of innate immune receptors by a range of microbial molecules18–22. Many infections induce the expression of CCL2 and result in high circulating levels of CCL2 in serum and within inflamed tissues.
Although CCL2 is important for monocyte recruitment in vivo, its mechanism of action remains unclear. One idea, which is based on the finding that CCL2 dimerizes and associates with tissue glycosaminoglycans, is that circulating CCL2 associates with glycosaminoglycans in specific tissues and establishes gradients that guide monocytes towards sites of infection or inflammation. In support of this model, monocyte recruitment is impaired by amino acid substitutions in CCL2 that prevent dimerization or association with glycosaminoglycans23,24. CCL2 has been suggested to circulate in the lymph and bind to high endothelial venules in lymph nodes, thereby guiding monocytes into the lymph nodes6. Other studies have demonstrated that the Duffy antigen receptor for chemokines (DARC) — a non-signalling chemokine receptor that binds CCL2 and transports it across endothelial cells to the luminal side — is important for the recruitment of monocytes from the bloodstream into inflamed tissues25.
CCL7 is also induced by bacterial infection and contributes to LY6Chi monocyte recruitment18,26. Deletion of either Ccl2 or Ccl7 diminishes the recruitment of monocytes during infection by approximately 40–50%26, although the specific contributions of CCL2 and CCL7 to the process of recruitment remain unclear. One possibility is that CCL2 and CCL7 act in series within tissues, guiding monocytes to sequential sites in the bone marrow before they enter the bloodstream. Alternatively, CCL2 and CCL7 may act in parallel, driving monocyte recruitment from different regions within the bone marrow or other tissues. CCL8 and CCL12 also bind to CCR2, but deletion of the genes encoding these chemokines does not have a detectable impact on monocyte trafficking under homeostatic conditions18.
The CX3CL1–CX3CR1 axis
LY6Clow monocytes respond to CX3C-chemokine ligand 1 (CX3CL1; also known as fractalkine), a membrane-bound chemokine that is expressed in tissues and the marginal zone of the spleen. Deletion of CX3CR1 results in diminished patrolling by LY6Clow monocytes11 and also decreases the recruitment of LY6Chi monocytes to splenic sites of bacterial infection27. CX3CL1 expression was detected in the marginal zone of the spleen during bacterial infection and, as had been previously proposed, CX3CL1 mediated very early recruitment of LY6Clow monocytes to the spleen27. In addition to their role in monocyte trafficking, CX3CL1 and CX3CR1 provide a survival signal to LY6Clow monocytes under both steady-state and inflammatory conditions28.
CCR1 and CCR5
Monocytes also express CCR1 and CCR5 (REFS 29,30), and these receptors bind to a variety of chemokines, including the shared ligands CCL3 (also known as MIP1α) and CCL5 (REF. 31). In vitro transmigration assays have shown that CCR1 predominantly mediates the arrest of monocytes in the presence of shear flow, CCR5 contributes to monocyte ‘spreading’, and both CCR1 and CCR5 support transendothelial chemotaxis towards CCL5; this suggests that the two receptors have specialized roles in monocyte recruitment32. In vivo studies using mice deficient in CCR1 or CCR5 have demonstrated non-redundant roles for these chemokine receptors in inflammatory cell recruitment33–38 with implications for the development and/or progression of atherosclerosis, multiple sclerosis and rheumatoid arthritis31. CCR5-mediated signals, for example, contribute to the pathogenesis of atherosclerosis39,40. The recruitment of LY6Chi monocytes to atherosclerotic plaques in apolipoprotein E-deficient (Apoe−/−) mice is mediated in part by CCR2, CCR5 and CX3CR1. Recruitment of LY6Clow monocytes is partially dependent on CCR5, which is upregulated in the LY6Clow monocyte population in Apoe−/− mice41. In multiple sclerosis, infiltrating monocytes express both CCR1 and CCR5 (REFS 42,43), and the ligands for these receptors are present in the affected tissues44,45. Mice deficient in CCR1 are more resistant to the development of experimental autoimmune encephalomyelitis (EAE), a mouse model of multiple sclerosis46. These findings suggest that CCR1 and CCR5 may contribute to the recruitment of monocytes into or within inflamed tissues. Determining the role of CCR1 and CCR5 in monocyte recruitment is complicated by the fact that these chemokine receptors, unlike CCR2, are expressed by a wide range of cell types47–50. Thus, alterations in monocyte recruitment in CCR1− or CCR5-deficient mice may be secondary to defective recruitment of other cell populations.
CCR6, CCR7, CCR8 and CXCR2
Though less studied, CCR6, CCR7, CCR8 and CXC-chemokine receptor 2 (CXCR2) have been suggested to be involved in monocyte recruitment. Circulating monocytes do not express significant levels of CCR6 (REF. 7) and do not respond to CCL20 (the ligand for CCR6) in vitro51. Therefore, it is unlikely that CCR6 mediates extravasation of circulating monocytes into tissues. However, transfer of CCR6+ (but not CCR6−) LY6Chi monocytes restored the functional defects in Ccr6−/− mice in a model of epithelial immunization52 and in a model of intradermal infection with Salmonella enterica53, suggesting a role for CCR6 in the migration or function of monocytes in inflamed tissues. Blood GR1mid monocytes express Ccr7 and Ccr8 mRNAs and give rise to DCs that subsequently use CCR7 and CCR8 to migrate from the skin to the lymph nodes54. CXCR2 mediates monocyte arrest that is induced by macrophage migration inhibitory factor (MIF)55.
Adhesion molecules in monocyte trafficking
Monocyte recruitment is thought to follow the general paradigm of leukocyte adhesion and trafficking, which involves rolling, adhesion and transmigration. The migration of leukocytes, including monocytes, depends on integrins and other adhesion molecules56. LY6Chi monocytes in mice express L-selectin (also known as CD62L), P-selectin glycoprotein ligand 1 (PSGL1), lymphocyte function-associated antigen 1 (LFA1; also known as αLβ2 integrin), macrophage receptor 1 (MAC1; also known as integrin αMβ2), platelet endothelial cell adhesion molecule (PECAM1) and very late antigen 4 (VLA4; also known as integrin α4β1), which all contribute to leukocyte adhesion and migration.
Monocyte recruitment during thioglycollate-induced peritonitis is impaired in mice deficient in L-selectin57. Using antibody-mediated blockade of adhesion molecules, it was shown that, in mice infected with Leishmania major, monocyte migration through inflamed dermal venules depends on two sets of interactions: PSGL1 expressed on monocytes interacts with P-selectin and E-selectin expressed on the venule endothelium; and monocyte-expressed L-selectin binds to endothelial cell-expressed peripheral node addressin (PNAd; a mixture of glycoprotein ligands for L-selectin)58. Monocyte migration through lymph node high endothelial venules also relies on L-selectin–PNAd interactions58. In the setting of atherosclerosis, P-selectin and E-selectin mediate the rolling of monocytes on inflamed aortic endothelium, whereas vascular cell adhesion molecule 1 (VCAM1) contributes to slow rolling and tight adhesion59. The involvement of intercellular adhesion molecule 1 (ICAM1), PECAM1, endothelial junctional proteins and other vascular adhesion molecules in the recruitment of monocytes to atherosclerotic aortas has been well covered in previous reviews60,61. Administration of antibodies that block MAC1 (REF. 62) or ICAM1 (REF. 63) also inhibits inflammatory monocyte recruitment during acute inflammation.
Patrolling by LY6Clow monocytes along endothelial cells in resting dermal blood vessels was shown to be mediated by the integrin LFA1 (REF. 11). By contrast, the early recruitment of LY6Chi monocytes is not affected by LFA1 deficiency64. These results suggest that the adhesion mechanisms used by distinct monocyte subsets may differ, depending on the type of tissue and the inflammatory state. A summary of molecules involved in monocyte recruitment, which is not meant to be exhaustive, is shown in TABLE 2.
Table 2
Molecules involved in monocyte trafficking
Monocyte-expressed molecules | Binding partners | Functions in monocyte recruitment |
---|---|---|
Chemokines and their receptors | ||
CCR2 | CCL2, CCL7 and CCL12 | Emigration of LY6Chi monocytes from the bone marrow18,26 |
CX3CR1 | CX3CL1 | Patrolling; recruitment to splenic sites of bacterial infection27 |
CCR1 and CCR5 | Various, including the shared ligands CCL3 and CCL5 | Recruitment into or within inflamed tissues41–43,125 |
CCR6 | CCL20 | Possible role in the migration or function of LY6Chi monocytes in inflamed tissues52,53 |
CCR7 and CCR8 | CCL19 and CCL1, respectively | Migration of monocyte-derived DCs from the skin to lymph nodes54 |
CXCR2 | MIF | Arrest in atherosclerotic arteries of mice55 |
Adhesion molecules | ||
L-selectin | Glycoproteins, including CD34, GLYCAM1 and MADCAM1 | Tethering and rolling56,57; recruitment during thioglycollate-induced peritonitis57; migration to lymph nodes58 |
PSGL1 | P-selectin and E-selectin | Migration through inflamed dermal venules58; tethering and rolling on atherosclerotic endothelium61 |
LFA1 | ICAM1 | Patrolling during the steady state; not involved in the early recruitment of inflammatory monocytes11 |
MAC1 | ICAM1 | Adhesion during acute inflammation62,63 |
VLA4 | VCAM1 | Adhesion to inflamed endothelium60,61 |
PECAM1 | Endothelial PECAM1 | Transendothelial migration141,142 |
CCL, CC-chemokine ligand; CCR, CC-chemokine receptor; CX3CL1, CX3C-chemokine ligand 1; CX3CR1, CX3C-chemokine receptor 1; CXCR2, CXC-chemokine receptor 2; DC, dendritic cell; GLYCAM1, glycosylation-dependent cell adhesion molecule 1; ICAM1, intercellular adhesion molecule 1; LFA1, lymphocyte function-associated antigen 1; MAC1, macrophage receptor 1; MADCAM1, mucosal addressin cell adhesion molecule 1; MIF, macrophage migration inhibitory factor; PECAM1, platelet endothelial cell adhesion molecule; PSGL1, P-selectin glycoprotein ligand 1; VCAM1, vascular cell adhesion molecule 1; VLA4, very late antigen 4.
Constitutive trafficking of monocytes
Adoptively transferred CX3CR1+ (LY6Clow) blood monocytes patrol blood vessels and enter non-inflamed tissues, whereas LY6Chi monocytes selectively traffic to sites of inflammation7. In the absence of inflammation, LY6Chi monocytes return to the bone marrow, where they are thought to differentiate into LY6Clow monocytes65,66 (FIG. 1). Under normal circumstances, peripheral tissues are replete with the progeny of circulating monocytes, and it is difficult, on an experimental level, to detect replenishment of tissue-resident macrophages and DCs by circulating monocytes. Depletion of resident cells by a variety of experimental approaches has been used to demonstrate that, under certain conditions, circulating monocytes can reconstitute Langerhans cell populations in the skin67, microglia in the brain68,69 and CX3CR1+ mononuclear phagocytes in the intestinal lamina propria70,71. It is unclear whether the loss of these cells in tissues enhances monocyte recruitment (for example, by inducing the expression of chemokines or the upregulation of adhesion molecules) or simply opens up a niche that monocytes occupy. It is important to note that monocyte-mediated replenishment of tissue-resident cell populations following their experimental depletion does not necessarily reflect the natural processes that occur during the development of different tissues or under homeostatic conditions. For example, in the steady state, Langerhans cells are maintained by local self-renewal rather than through replenishment by blood-borne cells72. Also, during embryonic development, microglia develop from primitive myeloid progenitors that are distinct from inflammatory monocytes73. Nevertheless, the ability of monocytes to reconstitute distinct tissue macrophage and DC populations suggests that this may be a physiologically important and relevant role for monocytes following tissue trauma or infection.
Macrophage and DC progenitors (MDPs) give rise to monocyte progenitors and also to common DC progenitors (CDPs)74. Monocyte progenitors express CD115 (also known as the M-CSF receptor) and develop in response to macrophage colony-stimulating factor (M-CSF), whereas CDP development is driven by FMS-related tyrosine kinase 3 ligand (FLT3L). LY6Chi monocytes traffic to the intestinal lamina propria by mechanisms that remain unclear and differentiate into CD11b+CD11c+ DCs that also express CX3CR1 (which have been referred to as CX3CR1+ mononuclear phagocytes)75, whereas CDPs give rise to intestinal CD103+ DCs70,71. Following the depletion of CD11chi DCs in mice, LY6Chi monocytes are recruited to the lamina propria and undergo extensive proliferation to ‘fill the space’70. The resulting CX3CR1+ mononuclear phagocytes extend dendrites between intestinal epithelial cells, a process that depends on CX3CR1, and enhance the uptake of bacteria into underlying tissues76. CX3CR1+ mononuclear phagocytes, however, do not traffic to draining lymph nodes and thus are not thought to directly mediate T cell priming. Instead, CD103+ DCs, which express high levels of CCR7, carry antigen into draining mesenteric lymph nodes, where they prime T cells71,77.
Careful kinetic studies of adoptively transferred monocytes have demonstrated that, in the lung, LY6Chi monocytes give rise to CD103+ DCs, whereas LY6Clow monocytes give rise to CD11b+ DCs78. However, it has also been suggested that CD103+ DCs in the lung can be derived from CDPs79. LY6Clow monocytes also give rise to alveolar macrophages after first differentiating into parenchymal macrophages in the lung80,81.
Monocyte recruitment to sites of infection
In addition to their role in replenishing tissue macrophages and DCs in the steady state, monocytes are recruited to infected tissues and mediate direct antimicrobial activity at these sites3. During infection, monocytes and/or monocyte-derived cells can also enter draining lymph nodes and promote adaptive immune responses (BOX 1). Studies with infectious disease models in mice are providing insights into the mechanisms that mobilize and target monocytes to sites of microbial infection. LY6Clow monocytes can be recruited to sites of infection even earlier than LY6Chi monocytes, and can participate in the initial inflammatory response by releasing tumour necrosis factor (TNF) and chemokines11. However, in most cases of infection, the ensuing recruitment of LY6Chi monocytes is more prominent and robust. Because few studies using infection models have included a comparative analysis on the recruitment of different monocyte subsets, the relative contributions of LY6Chi versus LY6Clow monocytes in these settings are not adequately defined. In the following sections, we review some of the major infectious disease models in which a role for CCR2-mediated monocyte recruitment has been identified (FIG. 2).
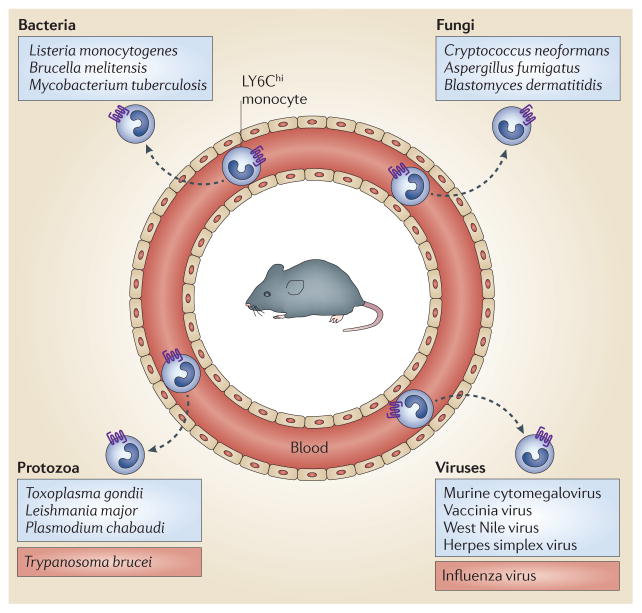
Infections with diverse pathogens, including bacteria, parasites, fungi and viruses, induce the recruitment of LY6Chi monocytes to sites of infection. For most pathogens (including those listed in the blue boxes), CC-chemokine receptor 2 (CCR2)-mediated monocyte recruitment enhances resistance to infection. However, during some infections (such as Trypanosoma brucei or influenza virus infection; listed in the red boxes), CCR2-mediated recruitment of monocytes is deleterious and enhances pathology.
Bacterial infections
Listeria monocytogenes is a facultative intracellular Gram-positive bacterium that infects mammals and, particularly in rabbits, induces monocytosis82. Inflammatory cell recruitment to the liver and spleen — two major sites of infection — is essential for early control of L. monocytogenes, and antibody-mediated blockade of CD11b reduces bacterial clearance and increases mortality83. CCR2 deficiency worsens infection with L. monocytogenes and reduces the recruitment of LY6Chi monocytes to infected tissues8,84. TNF and inducible nitric oxide synthase (iNOS) both contribute to innate defence against L. monocytogenes and are produced by TNF- and iNOS-producing (TIP) DCs, a cell population that is derived from LY6Chi monocytes that are recruited to the foci of infection84. LY6Chi monocytes also give rise to iNOS-producing cells following infection with Brucella melitensis85, Mycobacterium tuberculosis and various protozoa (see below). Infection with L. monocytogenes induces CCL2 and CCL7 expression, and these chemokines, at later stages of infection, can be detected in the serum, spleen, liver and lungs26. Monocyte recruitment following L. monocytogenes infection is reduced in mice lacking CCL2 or CCL7. Mice deficient in CCL2, CCL7 or CCR2 retain LY6Chi monocytes in the bone marrow, indicating that CCR2-mediated signals are principally required for LY6Chi monocyte recruitment from the bone marrow into the bloodstream86. Adoptive transfer of CCR2-deficient monocytes into L. monocytogenes-infected mice demonstrated that the trafficking of these monocytes to sites of infection in the spleen is normal86. Trafficking of LY6Chi monocytes to foci of L. monocytogenes infection in the liver is also CCR2 independent and predominantly mediated by CD11b, ICAM1 and CD44 (REF. 63).
Monocyte emigration from the bone marrow during the first 72 hours of L. monocytogenes infection can occur in the absence of myeloid differentiation primary response protein 88 (MYD88) and Toll-like receptor (TLR) signalling87. Monocyte emigration from the bone marrow is normal during early-stage infection of mice lacking either MYD88 or the type I interferon receptor (IFNAR); however, the combined loss of MYD88 and IFNAR results in a dramatic reduction in early monocyte recruitment88 (FIG. 3). Thus, the contributions of these two signalling pathways to the regulation of monocyte emigration from the bone marrow provide redundancy to ensure innate immune antimicrobial defence. On the other hand, monocytopoiesis and the replenishment of bone marrow monocytes are reduced in MYD88-deficient mice after the third day of infection with L. monocytogenes89.
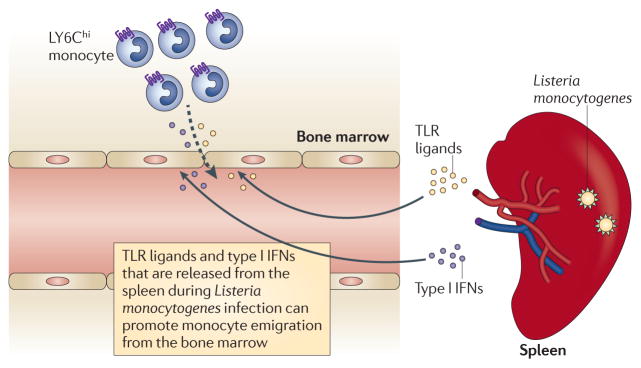
Monocyte emigration from the bone marrow during the early stage of infection with Listeria monocytogenes is normal in mice that lack either myeloid differentiation primary response protein 88 (MYD88; which is required for most responses to Toll-like receptor (TLR) ligands) or the type I interferon receptor (IFNAR). However, the combined loss of MYD88 and IFNAR impairs monocyte emigration from the bone marrow. IFN, interferon.
Recruitment of LY6Chi monocytes into the spleen during L. monocytogenes infection is mediated in part by CX3CR1 expression on monocytes and CX3CL1 expression in the marginal zone and T cell areas of the spleen27. Splenic DCs also coordinate monocyte and NK cell recruitment to the infected spleen by mechanisms that remain incompletely defined. Recruited NK cells, in proximity to recruited LY6Chi monocytes, produce interferon-γ (IFNγ) and thus drive monocyte differentiation into TIP DCs at the site of bacterial infection90.
M. tuberculosis is an inhaled, respiratory pathogen that infects macrophages and DCs91. Although CCR2-deficient mice respond normally to infection with a low-dose inoculum of M. tuberculosis92, CCR2-dependent monocyte recruitment protects mice following infection with a high-dose inoculum93. Monocyte CCR2 deficiency markedly reduces the expansion of F4/80lowCD11c+ cell populations in the lungs of infected mice, presumably because of diminished monocyte recruitment. T cell activation and recruitment in response to M. tuberculosis infection is defective in CCR2-deficient mice; however, loss of CCR2 expression on T cells does not interfere with the in vivo control of M. tuberculosis growth94. These results suggest that recruited inflammatory monocytes promote the activation of M. tuberculosis-specific T cells and/or their recruitment to the lung. In addition, recruited monocytes, following activation and differentiation in the lung, may directly defend against mycobacterial infection. LY6Chi monocytes are the major iNOS-producing cell population during pulmonary M. tuberculosis infection. LY6Chi monocytes isolated from the bone marrow and adoptively transferred into M. tuberculosis-infected mice traffic to the lung and differentiate into CD11chi DCs that express MHC class II and high levels of iNOS95. These cells are phenotypically similar to TIP DCs and require CCR2 to exit the bone marrow but not for trafficking into the infected lung.
Although monocytes provide protection against M. tuberculosis, a recent study using intranasal polyinosinic–polycytidylic acid (polyI:C) administration demonstrates that monocyte recruitment can also be deleterious96. M. tuberculosis-infected mice treated with polyI:C succumbed to infection and harboured higher numbers of mycobacteria in the lungs. Defective mycobacterial clearance in polyI:C-treated mice was dependent on type I IFN-mediated induction of CCL2, which triggered enhanced recruitment of monocytes. In this setting, monocyte recruitment impaired microbial clearance, in part because monocytes harboured live M. tuberculosis. Thus, although CCR2-dependent recruitment of monocytes is important for controlling M. tuberculosis infection, excessive recruitment of monocytes following polyI:C administration worsens the infection. It is possible that inflammatory monocytes differentiate abnormally in the presence of high levels of type I IFNs, rendering them incapable of killing M. tuberculosis.
Protozoal infections
Toxoplasma gondii is a protozoal pathogen that infects mammalian cells and causes severe infections in immunocompromised hosts. Experimental studies have demonstrated that diminished recruitment of LY6Chi monocytes renders CCR2− or CCL2-deficient mice more susceptible to T. gondii infection following peritoneal or oral inoculation97,98. By contrast, in vivo depletion of neutrophils, which are also recruited to the intestine, does not worsen infection99. LY6Chi monocytes are recruited to the lamina propria of the intestine, where they differentiate into major producers of iNOS and TNF. In CCR2− or CCL2-deficient mice, TNF and iNOS production are markedly reduced in intestinal villi because LY6Chi monocytes are retained in the bone marrow98. Adoptive transfer of LY6Chi monocytes to T. gondii-infected CCR2-deficient mice reduces mortality98. This study also demonstrated that, once monocytes have exited the bone marrow, their trafficking to the intestinal lamina propria during T. gondii infection is CCR2 independent98. It remains unclear how inflammatory monocytes are recruited to the lamina propria of the gut during T. gondii infection and how they mediate protection at this site. In contrast to the TIP DCs that are induced by L. monocytogenes infection84, the TNF- and iNOS-producing cells that differentiate from LY6Chi monocytes during T. gondii infection do not express CD11c, a result that may be attributable to distinct inflammatory responses to these two pathogens.
Inflammatory monocytes and DCs are recruited to dermal sites of infection and have been implicated in the induction of a T helper 1 (TH1)-type response to L. major infection100. In the skin, L. major multiplies within the vacuoles of phagocytic cells and induces an inflammatory response that includes the production of TNF and iNOS. A recent study demonstrated that TNF and iNOS are produced by CD11b+LY6C+CD11c+ DCs that are recruited to sites of infection in a CCR2-dependent manner101, although it is unclear whether CCR2 is involved in the step beyond bone marrow emigration. These recruited cells are probably derived from circulating LY6Chi monocytes and harbour most of the L. major parasites in cutaneous lesions and draining lymph nodes.
During infection of mice with Plasmodium chabaudi, which serves as a model for malaria, LY6Chi monocytes are recruited to the spleen, produce iNOS and phagocytose parasites. Adoptive transfer of LY6Chi monocytes protects otherwise susceptible Ccr2−/− mice during P. chabaudi infection, demonstrating the importance of monocytes for resistance to malaria infections102.
In contrast to the role of monocytes in L. major or P. chabaudi infections, CCR2-dependent recruitment of monocytes during Trypanosoma brucei infection is deleterious and enhances pathology. CCR2-deficient mice are more resistant to T. brucei infection and have decreased numbers of circulating parasites103. T. brucei infection induces CCR2-dependent monocyte emigration from the bone marrow and CCR2-independent trafficking into the liver, where monocytes undergo IFNγ- and MYD88-dependent differentiation into TIP DCs. During T. brucei infection, IL-10 administration reduces monocyte emigration from the bone marrow, potentially by preventing the induction of CCL2 expression104. In contrast to T. gondii, L. major and P. chabaudi, all of which are intracellular pathogens, T. brucei circulates in the plasma and remains extracellular during infection. Thus, differences in cellular localization may provide a partial explanation for the disparate effects of monocyte recruitment on host survival following infection with these pathogens.
Fungal infections
Yeasts and moulds are an important class of pathogens that cause infections in mammalian hosts. Cryptococcus neoformans is an environmental fungus that causes pulmonary infections on inhalation. Studies in CCR2-deficient mice have demonstrated that preventing the recruitment of monocytes alters the CD4+ T cell response from a predominantly TH1-type response to a TH2-type response, resulting in prolonged and more severe pulmonary infection and pathology105. CCR2 deficiency also resulted in decreased accumulation of CD11b+ DCs in lung-draining lymph nodes106 and decreased recruitment of LY6Chi monocytes to the lung during C. neoformans infection107. Thus, these studies suggest that LY6Chi monocytes are recruited in a CCR2-dependent manner and contribute to the accumulation of CD11b+ DCs in lung-draining lymph nodes during fungal infection.
Aspergillus fumigatus is an inhaled fungal pathogen that can cause invasive infections in immunocompromised patients108. A. fumigatus spores are common in the environment and are inhaled on a daily basis. In some individuals, A. fumigatus induces TH2 cell responses that exacerbate asthma and lead to allergic bronchopulmonary aspergillosis. The role of inflammatory monocytes in defence against A. fumigatus was recently investigated using a CCR2 depleter mouse strain, in which the Ccr2 promoter drives expression of the simian diphtheria toxin receptor109. In these mice, administration of diphtheria toxin rapidly but transiently depletes LY6Chi monocytes from the bone marrow, bloodstream and peripheral tissues. Depletion of monocytes at the time of pulmonary infection with A. fumigatus markedly decreases the delivery of fungal spores to draining lymph nodes and prevents priming of A. fumigatus-specific CD4+ T cells. Thus, LY6Chi monocytes — and potentially the CD11b+ pulmonary DCs that are derived from these cells — deliver spores from the lung to lymph nodes109. Although CD11b+ DCs are capable of activating naive T cells, it remains unclear whether inflammatory monocytes delivering A. fumigatus spores to the lung directly prime T cells or whether they transfer antigens to classical DCs in the lymph nodes. Depletion of monocytes at later stages of A. fumigatus infection does not impair the activation or proliferation of responding CD4+ T cells, but does impair TH1 cell development and promotes increased TH17 cell differentiation in the lung110. Thus, recruitment of monocytes to the lung during fungal infection influences the differentiation of responding CD4+ T cells.
Blastomyces dermatitidis is a fungus that causes pulmonary infections following inhalation of spores. A recent study of the immune response to an attenuated B. dermatitidis vaccine demonstrated that LY6Chi monocytes phagocytose this fungus at dermal sites of immunization and traffic to draining lymph nodes111. Analyses of vaccine antigens associated with DCs in lymph nodes support a model of antigen transfer from LY6Chi monocyte-derived DCs to classical DCs before T cell priming. Thus, in this model, monocyte-derived DCs do not directly induce naive T cells to upregulate activation markers or to proliferate, whereas classical DCs and migratory dermal DCs that express DEC205 potently activate naive antigen-specific T cells.
Viral infections
In mice infected with murine cytomegalovirus (MCMV), inflammatory monocytes are recruited to the liver and produce CCL3, which recruits NK cells112. Monocyte recruitment to the liver is CCR2 dependent but, as in other infectious diseases, CCR2 signalling is only required for monocyte emigration from the bone marrow. Although CCL2 is produced by hepatocytes during MCMV infection113, the induction of type I IFNs also drives expression of CCL2, CCL12 and, to a lesser extent, CCL7 in bone marrow cells. Surprisingly, F4/80+ monocytes produce CCL2 in the bone marrow, leading to the suggestion that these cells might promote their own entry into the bloodstream114.
MCMV and vaccinia virus induce type I IFN expression by LY6Chi monocytes115. This process requires TLR2 stimulation by viral ligands in endosomes of virusinfected cells. Following recruitment to sites of infection, inflammatory monocytes respond to distinct pathogen-derived ligands and differentiate along distinct pathways. In the case of MCMV and vaccinia virus infection, this response includes the induction of type I IFNs, in contrast to the response to bacterial and fungal infections.
LY6Chi monocytes are recruited to the brain and enhance viral clearance in mice infected with West Nile virus (WNV)116. Adoptive transfer of wild-type or CCR2-deficient monocytes into WNV-infected recipients showed that CCR2 mediates monocyte emigration from the bone marrow but not entry into the brain. CCR2-deficient mice also show delayed viral clearance and impaired T cell responses during herpes simplex virus infections. In this model, adoptive transfer of wild-type or CCR2-deficient monocytes showed that trafficking of monocytes from the blood into infected vaginal tissues is dependent on CCR2. Although this finding suggests that CCR2 is required for monocyte infiltration of virus-infected vaginal tissues, the possibility that transferred CCR2-deficient monocytes returned to and became trapped in the bone marrow was not excluded.
Monocytes appear to have both beneficial and detrimental roles during influenza virus infections. CCR2-deficient mice show impaired monocyte recruitment to the lungs during infection with influenza virus, and this is associated with decreased immunopathology and mortality117,118. On the other hand, CCR2-deficient mice also show delayed clearance of influenza virus as a result of diminished priming of virus-specific CD8+ T cells. Adoptive transfer of LY6Chi monocytes into CCR2-deficient mice restores CD8+ T cell responses and promotes clearance of the virus. Interestingly, activation of the nuclear receptor peroxisome proliferator-activated receptor-γ (PPARγ) with the drug pioglitazone led to decreased CCL2 expression and reduced recruitment of LY6Chi monocytes in vivo; these mice showed lower levels of mortality following influenza virus infection but were still able to effectively clear the infection118. This study suggests that monocyte recruitment can be pharmacologically manipulated during infection to minimize immunopathological tissue damage while maintaining microbial clearance.
Alveolar epithelial cells have been shown to produce CCL2 following infection with influenza virus in vitro, and this can facilitate monocyte trafficking across an epithelial cell monolayer119. However, the stage at which CCR2 and its chemokine ligands contribute to monocyte recruitment during respiratory viral infection in vivo remains unclear. It is possible that, in addition to mediating monocyte recruitment from the bone marrow to the bloodstream, CCR2 contributes to the direct recruitment of inflammatory monocytes from the bloodstream to the infected lungs, or from the bloodstream to lymph nodes that drain infected sites120.
Monocyte recruitment in sterile inflammation
Monocyte recruitment contributes to the pathogenesis of various aseptic inflammatory conditions. Studies in these disease models, particularly atherosclerosis4,59, provide insights into the mechanisms of monocyte recruitment in aseptic, and often chronic, inflammatory settings.
Monocytes have long been associated with atherosclerosis4,40. Monocytes are recruited to fatty streaks (the earliest visible atherosclerotic lesions, consisting of lipid deposits and infiltrating cells in the intima of arteries121), although the mechanisms of monocyte recruitment at this stage of disease remain undefined. During the progression of atherosclerosis, both LY6Chi and LY6Clow monocytes are recruited to atherosclerotic plaques. Monocytes initially undergo P-selectin- and E-selectin-dependent rolling and then use VCAM1 for firm adhesion61. LY6Chi monocytes are recruited to the lesions in a CCR2-, CCR5- and CX3CR1-dependent manner41,122,123, and they contribute to inflammation by differentiating into macrophages124. Combined inhibition of CCL2, CCR5 and CX3CR1 signalling abrogated monocyte recruitment and almost abolished atherosclerosis in Apoe−/− mice, which are predisposed to the disease125. LY6Clow monocytes infiltrate lesions to a lesser extent, and their recruitment has been less well characterized. They require CCR5, but not CX3CR1, for migration to lesions41. Although LY6Clow monocytes exhibit phagocytic and pro-angiogenic functions, and might differentiate into DCs in the lesion, their role in disease progression remains largely unknown126.
Myocardial infarction triggers an acute inflammatory response in patients, with an early peak of circulating inflammatory CD16− monocytes followed by an increase in circulating CD16+ monocytes, supporting a model of biphasic monocyte recruitment to the heart127. A similar temporal difference in the recruitment of LY6Chi and LY6Clow monocytes has been demonstrated in a mouse model of myocardial infarction128. These studies suggest that distinct subsets of monocytes are recruited at different stages of infarction, with the first wave of monocytes facilitating the removal of dead cardiac myocytes and the later phase promoting the resolution of inflammation and tissue repair129. A recent study demonstrated that monocyte recruitment to the myocardium following infarction begins with their release from a reservoir in the spleen130. In this setting, the vasoconstrictive hormone angiotensin II promotes the release of LY6Chi monocytes from the spleen, independently of CCR2 signalling. Whether angiotensin II-mediated release of LY6Chi monocytes from the spleen contributes to monocyte recruitment in other inflammatory settings is unclear.
From the bone marrow to the bloodstream
As discussed above, one of the key roles of CCR2 is in mediating monocyte egress from the bone marrow into the circulation. It has been shown that the increase in circulating monocytes that is associated with infection or sterile inflammation (such as that induced by high-fat diets) is mediated by CCL2 and CCL7 triggering CCR2 signalling in LY6Chi monocytes in the bone marrow18. However, how infections at peripheral sites can promote monocyte emigration from the bone marrow has remained a mystery.
Microbial infections induce granulocytosis, but the mechanisms that govern monocytosis and granulocytosis are distinct. Granulocytes are retained in the bone marrow by CXCL12-mediated CXCR4 signalling and exit in response to CXCR2 ligands, such as CXCL2 (also known as MIP2) and CXCL1 (also known as KC), which are produced during infection131,132. Although CCL2 and CCL7 enhance CCR2-mediated monocyte emigration from the bone marrow, it is unclear whether a retention mechanism is in place to retain LY6Chi monocytes in the bone marrow, as in the case of granulocytes. Inflammatory monocytes express CXCR4, but blocking CXCR4 under homeostatic conditions only minimally increases circulating monocyte frequencies (while markedly enhancing granulocyte frequencies)133. On the other hand, blockade of both CXCR4 and CCR2 does increase circulating monocyte frequencies, suggesting that CXCR4 may promote the return of monocytes from the bloodstream to the bone marrow133.
A recent study has provided new insights into the mechanisms controlling monocyte emigration from the bone marrow during infection134. During L. monocytogenes infection in mice, it was shown that CCL2 production by bone marrow stromal cells, but not by haematopoietic cells, could promote monocyte emigration into the bloodstream134. These bone marrow cells — which were identified as mesenchymal stem cells (MSCs)135 and CXCL12-abundant reticular cells (CAR cells)136 — express TLRs and respond to very low concentrations of TLR ligands by producing CCL2 (REF. 134). This provides a mechanism for peripheral infections to promote monocytosis (FIG. 4). Interestingly, in addition to TLR ligands, type I IFNs can promote the release of monocytes from the bone marrow during L. monocytogenes infection88. Thus, it is possible that bone marrow stromal cells can respond to either circulating microbial molecules or pro-inflammatory cytokines induced by infection. These results suggest that monocyte release from the bone marrow is a crucial defence mechanism that can be readily triggered.
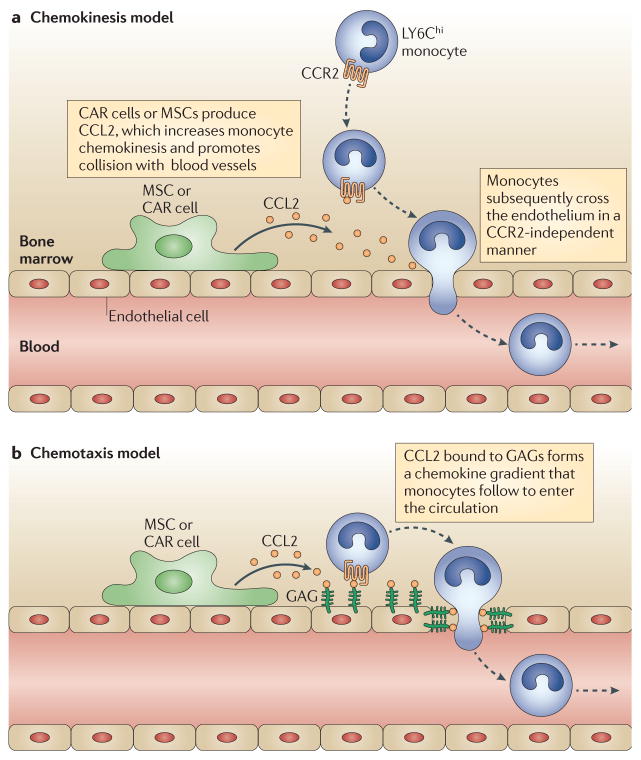
Release of LY6Chi monocytes from the bone marrow during Listeria monocytogenes infection is driven by CC-chemokine ligand 2 (CCL2). CCL2 is produced by mesenchymal stem cells (MSCs) and CXCL12-abundant reticular (CAR) cells following stimulation with circulating Toll-like receptor (TLR) ligands. Two models are proposed for the emigration of monocytes following CCL2 expression by these bone marrow stromal cells. In the first model, CCL2 production in the bone marrow increases monocyte chemokinesis, thereby increasing the likelihood that monocytes will come into contact with blood vessels. Subsequent movement of monocytes into the lumen may be independent of CC-chemokine receptor 2 (CCR2) (a). In the second model, CCL2 produced in proximity to vascular sinuses binds to glycosaminoglycans (GAGs) and forms a chemokine gradient that attracts monocytes to the abluminal aspect of the endothelium and then guides their transmigration into the circulation (b).
The cost of triggering monocyte release from the bone marrow is relatively low. The cells can enter the circulation and peruse the periphery, returning to the bone marrow in the absence of peripheral inflammation or entering sites of infection by adhering to the endothelial surface. How CCL2 production by local stromal cells induces monocytes to leave the bone marrow is unclear and requires further study. One possibility is that CCL2 production in proximity to vascular sinuses in the bone marrow attracts monocytes to the abluminal aspect of the endothelium and that subsequent movement to the lumen is automatic and independent of CCR2 or other chemokine-mediated signals. This scenario might explain the 80% reduction in homeostatic levels of monocyte emigration from the bone marrow that is seen in CCR2-deficient mice.
Such sensitive detection of circulating microbial molecules or pro-inflammatory cytokines by bone marrow stromal cells, with commensurate induction of CCL2, provides a mechanism to modulate the frequency of circulating inflammatory monocytes. Certain diseases, such as atherosclerosis, are associated with CCR2-mediated recruitment of inflammatory monocytes to the affected tissue site, and it is likely that circulating microbial molecules can, by modulating circulating monocyte frequencies, influence disease progression. It has been shown that bacterial cell wall fragments from the microbiota are present in the bloodstream and activate granulocytes, thereby enhancing their antimicrobial activity137. It is likely that these and other microbial molecules, such as those absorbed from the gastrointestinal tract or other mucosal tissues, can also stimulate the release of monocytes from the bone marrow by inducing CCL2 production from MSCs and CAR cells. On the one hand, this would enhance antimicrobial defence during infection but, on the other hand, it would also exacerbate inflammatory processes at distinct tissue sites in the absence of infection. Notably, this suggests a mechanism whereby microorganisms may drive inflammatory disease in an otherwise ‘sterile’ tissue environment.
Summary
Monocytes have an essential role in antimicrobial immune defence and promote tissue healing but, like a double-edged sword, they also contribute to tissue destruction during some infections and inflammatory diseases. Recent studies have furthered our understanding of monocyte heterogeneity and the mechanisms that mediate their trafficking. Infection with diverse pathogens (including bacteria, parasites, fungi and viruses) induces the recruitment of LY6Chi monocytes to sites of infection, where they restrict further microbial growth and invasion. Emigration of LY6Chi monocytes from the bone marrow is mediated by CCR2 and is modulated by bone marrow stromal cells, which sense circulating microbial molecules and produce CCL2. Although it has been shown that certain adhesion mechanisms target monocytes to sites of infection, much remains to be learnt about the trafficking of monocytes and the cues that direct them into different tissues once they emigrate from the bone marrow. Deciphering the process of monocyte recruitment may provide insights for the development of new therapeutics to manipulate the number and distribution of monocytes and to enhance antimicrobial immune defence or dampen detrimental inflammation.
Acknowledgments
This work was supported by US National Institutes of Health grants 5R37AI039031 and 5P01CA023766-31 (to E.G.P.).
Glossary
Polyinosinic–polycytidylic acid (polyI:C) | A substance that is used as a mimic of viral double-stranded RNA |
CXCL12-abundant reticular cells (CAR cells) | A type of stromal cell found in the bone marrow that expresses high levels of CXC-chemokine ligand 12. CAR cells can function as adipo-osteogenic progenitors, which are required for the proliferation of haematopoietic stem cells (HSCs), B cells and erythroid progenitor cells, and for maintaining HSCs in an undifferentiated state |
References
Full text links
Read article at publisher's site: https://doi.org/10.1038/nri3070
Read article for free, from open access legal sources, via Unpaywall:
https://www.ncbi.nlm.nih.gov/pmc/articles/PMC3947780
Citations & impact
Impact metrics
Article citations
Macrophage‑driven pathogenesis in acute lung injury/acute respiratory disease syndrome: Harnessing natural products for therapeutic interventions (Review).
Mol Med Rep, 31(1):16, 08 Nov 2024
Cited by: 0 articles | PMID: 39513609 | PMCID: PMC11551695
Review Free full text in Europe PMC
ZBTB18 regulates cytokine expression and affects microglia/macrophage recruitment and commitment in glioblastoma.
Commun Biol, 7(1):1472, 08 Nov 2024
Cited by: 0 articles | PMID: 39516530 | PMCID: PMC11549471
Single-cell RNA sequencing uncovers molecular mechanisms of intravenous immunoglobulin plus methylprednisolone in Kawasaki disease: attenuated monocyte-driven inflammation and improved NK cell cytotoxicity.
Front Immunol, 15:1455925, 25 Oct 2024
Cited by: 0 articles | PMID: 39524437
BLNK negatively regulates innate antifungal immunity through inhibiting c-Cbl-mediated macrophage migration.
Proc Natl Acad Sci U S A, 121(43):e2400920121, 16 Oct 2024
Cited by: 0 articles | PMID: 39413134 | PMCID: PMC11513953
Practical immunomodulatory landscape of glioblastoma multiforme (GBM) therapy.
J Egypt Natl Canc Inst, 36(1):33, 28 Oct 2024
Cited by: 0 articles | PMID: 39465481
Review
Go to all (1,680) article citations
Similar Articles
To arrive at the top five similar articles we use a word-weighted algorithm to compare words from the Title and Abstract of each citation.
Developmental and Functional Heterogeneity of Monocytes.
Immunity, 49(4):595-613, 01 Oct 2018
Cited by: 430 articles | PMID: 30332628
Review
Monocyte-mediated defense against microbial pathogens.
Annu Rev Immunol, 26:421-452, 01 Jan 2008
Cited by: 722 articles | PMID: 18303997 | PMCID: PMC2921669
Review Free full text in Europe PMC
Blood monocytes: development, heterogeneity, and relationship with dendritic cells.
Annu Rev Immunol, 27:669-692, 01 Jan 2009
Cited by: 980 articles | PMID: 19132917
Review
The Immune Function of Ly6Chi Inflammatory Monocytes During Infection and Inflammation.
Curr Mol Med, 17(1):4-12, 01 Jan 2017
Cited by: 7 articles | PMID: 28231755
Review
Funding
Funders who supported this work.
NCI NIH HHS (2)
Grant ID: 5P01CA023766‑31
Grant ID: P01 CA023766
NIAID NIH HHS (2)
Grant ID: R37 AI039031
Grant ID: 5R37AI039031